Abstract
The worldwide emergence of epidemic strains of Clostridium difficile linked to increased disease severity and mortality has resulted in greater research efforts toward determining the virulence factors and pathogenesis mechanisms used by this organism to cause disease. C. difficile is an opportunist pathogen that employs many factors to infect and damage the host, often with devastating consequences. This review will focus on the role of the 2 major virulence factors, toxin A (TcdA) and toxin B (TcdB), as well as the role of other putative virulence factors, such as binary toxin, in C. difficile-mediated infection. Consideration is given to the importance of spores in both the initiation of disease and disease recurrence and also to the role that surface proteins play in host interactions.
Abbreviations
C. difficile | = | Clostridium difficile |
TcdA | = | toxin A |
TcdB | = | toxin B |
AAD | = | antibiotic associated diarrhea |
CDI | = | C. difficile infection |
PCR | = | polymerase chain reaction |
REA | = | restriction endonuclease analysis |
PFGE | = | pulsed field gel electrophoresis |
PaLoc | = | pathogenicity locus |
ECF | = | extracytoplasmic function |
CDT | = | Clostridium difficile transferase |
ι-toxin | = | iota toxin |
CST | = | Clostridium spiroforme toxin |
CDTa | = | CDT enzymatic component |
CDTb | = | CDT binding/translocation component |
CDTLoc | = | CDT locus |
LSR | = | lipolysis-stimulated lipoprotein receptor |
S-layer | = | surface layer |
CWPs | = | cell wall protein |
SLPs | = | S-layer proteins |
HMW | = | high molecular weight |
LMW | = | low molecular weight |
Introduction
The notoriety of Clostridium difficile as an infectious agent has escalated in recent times because of increased disease incidence, severity and mortality rates. It is the leading cause of nosocomial antibiotic associated diarrhea (AAD) worldwide, costing in excess of US 3.2 billion dollars per annum for patient treatment in the USA alone.Citation1 This Gram-positive anaerobe causes a spectrum of diseases known collectively as C. difficile infections (CDI), which range in severity from mild, self-limiting diarrhea to pseudomembranous colitis and toxic megacolon, the most severe form of disease, which often results in death. C. difficile primarily causes nosocomial infections but is increasingly being recognized as a community acquired pathogen and it is also becoming more prevalent in a younger patient demographic.Citation2
C. difficile is a genetically heterogeneous species and efficient typing of strains is critical for epidemiological analysis. The major typing methods used to distinguish between C. difficile strains include PCR ribotyping, restriction endonuclease analysis (REA) and pulsed field gel electrophoresis (PFGE).Citation3 Use of these typing methods revealed that defined strains of C. difficile emerged and predominated over the past decade, resulting in epidemics in many countries. The strains causing these epidemics included those of REA type BI/PFGE type NAP1/ribotype 027 (BI/NAP1/027) in humans and those of ribotype 078 in animals.Citation4,5 Many investigations have been performed to determine which factors are responsible for the emergence and dissemination of these strain types. One of the main contributors, primarily in the BI/NAP1/027 isolates, is likely to be an increase in antibiotic resistance, particularly to fluoroquinolones.Citation6 Fluoroquinolone resistance is likely to have arisen in the 1990s in C. difficile as a result of the heavy use of this class of antibiotics, which is used to treat a range of infections. Other factors which have been suggested to contribute to the increased virulence of these strains include increased TcdA and TcdB production and the expression of a third toxin, binary toxin.Citation4,7 However, the precise factors resulting in the increased virulence of these strains still remain largely speculative.
In a healthy individual, the human microbiome consists of trillions of microorganisms working in synergy with the host. One of the benefits of this interaction is that the host is afforded protection from pathogens. C. difficile gains a foothold in the host when the gut microbiota is disturbed, usually as a result of antibiotic therapy that is administered for an unrelated condition although this does not always appear to be the case in community acquired CDI.Citation8 After entering the host in the spore form C. difficile passes through the stomach into the small intestine, where it begins the germination process, eventually transitioning into the metabolically active vegetative cell form. Colonisation and proliferation, which are likely to involve numerous surface structures and adhesins, predominantly occurs in the suitably anaerobic environment of the descending colon. Toxin production then occurs, leading to host tissue damage and disease. In this review, we present the current understanding of the major virulence factors of C. difficile, TcdA and TcdB, and other putative virulence factors which contribute to the disease-causing capacity of this gastrointestinal pathogen. We frame this discussion in the context of the recent epidemic strains when possible.
The Large Glucosylating Toxins, TcdA and TcdB
Once vegetative cell growth has been established the major virulence factors produced by C. difficile are the exotoxins TcdA and TcdB.Citation9 Clinical strains that do not produce at least one of these toxins are largely avirulent in animal modelsCitation10. The genes encoding both toxins are located within a 19.6 Kb region of the chromosome known as the pathogenicity locus or PaLoc.Citation11 In addition to the structural toxin genes, tcdA and tcdB, PaLoc also encodes 3 accessory proteins known as TcdR, TcdC and TcdE, which are thought to be involved in the regulation of toxin production Citation12,13 or in the extracellular export of the toxins.Citation14 TcdR is an extracytoplasmic function (ECF) alternative sigma factor that plays a critical role in up-regulating the expression of tcdA and tcdB as well as the other PaLoc genes, including tcdR.Citation12 Experimental evidence suggests that TcdC is an anti-sigma factor, which modulates the expression of tcdA and tcdB by blocking the association of TcdR and the core RNA polymerase.Citation13,15 The role of TcdC is, however, controversial with numerous studies providing conflicting data regarding the involvement of TcdC in the regulation of toxin production and the resulting virulence capacity of C. difficile.Citation13,16,17 TcdE appears to be a holin family protein which may be involved in the export of TcdA and TcdB from the cell. However, the role of this protein is also controversial, with 2 recent studies presenting conflicting data on the capacity of TcdE to facilitate TcdA and TcdB export from C. difficile.Citation14,18 Finally, the C. difficle PaLoc region from a toxigenic strain was recently shown to be mobilised to non-toxigenic isolates of this bacterium, lending support to the hypothesis that non-toxigenic strains can become toxigenic through horizontal gene transfer events.Citation19
TcdA and TcdB have approximately 66% similarity at the amino acid level, which suggests that the toxins might have arisen through a gene duplication event.Citation20 The toxins share multiple conserved domains including a catalytic glucosyltransferase domain located at the N-terminus and a cysteine protease domain which is required for auto-processing and delivery of the catalytic domain to the cytosol of the intoxicated cell. Additionally, a hydrophobic translocation domain, located centrally within the toxins, is thought to be involved in pore formation and insertion of the toxins into the endosomal membrane prior to their release into the cytosol. Finally, a C-terminal receptor binding domain, consisting of a series of repeating oligopeptides, is thought to bind to as yet unidentified receptors on target cells ()Citation21,22
Figure 1. The C. difficile TcdA and TcdB cellular intoxication process. (A) The domain structure of TcdB. The N-terminal catalytic domain is shown in red, the cysteine protease domain in blue, the central hydrophobic domain is shown in yellow, with the pore forming region (PFR) highlighted, finally the C-terminal receptor binding domain is colored green. The amino acid residue number defining the boundary of each domain is also indicated. (B) The cellular intoxication process mediated by TcdA and TcdB of C. difficile. The initial steps involve binding of TcdA (ToxA) or TcdB (ToxB) to unknown cellular receptors followed by endocytosis of the toxins into a cellular endosome. Acidification of the endosome then results in the insertion of the toxin into the endosomal membrane and the formation of a pore which, following an inositol hexakisphosphate (InsP6) dependent and cysteine protease domain mediated autocatalytic cleavage event, facilitates the release of the active glucosyltransferase domain into the cytosol. This results in glucosylation and inactivation of Rho family GTPases and the onset of pathogenic effects. © 2011 Nature Publishing Group. Reproduced by permission of Nature Publishing Group. Permission to reuse must be obtained from the rightsholder.Citation22
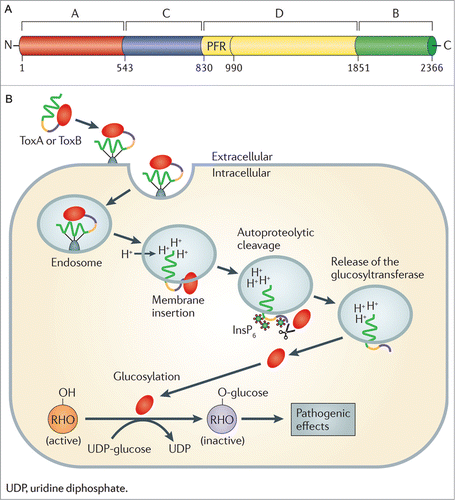
Intoxication of host cells with TcdA or TcdB is proposed to occur via an ABCD model (A, biological activity; B, binding; C, cutting; D, delivery) ().Citation21,22 In this model, the initial steps in the intoxication process are receptor recognition and binding by the C-terminal receptor binding domain of the toxins. This binding then triggers endocytosis of the toxins through an as yet uncharacterised clathrin- and dynamin-dependent pathway,Citation23 which results in trafficking of the toxins to early endosomes within the host cell. Acidification of the endosomal compartment then induces a conformational change in the toxins, leading to the formation of a protrusion,Citation24 which is thought to be involved in membrane insertion and the subsequent formation of a pore within the endosomal membrane. The toxins then bind to the host cell-derived molecule inositol hexakisphosphate,Citation25 which induces a conformational change within the cysteine protease domain and leads to autocatalytic cleavage at a site located between the cysteine protease and the glucosyltransferase domains.Citation26 This cleavage event results in the release of the active N-terminal glucosyltransferase domain of the toxin into the host cell cytosol.
Following their release into the cytosol, the N-terminal regions of TcdA and TcdB inactivate a number of cellular proteins belonging to the Rho family of small GTPases including RhoA, B, C, G, Rac1–3 and Cdc42.Citation27 TcdA and TcdB are both monoglucosyltransferases and inactivate their target Rho family GTPases through the covalent transfer of a glucose moiety to these proteins.Citation21 This toxin-mediated glucosylation locks the Rho family GTPases in an inactive conformation, which then affects a number of important downstream cellular pathways. Notably, intoxication of cells with TcdA and TcdB results in a loss of structural integrity due to the dysregulation of actin depolymerisation and an associated decline in the cellular levels of F-actin,Citation9 resulting in a characteristic “cell rounding” phenotype that normally precedes caspase-3 and caspase-9 mediated cellular apoptosis.Citation28 Colonocyte death then presumably leads to a disruption of cellular tight junctions within the intestinal epithelium and a concomitant loss of epithelial barrier function. Collectively, these effects result in increased intestinal permeability and fluid accumulation which subsequently manifests as diarrhea, a hallmark symptom of CDI.Citation9
The C. difficile toxins are known to activate the inflammasomeCitation29 and TLR4 and TLR5 signaling pathways,Citation30 resulting in the production of a variety of chemokines and cytokines and the recruitment of innate immune cells including neutrophils and mast cells to the site of infection. The toxins also activate enteric and sensory neurons within the intestinal lamina propria.Citation31 The subsequent production of multiple proinflammatory cytokines such as IL-12, IL-18, IL-1β, interferon γ and tumor necrosis factor α, as well as neuropeptides including neurotensin, substance P and corticotropin-releasing hormone, results in the onset of a profound inflammatory response.Citation30 This response is likely to play an important role in the severity of host tissue damage that occurs during infection. The toxin-mediated proinflammatory response is also another important characteristic of CDI.
The last decade has seen the emergence of epidemic, BI/NAP1/027 C. difficile isolates which are associated with higher rates of morbidity and mortality. Although the mechanisms responsible for increased virulence are not known precisely, it is likely that the major toxins produced by these strains play an important role. In addition, BI/NAP1/027 isolates produce a variant TcdB protein, which has been shown to be more cytotoxic and to display a broader tissue tropism than TcdB produced by historical strains.Citation32,33 These phenotypic differences may allow the variant toxin to inflict more severe and widespread damage to the host following infection with these particular C. difficile strains.
For many years TcdA was presumed to be the major virulence factor of C. difficile, a hypothesis that was based on studies using purified toxins. These studies showed that the intragastric administration of TcdA to hamsters resulted in CDI symptoms including intestinal inflammation, diarrhea and eventually death.Citation34 By contrast, no symptoms of disease were observed in hamsters following the administration of TcdB alone unless prior intestinal damage was present or sub-lethal concentrations of TcdA were co-administered.Citation34 The observation that TcdA could potentiate the activity of TcdB suggested that the toxins might act synergistically, with TcdB-mediated effects on the host occurring only once TcdA had caused prior damage to the intestinal epithelium. Another study supported these conclusions by showing that the addition of purified TcdA to rabbit ileal loops resulted in hemorrhage, cellular necrosis and an increase in intestinal permeability, whereas the addition of TcdB did not.Citation35 The importance of TcdA in CDI was also implied by the observation that vaccination with TcdA–specific toxoids fully protected animals against infection with toxigenic strains of C. difficile.Citation36 Moreover a strong humoral response against TcdA was known to be an important indicator of reduced CDI severity and relapse in human patients.Citation37
The importance of TcdA in CDI was questioned following the emergence of C. difficile isolates that were found to produce TcdB but not TcdA.Citation38 These isolates caused the full clinical spectrum of CDI with some studies suggesting that these strains caused more severe disease than strains producing both TcdA and TcdB.Citation38 By contrast to the studies which used purified toxins, the isolation of these variant strains suggested that TcdA was not essential for CDI onset and that strains producing only TcdB were equally as virulent as strains producing both toxins in human patients. Therefore, these studies suggested that TcdB plays a more important role in disease than the earlier work indicated. Further support for this hypothesis was gained when purified TcdB was found to induce an acute inflammatory response and to cause more severe damage than TcdA to the intestinal epithelium of mice carrying human intestinal explants.Citation39 In this model, TcdB was capable of eliciting the hallmark features of CDI in the absence of TcdA. Experiments in a zebrafish embryo model of intoxication also showed the capacity of TcdB to cause damage to the host without TcdA since exposure of these animals to purified TcdB resulted in systemic tissue damage, particularly to the heart.Citation40 These results clearly showed that TcdB was responsible for causing systemic damage in the zebrafish in addition to the damage observed in the gut. In support of the hypothesis that TcdB can cause systemic effects in the host during CDI is the finding that systemic damage was detected in piglets infected with C. difficile, which could be neutralised by the addition of anti-TcdB, but not anti-TcdA, antibodies.Citation41
More recently, isogenic toxin gene mutants have been constructed and used in infection studies to dissect the individual roles of TcdA and TcdB in disease. Two studies have utilized derivatives of C. difficile strain 630, a low toxin producing clinical isolate, for this work.Citation42,43 In the first study TcdB was found to be essential for disease, with approximately 80% of hamsters infected with independent tcdB mutants (which continued to produce TcdA) surviving disease.Citation42 By contrast, 90% and 94% of animals infected with the wild type strain or independent tcdA mutants (which produced TcdB) succumbed to infection. These data suggested, for the first time, that TcdB was the major virulence factor of C. difficile, not TcdA. The second study supported these results by showing that a tcdA mutant (which produced only TcdB) was able to cause fulminant disease.Citation43 However, in contrast to the earlier study, infection with a tcdB mutant (which produced only TcdA) also resulted in the death of all hamsters following infection, although the animals took longer to succumb to infection compared to the wild type or tcdA-infected animals.Citation43 In this study, all animals challenged with a double toxin gene mutant that did not produce TcdA or TcdB survived infection.Citation43 More recently, a third study using isogenic mutants constructed in strain R20291, an epidemic BI/NAP1/027, has provided further support for the importance of TcdB in disease. In this study, both tcdA and tcdB mutants caused the death of all hamsters following infection, as did challenge with the wild type strain. However, there was a significant delay in time to death following infection with the tcdB mutant in comparison to both the wild type strain and the tcdA mutant while the majority of hamsters infected with the double tcdA and tcdB mutant survived the challenge.Citation44 Overall, the 3 hamster infection studies using the toxin gene mutants suggest that TcdB plays a more important role in disease than suggested by the results obtained from work involving purified toxins.
Although the exact roles of TcdA and TcdB in CDI require further clarification, it is clear that TcdB is a more important virulence factor than early experimental data suggested. This contention is supported by the continued isolation of variant strains that produce TcdB but not TcdA. For these reasons, TcdB is an important target in the future development of C. difficile therapeutics.
Given that CDI is a toxin-mediated disease, several non-antibiotic therapeutic strategies have been developed which target the toxins. Passive immunization strategies have focused on the use of toxin-specific antibodies, including humanised monoclonal antibodies,Citation45 alpacaCitation41 and ovine-derived antibodies,Citation46 as well as bovine hyperimmune colostrum.Citation47 In addition, a number of active vaccination strategies targeting the toxins are under development. These include the use of inactivated toxoids,Citation48 DNA vaccines,Citation49 Bacillus subtilis spore-basedCitation50 and adenovirus virus-basedCitation51 vaccine platforms, as well as recombinant chimeric toxin vaccines that contain both TcdA- and TcdB-specific domains.Citation52,53 Although many of these products have shown promise in laboratory-based experiments, further work is required to determine if they will be efficacious in the treatment and prevention of CDI in human patients.
Binary Toxin (CDT)
Clostridium difficile transferase (CDT), otherwise known as binary toxin, is a virulence-associated determinant whose exact role in disease pathogenesis remains undefined. However, recent studies have shed some light on the possible role that binary toxin plays with respect to adherence and colonisation of C. difficile in the host.Citation54,55 This toxin is interesting because of its prevalence in C. difficile strains commonly associated with severe CDI, such as B1/NAP1/027 and ribotype 078 isolates, as well as other variant ribotypes found to cause disease in hospitalised patients, particularly over the past decade.Citation56,57 CDT was first described in 1988 Citation58 and belongs to the binary ADP-ribosylating toxin family, specifically in the iota-like subfamily of binary toxins which includes C. perfringens iota toxin (ι-toxin) and C. spiroforme toxin (CST).Citation59 As with all binary toxins, CDT comprises 2 components; the enzymatic component (CDTa) that confers ADP ribosyltransferase activity and the binding/translocation component (CDTb) that enables passage of the enzymatic component to the cell cytosol.Citation60 In strains producing CDT the genes encoding CDTa and CDTb, cdtA and cdtB, respectively,Citation60 are located on a 6.2 kb genomic region referred to as the CDT locus, or CDTLoc, which also encodes a positive regulator of CDT production, CdtR.Citation61
The mechanism of action of CDT is suggested to mimic that of iota toxin. Upon activation by serine-like proteasesCitation62 the monomeric form of CDTb binds to lipolysis-stimulated lipoprotein receptor, or LSR,Citation63,74 a cell surface receptor found in many tissues including the liver and gut.Citation64 Once bound to LSR, CDTb is thought to oligomerise within lipid rafts, prompting binding of the enzymatic component, CDTa.Citation65 This complex is then internalised into the acidic endosomal compartment, triggering membrane insertion of CDTb which is then followed by pore formation that enables the translocation of CDTa, the enzymatic component, to the cytosol.Citation66,74 Once in the cytosol CDTa ribosylates actin, a multi-functional protein involved in many cellular processes including muscle contractility, cell signaling and motility. Actin is also a major constituent of the cell cytoskeleton, maintaining cellular integrity. Toxin-induced ribosylation of actin at arginine 177Citation67 has a dual effect wherein G-actin polymerization is inhibited and F-actin depolymerisation is favored, resulting in the complete destruction of the actin cytoskeleton and, ultimately, cell death.Citation68
Despite understanding the mechanism of action of CDT on intoxicated cells, the role of this toxin in disease pathogenesis is not clear. Recent work has shown that the application of purified CDT to colonic epithelial cells had a dramatic effect, with a compact network of protrusions, comprised of microtubules, seen to develop on the cell surface ().Citation54 A similar result was seen on colonic epithelial cells upon the application of other ADP ribosylating toxins from the same family.Citation54 Using a combination of electron microscopy and adherence assays, these protrusions were shown to significantly enhance bacterial adherence to epithelial cells. Moreover, these microtubules were recently found to translocate fibronectin-containing vesicles and endoplasmic reticulum from the basolateral to the apical membrane side of Caco-2 cells in what appears to be both a microtubule- and Stim-1(calcium sensor)–dependent manner.Citation55 This study suggests a possible mechanism for C. difficile adherence to host cells whereby ECM components, like fibronectin, which are known to be involved in bacterial adherence, are secreted in response to CDT intoxication to increase the adherence capacity of C. difficile to host cells.
Figure 2. CDT-induced disruption of the actin cytoskeleton of Caco-2 cells and the development of cellular protrusions comprised of microtubules. CDT-induced disruption of cells and development of microtubules using 20 ng/ml CDTa and 40 ng/ml CDTb are shown to increase over time (1 hr–2 hrs). TRITC-conjugated phalloidin (red) was used to stain for actin; α-tubulin (using indirect immunofluorescence) is shown in green and cell nuclei (blue) were stained using DAPI. Dotted line delineates the cell border; a magnified view of the protrusions and cytoskeletal disruption is shown in the lower, middle panel. Scale bar denotes 20 μm. © Copyright Clearance Centre. Reproduced by permission of American Society of Microbiology. Permission to reuse must be obtained from the rightsholder. Citation54
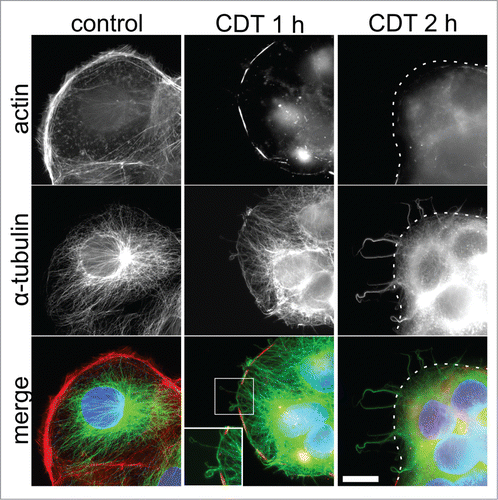
Results from in vivo studies using mice pre-treated with a CDT-neutralizing anti-iota toxin antibody or with a serum control and then infected with a CDT-producing ribotype 027 strain of C. difficile support the hypothesis that CDT acts as a colonization factor.Citation54 In these experiments, infected mice receiving the control serum showed typical signs of CDI including diarrhea and colitis 24 h post infection; these symptoms were not observed in mice pretreated with the neutralizing antibody. Immunofluorescent staining of colonic and cecal tissues from the infected animals using a C. difficile antiserum showed reduced bacterial numbers in mice pretreated with the CDT-neutralizing serum.Citation54 Hamster infection studies using clinical isolates that produce CDT but not TcdA and TcdB suggest that CDT alone may be unable to cause CDI but may work synergistically with the major toxins to enhance disease.Citation10 Infection experiments using isogenic toxin gene mutants go some way toward supporting this hypothesis since a TcdA+TcdB−CDT+ strain appeared to be more virulent in hamsters than an isogenic TcdA+TcdB−CDT− derivative, suggesting that CDT may work in synergy with TcdA.Citation44 This study also suggested that CDT alone may contribute to strain virulence since 33% (3 out of 9) of hamsters infected with a TcdA−TcdB−CDT+ derivative succumbed to disease. However, these animals did not display the typical cecal damage and diarrhea seen in hamsters infected with wild type toxigenic strains and the majority of animals (6 out of 9) infected with the TcdA−TcdB−CDT+ derivative did not exhibit any disease symptoms.Citation44 For this reason, additional infection studies are required to statistically validate these results.
Numerous studies have also suggested a correlation between CDT positive strains, including variant ribotypes, and increased mortality rates in CDI patients.Citation69,70 CDT positive isolates are also often associated with community-acquired cases of CDI that require patient hospitalisation.Citation71 Moreover, up to 100% of all animal isolates have been shown to encode CDT.Citation72,74 One of the most prevalent animal ribotypes is the 078 group of strains which has also been linked with C. difficile outbreaks in humans.Citation5 The close association of CDT with animal strains, and the prevalence of these strains in food animals, suggests that human outbreaks of CDT-encoding strains may originate from animals or food. Although epidemiological data supports this contention, foodborne transmission of C. difficile has yet to be confirmed.Citation73
Finally, the detection of CDT in patient clinical samples is currently limited since no reliable diagnostic assays are available. For this reason, the true impact of CDT on CDI morbidity and mortality is probably underestimated.Citation74
The endospore
C. difficile produces spores under stress conditions, which are a dormant form of the bacterium. Spores are the infectious particle and are critical for both C. difficile-mediated infection and transmission. They are found ubiquitously in the environmentCitation75 and are shed in the faeces of both humans and animals leading to infections in hospitalized patients, the community and animals when susceptibility to infection has been induced.Citation75 Spores are difficult to destroy as they are able to resist heat, radiation and many of the chemical and alcohol based disinfectants used to clean both hospital and community facilities.Citation76,77 Persistence of these infectious particles has been well noted, with spores found to exist on hospital surfaces for extended periods of time.Citation78
Following disruption of the normal microbiota, most commonly as a result of antibiotic treatment, infection and colonisation with C. difficile occurs. Sporulation can then ensue in the gut, enabling a sub-population of C. difficile to persist for prolonged periods of time and facilitating transmission of the organism even though it may encounter a hostile aerobic environment. In addition, even in the absence of antibiotics, mice have been shown to shed spores at a low level.Citation79 There is some debate regarding the association between C. difficile strain ribotypes and the production or germination efficiency of spores. Several studies noted that some clinical C. difficile isolates, especially those of the BI/NAP1/027 type, formed spores with greater proficiency than historical isolates, however, other studies reported no such association.Citation80
The ability of spores to persist in the environment is attributed to their structure with each spore containing a complete copy of the genome encased in a protective spore core. The core is surrounded by a thick coat of layered peptidoglycan Citation81,82 and a loose outer layer, termed the exosporium ().Citation83 This outermost layer, found in spores of B. cereus group speciesCitation84 as well as a number of clostridial species, including C. botulinum, C. sordellii and C. difficile,Citation83,85 is the first point of contact between the pathogen and its environment. Characterization of the exosporium in C. difficile has identified collagen-like proteins homologous to exosporial proteins from Bacillus speciesCitation83 as well as a cysteine-rich protein, CdeC, identified via proteomic characterization.Citation86
Figure 3. C. difficile spores imaged by electron microscopy. (A) Transmission electron micrograph of sectioned C. difficile 630 spore, demonstrating the ultrastructure including the exosporium, coat, cortex, core, membrane and ribosomes. Bar, 100 nm. (B) Transmission electron micrograph of thin sections of a C. difficile R20291 spore, showing the spore coat and exosporium. Bar, 200 nm. (C): Scanning electron micrograph of C. difficile 630 spores adhered to 5 day old cultured Caco-2 cells. Arrows indicate interaction with the microvilli of the Caco-2 cells. Bar, 1000 nm. Reproduced from Lawley et al. (2009) (A) ©2009 American Society for Microbiology. Reproduced by permission of American Society for Microbiology. Permission to reuse must be obtained from the rightsholder.83; Reproduced from Barra-Carrasco et al. (2013) (B)Citation ©2013 American Society for Microbiology. Reproduced by permission of American Society for Microbiology. Permission to reuse must be obtained from the rightsholder.86; (C) © 2012 Society for General Microbiology. Reproduced by permission of Society for General Microbiology. Permission to reuse must be obtained from the rightsholder.Citation92
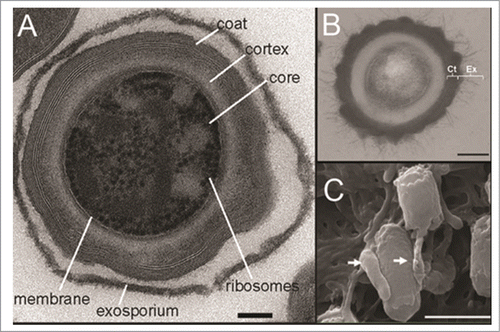
A better understanding of the C. difficile exosporium has been gained by extrapolation of work carried out in Bacillus. In Bacillus species, the exosporium is composed of a paracrystalline basal layer which anchors a hairy nap of fibers ()Citation86 principally made up of the collagen-like protein BclA.Citation87 BclA mediates interactions between the spore and the extracellular matrix (ECM), regulates germination, alters spore hydrophobicity,Citation88 and interacts with the epithelium.Citation89 C. difficile encodes 3 BclA-like proteins, of which one (BclA1) has been characterized.Citation90 In each species, the exosporium has been linked to germination proficiency, with spores that possess an intact exosporium germinating less readily than those without this outermost structure.Citation88,91 In C. difficile the ability of dormant spores to survive within and kill macrophages has also been described.Citation92
Disruption or loss of the exosporium reveals the spore coat, the layer which gives the spore its remarkable resistance to hostile environments. The spore coat in both Bacillus and Clostridium species is a thick, proteinaceous and electron dense layer surrounding the cortex and core of the spores. Proteomic and bioinformatic studies of the C. difficile spore have revealed that many proteins are included in the spore,Citation83,93 however, the assignment of function to these proteins is ongoing.Citation94 Proteins which have been characterized include cotA, cotB, cotCB, cotD, cotE and sodA.Citation94 Each of the Cot proteins has been detected in extracts from the C. difficile spore coat.Citation95 Mutagenesis studies show that cotA is essential for the formation of a functional spore coat, while cotD and cotG encode proteins with catalase activity.Citation94 SodA, encoded by the sodA gene, forms part of the superoxide dismutase family, and cotE encodes a protein with both peroxiredoxin and chitinase activity.Citation94 The CspC protein, encoded by the cspC gene, has been identified as an essential component of the system which senses bile acids and triggers germination.Citation96 One of the major morphogenetic spore proteins of Bacillus species, SpoIVA, has also been investigated in C. difficile, where it is known to interact directly with another spore morphogenetic protein, SipL, in the coat around the forespore.Citation97 Other studies have investigated spore surface proteins found in the soluble and insoluble fractions of C. difficile strain 630 extracts and have identified 54 proteins, many of which show similarity to proteins with proposed enzymatic functions including cell wall hydrolases, oxidoreductases and catalases.Citation98
Spores are highly infectious and are transmitted easily. A murine model of C. difficile infection was used to demonstrate that naïve mice housed in cages previously occupied by C. difficile-infected mice were reliably infected, even after vegetative C. difficile cells were killed by exposure to oxygen.Citation79 The same study also showed that C. difficile-infected animals undergoing antibiotic treatment shed in excess of 108 C. difficile spores per gram of faeces.Citation79 In C. difficile, Spo0A is the master regulator of sporulation.99 Construction of a Spo0A mutant in a clinically relevant isolate of C. difficile, strain R20291, resulted in an asporogenic phenotype. The mutant was found to be markedly deficient in host to host transmission and failed to cause relapse in naïve mice when tested in a mouse transmission model.Citation100 However, in addition to sporulation, defects in Spo0A affect other cellular processes as this protein has been shown to regulate numerous genes in BacillusCitation99; for this reason, modulation of phenotypes other than sporulation may also have influenced transmission efficiency.
Resistance and infectivity, coupled with the extreme longevity of dormant C. difficile spores, can lead to contamination and persistence in the environment. Once shed from an infected patient, C. difficile spores are rapidly disseminated among other patients and surfaces by healthcare workers.Citation101 For example, spores adhere well to stainless steel, a material commonly used in patient-contact areas of hospitals, with no strong correlation observed between particular ribotypes and the ability to adhere to steel.Citation102 Sampling of abiotic surfaces in hospitals has shown heavy contamination with C. difficile spores, where as many as 35% of surfaces sampled in a UK diarrheal ward, most notably bedframes and floors, were found to be contaminated.Citation103
As spores are transferred from surfaces to individuals, they may enter the body via the oral route and experiments performed in vitro indicate that spores adhere well to biotic surfaces (). In these studies, spores were exposed to various intestinal cell lines, notably Caco‑2 (a colonic carcinoma cell line which, upon differentiation, resembles the intestinal epithelium) and HT29 (human colorectal adenocarcinoma cells, which may differentiate into different intestinal morphologies). Binding of spores isolated from 4 toxigenic C. difficile strains to mucus-secreting HT29-MTX cells and enterocyte-like (15 day) Caco-2 cells was detected in one study, however, no binding was detected with undifferentiated or enterocyte-like HT29 cells.Citation104 By contrast, a recent study showed that spores from a number of C. difficile strains bound to undifferentiated Caco-2 cells, and did so in a manner dependent on the hydrophobicity of the spore surface. Adherence was likely to be protein dependent since spores treated with trypsin showed an approximate fourfold reduction in adherence.Citation92 Another study showed efficient binding (60–75%) of hydrophobic spores to undifferentiated (7 day) Caco-2 and HT29 cells, but less efficient binding (20–30%) to 15 day (enterocyte-like) Caco-2 and undifferentiated 15 day HT29 cells.Citation102 In support of the hypothesis that binding to cell lines is spore hydrophobicity-dependent, hydrophilic spores of strain DS1748, a ribotype 002 clinical isolate, were found to bind to each cell line less efficiently than hydrophobic spores,Citation102 however, other variations in spore composition may contribute to this phenotypic difference. Finally, the removal of collagen-like proteins from the C. difficile exosporium via collagenase treatment appeared to increase the binding ability of C. difficile spores to Caco-2 cells, but did so in a manner independent of spore surface hydrophobicity.Citation90
The fate of ingested spores is controlled by the balance of bile salts in the lower intestine, which can promote or inhibit germination. Cholate-containing bile salts, especially sodium taurocholate, promote germination but are degraded by the normal gut microbiota.Citation105 Chenodeoxycholic acids, which are reabsorbed by the gut more rapidly than the pro-germinatory cholic acids, act as competitive inhibitors, preventing signaling and decreasing germination rates.Citation106,107 As primary bile-acid metabolising species of the normal microbiota, such as Clostridium scindens, are disrupted, the relative abundance of these bile salts can shift, encouraging the germination of C. difficile spores and the establishment of a fulminant infection.Citation107 Although fulminant C. difficile infection is the result of vegetative growth of C. difficile, spores continue to be produced and shed during the course of infection. Shedding of C. difficile spores by infected individuals in hospitals is well documented,Citation108 with shedding of both epidemic and non-epidemic C. difficile spores by asymptomatic patients also demonstrated.Citation109
The production of endospores by C. difficile, initiated in response to unfavourable environmental conditions, is controlled by complex genetic regulatory systems. Spo0A is the master regulator of sporulation in C. difficile, with disruption of the spo0A gene leading to a sporulation deficient phenotype.Citation99,110 Mapping of C. difficile sporulation initiation pathways in comparison to those of B. subtilis suggests that C. difficile lacks the sporulation phosphorelay pathway complexity of this Bacillus species.Citation111 Three independent recent studies have explored these regulatory pathways in more detail through the construction and analysis of mutants in genes encoding the sigma factors σF, σE, σG and σK.Citation112,113,114 Each of these studies found that several hundred genes were differentially regulated by the various sigma factors, confirming the importance of sigma factors in C. difficile spore regulation. More importantly, these studies also highlighted similarities and differences between C. difficile and Bacillus species sporulation. Some pathways are conserved, such as the dependence of σK on σE and σG on σF. However, other pathways differed, for example, in Bacillus species, σG and σF depend on the action of σE, and σK depends on the action of σG, however, in C. difficile these factors were shown to act independently.Citation112,113
The spores of C. difficile are complex structures, formed under the control of intricate regulatory networks. The ability of spores to persist in hostile environments for extended time periods makes them important agents in disease transmission in both humans and animals and contributes to the virulence potential of C. difficile.
Other virulence properties: colonisation and adherence factors
For many pathogens, adhesion to host cells is a crucial first step for colonization and the establishment of infection. The adherence of spores and vegetative cells to gut epithelial cell lines, and the observation that spores of a ribotype 027 strain adhere better to human epithelial cell monolayers than spores from a ribotype 002 isolate,Citation102 suggest that adherence may be an important factor in C. difficile disease pathogenesis.Citation92,102 Although TcdA and TcdB are the major C. difficile virulence factors, other putative virulence factors include those that may play a role in adherence and colonisation. These include the surface layer (S-layer) and cell wall proteins (CWP), fibronectin binding proteins, flagella, fimbriae, and the heat shock protein GroEL. While the potency of the toxins in disease may obscure the role of these additional factors in C. difficile pathogenesis, many studies have examined the role of non-toxin related virulence factors in disease. The following section provides an overview of these factors.
S-layer and cell wall proteins
Cell surface layers or S-layers are surface-exposed proteinaceous layers that are produced by many bacteria. S-layers typically consist of identical monomeric glycoprotein subunits which form a regular 2-dimensional array visible by electron microscopy.Citation115 Functions of the S-layer vary between different bacterial species and include such roles as protection from bacterial parasites, adhesion and complement resistance.Citation115 The S-layer typically forms the outermost layer of the bacterium, giving it strong immunogenic potential. S-layer proteins (SLPs) have been detected in all C. difficile strains. In contrast to most bacteria, where the S-layer is composed of a singular protein species, the S-layer of C. difficile consists of 2 protein subunits commonly referred to as the HMW (high molecular weight) and LMW (low molecular weight) proteins. These forms are derived when their polypeptide precursor, SlpA, undergoes proteolytic cleavage by the protease Cwp84 ().Citation116 The HMW protein shows high interspecies conservation while the LMW protein shows inter-strain variability.Citation117 The HMW and LMW subunits form a tightly associated non-covalent complexCitation118 that is thought to play a role in host cell adhesion, evidenced by adherence to cells in vitro and to sections of human gastrointestinal tissue.Citation119 Furthermore, antibodies against SlpA are able to block adherence of C. difficile to cultured cells.Citation119,120 Adherence of C. difficile to epithelial cells has also been shown to be greatly reduced on monolayers pre-incubated with Slp preparations and purified SlpA proteins.Citation120 These studies highlight the important role that surface layer proteins may play in the infection process.
Figure 4. The S-Layer protein complex of C. difficile. The S-layer of C. difficile is composed of high and low molecular weight surface layer proteins (HMW and LMW SLPs). Figure shows the steps involved in the maturation of the S-layer protein complex. Three-stages are shown: (A) - the removal of the signal peptide, (B) - cleavage of SLP by the protease, Cwp84, to generate HMW and LMW, and (C) - The formation of the S-layer matrix by the re-association of the LMW and HMW SLPs. Reprinted with permission from Dang TH, de la Riva L, Fagan RP, Storck EM, Heal WP, Janoir C, Fairweather NF, Tate EW. Chemical probes of surface layer biogenesis in Clostridium difficile. ACS Chem Biol 2010; 5:279-85; PMID:20067320; http://dx.doi.org/10.1021/cb9002859. Copyright 2010 American Chemical Society.Citation116
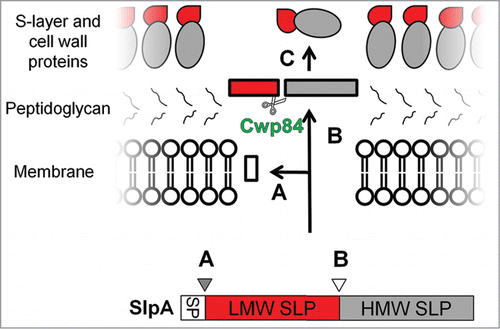
In addition to adherence, recent studies have shown that SLPs are important in immune recognition and the inflammatory response.Citation121 Furthermore, purified C. difficile SLPs applied to monocytes appear to modulate the immune response by inducing IL-1β, IL-6 and IL-10 production, although no differences in immunomodulatory patterns were identified between SLPs isolated from epidemic and non-epidemic C. difficile isolates.Citation122 SLPs have also been shown to induce dendritic cell maturation in mice, and a role for TLR4 in the recognition of SLPs by dendritic cells has been reported.Citation121 SLPs are the most well-characterized family of C. difficile cell wall proteins (CWPs) with 28 additional paralogs to the HMW SlpA identified in C. difficile strain 630 using bioinformatic analysis.Citation123 Collectively, these proteins possess 2 to 3 conserved cell wall binding domains responsible for anchoring of the protein to the outer surface of the bacterium along with a unique domain that may specify function.Citation124 Twelve of the genes encoding CWPs are clustered in a genomic region termed the slpA locus.Citation125 Recent bioinformatic analysis of the slpA genomic locus (>63 kb) from 57 C. difficile clinical isolates indicates that genetic diversity exists between the S-layer loci from various isolates. This antigenic variation may make vaccination strategies against this region more difficult.Citation126
The remaining 17 cwp loci are distributed throughout the C. difficile genome.Citation127 Bioinformatic analysis of 40 C. difficile clinical isolates indicates that the remaining cwp loci can be split into 2 groups: one composed of 9 cwp loci that are highly conserved both within and between ribotypes, and a second more variable group.Citation127 Transcriptomic and proteomic analyses have shown that a number of these genes and proteins are expressed in vitro.Citation128,129 Moreover, analysis of patient serum has detected antibodies to a number of cell wall proteins, in addition to the HMW and LMW SLPs. This analysis suggests that these CWPs are expressed in vivo and are recognized by the immune system,Citation130 serving as potential therapeutic targets.
Of the cell wall proteins investigated thus far perhaps the best characterized is Cwp84, a cysteine protease involved in processing of the S-layer.Citation131 Cwp84 has also been shown to degrade ECM proteins in vitro, suggesting that this protein may play a role in tissue degradation and bacterial dissemination during infection.Citation132 Two forms of this protease are associated with the bacterium, an ∼80 kDa protein and its cleaved 47 kDa mature protease form, each of which may display different proteolytic activities and have different roles in vivo that may aid in the infection process.Citation133 A protein homolog of Cwp84, Cwp13, has also been characterized and shown to play a role in the processing of Cwp84 but exhibits weak activity toward SlpA, and is not considered essential for Cwp84 activity or Slp processing.Citation131 Another cell surface protein implicated in adherence is Cwp66 (66 kDa) which harbours 2 domains, each with 3 imperfect repeats, and an additional domain homologous to the B. subtilis autolysin, CwlB.Citation134 This protein was detected with antibodies raised against surface proteins of heat-shocked bacteria, suggesting that it could be a surface-associated heat shock protein. Adherence of heat-shocked C. difficile to cultured cells in vitro has been shown to be reduced in the presence of antibodies to the N- and C- terminal portions of Cwp66, whereas adherence of non-heat shocked cells was unaffected.Citation134 This suggests that heat-shock is important for Cwp66 to function as an adhesive protein and indicates that other proteins are involved in cell adherence in vivo.
The largest characterized member of the cell wall proteins, CwpV (167 kDa) from C. difficile strain 630, has phase variable expression.Citation135 The C-terminal repeat region of this protein is highly variable between C. difficile strains, with 5 antigenically distinct repeat types identified.Citation136 It is thought that CwpV is post-translationally processed into 2 fragments that re-associate to form a stable, non-covalently associated complex.Citation135,136 Studies propose that CwpV promotes aggregation of the bacteria via the C-terminal repetitive domain indicating a potential role for the protein in bacterial interaction and biofilm-like formation in the host gut.Citation136
Polysaccharides
Three cell surface polysaccharides, PSI, PSII and PSIII, have been described for vegetative cells of C. difficile, with all ribotypes, including ribotype O27, found to express PSII most abundantly (for a recent review see Monteiro et al., 2013).Citation137 Vaccine candidates have therefore largely centered around this carbohydrate, with the immunogenicity of different vaccines consisting of native and synthetic PSII conjugates being tested in pig, mouse and hamster models.Citation137 Glycan microarrays carrying synthetic PSI, PSII and PSIII are able to detect IgG antibodies in the sera of CDI patients,Citation138 and anti-PSII IgA antibodies have been detected in the feces of patients,Citation139 highlighting the importance of this immunogen. Recent studies using a naïve mouse model and a PSII glycoprotein conjugated to recombinant TcdA and TcdB fragments showed that antibodies against PSII were produced, as well as neutralizing toxin antibodies, suggesting that vaccines comprising a combination of such antigens could be successfully developed in the future.Citation140
Fimbriae and pili
Some C. difficile strains possess fimbriae while others do not.Citation141,142 When fimbriae have been detected, they were found to be 4–9 nm in diameter and up to 6 μm long, and appeared to be polar in origin.Citation141 In this early study, no correlation was observed between the presence of fimbriae and the ability to cause disease in humans or animals.Citation141 More recent analysis has identified human disease-causing isolates that also do not contain fimbriae,Citation142 suggesting that fimbriae may not be important in disease pathogenesis of this organism. Bioinformatic analysis has shown that the majority of clostridia which have been genome sequenced encode putative Type IV pilus genes.Citation143 Analysis of C. difficile strains 630Citation143 and R20291Citation144 has revealed 9 pilin and putative pilin-like genes. Immunization studies using mice and small mammals with proteins produced from 6 of the genes identified from R20291 yielded antibody responses with varying titres and cross-reactivity.Citation144 While future studies will shed more light on the structure and function of Type IV pili in C. difficile, the preliminary studies with R20291 form the basis of a potential anti–C. difficile pilin-based vaccine.Citation144
Flagella
Flagella are an important virulence determinant for many enteric pathogens, playing a role in host invasion and colonisation. Likewise, strains of C. difficile are known to be motile and to possess flagella, although this is a variable trait among clonal clinical isolates.Citation145 The 2 best characterized C. difficile flagellar proteins include FliC, the major flagellin structural monomer, and FliD, the cap protein.Citation146,147 The role of flagella in the pathogenesis of CDI is contentious and appears to be strain dependent.Citation148,149 Studies with some isolates, including the epidemic PCR ribotype 027 strain R20291, showed flagella to be important in adherence to cells and intestinal tissue.Citation149 However, the absence of flagella in a non-epidemic strain (630Δerm) did not reduce adherence to intestinal epithelial cell lines but rather mediated increased adherence to cells.Citation148,149 This inconsistency has also been observed in vivo. In one study 630Δerm fliC and fliD mutants were found to be more virulent than the wild-type strain Citation148 whereas others using the same model showed no difference between a fliC mutant and its parent.Citation150 The latter study agrees with a single infection study in a monoxenic mouse model where a 630Δerm fliC mutant exhibited no difference in adherence during colonization when compared to the wild-type strain.Citation149
Also of note is a study by Aubry et al. (2012) that showed increased transcription of the 4 pathogenicity locus genes, tcdA, tcdB, tcdE, and tcdR, in a 630Δerm fliC mutant.Citation150 This mutant also produced more TcdA and TcdB than the wild-type strain. Similarly, QRT-PCR was used to show strong upregulation of the tcdA gene in both 630Δerm fliC and fliD mutants,Citation149 suggesting a more complex role for flagella in C. difficile virulence than motility alone. Furthermore, as with other bacteria, flagella from C. difficile have been implicated in the development of biofilms,Citation151 a matrix that shelters the bacterial community from environmental stresses such as antibiotic exposure. This matrix also enables more efficient bacterial colonization due to its adhesive properties.
Fibronectin-binding protein
Fibronectin is a large molecular weight glycoprotein which is important in the adhesion of many bacterial pathogens to the host. C. difficile binds to extracellular matrix proteins such as fibronectin, fibrinogen, collagen and vitronectinCitation152 C. difficile possesses a 68 kDa fibronectin-binding protein (Fbp68), annotated FbpA in strain 630, the encoding gene of which appears to be highly conserved in C. difficile isolates.Citation153 Fbp68 is a manganese binding protein, requiring the ion for structural stability and fibronectin binding.Citation154 Like many virulence factors of C. difficile, the role of the fibronectin binding protein in disease pathogenesis is not completely understood. Fbp68 has been shown to bind to ECM constituents including fibronectin, fibrinogen and vitronectin, and antibodies against Fbp68 have been shown to partially inhibit binding to cells in vitro.Citation153 Mutagenesis studies of fbpA from strain 630 unexpectedly showed that an fbpA mutant adhered more efficiently in vitro than the wild-type control. However, differing results were observed in vivo with various distinct mouse models. For example, an fbpA mutant was found to be shed in faeces at the same rate as the wild-type strain in monoxenic mice but showed reduced colonization of the cecal wall.Citation155 In dixenic mice, shedding of the fbpA mutant strain was reduced early in infection but there was no difference in cecal colonization in comparison to the parent strain.Citation155 Similarly, no difference in intestinal implantation and cecal colonization was observed between the wild-type and the fbpA mutant in studies with human microbiota-associated mice.Citation155
A recent study identified a new metalloprotease, ZmpI, with sequence similarity to B. anthracis lethal factor, which was also able to degrade ECM-associated proteins, specifically fibrinogen and fibronectin.Citation156 This degradative property is suggested to enhance the invasiveness and spread of C. difficile within the infected host, as has been shown for other pathogens.Citation157 Also reported recently is the identification of a collagen binding protein, CbpA, found to bind to collagens I and V, the most common of the collagen components found in many tissues including the gut.Citation158 It is clear that further research is required to fully elucidate the function that these ECM binding proteins provide in disease pathogenesis of C. difficile, particularly with respect to the interaction of this organism with the gut mucosa.
Heat-shock protein GroEL (Hsp60)
Adherence of C. difficile to epithelial cells in vitro has been shown to increase following heat shock.Citation159 This phenomenon has led to the identification and characterization of Cwp66 and has also suggested a role for other heat shock proteins such as GroEL in the adherence properties of this bacterium. GroEL is a 58 kDa protein that is expressed following exposure to a number of stresses including heat, acid or osmotic shock, iron deprivation, the presence of sub-inhibitory concentrations of the antibiotic ampicillin, or contact with eukaryotic cells in vitro.Citation159 Following heat-shock of C. difficile, GroEL was found to be surface associated as well as being released into the extracellular space.Citation159,160 In support of this finding, fractionation experiments showed that GroEL can be found in the bacterial cell cytoplasm as well as in the membrane.Citation159,160 Antibodies to GroEL and purified GroEL protein have both been shown to inhibit the attachment of C. difficile to eukaryotic cells in vitro, suggesting that this protein plays a role in adherence.Citation160 More recently, immunization studies using recombinant GroEL were found to reduce the gut colonization efficiency of C. difficile in infected mice,Citation161 supporting a role for GroEL in C. difficile intestinal adhesion.
Conclusions
Host gut colonization with C. difficile, usually following antibiotic treatment, can lead to CDI, which is the most common cause of bacterial AAD worldwide. C. difficile is becoming increasingly resistant to numerous antibiotics and this pathogen has been described as an urgent threat to public health.Citation162 Every facet of the C. difficile lifecycle must be considered in the development of preventative or therapeutic agents. Spore contamination of hospitals and other facilities, including animal facilities and the environment, is of utmost concern, since spores are easily transmitted to humans and animals but are difficult to eradicate. Research efforts directed toward understanding the transmission of this bacterium have already resulted in decreased incidence rates of CDI in various facilities,Citation163 with stringent clinical practices now in place in many countries.
Studies dissecting C. difficile pathogenesis have provided important new insights into the role of virulence factors in disease, particularly in relation to the role of toxins. These studies have highlighted the critical role played by TcdB in CDICitation42–44 and the inclusion of TcdB in toxin-directed therapeutics has now become a priority. Further to this, other studies currently underway are investigating the efficacy of using many of the virulence factors discussed in this review as targets for disease prevention or treatment. One such example involves the use of spore germination inhibitors as novel therapeutics against CDI, with promising results obtained using the bile salt analog, CamSA, in preventing disease in mice.Citation164
The rapid progress in developing an understanding of C. difficile virulence factors in the last decade, coupled with the development of new and diverse animal models,Citation165 has facilitated the development of new strategies to combat this bacterium. Although antibiotic therapy is currently still the best treatment option for C. difficile, it is likely that studies dissecting the role of spore and vegetative cell factors, as well as toxins, in establishing infection and causing disease will allow prevention and treatment strategies to move beyond antibiotics through the development of new and targeted therapeutics.
Disclosure of Potential Conflicts of Interest
No potential conflicts of interest were disclosed.
Acknowledgments
The researchers were supported by Project Grants from the Australian National Health and Medical Research Council (NHMRC). DL was supported by an ARC Future Fellowship from the Australian Research Council (ARC).
References
- O'Brien JA, Lahue BJ, Caro JJ, Davidson DM. The emerging infectious challenge of Clostridium difficile–associated disease in Massachusetts hospitals: clinical and economic consequences. Infect Control Hosp Epidemiol 2007; 28:1219-27; PMID:17926270; http://dx.doi.org/10.1086/522676
- Khanna S, Pardi DS, Aronson SL, Kammer PP, Orenstein R, St Sauver JL, Harmsen WS, Zinsmeister AR. The epidemiology of community-acquired Clostridium difficile infection: a population-based study. Am J Gastroenterol 2012; 107:89-95; PMID:22108454; http://dx.doi.org/10.1038/ajg.2011.398
- Rupnik M. Heterogeneity of large clostridial toxins: importance of Clostridium difficile toxinotypes. FEMS Microbiol Rev 2008; 32:541-55; PMID:18397287; http://dx.doi.org/10.1111/j.1574-6976.2008.00110.x
- McDonald LC, Killgore GE, Thompson A, Owens RC, Kazakova SV, Sambol SP, Johnson S, Gerding DN. An epidemic, toxin gene–variant strain of Clostridium difficile. New Engl J Med 2005; 353:2433-41; PMID:16322603; http://dx.doi.org/10.1056/NEJMoa051590
- Goorhuis A, Debast SB, van Leengoed LA, Harmanus C, Notermans DW, Bergwerff AA, Kuijper EJ. Clostridium difficile PCR ribotype 078: an emerging strain in humans and in pigs? J Clin Microbiol 2008; 46:1157; PMID:18326836; http://dx.doi.org/10.1128/JCM.01536-07
- Spigaglia P, Barbanti F, Mastrantonio P, Brazier JS, Barbut F, Delmee M, Kuijper E, Poxton IR. Fluoroquinolone resistance in Clostridium difficile isolates from a prospective study of C. difficile infections in Europe. J Med Microbiol 2008; 57:784-9; PMID:18480338; http://dx.doi.org/10.1099/jmm.0.47738-0
- Akerlund T, Persson I, Unemo M, Noren T, Svenungsson B, Wullt M, Burman LG. Increased sporulation rate of epidemic Clostridium difficile Type 027/NAP1. J Clin Microbiol 2008; 46:1530-3; PMID:18287318; http://dx.doi.org/10.1128/JCM.01964-07
- Naggie S, Miller BA, Zuzak KB, Pence BW, Mayo AJ, Nicholson BP, Kutty PK, McDonald LC, Woods CW. A case-control study of community-associated Clostridium difficile infection: no role for proton pump inhibitors. Am J Med 2011; 124:276 e1-7; PMID:21396512; http://dx.doi.org/10.1016/j.amjmed.2010.10.013
- Carter GP, Rood JI, Lyras D. The role of toxin A and toxin B in the virulence of Clostridium difficile. Trends Microbiol 2012; 20:21-9; PMID:22154163; http://dx.doi.org/10.1016/j.tim.2011.11.003
- Geric B, Carman RJ, Rupnik M, Genheimer CW, Sambol SP, Lyerly DM, Gerding DN, Johnson S. Binary toxin-producing, large clostridial toxin-negative Clostridium difficile strains are enterotoxic but do not cause disease in hamsters. J Infect Dis 2006; 193:1143-50; PMID:16544255; http://dx.doi.org/10.1086/501368
- Braun V, Hundsberger T, Leukel P, Sauerborn M, Eichel-Streiber Cv. Definition of the single integration site of the pathogenicity locus in Clostridium difficile. Gene 1996; 181:29-38; PMID:8973304; http://dx.doi.org/10.1016/S0378-1119(96)00398-8
- Mani N, Dupuy B. Regulation of toxin synthesis in Clostridium difficile by an alternative RNA polymerase sigma factor. Proc Natl Acad Sci USA 2001; 98:5844-9; PMID:11320220; http://dx.doi.org/10.1073/pnas.101126598
- Carter GP, Douce GR, Govind R, Howarth PM, Mackin KE, Spencer J, Buckley AM, Antunes A, Kotsanas D, Jenkin GA, et al. The Anti-Sigma Factor TcdC Modulates Hypervirulence in an Epidemic BI/NAP1/027 Clinical Isolate of Clostridium difficile. PLoS Pathog 2011; 7:e1002317; PMID:22022270; http://dx.doi.org/10.1371/journal.ppat.1002317
- Govind R, Dupuy B. Secretion of Clostridium difficile toxins A and B requires the holin-like protein TcdE. PLoS Pathog 2012; 8:e1002727; PMID:22685398; http://dx.doi.org/10.1371/journal.ppat.1002727
- Matamouros S, England P, Dupuy B. Clostridium difficile toxin expression is inhibited by the novel regulator TcdC. Mol Microbiol 2007; 64:1274-88; PMID:17542920; http://dx.doi.org/10.1111/j.1365-2958.2007.05739.x
- Cartman ST, Kelly ML, Heeg D, Heap JT, Minton NP. Precise manipulation of the Clostridium difficile chromosome reveals a lack of association between the tcdC genotype and toxin production. Appl Environ Microbiol 2012; 78:4683-90; PMID:22522680; http://dx.doi.org/10.1128/AEM.00249-12
- Bakker D, Smits WK, Kuijper EJ, Corver J. TcdC does not significantly repress toxin expression in Clostridium difficile 630DeltaErm. PLoS One 2012; 7:e43247; PMID:22912837; http://dx.doi.org/10.1371/journal.pone.0043247
- Olling A, Seehase S, Minton NP, Tatge H, Schroter S, Kohlscheen S, Pich A, Just I, Gerhard R. Release of TcdA and TcdB from Clostridium difficile cdi 630 is not affected by functional inactivation of the tcdE gene. Microb Pathog 2012; 52:92-100; PMID:22107906; http://dx.doi.org/10.1016/j.micpath.2011.10.009
- Brouwer MS, Roberts AP, Hussain H, Williams RJ, Allan E, Mullany P. Horizontal gene transfer converts non-toxigenic Clostridium difficile strains into toxin producers. Nat Commun 2013; 4:2601; PMID:24131955; http://dx.doi.org/10.1038/ncomms3601
- von Eichel-Streiber C, Laufenberg-Feldmann S, Sartingen S, Schulze J, Sauerborn M. Comparative sequence analysis of the Clostridium difficile toxins A and B. Mol Gen Genet 1992; 233:260-8; PMID:1603068; http://dx.doi.org/10.1007/BF00587587
- Jank T, Aktories K. Structure and mode of action of clostridial glucosylating toxins: the ABCD model. Trends Microbiol 2008; 16:222-9; PMID:18394902; http://dx.doi.org/10.1016/j.tim.2008.01.011
- Aktories K. Bacterial protein toxins that modify host regulatory GTPases. Nat Rev Microbiol 2011; 9:487-98; PMID:21677684; http://dx.doi.org/10.1038/nrmicro2592
- Papatheodorou P, Zamboglou C, Genisyuerek S, Guttenberg G, Aktories K. Clostridial glucosylating toxins enter cells via clathrin-mediated endocytosis. PLoS One 2010; 5:e10673; PMID:20498856; http://dx.doi.org/10.1371/journal.pone.0010673
- Pruitt RN, Chambers MG, Ng KK, Ohi MD, Lacy DB. Structural organization of the functional domains of Clostridium difficile toxins A and B. Proc Natl Acad Sci U S A 2010; 107:13467-72; PMID:20624955; http://dx.doi.org/10.1073/pnas.1002199107
- Egerer M, Giesemann T, Herrmann C, Aktories K. Autocatalytic processing of Clostridium difficile toxin B. Binding of inositol hexakisphosphate. J Biol Chem 2009; 284:3389-95; PMID:19047051; http://dx.doi.org/10.1074/jbc.M806002200
- Pruitt RN, Chagot B, Cover M, Chazin WJ, Spiller B, Lacy DB. Structure-function analysis of inositol hexakisphosphate-induced autoprocessing in Clostridium difficile toxin A. J Biol Chem 2009; 284:21934-40; PMID:19553670; http://dx.doi.org/10.1074/jbc.M109.018929
- Jank T, Giesemann T, Aktories K. Rho-glucosylating Clostridium difficile toxins A and B: new insights into structure and function. Glycobiology 2007; 17:15R-22R; PMID:17237138; http://dx.doi.org/10.1093/glycob/cwm004
- Gerhard R, Nottrott S, Schoentaube J, Tatge H, Olling A, Just I. Glucosylation of Rho GTPases by Clostridium difficile toxin A triggers apoptosis in intestinal epithelial cells. J Med Microbiol 2008; 57:765-70; PMID:18480335; http://dx.doi.org/10.1099/jmm.0.47769-0
- Ng J, Hirota SA, Gross O, Li Y, Ulke-Lemee A, Potentier MS, Schenck LP, Vilaysane A, Seamone ME, Feng H, et al. Clostridium difficile toxin-induced inflammation and intestinal injury are mediated by the inflammasome. Gastroenterology 2010; 139:542-52, 52 e1-3; PMID:20398664; http://dx.doi.org/10.1053/j.gastro.2010.04.005
- Madan R, Petri Jr WA. Immune responses to Clostridium difficile infection. Trends Mol Med 2012; 18:658-66; PMID:23084763; http://dx.doi.org/10.1016/j.molmed.2012.09.005
- Pothoulakis C, Castagliuolo I, LaMont JT. Nerves and intestinal mast cells modulate responses to enterotoxins. News Physiol Sci 1998; 13:58-63; PMID:11390763;
- Lanis JM, Barua S, Ballard JD. Variations in TcdB activity and the hypervirulence of emerging strains of Clostridium difficile. PLoS Pathog 2010; 6; PMID:20808849; http://dx.doi.org/10.1371/journal.ppat.1001061
- Lanis JM, Heinlen LD, James JA, Ballard JD. Clostridium difficile 027/BI/NAP1 encodes a hypertoxic and antigenically variable form of TcdB. PLoS Pathog 2013; 9:e1003523; PMID:23935501; http://dx.doi.org/10.1371/journal.ppat.1003523
- Lyerly DM, Saum KE, MacDonald DK, Wilkins TD. Effects of Clostridium difficile toxins given intragastrically to animals. Infect Immun 1985; 47:349-52; PMID:3917975;
- Mitchell TJ, Ketley JM, Haslam SC, Stephen J, Burdon DW, Candy DC, Daniel R. Effect of toxin A and B of Clostridium difficile on rabbit ileum and colon. Gut 1986; 27:78-85; PMID:3949240; http://dx.doi.org/10.1136/gut.27.1.78
- Kim PH, Iaconis JP, Rolfe RD. Immunization of adult hamsters against Clostridium difficile-associated ileocecitis and transfer of protection to infant hamsters. Infect Immun 1987; 55:2984-92; PMID:3679541;
- Kyne L, Warny M, Qamar A, Kelly CP. Association between antibody response to toxin A and protection against recurrent Clostridium difficile diarrhoea. Lancet 2001; 357:189-93; PMID:11213096; http://dx.doi.org/10.1016/S0140-6736(00)03592-3
- Drudy D, Fanning S, Kyne L. Toxin A-negative, toxin B-positive Clostridium difficile. Int J Infect Dis 2007; 11:5-10; PMID:16857405; http://dx.doi.org/10.1016/j.ijid.2006.04.003
- Savidge TC, Pan WH, Newman P, O'Brien M, Anton PM, Pothoulakis C. Clostridium difficile toxin B is an inflammatory enterotoxin in human intestine. Gastroenterology 2003; 125:413-20; PMID:12891543; http://dx.doi.org/10.1016/S0016-5085(03)00902-8
- Hamm EE, Voth DE, Ballard JD. Identification of Clostridium difficile toxin B cardiotoxicity using a zebrafish embryo model of intoxication. Proc Natl Acad Sci U S A 2006; 103:14176-81; PMID:16966605; http://dx.doi.org/10.1073/pnas.0604725103
- Steele J, Mukherjee J, Parry N, Tzipori S. Antibody against TcdB, but not TcdA, prevents development of gastrointestinal and systemic Clostridium difficile disease. J Infect Dis 2013; 207:323-30; PMID:23125448; http://dx.doi.org/10.1093/infdis/jis669
- Lyras D, O’Connor JR, Howarth PK, Sambol SP, Carter GP, Phumoonna T, Poon R, Adams V, Vedantam G, Johnson S, et al. Toxin B is essential for virulence of Clostridium difficile. Nature 2009; 458:1176-9; PMID:19252482; http://dx.doi.org/10.1038/nature07822
- Kuehne SA, Cartman ST, Heap JT, Kelly ML, Cockayne A, Minton NP. The role of toxin A and toxin B in Clostridium difficile infection. Nature 2010; 467:711-3; PMID:20844489; http://dx.doi.org/10.1038/nature09397
- Kuehne SA, Collery MM, Kelly ML, Cartman ST, Cockayne A, Minton NP. Importance of Toxin A, Toxin B, and CDT in Virulence of an Epidemic Clostridium difficile Strain. J Infect Dis 2013; 209:83-6; PMID:23935202; http://dx.doi.org/10.1093/infdis/jit426
- Lowy I, Molrine DC, Leav BA, Blair BM, Baxter R, Gerding DN, Nichol G, Thomas WD, Jr., Leney M, Sloan S, et al. Treatment with monoclonal antibodies against Clostridium difficile toxins. N Engl J Med 2010; 362:197-205; PMID:20089970; http://dx.doi.org/10.1056/NEJMoa0907635
- Roberts A, McGlashan J, Al-Abdulla I, Ling R, Denton H, Green S, Coxon R, Landon J, Shone C. Development and evaluation of an ovine antibody-based platform for treatment of Clostridium difficile infection. Infect Immun 2012; 80:875-82; PMID:22144483; http://dx.doi.org/10.1128/IAI.05684-11
- Steele J, Sponseller J, Schmidt D, Cohen O, Tzipori S. Hyperimmune bovine colostrum for treatment of GI infections: a review and update on Clostridium difficile. Hum Vaccin Immunother 2013; 9:1565-8; PMID:23435084; http://dx.doi.org/10.4161/hv.24078
- Greenberg RN, Marbury TC, Foglia G, Warny M. Phase I dose finding studies of an adjuvanted Clostridium difficile toxoid vaccine. Vaccine 2012; 30:2245-9; PMID:22306375; http://dx.doi.org/10.1016/j.vaccine.2012.01.065
- Jin K, Wang S, Zhang C, Xiao Y, Lu S, Huang Z. Protective antibody responses against Clostridium difficile elicited by a DNA vaccine expressing the enzymatic domain of toxin B. Hum Vaccin Immunother 2013; 9:63-73; PMID:23143772; http://dx.doi.org/10.4161/hv.22434
- Permpoonpattana P, Hong HA, Phetcharaburanin J, Huang JM, Cook J, Fairweather NF, Cutting SM. Immunization with Bacillus spores expressing toxin A peptide repeats protects against infection with Clostridium difficile strains producing toxins A and B. Infect Immun 2011; 79:2295-302; PMID:21482682; http://dx.doi.org/10.1128/IAI.00130-11
- Seregin SS, Aldhamen YA, Rastall DP, Godbehere S, Amalfitano A. Adenovirus-based vaccination against Clostridium difficile toxin A allows for rapid humoral immunity and complete protection from toxin A lethal challenge in mice. Vaccine 2012; 30:1492-501; PMID:22200503; http://dx.doi.org/10.1016/j.vaccine.2011.12.064
- Wang H, Sun X, Zhang Y, Li S, Chen K, Shi L, Nie W, Kumar R, Tzipori S, Wang J, et al. A chimeric toxin vaccine protects against primary and recurrent Clostridium difficile infection. Infect Immun 2012; 80:2678-88; PMID:22615245; http://dx.doi.org/10.1128/IAI.00215-12
- Tian JH, Fuhrmann SR, Kluepfel-Stahl S, Carman RJ, Ellingsworth L, Flyer DC. A novel fusion protein containing the receptor binding domains of C. difficile toxin A and toxin B elicits protective immunity against lethal toxin and spore challenge in preclinical efficacy models. Vaccine 2012; 30:4249-58; PMID:22537987; http://dx.doi.org/10.1016/j.vaccine.2012.04.045
- Schwan C, Stecher B, Tzivelekidis T, van Ham M, Rohde M, Hardt WD, Wehland J, Aktories K. Clostridium difficile toxin CDT induces formation of microtubule-based protrusions and increases adherence of bacteria. PLoS Pathog 2009; 5:e1000626; PMID:19834554; http://dx.doi.org/10.1371/journal.ppat.1000626
- Schwan C, Kruppke AS, Nolke T, Schumacher L, Koch-Nolte F, Kudryashev M, Stahlberg H, Aktories K. Clostridium difficile toxin CDT hijacks microtubule organization and reroutes vesicle traffic to increase pathogen adherence. Proc Natl Acad Sci U S A 2014; 111:2313-8; PMID:24469807; http://dx.doi.org/10.1073/pnas.1311589111
- Stubbs S, Rupnik M, Gibert M, Brazier J, Duerden B, Popoff M. Production of actin-specific ADP-ribosyltransferase (binary toxin) by strains of Clostridium difficile. FEMS Microbiol Lett 2000; 186:307-12; PMID:10802189; http://dx.doi.org/10.1111/j.1574-6968.2000.tb09122.x
- Bacci S, Molbak K, Kjeldsen MK, Olsen KE. Binary toxin and death after Clostridium difficile infection. Emerg Infect Dis 2011; 17:976-82; PMID:21749757; http://dx.doi.org/10.3201/eid/1706.101483
- Popoff MR, Rubin EJ, Gill DM, Boquet P. Actin-specific ADP-ribosyltransferase produced by a Clostridium difficile strain. Infect Immun 1988; 56:2299-306; PMID:3137166
- Barth H, Aktories K, Popoff MR, Stiles BG. Binary bacterial toxins: biochemistry, biology, and applications of common Clostridium and Bacillus proteins. Microbiol Mol Biol Rev 2004; 68:373-402; PMID:15353562; http://dx.doi.org/10.1128/MMBR.68.3.373-402.2004
- Perelle S, Gibert M, Bourlioux P, Corthier G, Popoff M. Production of a complete binary toxin (actin-specific ADP-ribosyltransferase) by Clostridium difficile CD196. Infect Immun 1997; 65:1402-7; PMID:9119480
- Carter GP, Lyras D, Allen DL, Mackin KE, Howarth PM, O'Connor JR, Rood JI. Binary toxin production in Clostridium difficile is regulated by CdtR, a LytTR family response regulator. J Bacteriol 2007; 189:7290-301; PMID:17693517; http://dx.doi.org/10.1128/JB.00731-07
- Perelle S, Scalzo S, Kochi S, Mock M, Popoff MR. Immunological and functional comparison between Clostridium perfringens iota toxin, C. spiroforme toxin, and anthrax toxins. FEMS Microbiol Lett 1997; 146:117-21; PMID:8997715; http://dx.doi.org/10.1111/j.1574-6968.1997.tb10180.x
- Papatheodorou P, Carette JE, Bell GW, Schwan C, Guttenberg G, Brummelkamp TR, Aktories K. Lipolysis-stimulated lipoprotein receptor (LSR) is the host receptor for the binary toxin Clostridium difficile transferase (CDT). Proc Natl Acad Sci U S A 2011; 108:16422-7; PMID:21930894; http://dx.doi.org/10.1073/pnas.1109772108
- Mesli S, Javorschi S, Berard AM, Landry M, Priddle H, Kivlichan D, Smith AJ, Yen FT, Bihain BE, Darmon M. Distribution of the lipolysis stimulated receptor in adult and embryonic murine tissues and lethality of LSR-/- embryos at 12.5 to 14.5 days of gestation. Eur J Biochem 2004; 271:3103-14; PMID:15265030; http://dx.doi.org/10.1111/j.1432-1033.2004.04223.x
- Nagahama M, Yamaguchi A, Hagiyama T, Ohkubo N, Kobayashi K, Sakurai J. Binding and internalization of Clostridium perfringens iota-toxin in lipid rafts. Infect Immun 2004; 72:3267-75; PMID:15155629; http://dx.doi.org/10.1128/IAI.72.6.3267-3275.2004
- Blocker D, Behlke J, Aktories K, Barth H. Cellular uptake of the Clostridium perfringens binary iota-toxin. Infect Immun 2001; 69:2980-7; PMID:11292715; http://dx.doi.org/10.1128/IAI.69.5.2980-2987.2001
- Gülke I, Pfeifer G, Liese J, Fritz M, Hofmann F, Aktories K, Barth H. Characterization of the enzymatic component of the ADP-ribosyltransferase toxin CDTa from Clostridium difficile. Infect Immun 2001; 69:6004-11; PMID:11553537; http://dx.doi.org/10.1128/IAI.69.10.6004-6011.2001
- Aktories K, Lang AE, Schwan C, Mannherz HG. Actin as target for modification by bacterial protein toxins. Febs J 2011; 278:4526-43; PMID:21466657; http://dx.doi.org/10.1111/j.1742-4658.2011.08113.x
- Barbut F, Gariazzo B, Bonne L, Lalande V, Burghoffer B, Luiuz R, Petit JC. Clinical features of Clostridium difficile-associated infections and molecular characterization of strains: results of a retrospective study, 2000-2004. Infect Control Hosp Epidemiol 2007; 28:131-9; PMID:17265393; http://dx.doi.org/10.1086/511794
- Goldenberg SD, French GL. Lack of association of tcdC type and binary toxin status with disease severity and outcome in toxigenic Clostridium difficile. J Infect 2011; 62:355-62; PMID:21396957; http://dx.doi.org/10.1016/j.jinf.2011.03.001
- Barbut F, Decré D, Lalande V, Burghoffer B, Nousssair L, Gigandon A, Espinasse F, Raskine L, Robert J, Mangeol A, et al. Clinical features of Clostridium difficile-associated diarrhoea due to binary toxin (actin-specfic ADP-ribosyltransferase)-producing strains. J Med Microbiol 2005; 54:181-5; PMID:15673514; http://dx.doi.org/10.1099/jmm.0.45804-0
- Rupnik M. Is Clostridium difficile-associated infection a potentially zoonotic and foodborne disease? Clin Microbiol Infect 2007; 13:457-9; PMID:17331126; http://dx.doi.org/10.1111/j.1469-0691.2007.01687.x
- Gould LH, Limbago B. Clostridium difficile in food and domestic animals: a new foodborne pathogen? Clin Infect Dis 2010; 51:577-82; PMID:20642351; http://dx.doi.org/10.1086/655692
- Gerding DN, Johnson S, Rupnik M, Aktories K. Clostridium difficile binary toxin CDT: Mechanism, epidemiology, and potential clinical importance. Gut Microbes 2014; 5:15-27; PMID:24253566; http://dx.doi.org/10.4161/gmic.26854
- Freeman J, Bauer MP, Baines SD, Corver J, Fawley WN, Goorhuis B, Kuijper EJ, Wilcox MH. The changing epidemiology of Clostridium difficile infections. Clin Microbiol Rev 2010; 23:529-49; PMID:20610822; http://dx.doi.org/10.1128/CMR.00082-09
- Lawley TD, Clare S, Deakin LJ, Goulding D, Yen JL, Raisen C, Brandt C, Lovell J, Cooke F, Clark TG, et al. Use of purified Clostridium difficile spores to facilitate evaluation of health care disinfection regimens. Appl Environ Microbiol 2010; 76:6895-900; PMID:20802075; http://dx.doi.org/10.1128/AEM.00718-10
- Maillard JY. Innate resistance to sporicides and potential failure to decontaminate. J Hosp Infect 2011; 77:204-9; PMID:20850897; http://dx.doi.org/10.1016/j.jhin.2010.06.028
- Weber DJ, Rutala WA, Miller MB, Huslage K, Sickbert-Bennett E. Role of hospital surfaces in the transmission of emerging health care-associated pathogens: norovirus, Clostridium difficile, and Acinetobacter species. Am J Infect Control 2010; 38:S25-33; PMID:20569853; http://dx.doi.org/10.1016/j.ajic.2010.04.196
- Lawley TD, Clare S, Walker AW, Goulding D, Stabler RA, Croucher N, Mastroeni P, Scott P, Raisen C, Mottram L, et al. Antibiotic treatment of Clostridium difficile carrier mice triggers a supershedder state, spore-mediated transmission, and severe disease in immunocompromised hosts. Infect Immun 2009; 77:3661-9; PMID:19564382; http://dx.doi.org/10.1128/IAI.00558-09
- Burns DA, Minton NP. Sporulation studies in Clostridium difficile. J Microbiol Methods 2011; 87:133-8; PMID:21864584; http://dx.doi.org/10.1016/j.mimet.2011.07.017
- Henriques AO, Moran CP, Jr. Structure, assembly, and function of the spore surface layers. Annu Rev Microbiol 2007; 61:555-88; PMID:18035610; http://dx.doi.org/10.1146/annurev.micro.61.080706.093224
- Popham DL, Helin J, Costello CE, Setlow P. Muramic lactam in peptidoglycan of Bacillus subtilis spores is required for spore outgrowth but not for spore dehydration or heat resistance. Proc Natl Acad Sci U S A 1996; 93:15405-10; PMID:8986824; http://dx.doi.org/10.1073/pnas.93.26.15405
- Lawley TD, Croucher NJ, Yu L, Clare S, Sebaihia M, Goulding D, Pickard DJ, Parkhill J, Choudhary J, Dougan G. Proteomic and genomic characterization of highly infectious Clostridium difficile 630 spores. J Bacteriol 2009; 191:5377-86; PMID:19542279; http://dx.doi.org/10.1128/JB.00597-09
- Leski TA, Caswell CC, Pawlowski M, Klinke DJ, Bujnicki JM, Hart SJ, Lukomski S. Identification and classification of bcl genes and proteins of Bacillus cereus group organisms and their application in Bacillus anthracis detection and fingerprinting. Appl Environ Microbiol 2009; 75:7163-72; PMID: 19767469; http://dx.doi.org/10.1128/AEM.01069-09
- Hodgkiss W, Ordal ZJ, Cann DC. The morphology and ultrastructure of the spore and exosporium of some Clostridium species. J Gen Microbiol 1967; 47:213-25; PMID:6045662; http://dx.doi.org/10.1099/00221287-47-2-213
- Barra-Carrasco J, Olguin-Araneda V, Plaza-Garrido A, Miranda-Cardenas C, Cofre-Araneda G, Pizarro-Guajardo M, Sarker MR, Paredes-Sabja D. The Clostridium difficile exosporium cysteine (CdeC)-rich protein is required for exosporium morphogenesis and coat assembly. J Bacteriol 2013; 195:3863-75; PMID:23794627; http://dx.doi.org/10.1128/JB.00369-13
- Sylvestre P, Couture-Tosi E, Mock M. A collagen-like surface glycoprotein is a structural component of the Bacillus anthracis exosporium. Mol Microbiol 2002; 45:169-78; PMID:12100557; http://dx.doi.org/10.1046/j.1365-2958.2000.03000.x
- Brahmbhatt TN, Janes BK, Stibitz ES, Darnell SC, Sanz P, Rasmussen SB, O'Brien AD. Bacillus anthracis exosporium protein BclA affects spore germination, interaction with extracellular matrix proteins, and hydrophobicity. Infect Immun 2007; 75:5233-9; PMID:17709408; http://dx.doi.org/10.1128/IAI.00660-07
- Bozue J, Moody KL, Cote CK, Stiles BG, Friedlander AM, Welkos SL, Hale ML. Bacillus anthracis spores of the bclA mutant exhibit increased adherence to epithelial cells, fibroblasts, and endothelial cells but not to macrophages. Infect Immun 2007; 75:4498-505; PMID:17606596; http://dx.doi.org/10.1128/IAI.00434-07
- Pizarro-Guajardo M, Olguin-Araneda V, Barra-Carrasco J, Brito-Silva C, Sarker MR, Paredes-Sabja D. Characterization of the collagen-like exosporium protein, BclA1, of Clostridium difficile spores. Anaerobe 2014; PMID:24269655
- Escobar-Cortes K, Barra-Carrasco J, Paredes-Sabja D. Proteases and sonication specifically remove the exosporium layer of spores of Clostridium difficile strain 630. J Microbiol Methods 2013; 93:25-31; PMID:23384826; http://dx.doi.org/10.1016/j.mimet.2013.01.016
- Paredes-Sabja D, Sarker MR. Adherence of Clostridium difficile spores to Caco-2 cells in culture. J Med Microbiol 2012; 61:1208-18; PMID:22595914; http://dx.doi.org/10.1099/jmm.0.043687-0
- Sebaihia M, Wren BW, Mullany P, Fairweather NF, Minton N, Stabler R, Thomson NR, Roberts AP, Cerdeno-Tarraga AM, Wang H, et al. The multidrug-resistant human pathogen Clostridium difficile has a highly mobile, mosaic genome. Nat Genet 2006; 38:779-86; PMID:16804543; http://dx.doi.org/10.1038/ng1830
- Permpoonpattana P, Phetcharaburanin J, Mikelsone A, Dembek M, Tan S, Brisson MC, La Ragione R, Brisson AR, Fairweather N, Hong HA, et al. Functional characterization of Clostridium difficile spore coat proteins. J Bacteriol 2013; 195:1492-503; PMID:23335421; http://dx.doi.org/10.1128/JB.02104-12
- Permpoonpattana P, Tolls EH, Nadem R, Tan S, Brisson A, Cutting SM. Surface layers of Clostridium difficile endospores. J Bacteriol 2011; 193:6461-70; PMID:21949071; http://dx.doi.org/10.1128/JB.05182-11
- Francis MB, Allen CA, Shrestha R, Sorg JA. Bile acid recognition by the Clostridium difficile germinant receptor, CspC, is important for establishing infection. PLoS Pathog 2013; 9:e1003356; PMID:23675301; http://dx.doi.org/10.1371/journal.ppat.1003356
- Putnam EE, Nock AM, Lawley TD, Shen A. SpoIVA and SipL are Clostridium difficile spore morphogenetic proteins. J Bacteriol 2013; 195:1214-25; PMID:23292781; http://dx.doi.org/10.1128/JB.02181-12
- Abhyankar W, Hossain AH, Djajasaputra A, Permpoonpattana P, Ter Beek A, Dekker HL, Cutting SM, Brul S, de Koning LJ, de Koster CG. In pursuit of protein targets: proteomic characterization of bacterial spore outer layers. J Proteome Res 2013; 12:4507-21; PMID:23998435; http://dx.doi.org/10.1021/pr4005629
- Heap JT, Pennington OJ, Cartman ST, Carter GP, Minton NP. The ClosTron: a universal gene knock-out system for the genus Clostridium. J Microbiol Methods 2007; 70:452-64; PMID:17658189; http://dx.doi.org/10.1016/j.mimet.2007.05.021
- Deakin LJ, Clare S, Fagan RP, Dawson LF, Pickard DJ, West MR, Wren BW, Fairweather NF, Dougan G, Lawley TD. The Clostridium difficile spo0A Gene Is a Persistence and Transmission Factor. Infect Immun 2012; 80:2704-11; PMID:22615253; http://dx.doi.org/10.1128/IAI.00147-12
- Donskey CJ. Preventing transmission of Clostridium difficile: is the answer blowing in the wind? Clin Infect Dis 2010; 50:1458-61; PMID:20415566; http://dx.doi.org/10.1086/652649
- Joshi LT, Phillips DS, Williams CF, Alyousef A, Baillie L. Contribution of spores to the ability of Clostridium difficile to adhere to surfaces. Appl Environ Microbiol 2012; 78:7671-9; PMID:22923404; http://dx.doi.org/10.1128/AEM.01862-12
- Verity P, Wilcox MH, Fawley W, Parnell P. Prospective evaluation of environmental contamination by Clostridium difficile in isolation side rooms. J Hosp Infect 2001; 49:204-9; PMID:11716638; http://dx.doi.org/10.1053/jhin.2001.1078
- Eveillard M, Fourel V, Barc M-C, Kerneis S, Coconnier MH, Karjalainen T, Bourlioux P, Servin A. Identification and characterization of adhesive factors of Clostridium difficile involved in adhesion to human colonic enterocyte-like Caco-2 and mucus-secreting HT29 cells in culture. Mol Microbiol 1993; 7:371-81; PMID:8459765; http://dx.doi.org/10.1111/j.1365-2958.1993.tb01129.x
- Sorg JA, Sonenshein AL. Bile salts and glycine as cogerminants for Clostridium difficile spores. J Bacteriol 2008; 190:2505-12; PMID:18245298; http://dx.doi.org/10.1128/JB.01765-07
- Francis MB, Allen CA, Sorg JA. Muricholic acids inhibit Clostridium difficile spore germination and growth. PLoS One 2013; 8:e73653; http://dx.doi.org/10.1371/annotation/1e464689-3c86-4399-b229-1e00d65593a5
- Sorg JA, Sonenshein AL. Inhibiting the initiation of Clostridium difficile spore germination using analogs of chenodeoxycholic acid, a bile acid. J Bacteriol 2010; 192:4983-90; PMID:20675492; http://dx.doi.org/10.1128/JB.00610-10
- Sethi AK, Al-Nassir WN, Nerandzic MM, Bobulsky GS, Donskey CJ. Persistence of skin contamination and environmental shedding of Clostridium difficile during and after treatment of C. difficile infection. Infect Control Hosp Epidemiol 2010; 31:21-7; PMID:19929371; http://dx.doi.org/10.1086/649016
- Riggs MM, Sethi AK, Zabarsky TF, Eckstein EC, Jump RL, Donskey CJ. Asymptomatic carriers are a potential source for transmission of epidemic and nonepidemic Clostridium difficile strains among long-term care facility residents. Clin Infect Dis 2007; 45:992-8; PMID:17879913; http://dx.doi.org/10.1086/521854
- Mackin KE, Carter GP, Howarth P, Rood JI, Lyras D. Spo0A differentially regulates toxin production in evolutionarily diverse strains of Clostridium difficile. PLoS One 2013; 8:e79666; PMID:24236153; http://dx.doi.org/10.1371/journal.pone.0079666
- Stephenson K, Hoch JA. Evolution of signalling in the sporulation phosphorelay. Mol Microbiol 2002; 46:297-304; PMID:12406209; http://dx.doi.org/10.1046/j.1365-2958.2002.03186.x
- Saujet L, Pereira FC, Serrano M, Soutourina O, Monot M, Shelyakin PV, Gelfand MS, Dupuy B, Henriques AO, Martin-Verstraete I. Genome-wide analysis of cell type-specific gene transcription during spore formation in Clostridium difficile. PLoS Genet 2013; 9:e1003756; PMID:24098137; http://dx.doi.org/10.1371/journal.pgen.1003756
- Fimlaid KA, Bond JP, Schutz KC, Putnam EE, Leung JM, Lawley TD, Shen A. Global analysis of the sporulation pathway of Clostridium difficile. PLoS Genet 2013; 9:e1003660; PMID:23950727; http://dx.doi.org/10.1371/journal.pgen.1003660
- Pereira FC, Saujet L, Tome AR, Serrano M, Monot M, Couture-Tosi E, Martin-Verstraete I, Dupuy B, Henriques AO. The spore differentiation pathway in the enteric pathogen Clostridium difficile. PLoS Genet 2013; 9:e1003782; PMID:24098139; http://dx.doi.org/10.1371/journal.pgen.1003782
- Sara M, Sleytr UB. S-Layer proteins. J Bacteriol 2000; 182:859-68; PMID:10648507; http://dx.doi.org/10.1128/JB.182.4.859-868.2000
- Dang TH, de la Riva L, Fagan RP, Storck EM, Heal WP, Janoir C, Fairweather NF, Tate EW. Chemical probes of surface layer biogenesis in Clostridium difficile. ACS Chem Biol 2010; 5:279-85; PMID:20067320; http://dx.doi.org/10.1021/cb9002859
- Calabi E, Fairweather N. Patterns of sequence conservation in the S-Layer proteins and related sequences in Clostridium difficile. J Bacteriol 2002; 184:3886-97; PMID:12081960; http://dx.doi.org/10.1128/JB.184.14.3886-3897.2002
- Fagan RP, Albesa-Jove D, Qazi O, Svergun DI, Brown KA, Fairweather NF. Structural insights into the molecular organization of the S-layer from Clostridium difficile. Mol Microbiol 2009; 71:1308-22; PMID:19183279; http://dx.doi.org/10.1111/j.1365-2958.2009.06603.x
- Calabi E, Calabi F, Phillips AD, Fairweather NF. Binding of Clostridium difficile surface layer proteins to gastrointestinal tissues. Infect Immun 2002; 70:5770-8; PMID:12228307; http://dx.doi.org/10.1128/IAI.70.10.5770-5778.2002
- Merrigan MM, Venugopal A, Roxas JL, Anwar F, Mallozzi MJ, Roxas BA, Gerding DN, Viswanathan VK, Vedantam G. Surface-Layer Protein A (SlpA) Is a Major Contributor to Host-Cell Adherence of Clostridium difficile. PLoS One 2013; 8:e78404; PMID:24265687; http://dx.doi.org/10.1371/journal.pone.0078404
- Ryan A, Lynch M, Smith SM, Amu S, Nel HJ, McCoy CE, Dowling JK, Draper E, O'Reilly V, McCarthy C, et al. A role for TLR4 in Clostridium difficile infection and the recognition of surface layer proteins. PLoS Pathog 2011; 7:e1002076; PMID:21738466; http://dx.doi.org/10.1371/journal.ppat.1002076
- Bianco M, Fedele G, Quattrini A, Spigaglia P, Barbanti F, Mastrantonio P, Ausiello CM. Immunomodulatory activities of surface-layer proteins obtained from epidemic and hypervirulent Clostridium difficile strains. J Med Microbiol 2011; 60:1162-7; PMID:21349985; http://dx.doi.org/10.1099/jmm.0.029694-0
- Sebaihia M, Peck MW, Minton NP, Thomson NR, Holden MT, Mitchell WJ, Carter AT, Bentley SD, Mason DR, Crossman L, et al. Genome sequence of a proteolytic (Group I) Clostridium botulinum strain Hall A and comparative analysis of the clostridial genomes. Genome Res 2007; 17:1082-92; PMID:17519437; http://dx.doi.org/10.1101/gr.6282807
- Emerson JE, Fairweather N. Surface structures of C. difficile and other clostridia. In: Bruggemann H, Gottschalk G. ed. Clostridia - Molecular Biology in the Post-genomic Era. Norfolk, UK: Caister Academic Press, 2009:157-67.;
- Karjalainen T, Waligora-Dupriet AJ, Cerquetti M, Spigaglia P, Maggioni A, Mauri P, Mastrantonio P. Molecular and genomic analysis of genes encoding surface-anchored proteins from Clostridium difficile. Infect Immun 2001; 69:3442-6; PMID:11292772; http://dx.doi.org/10.1128/IAI.69.5.3442-3446.2001
- Dingle KE, Didelot X, Ansari MA, Eyre DW, Vaughan A, Griffiths D, Ip CL, Batty EM, Golubchik T, Bowden R, et al. Recombinational switching of the Clostridium difficile S-layer and a novel glycosylation gene cluster revealed by large-scale whole-genome sequencing. J Infect Dis 2013; 207:675-86; PMID:23204167; http://dx.doi.org/10.1093/infdis/jis734
- Biazzo M, Cioncada R, Fiaschi L, Tedde V, Spigaglia P, Mastrantonio P, Pizza M, Barocchi MA, Scarselli M, Galeotti CL. Diversity of cwp loci in clinical isolates of Clostridium difficile. J Med Microbiol 2013; 62:1444-52; PMID:23722432; http://dx.doi.org/10.1099/jmm.0.058719-0
- Wright A, Wait R, Begum S, Crossett B, Nagy J, Brown K, Fairweather N. Proteomic analysis of cell surface proteins from Clostridium difficile. Proteomics 2005; 5:2443-52; PMID:15887182; http://dx.doi.org/10.1002/pmic.200401179
- Emerson JE, Stabler RA, Wren BW, Fairweather NF. Microarray analysis of the transcriptional responses of Clostridium difficile to environmental and antibiotic stress. J Med Microbiol 2008; 57:757-64; PMID:18480334; http://dx.doi.org/10.1099/jmm.0.47657-0
- Wright A, Drudy D, Kyne L, Brown K, Fairweather NF. Immunoreactive cell wall proteins of Clostridium difficile identified by human sera. J Med Microbiol 2008; 57:750-6; PMID:18480333; http://dx.doi.org/10.1099/jmm.0.47532-0
- de la Riva L, Willing SE, Tate EW, Fairweather NF. Roles of cysteine proteases Cwp84 and Cwp13 in biogenesis of the cell wall of Clostridium difficile. J Bacteriol 2011; 193:3276-85; PMID:21531808; http://dx.doi.org/10.1128/JB.00248-11
- Janoir C, Pechine S, Grosdidier C, Collignon A. Cwp84, a surface-associated protein of Clostridium difficile, is a cysteine protease with degrading activity on extracellular matrix proteins. J Bacteriol 2007; 189:7174-80; PMID:17693508; http://dx.doi.org/10.1128/JB.00578-07
- ChapetonMontes D, Candela T, Collignon A, Janoir C. Localization of the Clostridium difficile cysteine protease Cwp84 and insights into its maturation process. J Bacteriol 2011; 193:5314-21; PMID:21784932; http://dx.doi.org/10.1128/JB.00326-11
- Waligora AJ, Hennequin C, Mullany P, Bourlioux P, Collignon A, Karjalainen T. Characterization of a cell surface protein of Clostridium difficile with adhesive properties. Infect Immun 2001; 69:2144-53; PMID: 11254569; http://dx.doi.org/10.1128/IAI.69.4.2144-2153.2001
- Emerson JE, Reynolds CB, Fagan RP, Shaw HA, Goulding D, Fairweather NF. A novel genetic switch controls phase variable expression of CwpV, a Clostridium difficile cell wall protein. Mol Microbiol 2009; 74:541-56; PMID:19656296; http://dx.doi.org/10.1111/j.1365-2958.2009.06812.x
- Reynolds CB, Emerson JE, de la Riva L, Fagan RP, Fairweather NF. The Clostridium difficile cell wall protein CwpV is antigenically variable between strains, but exhibits conserved aggregation-promoting function. PLoS Pathog 2011; 7:e1002024; PMID:21533071; http://dx.doi.org/10.1371/journal.ppat.1002024
- Monteiro MA, Ma Z, Bertolo L, Jiao Y, Arroyo L, Hodgins D, Mallozzi M, Vedantam G, Sagermann M, Sundsmo J, et al. Carbohydrate-based Clostridium difficile vaccines. Expert Rev Vaccines 2013; 12:421-31; PMID:23560922; http://dx.doi.org/10.1586/erv.13.9
- Martin CE, Broecker F, Eller S, Oberli MA, Anish C, Pereira CL, Seeberger PH. Glycan arrays containing synthetic Clostridium difficile lipoteichoic acid oligomers as tools toward a carbohydrate vaccine. Chem Commun (Camb) 2013; 49:7159-61; PMID:23836132; http://dx.doi.org/10.1039/c3cc43545h
- Oberli MA, Hecht ML, Bindschadler P, Adibekian A, Adam T, Seeberger PH. A possible oligosaccharide-conjugate vaccine candidate for Clostridium difficile is antigenic and immunogenic. Chem Biol 2011; 18:580-8; PMID:21609839; http://dx.doi.org/10.1016/j.chembiol.2011.03.009
- Romano MR, Leuzzi R, Cappelletti E, Tontini M, Nilo A, Proietti D, Berti F, Costantino P, Adamo R, Scarselli M. Recombinant Clostridium difficile toxin fragments as carrier protein for PSII surface polysaccharide preserve their neutralizing activity. Toxins (Basel) 2014; 6:1385-96; PMID:24759173; http://dx.doi.org/10.3390/toxins6041385
- Borriello S P, Davies HA, Barclay FE. Detection of fimbriae amongst strains of Clostridium difficile. FEMS Microbiol Lett 1988; 49:65-7; http://dx.doi.org/10.1111/j.1574-6968.1988.tb02683.x
- Taha S, Johansson O, Rivera Jonsson S, Heimer D, Krovacek K. Toxin production by and adhesive properties of Clostridium difficile isolated from humans and horses with antibiotic-associated diarrhea. Comp Immunol Microbiol Infect Dis 2007; 30:163-74; PMID:17239950; http://dx.doi.org/10.1016/j.cimid.2006.11.006
- Melville S, Craig L. Type IV pili in Gram-positive bacteria. Microbiol Mol Biol Rev 2013; 77:323-41; PMID:24006467; http://dx.doi.org/10.1128/MMBR.00063-12
- Maldarelli GA, De Masi L, von Rosenvinge EC, Carter M, Donnenberg MS. Identification, immunogenicity, and cross-reactivity of type IV pilin and pilin-like proteins from Clostridium difficile. Pathog Dis 2014; PMID:24550179
- Pituch H, Obuch-Woszczatynski P, van den Braak N, van Belkum A, Kujawa M, Luczak M, Meisel-Mikolajczyk F. Variable flagella expression among clonal toxin A−/B+ Clostridium difficile strains with highly homogeneous flagellin genes. Clin Microbiol Infect 2002; 8:187-8; PMID:12010175; http://dx.doi.org/10.1046/j.1469-0691.2002.00394.x
- Tasteyre A, Karjalainen T, Avesani V, Delmeé M, Collignon A, Bourlioux P, Barc M-C. Phenotypic and genotypic diversity of the flagellin gene (fliC) among Clostridium difficile isolates from different serogroups. J Clin Microbiol 2000; 38:3179-86; PMID:10970353
- Tasteyre A, Karjalainen T, Avesani V, Delmee M, Collignon A, Bourlioux P, Barc MC. Molecular characterization of fliD gene encoding flagellar cap and its expression among Clostridium difficile isolates from different serogroups. J Clin Microbiol 2001; 39:1178-83; PMID:11230454; http://dx.doi.org/10.1128/JCM.39.3.1178-1183.2001
- Dingle TC, Mulvey GL, Armstrong GD. Mutagenic analysis of the Clostridium difficile flagellar proteins, FliC and FliD, and their contribution to virulence in hamsters. Infect Immun 2011; 79:4061-7; PMID:21788384; http://dx.doi.org/10.1128/IAI.05305-11
- Baban ST, Kuehne SA, Barketi-Klai A, Cartman ST, Kelly ML, Hardie KR, Kansau I, Collignon A, Minton NP. The Role of Flagella in Clostridium difficile Pathogenesis: Comparison between a Non-Epidemic and an Epidemic Strain. PLoS One 2013; 8:e73026; PMID:24086268; http://dx.doi.org/10.1371/journal.pone.0073026
- Aubry A, Hussack G, Chen W, KuoLee R, Twine SM, Fulton KM, Foote S, Carrillo CD, Tanha J, Logan SM. Modulation of toxin production by the flagellar regulon in Clostridium difficile. Infect Immun 2012; 80:3521-32; PMID:22851750; http://dx.doi.org/10.1128/IAI.00224-12
- Ethapa T, Leuzzi R, Ng YK, Baban ST, Adamo R, Kuehne SA, Scarselli M, Minton NP, Serruto D, Unnikrishnan M. Multiple factors modulate biofilm formation by the anaerobic pathogen Clostridium difficile. J Bacteriol 2013; 195:545-55; PMID:23175653; http://dx.doi.org/10.1128/JB.01980-12
- Cerquetti M, Serafino A, Sebastianelli A, Mastrantonio P. Binding of Clostridium difficile to Caco-2 epithelial cell line and to extracellular matrix proteins. Fed Euro Microbiol Soc Immunol Med Microbiol 2002; 32:211-8; http://dx.doi.org/10.1111/j.1574-695X.2002.tb00556.x
- Hennequin C, Janoir C, Barc MC, Collignon A, Karjalainen T. Identification and characterization of a fibronectin-binding protein from Clostridium difficile. Microbiology 2003; 149:2779-87; PMID:14523111; http://dx.doi.org/10.1099/mic.0.26145-0
- Lin YP, Kuo CJ, Koleci X, McDonough SP, Chang YF. Manganese binds to Clostridium difficile Fbp68 and is essential for fibronectin binding. J Biol Chem 2011; 286:3957-69; PMID:21062746; http://dx.doi.org/10.1074/jbc.M110.184523
- Barketi-Klai A, Hoys S, Lambert-Bordes S, Collignon A, Kansau I. Role of fibronectin-binding protein A in Clostridium difficile intestinal colonization. J Med Microbiol 2011; 60:1155-61; PMID:21349990; http://dx.doi.org/10.1099/jmm.0.029553-0
- Cafardi V, Biagini M, Martinelli M, Leuzzi R, Rubino JT, Cantini F, Norais N, Scarselli M, Serruto D, Unnikrishnan M. Identification of a Novel Zinc Metalloprotease through a Global Analysis of Clostridium difficile Extracellular Proteins. PLoS One 2013; 8:e81306; PMID:24303041; http://dx.doi.org/10.1371/journal.pone.0081306
- Duesbery NS, Webb CP, Leppla SH, Gordon VM, Klimpel KR, Copeland TD, Ahn NG, Oskarsson MK, Fukasawa K, Paull KD, et al. Proteolytic inactivation of MAP-kinase-kinase by anthrax lethal factor. Science 1998; 280:734-7; PMID:9563949; http://dx.doi.org/10.1126/science.280.5364.734
- Tulli L, Marchi S, Petracca R, Shaw HA, Fairweather NF, Scarselli M, Soriani M, Leuzzi R. CbpA: a novel surface exposed adhesin of Clostridium difficile targeting human collagen. Cell Microbiol 2013; 15:1674-87; PMID:23517059
- Hennequin C, Collignon A, Karjalainen T. Analysis of expression of GroEL (Hsp60) of Clostridium difficile in response to stress. Microbial Pathogenesis 2001; 31:255-60; PMID:11710845; http://dx.doi.org/10.1006/mpat.2001.0468
- Hennequin C, Porcheray F, Waligora-Dupriet A, Collignon A, Barc M, Bourlioux P, Karjalainen T. GroEL (Hsp60) of Clostridium difficile is involved in cell adherence. Microbiology 2001; 147:87-96; PMID:11160803
- Pechine S, Hennequin C, Boursier C, Hoys S, Collignon A. Immunization Using GroEL Decreases Clostridium difficile Intestinal Colonization. PLoS One 2013; 8:e81112; PMID:24303034; http://dx.doi.org/10.1371/journal.pone.0081112
- Prevention CDC. Antibiotic resistance threats in the United States, 2013. Threat Report 2013:50-2.;
- Gandra S, Ellison RT, 3rd. Modern Trends in Infection Control Practices in Intensive Care Units. J Intensive Care Med 2013; PMID:23753240
- Howerton A, Patra M, Abel-Santos E. A new strategy for the prevention of Clostridium difficile infection. J Infect Dis 2013; 207:1498-504; PMID:23420906; http://dx.doi.org/10.1093/infdis/jit068
- Hutton ML, Mackin KE, Chakravorty A, Lyras D. Small animal models for the study of Clostridium difficile disease pathogenesis. FEMS Microbiol Lett 2013; 352:140-9; PMID:24372713; http://dx.doi.org/10.1111/1574-6968.12367