Abstract
Numerous large scale genomics studies have demonstrated that cancer is a molecularly heterogeneous disease, characterized by acquired changes in the structure and DNA sequence of tumor genomes. More recently, the role of the equally complex tumor microenvironment in driving the aggressiveness of this disease is increasingly being realized. Tumor cells are surrounded by activated stroma, creating a dynamic environment that promotes cancer development, metastasis and chemoresistance. The Rho family of small GTPases plays an essential role in the regulation of cell shape, cytokinesis, cell adhesion, and cell motility. Importantly, these processes need to be considered in the context of a complex 3-dimensional (3D) environment, with reciprocal feedback and cross-talk taking place between the tumor cells and host environment. Here we discuss the role of molecular networks involving Rho GTPases in cancer, and the therapeutic implications of inhibiting Rho signaling in both cancer cells and the emerging concept of targeting the surrounding stroma.
Introduction
Over the last 2 decades, a deeper understanding of the genetic and molecular basis of cancer has led to new classes of therapies to selectively target the molecular mechanisms that affect survival and proliferation of cancer cells. Large-scale genomics efforts are providing new opportunities to improve current approaches to cancer therapy.Citation1-3 However, contribution of the equally complex and dynamic tumor microenvironment to therapeutic resistance is increasingly being realized. For example, stromal cells have previously been shown to directly confer chemoresistance to a variety of agents, particularly influencing the response to targeted therapies.Citation4 In several solid cancer types,Citation5,6 including those of the prostate, pancreas and ovary, a high proportion of the total tumor mass consists of activated (myo)fibroblasts, lymphatic and vascular endothelial cells, immune cells and extracellular matrix (ECM), all of which make up the tumor stromal environment. This dynamic tumor microenvironment promotes cancer initiation, progression, metastasis and chemoresistance, creating a substantial barrier to reducing the morbidity and mortality that is attributable to aggressive malignancies. At the same time, it has created new avenues for the development and testing of novel therapeutic strategies, by targeting cellular mechanisms contributed by the tumor microenvironment.
Rho GTPases comprise a branch of the Ras super family, encompassing 22 genes in humans, of which RhoA, Rac1 and Cdc42 are the best characterized. They play essential roles in a number of biological processes, especially in the regulation of cell morphology, cytokinesis, cell adhesion, and cell migration. Alternation between their GDP-bound (inactive) and GTP-bound (active) forms is a tightly regulated process. GTPase activating proteins (GAPs) stimulate GTP hydrolysis and inactivation, while the guanine nucleotide exchange factors (GEFs) facilitate GDP dissociation and activate downstream pathways through effector proteins.Citation7
Activation of Rho GTPases results in binding to downstream effector proteins, for example the Rho-associated protein kinase ROCK, and interaction with various well characterized pathways, including the PI3K, FAK, Src, LIMK, MEK/Erk protein networks,Citation8-12 leading to actin cytoskeleton remodeling and increased cell motility. These key processes are also critical during cell cycle progression and mitosis, including the process of cell rounding at mitosis onset, during chromosomal alignment and the contraction of the actomyosin ring that separates daughter cells at cytokinesis, all of which are tightly regulated by the Rho family of GTPases in a cell type specific manner.Citation13-15
The effect of Rho/ROCK activation on myosin-light chain (MLC) phosphorylation in smooth muscle and its involvement in the maintenance of aspects of stromal feedback such as vascular tone,Citation16-18 as well as on ECM deposition, has generated considerable interest in the use of Rho pathway inhibition to treat cardiovascular disease,Citation17 but also for the treatment of stroke,Citation19 inflammatory conditions,Citation20 Alzheimer's diseaseCitation21,22 and neuropathic pain.Citation23-25 Specifically, several studies have shown improved recovery from spinal cord injury in rat models of the disease following treatment with the ROCK inhibitors fasudil and Y-27632.Citation23,24,26 Regulation of mechanical and contractile properties of the pressure-sensitive smooth muscle cells is recognized to play a significant role in blood pressure homeostasis and regulation of vascular tone.Citation18 Interestingly, due to its vasodilatory effect, fasudil is clinically used for the treatment of cerebral vasospasm.Citation27 Y-27632 is a pre-clinical molecular tool, with newer selective RhoA/ROCK inhibitors also in development, including H-1152 and aminofurazan compounds.Citation28,29 The potential for the application of downstream Rho signaling inhibitors from the pathologies outlined above to target the ECM, vascular alterations as well as tumor-specific changes in a variety of cancer types and therefore, re-purposing as cancer therapy, will be discussed in this review.
Rho GTPase Signaling in Cancer
To promote transformation, cancer development, invasion and metastasis, tumor cells frequently hijack the multilayered and dynamic regulation of Rho GTPase activity that is required for coordinated cell migration under physiological conditions.Citation30 Increased levels of RhoA, RhoB, RhoC, Rac1, Cdc42 and ROCK, have been found in late-stage tumors and metastasesCitation31-35 with prognostic relevance in breast cancer.Citation32 Interestingly, the RhoGAP Dlc1 was found to regulate the metastatic colonization of circulating breast cancer cells in bone, but not lungs of the MDA-MB-231 orthotopic in vivo model of breast cancer.Citation36 In the same study, this organ-specific metastatic phenotype was further attributed to the Dlc1-Rho regulation of the response of cancer cells to TGF-β stimulation from the bone stroma and the subsequent remodeling of the osteolytic microenvironment for metastatic colonization. Moreover, Dlc1 suppressed the formation of bone but not lung metastasis by inhibiting TGF-β-induced bone degradation via PTHLH,Citation36 a critical regulator of osteoclastogenesis.Citation37 Hence, targeting the Rho-ROCK signaling axis in this molecular setting could provide a more effective approach for the treatment of breast-to-bone-metastasis than by directly inhibiting TGF-β and importantly, specific tumor suppressor functions of this protein.Citation38
Increased expression of Rho/ROCK proteins has also been detected in pancreatic cancer,Citation39 testicular germ cell tumors,Citation40 squamous cell carcinomasCitation41 and several other cancer types.Citation42-44 Constitutive overexpression of RhoA in tumor cells leads to increased translocation of this protein to the cell membrane, where it is activated and causes increased tumor invasion.Citation45 Similarly, RhoC facilitates tumor cell invasion and promotes metastasis in breast and pancreatic cancer.Citation34,35 Overexpression of Cdc42 in a tetracycline-inducible MMTV-driven mouse model was found to disrupt mammary gland branching morphogenesis by changes in Rho GTPase and MAPK signaling, leading to increased contractility and migration in association with further stromal alterations, including elevated ECM deposition.Citation46
A delicate balance between Rac and Rho signaling governs the diversity of tumor cell invasion mechanisms (reviewed inCitation47). For example, in Matrigel invasion assays RhoA/ROCK activity promotes the amoeboid motility of rounded cells, while Rac regulates migration of elongated cells that depend on the proteolysis and remodeling of the stromal ECM.Citation48 Cancer cells can readily switch between these 2 modes and importantly, with combined inhibition of proteolysis and Rho/ROCK signaling, the switch was effectively impaired and cancer cell invasion was inhibited.Citation48 Similarly, only dual inhibition of the Rac1 and RhoA signaling axes significantly decreased invasive potential of fibrosarcoma cells in 3D matricesCitation49 or in vivo tumor growth of selected orthotopic xenografts of pancreatic cancer.Citation50 However, this observation appears to be context- and or tumor type-dependent. For example, a marked decrease of active Rac1 and Cdc42 correlated with the high invasive potential of tumor cell lines isolated from metastatic sites of colorectal adenocarcinoma.Citation51 Moreover, the combined activation of Rac1/Cdc42 signaling and inhibition of ROCK in this model, with PDGF and Y-27632 treatment, significantly decreased the invasive potential of colorectal cancer cells and this effect was accompanied by the re-establishment of E-cadherin-dependent adherens junctions.Citation51
In addition to their role as master regulators of cellular processes that contribute to cell motility, including protrusion formation, adhesion remodeling, and contractility, Rho GTPases have also been implicated in the regulation of the G1 cell cycle checkpoint activation,Citation52 malignant transformation,Citation53-55 tumor angiogenesis,Citation56 chemoresistanceCitation57,58 and inflammation.Citation59,60 Epithelial-mesenchymal transition (EMT), which is characterized by the transformation of tumor cells from an epithelial to a mesenchymal phenotype correlates with the acquisition of invasive and metastatic properties in cancer. Through interaction with key components of the aberrantly activated Wnt/β -catenin signaling pathway, Rho GTPases have also been shown to coordinate activation of specific receptor tyrosine kinases and promote EMT in several cancer types.Citation61-63 Key aspects of how this family of proteins regulate cancer development and progression have recently been reviewed.Citation64
Rho GTPase Mutations in Cancer: Implications for Therapeutic Targeting
Historically, large scale sequencing efforts have revealed that mutations in the Rho GTPase family are rare, where generally aberrant activation of this pathway occurs through overexpression of Rho GTPases or by changes in the levels of regulators of Rho activity, including increased activation of GEFsCitation65-68 and inactivation or loss of GAPsCitation69,70 or GDIs.Citation71-73 However, increasing evidence indicates that certain cancers harbor significant genomic aberrations in this complex signaling network. For example, an early sequencing study of B-cell diffuse large-cell lymphomas (DLCL) has demonstrated that a significant proportion (46%) of tumors carried mutations in the RHOH/TTF gene, which encodes a small GTP-binding protein of the RAS superfamily.Citation74 The identified sequence variants included largely single base substitutions and were scattered throughout the first 1.6 kb of the RHOH/TTF gene, within non-coding sequences, thus suggesting a potential effect on the regulation of RHOH/TTF gene expression in subtypes of DLCL.Citation74 Recurrent chromosomal alterations of the RHOH/TTF gene at band 4p13 have also been detected in non-Hodgkins lymphoma and multiple myeloma.Citation75
Most recently, 2 independent exome and transcriptome sequencing studies have revealed a frequent somatic mutation in the RHOA gene (p.Gly17Val) which occurs in 53–68% of angioimmunoblastic T cell lymphomas (AITL).Citation76,77 Yoo et al.Citation77 further showed that this mutation was specific to T cell lymphoma and was absent from B cell lymphoma. Importantly, these seminal works were the first to demonstrate that the RHOA p.Gly17Val substitution in the GTP-binding domain leads to dramatically reduced GTP and GTPγS binding, impaired RhoA function, contributing to AITL-specific pathogenesis.Citation76,77 Since AITL is a common subtype of T cell lymphoma and a disease with very poor prognosis and 5-year overall survival of only 33%,Citation78 future studies on the detailed molecular characterization of the RHOA p.Gly17Val mutation may hold important implications for the development of novel, clinically useful diagnostic biomarkers and therapeutic targets.
In contrast with haematological malignancies, the contribution of genomic aberrations in Rho GTPase family members to carcinogenesis and disease progression in solid cancers is less understood. Interestingly, a recent comprehensive molecular characterization of 295 primary gastric adenocarcinomas as part of The Cancer Genome Atlas (TCGA) project revealed mutations in RHOA gene in 5.5% of gastric tumors.Citation79 RHOA mutations were enriched in a specific subtype of gastric cancer, preferentially occurring in cases classified as genomically stable and appeared to cluster in 2 adjacent amino-terminal regions of RhoA that are predicted to be at the interface of RhoA with ROCK1 and other effectors, thus potentially modulating downstream signaling.Citation79
Another recent study on the mutational landscape in melanoma has identified a recurrent activating mutation in the Rho GTPase RAC1 in 9.2% of sun-exposed melanomas.Citation80 This somatic missense mutation leads to a change from proline 29 to serine in the highly conserved switch I domain of Rac1, causing increased activation of downstream signaling, melanocyte proliferation and migration.Citation80,81 In parallel, Hodis et al.Citation82 have identified the RAC1 (P29S) mutation as the most frequent hot spot mutation after those in BRAF and NRAS in an independent melanoma patient cohort. In addition, mutations in homologous residues in RAC2 (P29L) and RHOT1 (P30L) were also detected, albeit at very low frequency (0.8%), suggesting the importance of the P29 residue as a possible codon targeted by hot spot mutations in Rho family GTPases.Citation82 A well-characterized RAS family-activating mutation (G12D) in the CDC42 gene was also identified in a melanoma patient.Citation82 Moreover, Matos et al.Citation83 have shown that Rac1b, a hyperactive splice variant of the RAC1 gene, and B-Raf(V600E) mutation functionally cooperate to sustain colorectal tumor cell viability, suggesting an alternative survival pathway to oncogenic K-Ras in these tumors. A personalized treatment strategy using pharmacological inhibition of Rac1 signaling in tumor subtypes carrying these aberrations could be beneficial and remains to be examined. Importantly, as integration of molecular data, including DNA copy-number alteration, mRNA and protein, metabolomic and clinical information, becomes routine research practice, delineating the extent of the deregulation of Rho GTPases in cancer will pave the way for the more accurate and rapid implementation of the inhibitors of Rho signaling as personalized cancer therapeutics.
Dynamics of Rho GTPase Signaling in Living Systems
The application of Förster resonance energy transfer (FRET) imaging for the study of molecular dynamics in living cells has dramatically improved our current understanding of the spatiotemporal regulation of Rho activation.Citation13,84-87 Comprehensive studies have demonstrated that different extracellular cues induce distinct patterns of RhoA/Rac1 signaling during membrane protrusion in migrating Mouse Embryo Fibroblast (MEF) cultures in vitro.Citation84-86 Although the mechanisms regulating single-cell migration and Rho activation in vitro are relatively well understood, it is necessary to understand the intricacy of Rho signaling in live tissue. Consequently, key studies using FRET have been performed in various multicellular organisms, increasing in complexity from the transparent DrosophilaCitation88,89 and Zebrafish modelsCitation90,91 to technically challenging in vivo mouse imaging.Citation87,92,93 For example, the GEF Vav was identified as a key regulator of Rac1 activity during guided cell morphogenetic movements in the developing Drosophila embryo.Citation88 Further, in vivo FRET has also revealed the requirement for Rac1 and RhoA activity in the regulation of chemokine-guided germ cell motility within Zebrafish embryos, where Rac1 was essential for the formation of actin-rich structures, with RhoA promoting retrograde actin flow.Citation90 Although these studies have collectively revealed new detail regarding the synchronized and coordinated role of Rho GTPases in fundamental developmental and disease processes, Rho signaling should also be examined in a mammalian 3-dimensional (3D) tissue environment. For example, in cancer, it is well established that continuous and reciprocal cross-talk takes place between the tumor cells and the host environment.
Using FRET imaging in a live animal model of invasive pancreatic ductal adenocarcinoma (PDAC, Pdx1-Cre, LSL-KRasG12D/+, LSL-Trp53R172H/+; KPC model) we have previously identified distinct sub-cellular patterns of RhoA activity during tumor invasion.Citation87 Moreover, therapeutic intervention with dasatinib, an anti-invasive agent that inhibits c-Src tyrosine kinase, specifically inactivated RhoA activity at the poles of the invading cells, providing a new level of detail regarding the regulation of RhoA during cell-ECM interactions in cancer.Citation94,95 It is also plausible that the spatiotemporal regulation of other Rho GTPases will similarly be tightly controlled.Citation87 For example, the relative balance of Rac1, Cdc42 and RhoA activities was found to directly affect the invasiveness of glioblastoma cells at perivascular and intraparenchymal regions of the brain in a C6 allograft glioblastoma model.Citation92
Using the K14-ROCK:ER genetically engineered mouse (GEM), it was also recently shown that conditional activation of Rho/ROCK in the mouse skin led to increased ECM deposition, stromal tissue stiffness and promoted tumor growth and progression in vivo.Citation41 Moreover, combination of an agent that breaks down ECM, a key component of the desmoplastic stroma in PDAC, with the standard chemotherapeutic, gemcitabine, led to remodeling of the tumor microenvironment and objective responses in tumor-bearing KPC mice, resulting in a near doubling of overall survival and decreased metastatic burden in this aggressive disease model.Citation96-98 Hence, combining Rho inhibition with agents that improve tissue permeability through stromal ECM degradationCitation97,98 may increase the therapeutic benefit of Rho GTPase targeting in cancer. The relative contribution of the tumor cells and/or their microenvironment to these multilayered processes is yet to be elucidated. We envisage that these complex networks will be best examined using novel genetically engineered FRET biosensor mouse modelsCitation93,99-101 which will enable direct imaging of the dynamic regulation of Rho activity in native live tissues. To this end, we have recently developed a Rac-FRET GEM model that ubiquitously expresses the Raichu-Rac FRET biosensor at low level under the control of the ROSA26 promoter.Citation93 This mouse model has enabled detailed quantification of the spatiotemporal activity of Rac in living primary mammalian cells and tissues. Using this system, we have observed exquisite regulation of Rac activity in primary neutrophils, described best by transient and locally restricted bursts of Rac activity, which coincided with lamellipodial protrusions during neutrophil chemotaxis, highlighted in . Crossing the Rac-FRET mouse to various disease models has also proven its potential utility for assessing the effects of oncogenic mutations involved in carcinogenesis and tumor progression on Rac activity in a time- and tissue-specific manner, lending this model to the examination of drug targeting.Citation100 Recently, we have also shown that Rac1 facilitates colorectal tumorigenesis following the loss of the Adenomatous Polyposis Coli (APC) gene.Citation102
Figure 1 (See previous page). The Rac-FRET mouse is an invaluable tool to assess and quantify Rac1 activity in primary cells (A and B) and in vivo (C and D). (A) Neutrophil harvested from a Rac-FRET mouse migrating toward the chemoattractant N-formyl-methionyl-leucyl-phenyalanine (fMLP) south of the cell. Time series of Rac1 activity was obtained by ratiometric FRET live imaging and illustrated as a heat map of high (yellow to red) and low (blue to green) Rac1 activity. High Rac1 activity was localized to leading edge protrusions (green box) with short-lived bursts at the cell's periphery and at the trailing edge. (B) Maximum Rac1 activity along the longitudinal axis of a neutrophil (blue) migrating toward an fLMP gradient oscillated between the leading (green) and lagging edge (red) illustrated by fitting the experimental data (purple). (C) Intravital FLIM-FRET imaging demonstrated that Cre-mediated loss of APC in intestinal crypts promotes Rac1 activity. Low Rac1 activity in APC wild type mice (left panel) is represented by high fluorescence lifetimes (low FRET-efficiency, green), while loss of APC (right panel) results in high FRET-efficiency (low lifetimes, blue) indicating increased Rac1 activity. Each panel consists of fluorescence images of Rac-FRET expressing crypt cells (blue) and collagen (red, assessed by SHG imaging) on the left and FLIM images on the right. (D) Quantification of Rac1 activity by FLIM-FRET imaging reveals a significant decrease in the fluorescence lifetime upon deletion of APC correlating with an increase in Rac1 activity compared to APC wild type cells (mean± SEM; **P < 0.05). Figure adapted from Johnsson et al. Cell Reports Citation93.
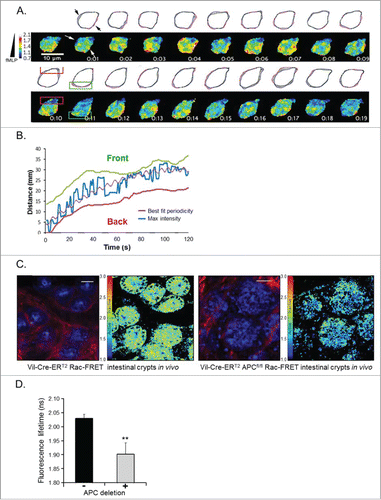
By crossing the Rac-FRET mouse strain to the Vil-Cre-ERT2 APCfl/fl model of colorectal cancer, we subsequently characterized the spatiotemporal Rac activity in the intestinal stem and progenitor cells of the established Vil-Cre-ERT2 APCfl/fl Rac-FRET mice.Citation93 In vivo FRET imaging revealed, for the first time, increased Rac activity at the base of the intestinal crypts following APC loss, demonstrating a critical role for Rac activation during disease initiation (illustrated in ). We have also generated a KPC PDAC Rac-FRET model and the breast cancer PyMT Rac-FRET mouse to analyze the dynamics of tumor-specific Rac signaling following administration of a targeted therapeutic agent, specifically the Rac inhibitor NSC23766.Citation93 Possible future applications could involve crossing the Rac-FRET strain with Lgr5-Cre miceCitation103 to examine Rac activity in the stem cells of the small intestine, providing fundamental insight in normal tissue homeostasis/development. Finally, the intricate control of the in vivo distribution, function, as well as potential redundancy of the numerous upstream and downstream regulators of Rho GTPase activity could be examined by crossing the biosensor mice onto GEF/GAP knockout strains Citation104 or knockout mice of key downstream effectors, for example the Rho kinases.Citation105,106 Collectively, current evidence emphasizes the general utility of novel GEM biosensor models as a tool for monitoring the complex mechanisms of Rho GTPase signaling under physiological conditions and this along with the current generation of other small G protein biosensor mice Citation101 should significantly improve our understanding of Rho GTPase signaling in the context of environmental cues from the surrounding tissue.
Rho GTPase Inhibitors in Cancer
Several inhibitors that target either Rho GTPase directly, or its regulators, have shown measurable anti-tumor activity in several in vitro and in vivo models of cancer.Citation12,107 A virtual drug-screen using the 3D structure of the Rho GTPase Rac1, was successfully used to screen for compounds that may directly modulate the Rho GTPase-GEF interaction, by binding to an area of the Rac1 protein important for interactions with specific GEFs.Citation107 NSC23766 was identified as a selective inhibitor of the Rac1-GEF interaction, which, when administered in vitro, reduced transformation of mouse NIH-3T3 fibroblast cells by active Rac1 or Rac-GEFs and significantly decreased proliferation, survival and invasiveness of a prostate cancer cell line PC-3, previously shown to have elevated levels of active Rac1.Citation107 Similarly, inhibition of Rac activity by EHT 1864 Citation108 significantly decreased estrogen-induced breast cancer cell proliferation and the Rac1-mediated induction of transcriptional activity of estrogen receptor α.Citation109 It is plausible to hypothesize that NSC23766 or similar novel inhibitors may prove effective when used in combination with other therapies to treat tumor subtypes with increased Rac1 activity, for example those tumors harboring the RAC1 (P29S) mutation or following APC loss described previously.Citation80,81,102
A recent study has also shown that a small molecule inhibitor of the Rho GTPase Cdc42, AZA197, effectively inhibited the proliferative potential and invasiveness of colorectal tumor cells and xenografts with high levels of Cdc42.Citation110 Interestingly, efficacy of this agent was cell line dependent, indicating that a personalized companion biomarker may be necessary to identify tumor types most likely to respond to this type of therapy.
Another approach would be to target the downstream effectors of Rho signaling. Several in vitro and in vivo studies have demonstrated that administration of the ROCK inhibitors, fasudil and Y-27632, leads to decreased tumor proliferation, invasiveness and metastatic potential for several cancer types.Citation33,111,112 The ROCK inhibitor fasudil has also been used effectively in combination with bortezomib, the first clinically approved proteasome inhibitor, to effectively treat the RAS oncogene-driven non-small cell lung cancer GEM model of the disease, through suppression of GATA2-regulated pathways.Citation113 Similarly, fasudil treatment in an intracerebral human glioma xenograft model was shown to suppress both neovascularity and tumor growth in vivo.Citation114 Whether the effect of these inhibitors is elicited purely on tumor cells themselves, the reactive stromal cells within the microenvironment, or most likely a combination of both, remains to be fully elucidated.
The tumor stroma is a complex environment mainly consisting of the basement membrane, fibroblasts, extracellular matrix, infiltrating immune cells, endothelial cells and associated vascular pericytes. The role of small GTPases in the stromal compartment of a developing tumor is less defined and needs further investigation. Wozniak et al.Citation115 have shown that the surrounding environment can directly govern the cellular behavior of breast epithelial cells in a 3D environment. For example, the authors of this study have shown that in free-floating 3D collagen matrices, the breast immortalized MCF10A cells differentiate into tubular structures. In contrast, if collagen matrix stiffness is increased by attachment to the bottom of a dish, the same cells do not differentiate, but proliferate and spread extensively.Citation115 In concordance, women with dense breast tissue, which is associated with a substantial increase in collagen deposition in the stroma, have a 4- to 6-fold increased risk of developing breast cancer.Citation116 Gene expression profiling of patient material containing high density mammary fibroblasts showed striking similarities in expression profiles to established cancer-associated fibroblasts (CAFs), with several key enriched signaling pathways, including JNK1, Rho GTPase(s), iNOS, FGF-R, EGF-R, and PDGF-R-mediated signal transduction, thereby creating a pro-inflammatory, pro-proliferative, cytokine, and chemokine-rich microenvironment.Citation117 Importantly, functional experiments revealed that the mechanism by which breast epithelial cells sense and respond to the mechanical properties of their surrounding environment involved tight regulation by RhoA, where stiff matrices promoted RhoA activity and localization of phosphorylated Y397 FAK into 3D cell adhesions in a ROCK-dependent manner.Citation115 Recent reports confirmed higher RhoA activity is found in stiff versus compliant matrices and demonstrated that in 3D collagen matrices, RhoA activity is considerably increased in cell-matrix adhesions, compared with cell-cell contacts.Citation118 As such, RhoA activation appears to be a central part of mechanotransduction and has been linked to enhanced ECM-focal adhesion signaling via Fak to drive enhanced tumor progression.Citation41,119 In , we demonstrate the function of ROCK activity in fibroblast-driven collagen I contraction on a 3D level by second harmonic generation (SHG) imaging. Alterations in collagen matrix structure can be correlated to elevated collagen density, crosslinking and stiffness visualized by SHG imaging.Citation41,120,121 The presence of the ROCK inhibitor Y-27632 significantly reduced the contraction of collagen gels, accompanied by reduced collagen density and crosslinking (). These changes in biomechanical matrix properties may contribute to the observed Y-27632-mediated decrease in cancer cell invasion when assessed in 3D organotypic matrices that incorporate cancer and stromal cell types.Citation87,122 In agreement, others have also demonstrated that ROCK activity is required for collagen crosslinking during wound healing in fibroblast-driven contraction assays,Citation123 indicating a positive feedback loop, in which ROCK signaling promotes matrix stiffening that subsequently enhances RhoA / ROCK activation to further crosslink ECM (described inCitation124). Recently, reorganization of the stromal ECM, tightly regulated by distinct Rho GTPases, has been shown to guide key aspects of branching morphogenesis in developing mammary glands, where Rac1 modulates signaling required for branch orientation, whereas the ability of the mammary epithelium to reinforce directional cues during branching morphogenesis appears to be mediated by RhoA/ROCK-mediated contractions.Citation125 Although tightly controlled during normal development and organ homeostasis, deregulation of this complex network could play an important role in the initiation and/ or progression of breast cancer.
Figure 2. The ROCK inhibitor Y-27632 decreases collagen contraction and crosslinking driven by fibroblasts in 3D organotypic assays. (A) Top panel: Schematic representation and representative pictures of contracting collagen-fibroblast matrices over a 12-day time course. Lower panel: Area of collagen in matrices treated with DMSO (control) and with Y-27632 (10 μM) as a function of time, p* = 0.0268. Scale bar: 10 mm. (B) Collagen coverage (%) quantified by multi-photon SHG imaging as a variable associated with depth (μm) within the 12-day contracted collagen-fibroblast matrices treated with control (DMSO) or with Y-27632 (10 μM) P*** < 0.0001. (C) Maximum intensity projection of SHG signal in representative collagen-fibroblast matrices. D. 3D projection of SHG signal in representative collagen-fibroblast matrices n = 3. Scale bar: 100 μm.
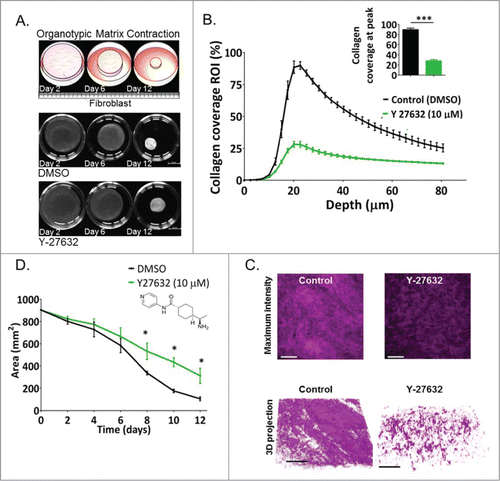
Imaging studies of collectively invading 3D co-cultures of squamous cell carcinoma cells and stromal fibroblasts have revealed that during invasion, carcinoma cells are led by stromal fibroblasts and move within tracks in the ECM created by CAFs through protease and force-mediated matrix remodeling.Citation122 Moreover, RhoA/ ROCK, LIMK, integrin alpha3 and alpha5 function were required for the leading fibroblasts to generate tracks in the matrix and enable tumor invasion, whereas the cancer cells depended on the activity of Cdc42 (but not RhoA) to follow the fibroblasts and invade into the organotypic collagen matrices.Citation122,126 Similarly, pro-inflammatory cytokine signaling through the JAK/STAT3 pathway and subsequent activation of RhoA/ ROCK is involved in generating actomyosin contractility in CAFs and melanoma cells.Citation127 In cholangiocarcinoma, a disease characterized by abundant desmoplastic stroma, cancer cells can effectively recruit CAFs through secretion of PDGF-D, which further stimulates fibroblast migration through PDGFRβ, Rho GTPase (Rac1, RhoA and Cdc42) and JNK activation.Citation128 Moreover, selective inhibition of Rho signaling, in particular Rac1 (NSC23766), but also ROCK (Y-27632) and Cdc42 (CASIN), dramatically decreased PDGF-induced fibroblast motility in this experimental model.Citation128 In pancreatic CAFs, high expression of the actin-associated protein Palladin significantly enhances the ability of CAFs to remodel the stromal ECM by regulating Cdc42 activity, which in turn promotes the assembly of matrix-degrading invadopodia in CAFs and enhance tumor cell invasion.Citation129 However, the precise roles of small GTPases in this complex interaction (also considering the roles of RhoA and Cdc42 in fibroblast track generation) remain unclear and need to be elucidated.
Furthermore, small GTPases have been implicated to function at the tumor-stromal interface during transendothelial migration of cancer cells. For example, arachidonic acid secreted by bone marrow adipocytes in vitro was shown to induce transendothelial migration of prostate cancer lines via Rho/ROCK-dependent amoeboid migration.Citation130 In line with this, a Rho GTPase RNAi screening study revealed a novel role of Cdc42 as a key regulator of prostate cancer cell transendothelial migration, where Cdc42 was found to be essential for in vivo cancer cell spreading and protrusion extension along blood vessels and colonization in the lungs.Citation131 Transient Cdc42 suppression in vivo led to a significant decrease in the formation of lung metastases following tail vein injection of prostate cancer PC-3 cells, suggesting that the role of Cdc42 in endothelial attachment is crucial for metastasis.Citation131 Moreover, glioblastoma cells have recently been shown to directly modulate the contractile activity of neighboring brain pericytes via Cdc42-dependent mechanisms, supporting vascular expansion and tumor progression.Citation132 Overexpression of RhoJ, a Rho GTPase mainly present in endothelial cells, is associated with increased prevalence of lymphovascular invasion, lymph node metastases and decreased overall survival in colon cancer.Citation56 Using an inducible endothelial cell-specific RhoJ loss-of-function GEM, generated by crossing RhojGFP/GFP knock-out miceCitation56 with the Cdh5(PAC)-CreERT2 model,Citation133 the authors further demonstrated that RhoJ deletion in this context disrupted tumor vessel formation and vascular integrity, suppressed tumor angiogenesis, presenting a feasible target for clinical drug development.Citation56 Collectively, these studies highlight the increasing relevance of the Rho GTPases within divergent cellular components of the tumor microenvironment and further underline the significance of distinguishing drug effects on cancer cells vs. those on the surrounding host stroma. A more comprehensive understanding of the contextual dependence of Rho GTPase signaling in the tumor cells and the surrounding stroma will be a necessary step toward successful implementation of therapeutics that target Rho signaling as cancer therapy, providing interesting avenues for the development of combination therapies.
Future Perspectives
Numerous conceptual advances in biology have been achieved by experimental studies using 2-dimensional cell culture systems. Recent adaptations of molecular imaging techniques to 3-dimensional model systems, increasing in complexity from the 3D-spheroid cultures, the transparent Drosophila, Xenopus and Zebrafish, to the complex mammalian xenograft and GEM models, are bridging the gap in our understanding of biological events in vitro and in vivo, establishing an important role for Rho GTPases in disease progression and therapeutic targeting. We envisage that future applications will involve generation of transgenic mice that co-express combinations of Rho GTPase FRET biosensors to provide a detailed map of physiological signal transduction events in an intact mammalian organism. Stromal cells and the role that they have on cancer initiation and progression will have important implications on the examination of Rho GTPase activity in live tissues as well as therapeutic targeting. A major application already underway will involve crossing other disease models with the Rho GTPase FRET biosensor mice to examine disease etiology and improve drug development and screening for progressing novel agents into clinical trials. Similarly, crossing the Rac GTPase FRET mouseCitation93 with transgenic mice expressing stroma-specific Cre recombinase, for example in fibroblastsCitation134-136 or endothelium,Citation137-139 in the future could provide detailed insight into the intricacy of stroma-specific Rac signaling in distinct stromal compartments in real-time.
Disclosure of Potential Conflicts of Interest
No potential conflicts of interest were disclosed.
Funding
This work was supported by the following grants: Cancer Australia (APP1065022), Cancer Institute New South Wales (13CDF1-01), Cancer Council NSW (RG 14-08) and the Philip Hemstritch Fellowship.
References
- Biankin AV, Waddell N, Kassahn KS, Gingras MC, Muthuswamy LB, Johns AL, Miller DK, Wilson PJ, Patch AM, Wu J, et al. Pancreatic cancer genomes reveal aberrations in axon guidance pathway genes. Nature 2012; 491:399-405; PMID:23103869; http://dx.doi.org/10.1038/nature11547
- Brennan CW, Verhaak RG, McKenna A, Campos B, Noushmehr H, Salama SR, Zheng S, Chakravarty D, Sanborn JZ, Berman SH, et al. The somatic genomic landscape of glioblastoma. Cell 2013; 155:462-77; PMID:24120142; http://dx.doi.org/10.1016/j.cell.2013.09.034
- Kandoth C, McLellan MD, Vandin F, Ye K, Niu B, Lu C, Xie M, Zhang Q, McMichael JF, Wyczalkowski MA, et al. Mutational landscape and significance across 12 major cancer types. Nature 2013; 502:333-9; PMID:24132290; http://dx.doi.org/10.1038/nature12634
- Straussman R, Morikawa T, Shee K, Barzily-Rokni M, Qian ZR, Du J, Davis A, Mongare MM, Gould J, Frederick DT, et al. Tumour micro-environment elicits innate resistance to RAF inhibitors through HGF secretion. Nature 2012; 487:500-4; PMID:22763439; http://dx.doi.org/10.1038/nature11183
- Fang H, Declerck YA. Targeting the tumor microenvironment: from understanding pathways to effective clinical trials. Cancer Res 2013; 73:4965-77; PMID:23913938; http://dx.doi.org/10.1158/0008-5472.CAN-13-0661
- Tredan O, Galmarini CM, Patel K, Tannock IF. Drug resistance and the solid tumor microenvironment. J Natl Cancer Inst 2007; 99:1441-54; PMID:17895480; http://dx.doi.org/10.1093/jnci/djm135
- Cherfils J, Zeghouf M. Regulation of small GTPases by GEFs, GAPs, and GDIs. Physiol Rev 2013; 93:269-309; PMID:23303910; http://dx.doi.org/10.1152/physrev.00003.2012
- Samarakoon R, Higgins CE, Higgins SP, Higgins PJ. Differential requirement for MEK/ERK and SMAD signaling in PAI-1 and CTGF expression in response to microtubule disruption. Cell Signal 2009; 21:986-95; PMID:19249354; http://dx.doi.org/10.1016/j.cellsig.2009.02.007
- Samarakoon R, Higgins PJ. Integration of non-SMAD and SMAD signaling in TGF-beta1-induced plasminogen activator inhibitor type-1 gene expression in vascular smooth muscle cells. Thromb Haemost 2008; 100:976-83; PMID:19132220
- Timpson P, Jones GE, Frame MC, Brunton VG. Coordination of cell polarization and migration by the Rho family GTPases requires Src tyrosine kinase activity. Curr Biol 2001; 11:1836-46; PMID:11728306; http://dx.doi.org/10.1016/S0960-9822(01)00583-8
- Tomar A, Schlaepfer DD. Focal adhesion kinase: switching between GAPs and GEFs in the regulation of cell motility. Curr Opin Cell Biol 2009; 21:676-83; PMID:19525103; http://dx.doi.org/10.1016/j.ceb.2009.05.006
- Walker K, Olson MF. Targeting Ras and Rho GTPases as opportunities for cancer therapeutics. Current Opin Genet Dev 2005; 15:62-8; PMID:15661535; http://dx.doi.org/10.1016/j.gde.2004.11.001
- Yoshizaki H, Ohba Y, Kurokawa K, Itoh RE, Nakamura T, Mochizuki N, Nagashima K, Matsuda M. Activity of Rho-family GTPases during cell division as visualized with FRET-based probes. J Cell Biol 2003; 162:223-32; PMID:12860967; http://dx.doi.org/10.1083/jcb.200212049
- Yoshizaki H, Ohba Y, Parrini MC, Dulyaninova NG, Bresnick AR, Mochizuki N, Matsuda M. Cell type-specific regulation of RhoA activity during cytokinesis. J Biol Chem 2004; 279:44756-62; PMID:15308673; http://dx.doi.org/10.1074/jbc.M402292200
- Maddox AS, Burridge K. RhoA is required for cortical retraction and rigidity during mitotic cell rounding. J Cell Biol 2003; 160:255-65; PMID:12538643; http://dx.doi.org/10.1083/jcb.200207130
- Bai X, Lenhart KC, Bird KE, Suen AA, Rojas M, Kakoki M, Li F, Smithies O, Mack CP, Taylor JM. The smooth muscle-selective RhoGAP GRAF3 is a critical regulator of vascular tone and hypertension. Nature Commun 2013; 4:2910; PMID:24335996; http://dx.doi.org/10.1038/ncomms3910
- Loirand G, Pacaud P. The role of Rho protein signaling in hypertension. Nature Rev Cardiol 2010; 7:637-47; PMID:20808285; http://dx.doi.org/10.1038/nrcardio.2010.136
- Loirand G, Guerin P, Pacaud P. Rho kinases in cardiovascular physiology and pathophysiology. Circ Res 2006; 98:322-34; PMID:16484628; http://dx.doi.org/10.1161/01.RES.0000201960.04223.3c
- Vesterinen HM, Currie GL, Carter S, Mee S, Watzlawick R, Egan KJ, Macleod MR, Sena ES. Systematic review and stratified meta-analysis of the efficacy of RhoA and Rho kinase inhibitors in animal models of ischaemic stroke. Syst Rev 2013; 2:33; PMID:23687965; http://dx.doi.org/10.1186/2046-4053-2-33
- Biro M, Munoz MA, Weninger W. Targeting Rho-GTPases in immune cell migration and inflammation. Br J Pharmacol 2014; 171:5491-506; PMID:24571448; http://dx.doi.org/10.1111/bph.12658
- Musilli M, Nicolia V, Borrelli S, Scarpa S, Diana G. Behavioral effects of Rho GTPase modulation in a model of Alzheimer's disease. Behav Brain Res 2013; 237:223-9; PMID:23026376; http://dx.doi.org/10.1016/j.bbr.2012.09.043
- Petratos S, Li QX, George AJ, Hou X, Kerr ML, Unabia SE, Hatzinisiriou I, Maksel D, Aguilar MI, Small DH. The beta-amyloid protein of Alzheimer's disease increases neuronal CRMP-2 phosphorylation by a Rho-GTP mechanism. Brain 2008; 131:90-108; PMID:18000012; http://dx.doi.org/10.1093/brain/awm260
- Dergham P, Ellezam B, Essagian C, Avedissian H, Lubell WD, McKerracher L. Rho signaling pathway targeted to promote spinal cord repair. J Neurosci 2002; 22:6570-7; PMID:12151536
- Hara M, Takayasu M, Watanabe K, Noda A, Takagi T, Suzuki Y, Yoshida J. Protein kinase inhibition by fasudil hydrochloride promotes neurological recovery after spinal cord injury in rats. J Neurosurg 2000; 93:94-101; PMID:10879764
- Inoue M, Rashid MH, Fujita R, Contos JJ, Chun J, Ueda H. Initiation of neuropathic pain requires lysophosphatidic acid receptor signaling. Nat Med 2004; 10:712-8; PMID:15195086; http://dx.doi.org/10.1038/nm1060
- Sung JK, Miao L, Calvert JW, Huang L, Louis Harkey H, Zhang JH. A possible role of RhoA/Rho-kinase in experimental spinal cord injury in rat. Brain Res 2003; 959:29-38; PMID:12480155; http://dx.doi.org/10.1016/S0006-8993(02)03717-4
- Shibuya M, Suzuki Y, Sugita K, Saito I, Sasaki T, Takakura K, Nagata I, Kikuchi H, Takemae T, Hidaka H, et al. Effect of AT877 on cerebral vasospasm after aneurysmal subarachnoid hemorrhage. Results of a prospective placebo-controlled double-blind trial. J Neurosurg 1992; 76:571-7; PMID:1545249; http://dx.doi.org/10.3171/jns.1992.76.4.0571
- Doe C, Bentley R, Behm DJ, Lafferty R, Stavenger R, Jung D, Bamford M, Panchal T, Grygielko E, Wright LL, et al. Novel Rho kinase inhibitors with anti-inflammatory and vasodilatory activities. J Pharmacol Exp Ther 2007; 320:89-98; PMID:17018693; http://dx.doi.org/10.1124/jpet.106.110635
- Stavenger RA, Cui H, Dowdell SE, Franz RG, Gaitanopoulos DE, Goodman KB, Hilfiker MA, Ivy RL, Leber JD, Marino JP Jr, et al. Discovery of aminofurazan-azabenzimidazoles as inhibitors of Rho-kinase with high kinase selectivity and antihypertensive activity. J Med Chem 2007; 50:2-5; PMID:17201404; http://dx.doi.org/10.1021/jm060873p
- Sahai E, Marshall CJ. RHO-GTPases and cancer. Nat Rev Cancer 2002; 2:133-42; PMID:12635176; http://dx.doi.org/10.1038/nrc725
- Fritz G, Brachetti C, Bahlmann F, Schmidt M, Kaina B. Rho GTPases in human breast tumours: expression and mutation analyses and correlation with clinical parameters. Br J Cancer 2002; 87:635-44; PMID:12237774; http://dx.doi.org/10.1038/sj.bjc.6600510
- Lane J, Martin TA, Watkins G, Mansel RE, Jiang WG. The expression and prognostic value of ROCK I and ROCK II and their role in human breast cancer. Int J Oncol 2008; 33:585-93; PMID:18695890
- Liu S, Goldstein RH, Scepansky EM, Rosenblatt M. Inhibition of rho-associated kinase signaling prevents breast cancer metastasis to human bone. Cancer Res 2009; 69:8742-51; PMID:19887617; http://dx.doi.org/10.1158/0008-5472.CAN-09-1541
- Li NF, Gemenetzidis E, Marshall FJ, Davies D, Yu Y, Frese K, Froeling FE, Woolf AK, Feakins RM, Naito Y, et al. RhoC interacts with integrin alpha5beta1 and enhances its trafficking in migrating pancreatic carcinoma cells. PLoS One 2013; 8:e81575; PMID:24312560; http://dx.doi.org/10.1371/journal.pone.0081575
- Rosenthal DT, Iyer H, Escudero S, Bao L, Wu Z, Ventura AC, Kleer CG, Arruda EM, Garikipati K, Merajver SD. p38gamma promotes breast cancer cell motility and metastasis through regulation of RhoC GTPase, cytoskeletal architecture, and a novel leading edge behavior. Cancer Res 2011; 71:6338-49; PMID:21862636; http://dx.doi.org/10.1158/0008-5472.CAN-11-1291
- Wang Y, Lei R, Zhuang X, Zhang N, Pan H, Li G, Hu J, Pan X, Tao Q, Fu D, et al. DLC1-dependent parathyroid hormone-like hormone inhibition suppresses breast cancer bone metastasis. J Clin Invest 2014; 124:1646-59; PMID:24590291; http://dx.doi.org/10.1172/JCI71812
- Guise TA, Yin JJ, Taylor SD, Kumagai Y, Dallas M, Boyce BF, Yoneda T, Mundy GR. Evidence for a causal role of parathyroid hormone-related protein in the pathogenesis of human breast cancer-mediated osteolysis. J Clin Invest 1996; 98:1544-9; PMID:8833902; http://dx.doi.org/10.1172/JCI118947
- Derynck R, Akhurst RJ, Balmain A. TGF-beta signaling in tumor suppression and cancer progression. Nat Genet 2001; 29:117-29; PMID:11586292; http://dx.doi.org/10.1038/ng1001-117
- Kaneko K, Satoh K, Masamune A, Satoh A, Shimosegawa T. Expression of ROCK-1 in human pancreatic cancer: its down-regulation by morpholino oligo antisense can reduce the migration of pancreatic cancer cells in vitro. Pancreas 2002; 24:251-7; PMID:11893932; http://dx.doi.org/10.1097/00006676-200204000-00007
- Kamai T, Yamanishi T, Shirataki H, Takagi K, Asami H, Ito Y, Yoshida K. Overexpression of RhoA, Rac1, and Cdc42 GTPases is associated with progression in testicular cancer. Clin Cancer Res 2004; 10:4799-805; PMID:15269155; http://dx.doi.org/10.1158/1078-0432.CCR-0436-03
- Samuel MS, Lopez JI, McGhee EJ, Croft DR, Strachan D, Timpson P, Munro J, Schroder E, Zhou J, Brunton VG, et al. Actomyosin-mediated cellular tension drives increased tissue stiffness and beta-catenin activation to induce epidermal hyperplasia and tumor growth. Cancer Cell 2011; 19:776-91; PMID:21665151; http://dx.doi.org/10.1016/j.ccr.2011.05.008
- Bellovin DI, Simpson KJ, Danilov T, Maynard E, Rimm DL, Oettgen P, Mercurio AM. Reciprocal regulation of RhoA and RhoC characterizes the EMT and identifies RhoC as a prognostic marker of colon carcinoma. Oncogene 2006; 25:6959-67; PMID:16715134; http://dx.doi.org/10.1038/sj.onc.1209682
- Shepelev MV, Korobko IV. The RHOV gene is overexpressed in human non-small cell lung cancer. Cancer Genet 2013; 206:393-7; PMID:24388711; http://dx.doi.org/10.1016/j.cancergen.2013.10.006
- Zhao Y, Zheng HC, Chen S, Gou WF, Xiao LJ, Niu ZF. The role of RhoC in ovarian epithelial carcinoma: a marker for carcinogenesis, progression, prognosis, and target therapy. Gynecol Oncol 2013; 130:570-8; PMID:23764197; http://dx.doi.org/10.1016/j.ygyno.2013.06.004
- Yoshioka K, Nakamori S, Itoh K. Overexpression of small GTP-binding protein RhoA promotes invasion of tumor cells. Cancer Res 1999; 59:2004-10; PMID:10213513
- Bray K, Gillette M, Young J, Loughran E, Hwang M, Sears JC, Vargo-Gogola T. Cdc42 overexpression induces hyperbranching in the developing mammary gland by enhancing cell migration. Breast Cancer Res 2013; 15:R91; PMID:24074261; http://dx.doi.org/10.1186/bcr3487
- Parri M, Chiarugi P. Rac and Rho GTPases in cancer cell motility control. Cell Commun Signal 2010; 8:23; PMID:20822528; http://dx.doi.org/10.1186/1478-811X-8-23
- Sahai E, Marshall CJ. Differing modes of tumour cell invasion have distinct requirements for Rho/ROCK signalling and extracellular proteolysis. Nat Cell Biol 2003; 5:711-9; PMID:12844144; http://dx.doi.org/10.1038/ncb1019
- Yamazaki D, Kurisu S, Takenawa T. Involvement of Rac and Rho signaling in cancer cell motility in 3D substrates. Oncogene 2009; 28:1570-83; PMID:19234490; http://dx.doi.org/10.1038/onc.2009.2
- Guo X, Wang M, Jiang J, Xie C, Peng F, Li X, Tian R, Qin R. Balanced Tiam1-rac1 and RhoA drives proliferation and invasion of pancreatic cancer cells. Mol Cancer Res 2013; 11:230-9; PMID:23322732; http://dx.doi.org/10.1158/1541-7786.MCR-12-0632
- de Toledo M, Anguille C, Roger L, Roux P, Gadea G. Cooperative anti-invasive effect of Cdc42/Rac1 activation and ROCK inhibition in SW620 colorectal cancer cells with elevated blebbing activity. PLoS One 2012; 7:e48344; PMID:23144867; http://dx.doi.org/10.1371/journal.pone.0048344
- Coleman ML, Marshall CJ, Olson MF. RAS and RHO GTPases in G1-phase cell-cycle regulation. Nat Rev Mol Cell Biol 2004; 5:355-66; PMID:15122349; http://dx.doi.org/10.1038/nrm1365
- Boerner JL, Danielsen A, McManus MJ, Maihle NJ. Activation of Rho is required for ligand-independent oncogenic signaling by a mutant epidermal growth factor receptor. J Biol Chem 2001; 276:3691-5; PMID:11110781; http://dx.doi.org/10.1074/jbc.M003801200
- Servitja JM, Marinissen MJ, Sodhi A, Bustelo XR, Gutkind JS. Rac1 function is required for Src-induced transformation. Evidence of a role for Tiam1 and Vav2 in Rac activation by Src. J Biol Chem 2003; 278:34339-46; PMID:12810717; http://dx.doi.org/10.1074/jbc.M302960200
- Wu WJ, Tu S, Cerione RA. Activated Cdc42 sequesters c-Cbl and prevents EGF receptor degradation. Cell 2003; 114:715-25; PMID:14505571; http://dx.doi.org/10.1016/S0092-8674(03)00688-3
- Kim C, Yang H, Fukushima Y, Saw PE, Lee J, Park JS, Park I, Jung J, Kataoka H, Lee D, et al. Vascular RhoJ is an effective and selective target for tumor angiogenesis and vascular disruption. Cancer Cell 2014; 25:102-17; PMID:24434213; http://dx.doi.org/10.1016/j.ccr.2013.12.010
- Ho H, Aruri J, Kapadia R, Mehr H, White MA, Ganesan AK. RhoJ regulates melanoma chemoresistance by suppressing pathways that sense DNA damage. Cancer Res 2012; 72:5516-28; PMID:22971344; http://dx.doi.org/10.1158/0008-5472.CAN-12-0775
- Hofbauer SW, Krenn PW, Ganghammer S, Asslaber D, Pichler U, Oberascher K, Henschler R, Wallner M, Kerschbaum H, Greil R, et al. Tiam1/Rac1 signals contribute to the proliferation and chemoresistance, but not motility, of chronic lymphocytic leukemia cells. Blood 2014; 123:2181-8; PMID:24501217; http://dx.doi.org/10.1182/blood-2013-08-523563
- Montresor A, Bolomini-Vittori M, Toffali L, Rossi B, Constantin G, Laudanna C. JAK tyrosine kinases promote hierarchical activation of Rho and Rap modules of integrin activation. J Cell Biol 2013; 203:1003-19; PMID:24368807; http://dx.doi.org/10.1083/jcb.201303067
- Raptis L, Arulanandam R, Geletu M, Turkson J. The R(h)oads to Stat3: Stat3 activation by the Rho GTPases. Exp Cell Res 2011; 317:1787-95; PMID:21619876; http://dx.doi.org/10.1016/j.yexcr.2011.05.008
- Kawasaki Y, Senda T, Ishidate T, Koyama R, Morishita T, Iwayama Y, Higuchi O, Akiyama T. Asef, a link between the tumor suppressor APC and G-protein signaling. Science 2000; 289:1194-7; PMID:10947987; http://dx.doi.org/10.1126/science.289.5482.1194
- Sandilands E, Cans C, Fincham VJ, Brunton VG, Mellor H, Prendergast GC, Norman JC, Superti-Furga G, Frame MC. RhoB and actin polymerization coordinate Src activation with endosome-mediated delivery to the membrane. Dev Cell 2004; 7:855-69; PMID:15572128; http://dx.doi.org/10.1016/j.devcel.2004.09.019
- Machesky LM, Sansom OJ. Rac1 in the driver's seat for melanoma. Pigment Cell Melanoma Res 2012; 25:762-4; PMID:22882909; http://dx.doi.org/10.1111/pcmr.12004
- Orgaz JL, Herraiz C, Sanz-Moreno V. Rho GTPases modulate malignant transformation of tumor cells. Small GTPases 2014; 5:e29019; PMID:25036871; http://dx.doi.org/10.4161/sgtp.29019
- Eva A, Aaronson SA. Isolation of a new human oncogene from a diffuse B-cell lymphoma. Nature 1985; 316:273-5; PMID:3875039; http://dx.doi.org/10.1038/316273a0
- Katzav S, Martin-Zanca D, Barbacid M. vav, a novel human oncogene derived from a locus ubiquitously expressed in hematopoietic cells. EMBO J 1989; 8:2283-90; PMID:2477241
- Montero JC, Seoane S, Ocana A, Pandiella A. P-Rex1 participates in Neuregulin-ErbB signal transduction and its expression correlates with patient outcome in breast cancer. Oncogene 2011; 30:1059-71; PMID:21042280; http://dx.doi.org/10.1038/onc.2010.489
- Toksoz D, Williams DA. Novel human oncogene lbc detected by transfection with distinct homology regions to signal transduction products. Oncogene 1994; 9:621-8; PMID:8290273
- Jaiswal M, Dvorsky R, Amin E, Risse SL, Fansa EK, Zhang SC, Taha MS, Gauhar AR, Nakhaei-Rad S, Kordes C, et al. Functional cross-talk between ras and rho pathways: a Ras-specific GTPase-activating protein (p120RasGAP) competitively inhibits the RhoGAP activity of deleted in liver cancer (DLC) tumor suppressor by masking the catalytic arginine finger. J Biol Chem 2014; 289:6839-49; PMID:24443565
- Radu M, Rawat SJ, Beeser A, Iliuk A, Tao WA, Chernoff J. ArhGAP15, a Rac-specific GTPase-activating protein, plays a dual role in inhibiting small GTPase signaling. J Biol Chem 2013; 288:21117-25; PMID:23760270; http://dx.doi.org/10.1074/jbc.M113.459719
- Barone I, Brusco L, Gu G, Selever J, Beyer A, Covington KR, Tsimelzon A, Wang T, Hilsenbeck SG, Chamness GC, et al. Loss of Rho GDIalpha and resistance to tamoxifen via effects on estrogen receptor alpha. J Natl Cancer Inst 2011; 103:538-52; PMID:21447808; http://dx.doi.org/10.1093/jnci/djr058
- Cho HJ, Baek KE, Kim IK, Park SM, Choi YL, Nam IK, Park SH, Im MJ, Yoo JM, Ryu KJ, et al. Proteomics-based strategy to delineate the molecular mechanisms of RhoGDI2-induced metastasis and drug resistance in gastric cancer. J Proteome Res 2012; 11:2355-64; PMID:22364609; http://dx.doi.org/10.1021/pr2011186
- Wu Y, Moissoglu K, Wang H, Wang X, Frierson HF, Schwartz MA, Theodorescu D. Src phosphorylation of RhoGDI2 regulates its metastasis suppressor function. Proc Natl Acad Sci U S A 2009; 106:5807-12; PMID:19321744; http://dx.doi.org/10.1073/pnas.0810094106
- Pasqualucci L, Neumeister P, Goossens T, Nanjangud G, Chaganti RS, Kuppers R, Dalla-Favera R. Hypermutation of multiple proto-oncogenes in B-cell diffuse large-cell lymphomas. Nature 2001; 412:341-6; PMID:11460166; http://dx.doi.org/10.1038/35085588
- Preudhomme C, Roumier C, Hildebrand MP, Dallery-Prudhomme E, Lantoine D, Lai JL, Daudignon A, Adenis C, Bauters F, Fenaux P, et al. Nonrandom 4p13 rearrangements of the RhoH/TTF gene, encoding a GTP-binding protein, in non-Hodgkin's lymphoma and multiple myeloma. Oncogene 2000; 19:2023-32; PMID:10803463; http://dx.doi.org/10.1038/sj.onc.1203521
- Sakata-Yanagimoto M, Enami T, Yoshida K, Shiraishi Y, Ishii R, Miyake Y, Muto H, Tsuyama N, Sato-Otsubo A, Okuno Y, et al. Somatic RHOA mutation in angioimmunoblastic T cell lymphoma. Nat Genet 2014; 46:171-5; PMID:24413737; http://dx.doi.org/10.1038/ng.2872
- Yoo HY, Sung MK, Lee SH, Kim S, Lee H, Park S, Kim SC, Lee B, Rho K, Lee JE, et al. A recurrent inactivating mutation in RHOA GTPase in angioimmunoblastic T cell lymphoma. Nat Genet 2014; 46:371-5; PMID:24584070; http://dx.doi.org/10.1038/ng.2916
- Federico M, Rudiger T, Bellei M, Nathwani BN, Luminari S, Coiffier B, Harris NL, Jaffe ES, Pileri SA, Savage KJ, et al. Clinicopathologic characteristics of angioimmunoblastic T-cell lymphoma: analysis of the international peripheral T-cell lymphoma project. J Clin Oncol 2013; 31:240-6; PMID:22869878; http://dx.doi.org/10.1200/JCO.2011.37.3647
- Comprehensive molecular characterization of gastric adenocarcinoma. Nature 2014; 513:202-9; PMID:25079317; http://dx.doi.org/10.1038/nature13480
- Krauthammer M, Kong Y, Ha BH, Evans P, Bacchiocchi A, McCusker JP, Cheng E, Davis MJ, Goh G, Choi M, et al. Exome sequencing identifies recurrent somatic RAC1 mutations in melanoma. Nat Genet 2012; 44:1006-14; PMID:22842228; http://dx.doi.org/10.1038/ng.2359
- Davis MJ, Ha BH, Holman EC, Halaban R, Schlessinger J, Boggon TJ. RAC1P29S is a spontaneously activating cancer-associated GTPase. Proc Natl Acad Sci U S A 2013; 110:912-7; PMID:23284172; http://dx.doi.org/10.1073/pnas.1220895110
- Hodis E, Watson IR, Kryukov GV, Arold ST, Imielinski M, Theurillat JP, Nickerson E, Auclair D, Li L, Place C, et al. A landscape of driver mutations in melanoma. Cell 2012; 150:251-63; PMID:22817889; http://dx.doi.org/10.1016/j.cell.2012.06.024
- Matos P, Oliveira C, Velho S, Goncalves V, da Costa LT, Moyer MP, Seruca R, Jordan P. B-Raf(V600E) cooperates with alternative spliced Rac1b to sustain colorectal cancer cell survival. Gastroenterology 2008; 135:899-906; PMID:18602919; http://dx.doi.org/10.1053/j.gastro.2008.05.052
- Kraynov VS, Chamberlain C, Bokoch GM, Schwartz MA, Slabaugh S, Hahn KM. Localized Rac activation dynamics visualized in living cells. Science 2000; 290:333-7; PMID:11030651; http://dx.doi.org/10.1126/science.290.5490.333
- Machacek M, Hodgson L, Welch C, Elliott H, Pertz O, Nalbant P, Abell A, Johnson GL, Hahn KM, Danuser G. Coordination of Rho GTPase activities during cell protrusion. Nature 2009; 461:99-103; PMID:19693013; http://dx.doi.org/10.1038/nature08242
- Pertz O, Hodgson L, Klemke RL, Hahn KM. Spatiotemporal dynamics of RhoA activity in migrating cells. Nature 2006; 440:1069-72; PMID:16547516; http://dx.doi.org/10.1038/nature04665
- Timpson P, McGhee EJ, Morton JP, von Kriegsheim A, Schwarz JP, Karim SA, Doyle B, Quinn JA, Carragher NO, Edward M, et al. Spatial regulation of RhoA activity during pancreatic cancer cell invasion driven by mutant p53. Cancer Res 2011; 71:747-57; PMID:21266354; http://dx.doi.org/10.1158/0008-5472.CAN-10-2267
- Fernandez-Espartero CH, Ramel D, Farago M, Malartre M, Luque CM, Limanovich S, Katzav S, Emery G, Martin-Bermudo MD. GTP exchange factor Vav regulates guided cell migration by coupling guidance receptor signalling to local Rac activation. J Cell Sci 2013; 126:2285-93; PMID:23525006; http://dx.doi.org/10.1242/jcs.124438
- Stramer B, Wood W, Galko MJ, Redd MJ, Jacinto A, Parkhurst SM, Martin P. Live imaging of wound inflammation in Drosophila embryos reveals key roles for small GTPases during in vivo cell migration. J Cell Biol 2005; 168:567-73; PMID:15699212; http://dx.doi.org/10.1083/jcb.200405120
- Kardash E, Reichman-Fried M, Maitre JL, Boldajipour B, Papusheva E, Messerschmidt EM, Heisenberg CP, Raz E. A role for Rho GTPases and cell-cell adhesion in single-cell motility in vivo. Nat Cell Biol 2010; 12:47-53; sup pp 1-11; PMID:20010816; http://dx.doi.org/10.1038/ncb2003
- Xu H, Kardash E, Chen S, Raz E, Lin F. Gbetagamma signaling controls the polarization of zebrafish primordial germ cells by regulating Rac activity. Development 2012; 139:57-62; PMID:22096073; http://dx.doi.org/10.1242/dev.073924
- Hirata E, Yukinaga H, Kamioka Y, Arakawa Y, Miyamoto S, Okada T, Sahai E, Matsuda M. In vivo fluorescence resonance energy transfer imaging reveals differential activation of Rho-family GTPases in glioblastoma cell invasion. J Cell Sci 2012; 125:858-68; PMID:22399802; http://dx.doi.org/10.1242/jcs.089995
- Johnsson AK, Dai Y, Nobis M, Baker MJ, McGhee EJ, Walker S, Schwarz JP, Kadir S, Morton JP, Myant KB, et al. The Rac-FRET mouse reveals tight spatiotemporal control of Rac activity in primary cells and tissues. Cell Rep 2014; 6:1153-64; PMID:24630994; http://dx.doi.org/10.1016/j.celrep.2014.02.024
- Karim SA, Creedon H, Patel H, Carragher NO, Morton JP, Muller WJ, Evans TR, Gusterson B, Sansom OJ, Brunton VG. Dasatinib inhibits mammary tumour development in a genetically engineered mouse model. J Pathol 2013; 230:430-40; PMID:23616343; http://dx.doi.org/10.1002/path.4202
- Morton JP, Karim SA, Graham K, Timpson P, Jamieson N, Athineos D, Doyle B, McKay C, Heung MY, Oien KA, et al. Dasatinib inhibits the development of metastases in a mouse model of pancreatic ductal adenocarcinoma. Gastroenterology 2010; 139:292-303; PMID:20303350; http://dx.doi.org/10.1053/j.gastro.2010.03.034
- Jacobetz MA, Chan DS, Neesse A, Bapiro TE, Cook N, Frese KK, Feig C, Nakagawa T, Caldwell ME, Zecchini HI, et al. Hyaluronan impairs vascular function and drug delivery in a mouse model of pancreatic cancer. Gut 2013; 62:112-20; PMID:22466618; http://dx.doi.org/10.1136/gutjnl-2012-302529
- Olive KP, Jacobetz MA, Davidson CJ, Gopinathan A, McIntyre D, Honess D, Madhu B, Goldgraben MA, Caldwell ME, Allard D, et al. Inhibition of Hedgehog signaling enhances delivery of chemotherapy in a mouse model of pancreatic cancer. Science 2009; 324:1457-61; PMID:19460966; http://dx.doi.org/10.1126/science.1171362
- Provenzano PP, Cuevas C, Chang AE, Goel VK, Von Hoff DD, Hingorani SR. Enzymatic targeting of the stroma ablates physical barriers to treatment of pancreatic ductal adenocarcinoma. Cancer Cell 2012; 21:418-29; PMID:22439937; http://dx.doi.org/10.1016/j.ccr.2012.01.007
- Kamioka Y, Sumiyama K, Mizuno R, Matsuda M. Live imaging of transgenic mice expressing FRET biosensors. Conf Proc IEEE Eng Med Biol Soc 2013; 2013:125-8; PMID:24109640
- Conway JRW, Carragher NO, Timpson P. Developments in preclinical cancer imaging: innovating the discovery of therapeutics. Nat Rev Cancer 2014; 14:314-28; PMID:24739578; http://dx.doi.org/10.1038/nrc3724
- Goto A, Sumiyama K, Kamioka Y, Nakasyo E, Ito K, Iwasaki M, Enomoto H, Matsuda M. GDNF and endothelin 3 regulate migration of enteric neural crest-derived cells via protein kinase A and Rac1. J Neurosci 2013; 33:4901-12; PMID:23486961; http://dx.doi.org/10.1523/JNEUROSCI.4828-12.2013
- Myant KB, Cammareri P, McGhee EJ, Ridgway RA, Huels DJ, Cordero JB, Schwitalla S, Kalna G, Ogg EL, Athineos D, et al. ROS production and NF-kappaB activation triggered by RAC1 facilitate WNT-driven intestinal stem cell proliferation and colorectal cancer initiation. Cell Stem Cell 2013; 12:761-73; PMID:23665120; http://dx.doi.org/10.1016/j.stem.2013.04.006
- Barker N, van Es JH, Kuipers J, Kujala P, van den Born M, Cozijnsen M, Haegebarth A, Korving J, Begthel H, Peters PJ, et al. Identification of stem cells in small intestine and colon by marker gene Lgr5. Nature 2007; 449:1003-7; PMID:17934449; http://dx.doi.org/10.1038/nature06196
- Lindsay CR, Lawn S, Campbell AD, Faller WJ, Rambow F, Mort RL, Timpson P, Li A, Cammareri P, Ridgway RA, et al. P-Rex1 is required for efficient melanoblast migration and melanoma metastasis. Nat Commun 2011; 2:555; PMID:22109529; http://dx.doi.org/10.1038/ncomms1560
- Shimizu Y, Thumkeo D, Keel J, Ishizaki T, Oshima H, Oshima M, Noda Y, Matsumura F, Taketo MM, Narumiya S. ROCK-I regulates closure of the eyelids and ventral body wall by inducing assembly of actomyosin bundles. J Cell Biol 2005; 168:941-53; PMID:15753128; http://dx.doi.org/10.1083/jcb.200411179
- Thumkeo D, Keel J, Ishizaki T, Hirose M, Nonomura K, Oshima H, Oshima M, Taketo MM, Narumiya S. Targeted disruption of the mouse rho-associated kinase 2 gene results in intrauterine growth retardation and fetal death. Mol Cell Biol 2003; 23:5043-55; PMID:12832488; http://dx.doi.org/10.1128/MCB.23.14.5043-5055.2003
- Gao Y, Dickerson JB, Guo F, Zheng J, Zheng Y. Rational design and characterization of a Rac GTPase-specific small molecule inhibitor. Proc Natl Acad Sci U S A 2004; 101:7618-23; PMID:15128949; http://dx.doi.org/10.1073/pnas.0307512101
- Shutes A, Onesto C, Picard V, Leblond B, Schweighoffer F, Der CJ. Specificity and mechanism of action of EHT 1864, a novel small molecule inhibitor of Rac family small GTPases. J Biol Chem 2007; 282:35666-78; PMID:17932039; http://dx.doi.org/10.1074/jbc.M703571200
- Rosenblatt AE, Garcia MI, Lyons L, Xie Y, Maiorino C, Desire L, Slingerland J, Burnstein KL. Inhibition of the Rho GTPase, Rac1, decreases estrogen receptor levels and is a novel therapeutic strategy in breast cancer. Endocr Relat Cancer 2011; 18:207-19; PMID:21118977
- Zins K, Gunawardhana S, Lucas T, Abraham D, Aharinejad S. Targeting Cdc42 with the small molecule drug AZA197 suppresses primary colon cancer growth and prolongs survival in a preclinical mouse xenograft model by downregulation of PAK1 activity. J Transl Med 2013; 11:295; PMID:24279335; http://dx.doi.org/10.1186/1479-5876-11-295
- Yang X, Di J, Zhang Y, Zhang S, Lu J, Liu J, Shi W. The Rho-kinase inhibitor inhibits proliferation and metastasis of small cell lung cancer. Biomed Pharmacother 2012; 66:221-7; PMID:22425182; http://dx.doi.org/10.1016/j.biopha.2011.11.011
- Zhang C, Zhang S, Zhang Z, He J, Xu Y, Liu S. ROCK has a crucial role in regulating prostate tumor growth through interaction with c-Myc. Oncogene 2013; 33:5582-91; PMID:24317511; http://dx.doi.org/10.1038/onc.2013.505
- Kumar MS, Hancock DC, Molina-Arcas M, Steckel M, East P, Diefenbacher M, Armenteros-Monterroso E, Lassailly F, Matthews N, Nye E, et al. The GATA2 transcriptional network is requisite for RAS oncogene-driven non-small cell lung cancer. Cell 2012; 149:642-55; PMID:22541434; http://dx.doi.org/10.1016/j.cell.2012.02.059
- Nakabayashi H, Shimizu K. HA1077, a Rho kinase inhibitor, suppresses glioma-induced angiogenesis by targeting the Rho-ROCK and the mitogen-activated protein kinase kinase/extracellular signal-regulated kinase (MEK/ERK) signal pathways. Cancer Sci 2011; 102:393-9; PMID:21166955; http://dx.doi.org/10.1111/j.1349-7006.2010.01794.x
- Wozniak MA, Desai R, Solski PA, Der CJ, Keely PJ. ROCK-generated contractility regulates breast epithelial cell differentiation in response to the physical properties of a three-dimensional collagen matrix. J Cell Biol 2003; 163:583-95; PMID:14610060; http://dx.doi.org/10.1083/jcb.200305010
- Boyd NF, Lockwood GA, Byng JW, Tritchler DL, Yaffe MJ. Mammographic densities and breast cancer risk. Cancer Epidemiol Biomarkers Prev 1998; 7:1133-44; PMID:9865433
- Lisanti MP, Tsirigos A, Pavlides S, Reeves KJ, Peiris-Pages M, Chadwick AL, Sanchez-Alvarez R, Lamb R, Howell A, Martinez-Outschoorn UE, et al. JNK1 stress signaling is hyper-activated in high breast density and the tumor stroma: connecting fibrosis, inflammation, and stemness for cancer prevention. Cell Cycle 2014; 13:580-99; PMID:24434780; http://dx.doi.org/10.4161/cc.27379
- Ponik SM, Trier SM, Wozniak MA, Eliceiri KW, Keely PJ. RhoA is down-regulated at cell-cell contacts via p190RhoGAP-B in response to tensional homeostasis. Mol Biol Cell 2013; 24:1688-99; S1-3; PMID:23552690; http://dx.doi.org/10.1091/mbc.E12-05-0386
- Croft DR, Sahai E, Mavria G, Li S, Tsai J, Lee WM, Marshall CJ, Olson MF. Conditional ROCK activation in vivo induces tumor cell dissemination and angiogenesis. Cancer Res 2004; 64:8994-9001; PMID:15604264; http://dx.doi.org/10.1158/0008-5472.CAN-04-2052
- Cicchi R, Kapsokalyvas D, De Giorgi V, Maio V, Van Wiechen A, Massi D, Lotti T, Pavone FS. Scoring of collagen organization in healthy and diseased human dermis by multiphoton microscopy. J Biophotonics 2010; 3:34-43; PMID:19771581; http://dx.doi.org/10.1002/jbio.200910062
- Timpson P, McGhee EJ, Erami Z, Nobis M, Quinn JA, Edward M, Anderson KI. Organotypic collagen I assay: a malleable platform to assess cell behaviour in a 3-dimensional context. J Vis Exp 2011, (56):e3089; PMID:22025017; http://dx.doi.org/10.3791/3089
- Gaggioli C, Hooper S, Hidalgo-Carcedo C, Grosse R, Marshall JF, Harrington K, Sahai E. Fibroblast-led collective invasion of carcinoma cells with differing roles for RhoGTPases in leading and following cells. Nat Cell Biol 2007; 9:1392-400; PMID:18037882; http://dx.doi.org/10.1038/ncb1658
- Yamamoto M, Quantock AJ, Young RD, Okumura N, Ueno M, Sakamoto Y, Kinoshita S, Koizumi N. A selective inhibitor of the Rho kinase pathway, Y-27632, and its influence on wound healing in the corneal stroma. Mol Vis 2012; 18:1727-39; PMID:22815626
- Rath N, Olson MF. Rho-associated kinases in tumorigenesis: re-considering ROCK inhibition for cancer therapy. EMBO Rep 2012; 13:900-8; PMID:22964758; http://dx.doi.org/10.1038/embor.2012.127
- Brownfield DG, Venugopalan G, Lo A, Mori H, Tanner K, Fletcher DA, Bissell MJ. Patterned collagen fibers orient branching mammary epithelium through distinct signaling modules. Curr Biol 2013; 23:703-9; PMID:23562267; http://dx.doi.org/10.1016/j.cub.2013.03.032
- Scott RW, Hooper S, Crighton D, Li A, Konig I, Munro J, Trivier E, Wickman G, Morin P, Croft DR, et al. LIM kinases are required for invasive path generation by tumor and tumor-associated stromal cells. J Cell Biol 2010; 191:169-85; PMID:20876278; http://dx.doi.org/10.1083/jcb.201002041
- Sanz-Moreno V, Gaggioli C, Yeo M, Albrengues J, Wallberg F, Viros A, Hooper S, Mitter R, Feral CC, Cook M, et al. ROCK and JAK1 signaling cooperate to control actomyosin contractility in tumor cells and stroma. Cancer Cell 2011; 20:229-45; PMID:21840487; http://dx.doi.org/10.1016/j.ccr.2011.06.018
- Cadamuro M, Nardo G, Indraccolo S, Dall'olmo L, Sambado L, Moserle L, Franceschet I, Colledan M, Massani M, Stecca T, et al. Platelet-derived growth factor-D and Rho GTPases regulate recruitment of cancer-associated fibroblasts in cholangiocarcinoma. Hepatology 2013; 58:1042-53; PMID:23505219; http://dx.doi.org/10.1002/hep.26384
- Goicoechea SM, Garcia-Mata R, Staub J, Valdivia A, Sharek L, McCulloch CG, Hwang RF, Urrutia R, Yeh JJ, Kim HJ, et al. Palladin promotes invasion of pancreatic cancer cells by enhancing invadopodia formation in cancer-associated fibroblasts. Oncogene 2014; 33:1265-73; PMID:23524582; http://dx.doi.org/10.1038/onc.2013.68
- Brown M, Roulson JA, Hart CA, Tawadros T, Clarke NW. Arachidonic acid induction of Rho-mediated transendothelial migration in prostate cancer. Br J Cancer 2014; 110:2099-108; PMID:24595005; http://dx.doi.org/10.1038/bjc.2014.99
- Reymond N, Im JH, Garg R, Vega FM, Borda d'Agua B, Riou P, Cox S, Valderrama F, Muschel RJ, Ridley AJ. Cdc42 promotes transendothelial migration of cancer cells through beta1 integrin. J Cell Biol 2012; 199:653-68; PMID:23148235; http://dx.doi.org/10.1083/jcb.201205169
- Caspani EM, Crossley PH, Redondo-Garcia C, Martinez S. Glioblastoma: a pathogenic crosstalk between tumor cells and pericytes. PLoS One 2014; 9:e101402; PMID:25032689; http://dx.doi.org/10.1371/journal.pone.0101402
- Wang Y, Nakayama M, Pitulescu ME, Schmidt TS, Bochenek ML, Sakakibara A, Adams S, Davy A, Deutsch U, Luthi U, et al. Ephrin-B2 controls VEGF-induced angiogenesis and lymphangiogenesis. Nature 2010; 465:483-6; PMID:20445537; http://dx.doi.org/10.1038/nature09002
- Bhowmick NA, Chytil A, Plieth D, Gorska AE, Dumont N, Shappell S, Washington MK, Neilson EG, Moses HL. TGF-beta signaling in fibroblasts modulates the oncogenic potential of adjacent epithelia. Science 2004; 303:848-51; PMID:14764882; http://dx.doi.org/10.1126/science.1090922
- Cheng N, Bhowmick NA, Chytil A, Gorksa AE, Brown KA, Muraoka R, Arteaga CL, Neilson EG, Hayward SW, Moses HL. Loss of TGF-beta type II receptor in fibroblasts promotes mammary carcinoma growth and invasion through upregulation of TGF-alpha-, MSP- and HGF-mediated signaling networks. Oncogene 2005; 24:5053-68; PMID:15856015; http://dx.doi.org/10.1038/sj.onc.1208685
- Cheng N, Chytil A, Shyr Y, Joly A, Moses HL. Enhanced hepatocyte growth factor signaling by type II transforming growth factor-beta receptor knockout fibroblasts promotes mammary tumorigenesis. Cancer Res 2007; 67:4869-77; PMID:17495323; http://dx.doi.org/10.1158/0008-5472.CAN-06-3381
- Alva JA, Zovein AC, Monvoisin A, Murphy T, Salazar A, Harvey NL, Carmeliet P, Iruela-Arispe ML. VE-Cadherin-Cre-recombinase transgenic mouse: a tool for lineage analysis and gene deletion in endothelial cells. Dev Dyn 2006; 235:759-67; PMID:16450386; http://dx.doi.org/10.1002/dvdy.20643
- Gustafsson E, Brakebusch C, Hietanen K, Fassler R. Tie-1-directed expression of Cre recombinase in endothelial cells of embryoid bodies and transgenic mice. J Cell Sci 2001; 114:671-6; PMID:11171372
- Kisanuki YY, Hammer RE, Miyazaki J, Williams SC, Richardson JA, Yanagisawa M. Tie2-Cre transgenic mice: a new model for endothelial cell-lineage analysis in vivo. Dev Biol 2001; 230:230-42; PMID:11161575; http://dx.doi.org/10.1006/dbio.2000.0106