Abstract
The ability of tumors to evade detection by the immune system via inducing immunosuppression prompted the therapeutic development of immune checkpoint inhibitors. In our recent review, we discussed findings from preclinical and clinical investigations of these agents utilized in combination with radiation inducing abscopal (systemic) antitumor effects.
In our recent publication in Cancer Immunology Research, we reviewed the clinical potential of combining immunomodulatory agents with radiation as a systemic treatment for solid tumors.Citation1 As a first step, we considered the effects of radiation alone on the tumor microenvironment. Irradiation of tumors is well known to stimulate the production of immune-activating signals such as Fas ligand, MHC-1 and T-cell recruiting chemokines, simultaneously with immune-inhibitory signals such as programmed cell death ligand 1 (PDL1 [CD274]) and transforming growth factor β (TGFβ).Citation2,3 The immune system is also known to be integral in eliciting tumor responses to radiation. For example, Lee and colleagues showed that the antitumor effects of ablative radiation (20 Gy given in a single fraction) to mice implanted with melanoma tumor cells required the presence of activated CD8+ T cells; indeed, in mice that lacked such T cells, the implanted tumors were resistant to such radiation.Citation4 The knowledge that radiation can elicit strong cytotoxic T-lymphocyte responses led pioneers of this field such as Silvia Formenti to hypothesize that radiation may act as an “in-situ vaccine” that causes CD8+ T-cell priming by tumor antigens.Citation2 In other words, activated lymphocytes can not only promote local tumor regression (in the irradiated tumor) but also help to provide distant control of unirradiated tumors, a phenomenon commonly referred to as the abscopal response. These findings have prompted renewed interest in evaluating immunologic function of patients with cancer in terms of its influence on clinical radiation outcomes.
We recently found that absolute lymphocyte counts during chemoradiation for non-small lung cancer were inversely proportional to lung V5, the volume of normal lung exposed to ≥5 Gy of radiation.Citation5 We also found that lymphopenia during treatment correlated with inferior overall and event-free survival. Experiments to reveal the ideal fractionation and amount of radiation needed to enhance systemic immunity against local and distant tumors are currently ongoing. Nonetheless, these studies highlight the importance of the immune system during radiotherapy for solid tumors.
Tumors escape immune-mediated detection and killing by inducing a variety of cytokine and ligand signals to dampen the lymphocyte response. The first of these immunomodulating molecules, CTLA-4 [cytotoxic T-lymphocyte-associated protein 4, also known as CD152], was discovered by James Allison. Soon after, discovery of other immunostimulatory (e.g., OX40 [also known as CD134], CD137) and deactivating agents (e.g., PD1 [programmed cell death 1, also known as CD279]) followed. These discoveries led to the development of targeted therapies involving humanized antibodies such nivolumab (anti-PD1), ipilimumab (anti-CTLA4), and MDX-1105 (anti-PDL1). Initial trials testing ipilimumab and nivolumab as immunotherapeutic agents against various types of solid tumors have demonstrated promising results.Citation6,7
Preclinical experiments on the combination of radiation and immunotherapy have shed additional light on how radiation affects the tumor environment. Deng and colleagues observed that PDL1 levels in the tumor microenvironment increased after irradiation of tumors in mice and that adding a PDL1 inhibitor to irradiation led to further decreases in tumor volume via heightened CD8+ T-cell responses.Citation3 They further proved that this effect resulted from a decrease in the accumulation of tumor-infiltrating myeloid-suppressor cells, and that that decrease was related to increases in tumor necrosis factor α (TNFα) released from CD8+ T cells. Another group recently published findings implicating galectin-1, a β-galactoside-binding protein expressed by tumors, in the effects of radiation and CD8+ T-cell apoptosis in a model of non-small lung cancer in mice.Citation8 They found that galectin-1 levels increased during radiation therapy and promoted CD8+ T-cell apoptosis, and that inhibiting or reducing expression of gal-1 during radiation significantly improved CD8+ T-cell counts and reduced rates of lung metastasis. On the basis of these and other preclinical findings, clinical trials combining radiation and immunotherapy are ongoing at several institutions. In one case report, a woman who presented with melanoma that had metastasized to several sites was given concurrent ipilimumab and radiotherapy to a spinal lesion. Several months after treatment, a clear abscopal response was apparent, with complete resolution of lesions at other non-irradiated sites.Citation9 Results such as these demonstrate the potential of immunoradiation as a systemic treatment for cancer.
One future application of immunotherapy and radiation in the treatment of solid tumors could involve personalization of treatment according to characteristics of individual patients’ systemic immunity and their tumors’ capacity for instigating a durable immune response. In this way, patients could be stratified by immunogenic phenotype and their treatments tailored accordingly. For example, patients with a weaker immunogenic phenotype might benefit from surgery alone, but patients with a strong immunogenic phenotype could be treated with radiation and immunostimulatory agents, which may confer a higher likelihood of local and distant control via antigen-activated lymphocytes. How patients with intermediate immunogenicity would be treated is currently unknown. With the FDA approval of anti-PD1 and anti-CTLA4 antibodies, one potential approach could be to combine several immune checkpoint inhibitors, with the goal of shifting patients from intermediate immunogenicity to stronger immunogenicity. The idea of using more than one immunotherapy agent with radiation raises the question of their optimal sequencing (). Young and colleagues found that mice with CT26 colorectal adenocarcinoma tumors given either anti-CTLA4 antibody before or an OX40 agonist after ablative radiation (20 Gy in 1 fraction) survived longer and had higher CD8+ T-cell counts than control mice (Oral Presentation 102, ASTRO 56th Annual Meeting, San Francisco, September 2014).
Figure 1. Rationale for temporal sequencing of various immunotherapies with radiation. (A) Preclinical experiments suggest that neoadjuvant anti-CTLA4 antibody can augment tumor growth delay if given before radiation and that this effect could continue throughout treatment. Thus, giving anti-CTLA4 therapy before radiation would activate CD8+ T cells in a non-irradiated lymph node as shown. (B) Anti-PD1 and anti-PDL1 antibodies act directly at the tumor site, suggesting that these immunotherapeutic agents would need to be present during radiation delivery; thus concurrent administration would probably be the most effective. (C) An OX40 agonist induces CD8+ T-cell activation to the greatest extent when given after radiation; thus adjuvant delivery after radiotherapy may be the most beneficial for inducing systemic immune responses.
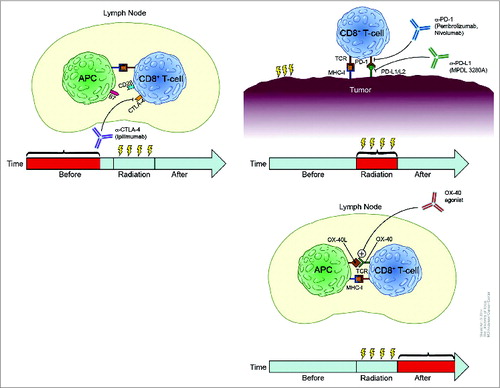
Although the concept of classifying patients by immunogenic phenotype is intriguing, the optimal method for doing so is unclear, and biomarkers are clearly needed to help address this issue. A group at Arizona State University recently developed an “immunosignature” assay involving antibodies from blood samples being incubated with a microarray of 10,000 random sequence peptides.Citation10 Knowing that cancers produce antigens that stimulate B cells to generate anti-tumor antibodies, this group analyzed serum samples from 100 patients with 5 different types of cancer, and 20 patients without cancer, and discovered peptide signatures that accurately identified the type of cancer in more than 98% of cases. Techniques such as these could help to classify patients into groups based on immunologic phenotype that are or are not likely to benefit from immunotherapy. The potential ability of localized radiation to induce “neo” antigens in combination with immunomodulating agents that stimulate T-cell responses could change the scope of radiation therapy from local to systemic.
Disclosure of Potential Conflicts of Interest
No potential conflicts of interest were disclosed.
References
- Tang C, Wang X, Soh H, Seyedin S, Cortez MA, Krishnan S, Massarelli E, Hong D, Naing A, Diab A, et al. Combining radiation and immunotherapy: a new systemic therapy for solid tumors? Cancer Immunol Res 2014; 2:831-8; PMID:25187273; http://dx.doi.org/10.1158/2326-6066.CIR-14-0069
- Formenti SC, Demaria S. Combining radiotherapy and cancer immunotherapy: a paradigm shift. J Natl Cancer Inst 2013; 105:256-65; PMID:23291374; http://dx.doi.org/10.1093/jnci/djs629
- Deng L, Liang H, Burnette B, Beckett M, Darga T, Weichselbaum RR, Fu YX. Irradiation and anti-PD-L1 treatment synergistically promote antitumor immunity in mice. J Clin Invest 2014; 124:687-95; PMID:24382348; http://dx.doi.org/10.1172/JCI67313
- Lee Y, Auh SL, Wang Y, Burnette B, Wang Y, Meng Y, Beckett M, Sharma R, Chin R, Tu T, et al. Therapeutic effects of ablative radiation on local tumor require CD8+ T cells: changing strategies for cancer treatment. Blood 2009; 114:589-95; PMID:19349616; http://dx.doi.org/10.1182/blood-2009-02-206870
- Tang C, Liao Z, Gomez D, Levy L, Zhuang Y, Gebremichael RA, Hong DS, Komaki R, Welsh JW. Lymphopenia association with gross tumor volume and lung v5 and its effects on non-small cell lung cancer patient outcomes. Int J Radiat Oncol Biol Phys 2014; 89:1084-91; PMID:25035212; http://dx.doi.org/10.1016/j.ijrobp.2014.04.025
- Hodi FS, O'Day SJ, McDermott DF, Weber RW, Sosman JA, Haanen JB, Gonzalez R, Robert C, Schadendorf D, Hassel JC, et al. Improved survival with ipilimumab in patients with metastatic melanoma. N Engl J Med 2010; 363:711-23; PMID:20525992; http://dx.doi.org/10.1056/NEJMoa1003466
- Topalian SL, Hodi FS, Brahmer JR, Gettinger SN, Smith DC, McDermott DF, Powderly JD, Carvajal RD, Sosman JA, Atkins MB, et al. Safety, activity, and immune correlates of anti-PD-1 antibody in cancer. N Engl J Med 2012; 366:2443-54; PMID:22658127; http://dx.doi.org/10.1056/NEJMoa1200690
- Kuo P, Bratman S, Shultz D, von Eyben R, Chan C, Wang Z, Say C, Gupta A, Loo B, Giaccia AJ, et al. Galectin-1 mediates radiation-related lymphopenia and attenuates NSCLC radiation response. Clin Cancer Res 2014; 20:5558-69; PMID:25189484; http://dx.doi.org/10.1158/1078-0432.CCR-14-1138
- Postow MA, Callahan MK, Barker CA, Yamada Y, Yuan J, Kitano S, Mu Z, Rasalan T, Adamow M, Ritter E, et al. Immunologic correlates of the abscopal effect in a patient with melanoma. N Engl J Med 2012; 366:925-31; PMID:22397654; http://dx.doi.org/10.1056/NEJMoa1112824
- Stafford P, Cichacz Z, Woodbury NW, Johnston SA. Immunosignature system for diagnosis of cancer. Proc Natl Acad Sci U S A 2014; 111:E3072-80; PMID:25024171; http://dx.doi.org/10.1073/pnas.1409432111