Abstract
The inability to target cancer stem cells (CSC) may be a significant factor contributing to treatment failure. We have developed a strategy to target the CSC populations in melanoma and squamous cell carcinoma using CSC lysate-pulsed dendritic cells (DCs). The CSC-DC vaccine was administered in the adjuvant setting after localized radiation therapy of established tumors. Using mouse models we demonstrated that DCs pulsed with CSCs enriched by virtue of their expression of the CSC marker ALDH (termed CSC-DC) significantly inhibited tumor growth, reduced development of pulmonary metastases and prolonged survival. The effect was associated with downregulation of chemokine (C-C motif) receptors CCR7 and CCR10 in tumor cells and decreased expression of the chemokine (C-C motif) ligands CCL21, CCL27 and CCL28 in lung tissue. The CSC-DC vaccine significantly reduced ALDHhigh CSC frequency in primary tumors. Direct targeting of CSCs was demonstrated by the specific binding of IgG produced by ALDHhigh CSC-DC vaccine-primed B cells to ALDHhigh CSCs, resulting in lysis of these target CSCs in the presence of complement. These data suggest that the CSC-DC vaccine approach may be useful in the adjuvant setting where local and systemic relapse are high after conventional treatment of cancers.
Introduction
Despite recent advances in immunologic therapy of cancer, the overall efficacy of these approaches remains limited.Citation1-6 Cancer stem cells (CSCs) mediate tumor metastasis by virtue of their relative resistance to chemotherapy and radiation therapy and contribute to relapse following treatment.Citation7,8 The inability to target CSCs with current therapies may be a significant factor for treatment failures. Current immunotherapies are primarily directed against tumor differentiation antigens, and thus, preferentially target differentiated cells rather than the CSC population lacking these antigens. On the other hand, CSCs express antigens which may be different from either differentiated tumor cell antigens or normal initiating cell antigens.Citation9 Novel therapeutic strategies are needed to target CSCs.
Several groups have described the generation of CSC-specific CD8+ T cells in vitro;Citation10,11 the killing of CSCs via non-specific immune cellsCitation12,13 as well as by oncolytic virusesCitation14 and antibodies.Citation15 We have reported that CXCR1 blockade selectively targeted human breast CSCs in vitro and in xenografts.Citation16 Nevertheless, the strategies designed to specifically target CSCs in vivo remain largely unexplored. To this end, a CSC-based vaccine may represent a novel effort.
ALDH (aldehyde dehydrogenase) activity, often measured via ALDEFLUOR assay, has been successfully used as a marker to enrich CSC populationsCitation11,17-22 in a variety of cancers including human melanomaCitation23 and head and neck squamous cell cancer.Citation18 We characterized CSC-enriched populations in 2 histologically distinct murine tumors (melanoma D5 and squamous cell cancer SCC7) and evaluated their immunogenicity by administering CSC-based vaccines in 2 genetically different syngeneic immunocompetent hosts followed by tumor challenge.Citation22 D5 and SCC7 cells contain approximately 5–10% ALDHhigh CSCs.Citation22 We obtained cell lysate from ALDHhigh D5 or SCC7 CSCs to pulse dendritic cells (DCs) that were subsequently used as a vaccine (termed CSC-DCs). DCs pulsed with unsorted heterogeneous D5 or SCC7 tumor cell lysate (H-DC), or pulsed with ALDHlow D5 or SCC7 non-CSC lysate (ALDHlow-DC) served as controls. Vaccination with ALDHhigh CSC-DC in immunocompetent mice significantly prevented lung metastasis and s.c tumor growth as compared with heterogeneous, unsorted cell lysate-pulsed dendritic cells (termed H-DCs)Citation2,6 Importantly, the CSC-DC vaccine inhibited tumor growth significantly more than ALDHlow-DC vaccination or H-DC vaccination in recipient mice implanted with either tumor model. These results indicate that enriched ALDHhigh CSCs are immunogenic and more effectively induce protective immunity against a tumor challenge than bulk tumor cells or ALDHlow tumor cells.
In this report, we evaluate the therapeutic efficacy of the CSC-DC vaccine in the setting of localized tumor radiation therapy (RT), and explore the mechanisms by which CSC-DC vaccine-induces immunity to target CSCs.
Results
Therapeutic efficacy of a CSC-DC vaccine
Our previous study has demonstrated that administration of ALDHhigh CSC-DC vaccine in the normal host can induce significant protection against tumor challenge.Citation22 In patients with locally advanced cancers wherein surgery is not the primary therapy, radiation therapy and/or chemotherapy may be offered as first-line treatment. We therefore examined the therapeutic efficacy of a CSC-DC vaccine in the treatment of established disease in which tumor irradiation is given. We hypothesized that CSC-based vaccines might be able to increase the efficacy of RT by targeting radiation resistant CSCs. To test this, we established D5 s.c. tumors, and treated the tumor-bearing mice with RT and DC vaccination as described in the Materials and Methods. Each vaccination included ALDHhighCSC-stimulated DCs (CSC-DCs) vs. ALDHlowCSC-stimulated DCs (ALDHlowDCs) and control H-DCs. The combination of RT and CSC-DC vaccine significantly decreased tumor burden () as compared with PBS treatment (P=0.0261); RT only (P=0.0227); RT plus ALDHlow-DC vaccination (P=0.0439) or RT plus H-DC (P=0.047). While CSC-DC treatment demonstrated significant advantages over the H-DC treatment, there was no significant difference between the ALDHlow-DC group and the H-DC group. The survival of RT plus CSC-DC vaccinated hosts was significantly prolonged (P < 0.03, RT + CSC-DC vs. all other groups, ).
Figure 1. Immunotherapeutic potential of cancer stem cell-stimulated dendritic cells. A cancer stem cell-dendritic cell (CSC-DC) vaccine significantly augments the therapeutic efficacy of local tumor radiation therapy (RT) in the established D5 melanoma model (A, B) and SCC7 squamous cell carcinoma model (C, D). (A, C) Mice (n = 5–11 mice/group) bearing 5-day established sc. tumors were subject to treatment with PBS, RT alone, RT plus heterogeneous DCs (H-DCs), RT plus ALDHlow-DCs or RT plus ALDHhigh-DCs (CSC-DCs) vaccine, as indicated. Treatment was repeated on day 12 and 19 respectively. Tumor volume (mean ± SEM) is shown. (B, D) Survival curves of tumor-bearing mice (n = 5–11 mice/group) subject to PBS, RT alone, RT plus H-DC, RT plus ALDHlow-DC or RT plus ALDHhigh-DC (CSC-DC) vaccine, respectively. Data are representative of 3 experiments performed.
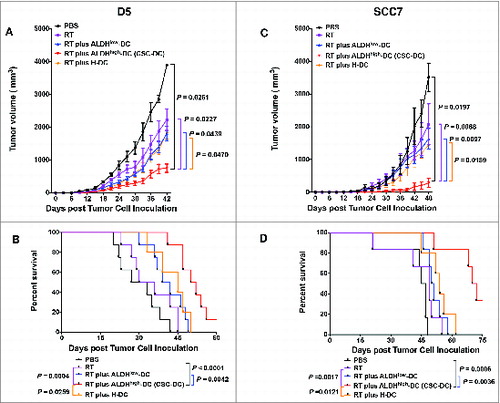
We conducted similar experiments utilizing established SCC7 tumors in the C3H hosts. SCC7 s.c. tumors were treated with localized RT followed by the CSC-DC vaccine in a similar schedule to that used for the treatment of established D5 tumors in the B6 mice. Therapeutic efficacy was compared between the groups subject to radiotherapy in addition to equal numbers of DCs pulsed with the lysate of ALDHhigh SCC7 CSCs (CSC-DCs) , ALDHlow SCC7 cells (ALDHlow-DCs) or unsorted heterogeneous SCC7 tumor cells (H-DCs). Growth of subcutaneous tumors in mice subjected to RT plus CSC-DC vaccine was significantly reduced () (P < 0.02 vs. all other groups). Furthermore, RT plus ALDHhigh-DC vaccine significantly improved survival (, P < 0.005 vs. all other groups). Of note, mice treated with RT plus ALDHhigh-DC vaccine had a 20.5-day survival advantage over the RT plus ALDHlow-DC vaccinated mice (P=0.0036), and a 17-day survival advantage over the RT plus H-DC vaccinated mice (P=0.0121). These results demonstrated that ALDHhigh CSC-DC vaccination significantly augmented the therapeutic efficacy of RT as evinced by significantly inhibited tumor growth as well as improved overall survival against established tumors as compared with ALDHlow-DC vaccination. This increment of effectiveness was similar to that observed upon comparison of the CSC-DC group with the H-DC group. This was true in both melanoma D5 and squamous cell carcinoma SCC7 tumor models.
CSC-DC vaccination prevents pulmonary metastasis
Prevention of metastasis has the greatest potential to improve patient outcome. Although local recurrence contributes to significant morbidity, it is the development of distant metastasis that is the leading cause of death in both head and neck cancer and melanoma.Citation24-27 We hypothesized that one of the mechanisms underlining ALDHhigh CSC-DC vaccine-conferred therapeutic efficacy (as demonstrated in ) may involve the inhibition of tumor metastasis. To test this possibility, we examined D5 metastatic lung tumor burden at the end of these experiments.
Following the treatment of established disease, we harvested the lungs and assessed the D5 tumor metastasis. RT plus ALDHhigh CSC-DC vaccine significantly inhibited lung metastasis, as compared with PBS, RT alone or RT plus ALDHlow-DC treatment (P < 0.05, ). Ten, 9, and 8 of a total of 11 mice in the PBS, RT alone, and RT plus ALDHlow-DC treated groups, respectively, developed lung metastases (). However, only 2 of 11 mice in the RT plus CSC-DC vaccinated group developed lung metastases. Massive tumor lesions were observed in the lungs harvested from PBS or RT alone treated hosts (). Mice subject to RT plus ALDHlow-DC treatment also showed multiple tumor lesions in the lungs. However, no tumor lesions were found in the RT plus ALDHhigh CSC-DC vaccinated group. These results indicate that CSC-DC vaccination significantly inhibited the development of lung metastases in the established disease setting. Further, administration of the CSC-DC vaccine was associated with significantly prolonged survival ().
Figure 2. CSC-DC vaccine inhibits the lung metastasis in the setting of local tumor irradiation in the established D5 model. The cancer stem cell-dendritic cell (CSC-DC) vaccine plus local tumor radiation therapy (RT) inhibits D5 melanoma metastatic spread. (A) P values comparing mice (n = 11/group) developing lung metastases (as determined by histological examination) in response to phosphate buffered saline (PBS), RT, RT plus ALDHlow-DC vaccine or RT plus ALDHhigh- DC vaccine. RT plus ALDHhigh- DC (CSC-DC) significantly inhibited distant lung metastasis. Statistical analysis was performed using the Fisher exact test. (B) Representative H&E staining of histologic specimens of lung tissues harvested from mice subjected to PBS, RT, RT plus ALDHlow-DC vaccine or RT plus ALDHhigh- DC vaccine. The histology of lung tissue harvested from normal B6 mice served as a control. The red arrows point to the tumor lesions in the lung tissues (magnification, 100 ×).
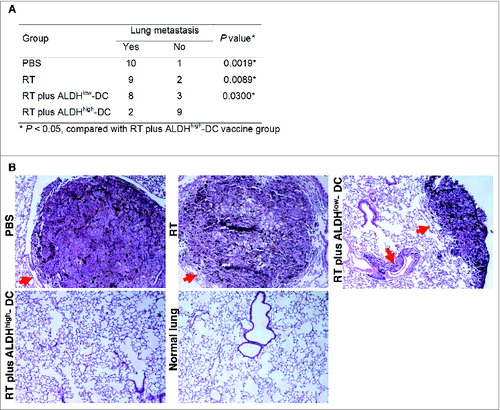
Cytokines have been demonstrated to play a substantial role in tumor metastasis.Citation28-30 Thus, we examined the expression of several chemokine receptors and their corresponding ligands. RT plus CSC-DC significantly reduced the expression of chemokine (C-C motif) receptor 10 (CCR10; P < 0.01 vs. all other groups, ). Tumor cells from RT alone treatment (24.1%, ) or RT plus ALDHlow-DC vaccine (21.3%) demonstrated moderately decreased expression of CCR10 as compared with the PBS control (41.7%). However, the RT plus ALDHhigh CSC-DC vaccine treatment combination significantly reduced the expression of CCR10 on D5 tumor cells to 4%. We also found significantly decreased expression of chemokine (C-C motif) receptor 10 (CCR7) after RT plus CSC-DC vaccination (P < 0.01 vs. all other groups, ). Specifically, after PBS, RT alone, or RT plus ALDHlow-DC vaccination, the expression levels of CCR7 were 27.9%, 16.4%, and 13.8%, respectively. In contrast, expression of CCR7 on D5 tumor cells was significantly reduced to 2.1% in the RT plus CSC-DC vaccination group ().
Figure 3. Downregulation of chemokine receptor CCR10 following radiation therapy plus CSC-DC vaccination in the established D5 melanoma model. D5 melanoma tumors from mice treated with phosphate buffered saline (PBS), radiotherapy (RT), RT plus ALDHlow-DC or RT plus ALDHhigh-DC (CSC-DC) were dissociated and tumor cells stained using with phycoerythrin (PE) conjugated anti-CCR10 or isotype control antibodies. The expression levels of the chemokine receptor were detected by cytofluorometric analysis. The experiment was repeated at least 3 times using the tumor cells harvested from at least 3 animals in each experiment group. (A) The bar graph shows the mean +/− SE. (B) Flow cytometry histograms were generated by mixing the cell suspensions from different hosts but in the same treatment group.
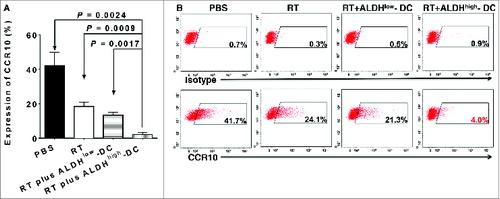
Figure 4. RT plus CSC-DCs significantly decreased CCR7 expression on primary s.c D5 tumor cells. D5 melanoma tumors from mice treated with phosphate buffered saline (PBS), radiotherapy (RT), RT plus ALDHlow-DC or RT plus ALDHhigh-DC (CSC-DC) were dissociated and tumor cells stained using with phycoerythrin (PE) conjugated anti-CCR7 or isotype control antibodies. The expression levels of the chemokine receptor were detected by cytofluorometric analysis. (A) The bar graph shows the mean +/− SE. (B) Flow cytometry histograms using mixed D5 cells harvested from multiple animals in each treatment group. Data are representative of 3 experiments performed.
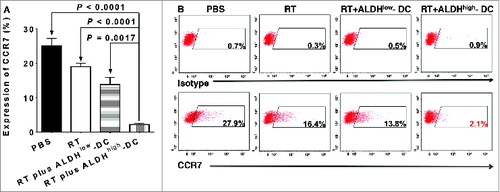
Coincident with the above analysis, we also carried out quantitative RT-PCR (qRT-PCR) to detect the expression levels of the corresponding chemokine (C-C motif) ligands in lung tissues of treated mice. There was a significant decrease in the expression of CCL21 (the ligand for CCR7) between the RT plus CSC-DC vaccination group and all other treatments (, P < 0.02 vs. all other groups). Furthermore, the expression levels of CCR10 cognate ligands, CCL27 () and CCL28 (), were both significantly decreased after RT plus ALDHhigh CSC-DC vaccine treatment (P < 0.02 vs. all other groups).
Figure 5. RT plus CSC-DC vaccine significantly reduced the mRNA levels of CCL21, CCL27 and CCL28 in the lung tissues harvested from D5-bearing host. Analysis of the expression levels of mRNA encoding the corresponding (C-C motif) chemokines for CCR7 and CCR10 in the lung tissues collected from treated D5 tumor-bearing mice by using real-time quantitative PCR. The mRNA expression of CCL21 (ligand of CCR7) (A), CCL27 (B) and CCL28 (C) (ligands of CCR10) in the lung tissues harvested from mice subjected to phosphate buffered saline (PBS), radiotherapy (RT) alone, RT plus ALDHlow-DC or RT plus ALDHhigh-DC (CSC-DC) treatment, respectively, were evaluated. Data were repeated in a second experiment.
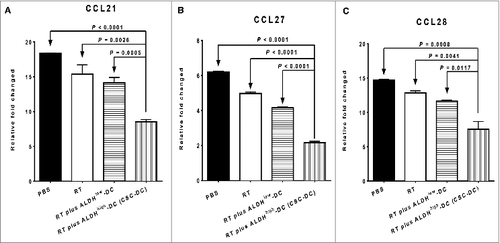
These data suggest that CSC-DC vaccination may inhibit pulmonary metastasis of the local tumor by significantly downregulating the expression of CCR7 and CCR10 on primary tumor cells and by reducing the production of their ligands (e.g., CCL21, CCL27 and CCL28) in the lung.
CSC-DC vaccine treatment reduces the ALDHhigh CSCs in the primary tumor
To provide direct evidence that CSC-DC vaccine can induce anti-CSC immunity by targeting CSCs, we assessed the percentage of ALDHhigh cells in the s.c tumors harvested from mice subjected to CSC-DC treatment. Assessment of the ALDHhigh population in tumors from multiple mice per group was performed essentially as previously describedCitation22 and the results displayed using standard error (). In addition, we mixed the tumor cells from multiple mice of each experimental group, and generated representative cytofluorometric graphs to demonstrate the ALDHhigh populations in each group (). As shown in , while radiation therapy (RT) alone increased the percentage of ALDHhigh cells in the treated primary tumor, RT plus CSC-DC significantly reduced the percentage of ALDHhigh cells as compared with PBS, RT alone, or RT plus ALDHlow-DC (P = 0.0018, P = 0.0018 and P = 0.0096, respectively). Distinctively, primary s.c. tumors harvested from RT plus CSC-DC vaccinated mice were found to contain significantly less ALDHhigh cells (<3%) as compared with PBS (14.2%), RT alone (20.5%), or RT plus ALDHlow-DC (12.8%) treated mice ().
Figure 6. RT plus CSC-DC vaccine treatment significantly decreased the percentage of ALDHhigh cells in D5 melanoma tumors. Cytofluorimetric analysis of the percentage of ALDHhigh cells in D5 melanoma tumors from mice treated with phosphate buffered saline (PBS), radiotherapy (RT), RT plus ALDHlow-DC or RT plus ALDHhigh-DC (CSC-DC). Primary tumors were dissociated and tumor cells were incubated with ALDEFLUOR with or without the ALDH inhibitor DEAB as the control. Experiments were repeated at least 3 times by using s.c tumors from multiple individual mice per treatment group (A) Shown are the mean +/− SE. (B) Mixed tumor cells from multiple mice of each treatment group were used to generate representative flow cytometry histograms. The experiments were repeated twice.
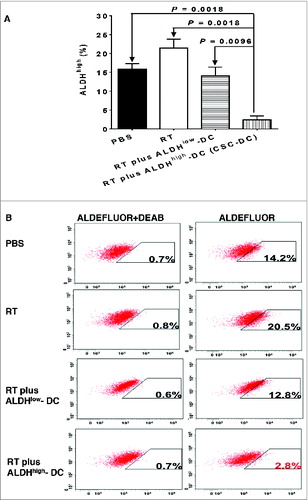
CSC-DC vaccine modulates host humoral responses specifically targeting CSCs
To determine the mechanism underlining CSC-DC vaccine-conferred reduction of ALDHhigh CSCs, we evaluate CSC-DC vaccine-induced antibody immune responses against CSCs. To this end, spleens were collected at the end of the treatments and dissociated. Purified splenic B cells were activated in vitro with lipopolysaccharide (LPS) and anti-CD40, and supernatants were collected. ELISA analysis was performed to detect IgG and IgM antibodies in the B cell culture supernatants. The level of IgG antibodies in the CSC-DC-primed B cell culture supernatant was much higher than other groups (data not shown). However, the concentration of IgM was very low and no significant differences were observed among the experimental groups (data not shown). To test the specificity of the CSC-DC vaccine-primed antibody, we assessed the binding of the immune supernatants to ALDHhigh D5 CSCs vs. ALDHlow D5 non-CSCs. In , culture supernatants of B cells collected from the various treated animals were used to test their binding to ALDHhigh D5 CSCs (left column of ) vs. their binding to ALDHlow D5 non-CSCs (right column of ) respectively. Fluorescein isothiocyanate (FITC) labeled anti-mouse IgG secondary antibody was then used for detection of immunoreactivity via fluorescence cytometry. As shown in , RT plus ALDHhigh CSC-DC vaccine-primed immune supernatant bound to ALDHhigh D5 CSCs (76.6%) significantly more than the supernatants collected from PBS (17.5%), RT alone (21.4%) or RT plus ALDHlow-DC (25.1%) treated hosts. In contrast, immune supernatants from RT plus ALDHlow-DC vaccine treated mice bound to ALDHlow D5 cells (69.8%) much more effectively than the supernatants generated from RT-treated mice (21%) or RT plus ALDHhigh-DC vaccine-primed immune supernatant (23.8%). Repeated experiments showed that RT plus ALDHhigh D5 CSC-DC vaccine-primed immune supernatants bound to ALDHhigh D5 CSCs significantly more than ALDHlow-DC vaccine-primed immune supernatants in the same setting of local tumor irradiation (P = 0.0008, ). In contrast, the ALDHlow-DC vaccine-primed immune supernatants bound to ALDHlow D5 cells much more effectively than the CSC-DC vaccine-primed immune supernatants (P = 0.0004, ).
Figure 7. CSC-based vaccination confers significantly systemic humoral response in the setting of local tumor irradiation. The specificity of the humoral immune response to CSCs was determined by assessing the binding abilities to ALDHhigh D5 CSCs (left column of A) vs. ALDHlow D5 non-CSCs (right column of A) of the immune supernatants of the cultured splenocytes harvested from mice subjected to phosphate buffered saline (PBS), radiation therapy (RT) alone, RT plus ALDHlow-DC or RT plus ALDHhigh-DCs, respectively as indicated. The culture supernatants of B cells from the cultured splenocytes were mixed from 5 mice of each treatment group (n = 5) to generate representative flow cytometry histograms (A). For statistical analysis, results from repeated experiments (each group contained 5 mice) showing the binding to ALDHhigh D5 CSCs were summarized in B to be consistent with the left column of A. Similarly, results from repeated experiments showing the binding to ALDHlow D5 cells were summarized in C to be consistent with the right column of A for statistical analysis.
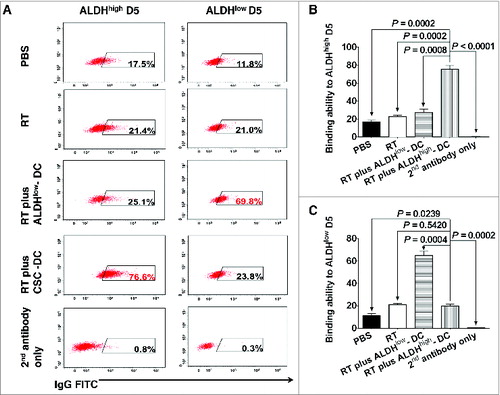
To understand the immunologic consequence of the binding of CSC-DC vaccine-primed antibody to CSCs, we performed antibody and complement-dependent cytotoxicity (CDC) assays directed against CSC targets. Immune supernatants generated from mice subjected to RT plus ALDHhigh CSC-DC vaccine were significantly more efficient mediators of ALDHhigh D5 CSC lysis (, P < 0.0001 vs. all other groups). In contrast, immune supernatants generated from mice subject to RT plus ALDHlow-DC vaccination were significantly more efficient mediators of ALDHlow D5 cell lysis (, P < 0.0001 vs. all other groups). These data support the conclusion that ALDHhigh D5 CSC-DC vaccine, in combination with RT to treat established D5 tumor, confers significant host anti-CSC immunity by producing D5 CSC-specific antibodies which bind and kill D5 CSCs.
Figure 8. CSC-DC vaccine-primed antibody selectively targets CSCs via complement-dependent cytotoxicity (CDC). Antibody and complement mediated cytotoxicity was measured by incubating viable ALDHhigh D5 cancer stem cells (CSCs) or ALDHlow D5 non-CSCs with immune B cell culture supernatants of the splenocytes harvested from D5 tumor-bearing hosts subjected to different therapies, as indicated. The data are expressed as the percentages of viable cells. The lower the percentage of viable cells, the higher the percentage of cell lysis. Data are representative of 3 experiments performed.
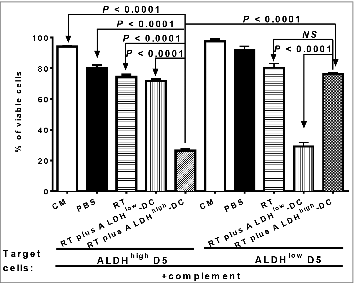
Discussion
Traditional DC vaccines target tumors that express tumor differentiation antigens. CSCs that do not express these differentiation antigens may escape immunological targeting. Despite tumor shrinkage following administration of conventional therapies, these tumors inevitably recur. Pre-clinical and clinical studies have demonstrated that CSCs are relatively resistant to chemotherapyCitation7,31 and radiation.Citation32-34 We presume that there will be minimal differences between the therapeutic efficacies of CSC-DC vaccination by itself vs. other treatments judged by tumor size in the established disease setting because the composition of the tumor harbors only a small fraction of CSCs.Citation22,35 Hence, we evaluated the therapeutic efficacy of CSC-DC vaccination on established tumors in the setting of local tumor irradiation. The initial therapy of established tumors with local radiation may result in destruction of non-CSC tumor cells with an increase in the percentage of CSCs. Our previous work suggested that CSC-targeted therapies may have greatest efficacy when they are employed in the adjuvant setting.Citation36 It is in this setting that subsequent CSC-DC vaccination may be of greatest therapeutic benefit by selectively targeting the CSCs resistant to traditional therapies. We thus used ALDHhigh CSC lysate-pulsed DC vaccine as an adjunctive treatment to radiation therapy of established tumors in this study. We found that RT plus ALDHhigh-DC (CSC-DC) significantly inhibited D5 tumor growth and prolonged animal survival. These observations were confirmed in a second model, SCC7. We then focused on the D5 model to investigate the potential mechanisms which may be involved in the induction of anti-CSC immunity by the CSC-DC vaccine.
Several studies have described the use of CSCs as a source of antigen for vaccine development.Citation37-40 However, the mechanisms involved have yet to be fully defined and no experimental evidence has been provided for direct targeting of CSCs by CSC-DC vaccine-induced anti-CSC immunity. We report herein that the therapeutic efficacy of our CSC-DC vaccine was associated with significantly inhibited metastasis to the lung. A number of studies have suggested that tumor cell metastasis is predicated on the expression levels of chemokine ligands in the target organs, as well as the expression of corresponding chemokine receptors on the malignant tumor cells.Citation28-30, Citation41-46 In our study, a substantial percentage of the subcutaneous primary D5 tumor cells harvested from PBS-treated mice expressed high levels of CCR7 and CCR10 (ranging from 20–40%). CSC-DC vaccination was associated with significantly reduced expression of these 2 receptors to 2–5%. On the other hand, the mRNA levels of CCL21 (ligand for CCR7), CCL27 and CCL28 (ligands for CCR10) were decreased significantly in the lung tissues harvested from the animals subjected to CSC-DC vaccine in the setting of RT. The expression of chemokine or chemokine receptors may lead to immune tolerance or immune escape, which may, in turn, result in tumor progression.Citation45,47,Citation48 Our data suggest that decreased interactions between CCR7/CCL21, CCR10/CCL27 and CCL28 may play an important role in CSC-DC vaccination-induced inhibition of tumor metastasis. We previously reported that blockade of the IL-8 receptor CXCR1 selectively depleted the CSC population.Citation16 The molecular and biochemical signaling pathways in CSC-DC vaccine-induced down-regulation of CCR7/CCL21, CCR10/CCL27 and CCL28 interactions in this study remain to be identified. Nevertheless, our results suggest that blockade of these chemokine/chemokine receptor interactions may result in retarded primary s.c tumor metastasis to the lung and therefore improve survival.
CSCs are found to be responsible for tumor metastasis and progression.Citation49-51 In this study, while we found that RT treatment increased the CSCs in the primary s.c. tumor compared with PBS-treated control, the percentage of ALDHhigh CSCs decreased significantly after RT plus CSC-DC vaccination from control (∼15%) to <3%, which was also significantly less than those after treatment with RT alone (∼20%) or with RT plus ALDHlow DC vaccination (∼12%). These results indicate that CSC-DC vaccine could induce direct targeting of CSCs.
We postulate that the reduction of CSCs shown above is related to CSC-DC vaccine-induced cellular and/or humoral anti-CSC immunity. So far, studies in these regards, while limited, have focused on cellular immunity, e.g., higher production of interferon γ (IFNγ) by T cells,Citation40 or enhanced tumor infiltration of CD8+ and CD4+ T lymphocytes.Citation38,39 In the present study, we evaluated CSC-DC vaccine-induced humoral anti-CSC immunity. We observed specific binding of ALDHhigh CSCs by IgG produced by RT + ALDHhigh CSC-DC vaccine-primed B cells. Importantly, specific binding of IgG produced by RT + ALDHhigh CSC-DC vaccine-primed B cells to ALDHhigh CSCs resulted in significant lysis of these target cells in the presence of complement. These results have provided direct experimental evidence that CSC-primed antibodies selectively target CSCs. Identification of CSC antigens represents a critical respect in CSC-targeted immunotherapy of cancer. Antigen detection, for example by antibody interaction using ELISA or western blotting, warrants further investigation. Together, these illuminating findings may foster the development of novel CSC-targeted immunological approaches for cancer treatment.
Materials and Methods
Mice and ethical statement
Female C57BL/6 (B6) and C3H/HeNCr MTV (C3H) mice were purchased from Jackson and Charles River Laboratories, respectively. All the mice were housed in specific pathogen-free condition at the University of Michigan Animal facilities. The mice used for experiments were at the age of 7∼8 weeks. The University of Michigan Laboratory of Animal Medicine approved all animal protocols.
Tumor cells
D5 is a poorly immunogenic clone of the melanoma cell line B16 syngeneic to B6 mice, and was originally established in our laboratory. Squamous carcinoma cell line SCC7 is syngeneic to C3H mice.Citation22 The cell lines were grown in complete medium consisting of RMPI 1640 supplemented with 10% heat-inactivated fetal bovine serum, 0.05 mM 2-mercaptoethanol, 0.1 mM nonessential amino acids, 1 mM sodium pyruvate, 100 μg/mL streptomycin, 100 U/mL penicillin, 50 μg/mL gentamicin and 0.5 μg/mL fungizone.
ALDEFLUOR assay
The ALDEFLUOR Kit (StemCell Technologies) was used to isolate ALDEFLUOR+/ALDHhigh CSCs and ALDEFLUOR-/ALDHlow non-CSCs from the D5 and SCC7 cells, as previously described.Citation22
Preparation of DC vaccine
To prepare tumor cell lysates, unsorted heterogeneous tumor cells, sorted ALDEFLUOR+/ALDHhigh or ALDEFLUOR-/ALDHlow cells were suspended at a concentration of 1 million cells in 1 mL complete medium. Cells were lysed by 5 rapid freeze-thaw cycles in 37°C water bath and liquid nitrogen. After centrifugation, tumor cell lysates were collected. Bone marrow-derived murine DCs were generated as we described previously.Citation22 Bone marrow cells from the mice were cultured in complete medium supplemented with 10 ng/mL IL-4 and 10 ng/mL GM-CSF at a concentration of 1 × 106 cells/mL. Fresh medium supplemented with GM-CSF and IL-4 was added on days 2 and 4. On day 5, DCs were harvested by gentle pipetting and enriched by Opti-Prep density gradient medium. Lysate of unsorted heterogeneous tumor cells, ALDHlow or ALDHhigh cells was added to DCs at a 1:3 cell equivalent ratio. The DCs were then incubated at 37°C for 24 h with 5% CO2. After incubation, the unsorted heterogeneous tumor cell lysate-pulsed DCs (H-DC), ALDHlow lysate-pulsed DCs (ALDHlow-DC) or ALDHhigh lysate-pulsed DCs (ALDHhigh-DC, e.g., CSC-DC) will be used as vaccine as specified in the subsequent experiments. Each mouse was inoculated with 2 million DCs per vaccine.
Tumor treatment protocols
B6 or C3H mice were inoculated s.c. with 0.05 × 106 D5 cells or 0.5 × 106 SCC7 cells respectively on day 0. The mice were treated with localized radiation therapy (RT) on day 5 and day 6 followed by the 1st DC vaccine on day 7. The combined RT + vaccine treatment was repeated on day 12, 13, 14 and 19, 20, 21 respectively. Thus, the RT was delivered 6 times, which were on days 5, 6, 12, 13, 19 and 20 with a total dose of 51 Gy (8.5 Gy × 6), while vaccines were administrated 3 times, 1 week apart, which were on days 7, 14 and 21. Each experimental group contained 5∼11 mice. Tumor volumes were measured 3 times per week. The long and short diameters of tumor mass were measured and the tumor volume was calculated as: tumor volume= (width2* length)/2. Survival was monitored and recorded as the percentage of surviving mice after tumor inoculation.
Hematoxylin and eosin (H&E) staining for histologic analysis
At the end of the experiments, the lungs were harvested and fixed with 10% formalin, paraffin embedded and stained with H&E to observe the histo-pathological alterations. The slides were observed under the microscope with 100× magnification.
Measurement of chemokine receptor and chemokine levels
Freshly harvested primary subcutaneous tumors were disaggregated into single cell suspensions.Citation22 Tumor cells were incubated with phycoerythrin (PE) conjugated anti-CCR7, anti-CCR10 or isotype control antibodies for 30 min at 4°C. The cells were then re-suspended in 2% formalin for flow cytometry analysis. The mRNA levels of chemokine CCL21, CCL27 or CCL28 in lung tissues were analyzed using real time quantitative PCR (qRT-PCR). The relative mRNA levels of various chemokines and GAPDH (as an internal control) were quantified by SYBR-GREEN master mix (Invitrogen Life Technology, Carlsbad, CA). The relative expression levels of chemokines and GAPDH were detected using the following primers: 5′-CAAGACACCATCCCCACA-3′ (F) and 5′-TGTGACCGCTCAGT-CCTC-3′ for CCL21; 5′-CTGCTGAGGAGGATTGTCCAC-3′ (F) and 5′-CACGACAGCCTGGAGGTGA-3′ for CCL27; 5′-CAGGGCTCACACTCATGGCT-3′ (F) and 5′-CCATGGGAAGTATGGCTTCTG-3′ for CCL28; and 5′-CTCCTCCT-GTTCGA- CAGTCAGC-3′ (F) and 5′- CCCAATACGACCAAATCCGTT- 3′ for GAPDH. The relative expression levels of the chemokines were then normalized to the geometric mean of the internal control gene (GAPDH) by using the comparative Ct method (2-ΔΔCT).
Purification and culture of B cells
Spleens were harvested at the end of the experiments from animals subjected to various treatments. Spleen B cells were purified using CD19 microbeads (Miltenyi Biotec, Bergisch Gladbach, Germany) and activated 5 days in complete medium supplemented with 5 μg/mL LPS, anti-CD40 (FGK45, 1/100 dilution) and IL-2 (60 IU/mL). The culture supernatants were collected and stored at −20°C for future experiments.
CSC binding by immune supernatant
To make the results comparable among different treatments, the concentrations of IgG in the mixed B cell culture supernatants of each treatment group (n = 5) were detected using ELISA before we performed the binding assays. Based on the ELISA results (concentration of IgG), we determined the volume we needed to take from each mixed sample (e.g. each treatment) to ensure that an equal quantity of IgG was taken from each treatment group to assay binding to ALDHhigh cells (or to ALDHlow cells). Sorted ALDHlow or ALDHhigh D5 cells were incubated with the appropriate volume of immune supernatants collected from the cultured B cells comprising an equal quantity of IgG followed by incubation with the FITC-conjugated anti-mouse IgG. The binding of supernatant antibody to ALDHlow vs. ALDHhigh D5 cells was subsequently assessed using flow cytometry.
Antibody and complement mediated cytotoxicity against CSCs
Viable ALDHhigh or ALDHlow D5 cells (105) were incubated with immune supernatants collected from the culture of spleen B cells collected from D5-bearing mice subjected to therapy. The cells were then incubated with rabbit complement for another 1 h. Trypan blue staining was used to assess cell lysis, which was expressed as: % viable cells = the number of viable cells after immune supernatant and complement incubation/105.
Reagents
The ALDEFLUOR kit was purchased from Stem Cell Technology. The antibodies and magnetic beads were purchased from BD. All chemicals were purchased from Sigma-Aldriche. The recombinant cytokines were purchased from PeproTech or R&D Systems.
Statistics
Data were analyzed using GraphPad Prism 6 (GraphPad software). Survival analysis was determined by the log-rank test. Analysis for the presence of lung metastasis was performed using the Fisher exact test. Other data were evaluated by unpaired Student's t-test (2 cohorts) or one-way analysis of variance (ANOVA) (> 2 cohorts). A two-tailed P value <0.05 was considered significant.
Disclosure of Potential Conflicts of Interest
No potential conflicts of interest were disclosed.
Funding
This work was supported by the Elsa U. Pardee Foundation, and partially supported by the Gillson Longenbaugh Foundation. This work was also partially supported by the Science and Technology Program of Guangdong, China (2012A0304000590) and the Science and Technology Program of Guangzhou, China (2013J4500005), and in part by 2011 National Science Fund of China (30971112).
References
- Chang AE, Li Q, Jiang G, Sayre DM, Braun TM, Redman BG. Phase II trial of autologous tumor vaccination, anti-CD3-activated vaccine-primed lymphocytes, and interleukin-2 in stage IV renal cell cancer. J Clin Oncol 2003; 21:884-90; PMID:12610189; http://dx.doi.org/10.1200/JCO.2003.08.023
- Chang AE, Redman BG, Whitfield JR, Nickoloff BJ, Braun TM, Lee PP, Geiger JD, Mulé JJ. A phase I trial of tumor lysate-pulsed dendritic cells in the treatment of advanced cancer. Clin Cancer Res 2002; 8:1021-32; PMID:11948109
- Dougan M, Dranoff G. Immune therapy for cancer. Annu Rev Immunol 2009; 27:83-117; PMID:19007331; http://dx.doi.org/10.1146/annurev.immunol.021908.132544
- Kalbasi A, June CH, Haas N, Vapiwala N. Radiation and immunotherapy: a synergistic combination. J Clin Invest 2013; 123:2756-63; PMID:23863633; http://dx.doi.org/10.1172/JCI69219
- Oldham RK. Biotherapy: the fourth modality of cancer treatment. J cell Physiol Suppl 1986; 4:91-9; PMID:2427531; http://dx.doi.org/10.1002/jcp.1041290416
- Redman BG, Chang AE, Whitfield J, Esper P, Jiang G, Braun T, Roessler B, Mulé JJ. Phase Ib trial assessing autologous, tumor-pulsed dendritic cells as a vaccine administered with or without IL-2 in patients with metastatic melanoma. J Immunother 2008; 31:591-8; PMID:18528294; http://dx.doi.org/10.1097/CJI.0b013e31817fd90b
- Dallas NA, Xia L, Fan F, Gray MJ, Gaur P, van Buren G 2nd, Samuel S, Kim MP, Lim SJ, Ellis LM. Chemoresistant Colorectal Cancer Cells, the Cancer Stem Cell Phenotype, and Increased Sensitivity to Insulin-like Growth Factor-I Receptor Inhibition. Cancer Res 2009; 69:1951-7; PMID:19244128; http://dx.doi.org/10.1158/0008-5472.CAN-08-2023
- Nandi S, Ulasov IV, Tyler MA, Sugihara AQ, Molinero L, Han Y, Zhu ZB, Lesniak MS. Low-dose radiation enhances survivin-mediated virotherapy against malignant glioma stem cells. Cancer Res 2008; 68:5778-84; PMID:18632631; http://dx.doi.org/10.1158/0008-5472.CAN-07-6441
- Medema JP. Cancer stem cells: The challenges ahead. Nat Cell Biol 2013; 15:338-44; PMID:23548926; http://dx.doi.org/10.1038/ncb2717
- Liao T, Kaufmann AM, Qian X, Sangvatanakul V, Chen C, Kube T, et al. Susceptibility to cytotoxic T cell lysis of cancer stem cells derived from cervical and head and neck tumor cell lines. J Cancer Res Clin Oncol 2013; 139:159-70; PMID:23001491; http://dx.doi.org/10.1007/s00432-012-1311-2
- Visus C, Wang Y, Lozano-Leon A, Ferris RL, Silver S, Szczepanski MJ, Brand RE, Ferrone CR, Whiteside TL, Ferrone S. Targeting ALDH(bright) human carcinoma-initiating cells with ALDH1A1-specific CD8(+) T cells. Clin Cancer Res 2011; 17:6174-84; PMID:21856769; http://dx.doi.org/10.1158/1078-0432.CCR-11-1111
- Tallerico R, Todaro M, Di Franco S, Maccalli C, Garofalo C, Sottile R, et al. Human NK cells selective targeting of colon cancer-initiating cells: a role for natural cytotoxicity receptors and MHC class I molecules. J Immunol 2013; 190:2381-90; http://dx.doi.org/10.4049/jimmunol.1201542
- Todaro M, D'Asaro M, Caccamo N, Iovino F, Francipane MG, Meraviglia S, et al. Efficient killing of human colon cancer stem cells by gammadelta T lymphocytes. Jo Immunol 2009; 182:7287-96; http://dx.doi.org/10.4049/jimmunol.0804288
- Bach P, Abel T, Hoffmann C, Gal Z, Braun G, Voelker I, Ball CR, Johnston IC, Lauer UM, Herold-Mende C, et al. Specific elimination of CD133+ tumor cells with targeted oncolytic measles virus. Cancer Res 2013; 73:865-74; PMID:23293278; http://dx.doi.org/10.1158/0008-5472.CAN-12-2221
- Skubitz AP, Taras EP, Boylan KL, Waldron NN, Oh S, Panoskaltsis-Mortari A, Vallera DA. Targeting CD133 in an in vivo ovarian cancer model reduces ovarian cancer progression. Gynecol Oncol 2013; 130:579-87; PMID:23721800; http://dx.doi.org/10.1016/j.ygyno.2013.05.027
- Ginestier C, Liu SL, Diebel ME, Korkaya H, Luo M, Brown M, Wicinski J, Cabaud O, Charafe-Jauffret E, Birnbaum D, et al. CXCR1 blockade selectively targets human breast cancer stem cells in vitro and in xenografts. J Clin Invest 2010; 120:485-97; PMID:20051626; http://dx.doi.org/10.1172/JCI39397
- Almanaa TN, Geusz ME, Jamasbi RJ. A new method for identifying stem-like cells in esophageal cancer cell lines. J Cancer 2013; 4:536-48; PMID:23983818; http://dx.doi.org/10.7150/jca.6477
- Clay MR, Tabor M, Owen JH, Carey TE, Bradford CR, Wolf GT, Wicha MS, Prince ME. Single-Marker Identification of Head and Neck Squamous Cell Carcinoma Cancer Stem Cells with Aldehyde Dehydrogenase. Head Neck 2010; 32:1195-201; http://dx.doi.org/10.1002/hed.21315
- Ginestier C, Hur MH, Charafe-Jauffret E, Monville F, Dutcher J, Brown M, Jacquemier J, Viens P, Kleer CG, Liu S, et al. ALDH1 is a marker of normal and malignant human mammary stem cells and a predictor of poor clinical outcome. Cell Stem Cell 2007; 1:555-67; PMID:18371393; http://dx.doi.org/10.1016/j.stem.2007.08.014
- Januchowski R, Wojtowicz K, Zabel M. The role of aldehyde dehydrogenase (ALDH) in cancer drug resistance. Biomed Pharmacother 2013; 67(7):669-80; http://dx.doi.org/10.1016/j.biopha.2013.04.005
- Le Magnen C, Bubendorf L, Rentsch CA, Mengus C, Gsponer J, Zellweger T, Rieken M, Thalmann GN, Cecchini MG, Germann M, et al. Characterization and clinical relevance of ALDHbright populations in Prostate cancer. Clin Cancer Res 2013; 19:5361-71; PMID:23969936; http://dx.doi.org/10.1158/1078-0432.CCR-12-2857
- Ning N, Pan Q, Zheng F, Teitz-Tennenbaum S, Egenti M, Yet J, Li M, Ginestier C, Wicha MS, Moyer JS, et al. Cancer stem cell vaccination confers significant antitumor immunity. Cancer Res 2012; 72:1853-64; PMID:22473314; http://dx.doi.org/10.1158/0008-5472.CAN-11-1400
- Luo Y, Dallaglio K, Chen Y, Robinson WA, Robinson SE, McCarter MD, Wang J, Gonzalez R, Thompson DC, Norris DA, et al. ALDH1A isozymes are markers of human melanoma stem cells and potential therapeutic targets. Stem Cells 2012; 30:2100-13; PMID:22887839; http://dx.doi.org/10.1002/stem.1193
- de Bree R, Haigentz M, Jr., Silver CE, Paccagnella D, Hamoir M, Hartl DM, Machiels JP, Paleri V, Rinaldo A, Shaha AR, et al. Distant metastases from head and neck squamous cell carcinoma. Part II. Diagnosis. Oral Oncol 2012; 48:780-6; PMID:22520053; http://dx.doi.org/10.1016/j.oraloncology.2012.03.014
- Leung AM, Hari DM, Morton DL. Surgery for distant melanoma metastasis. Cancer J 2012; 18:176-84; PMID:22453019; http://dx.doi.org/10.1097/PPO.0b013e31824bc981
- Schrader AJ. Combined chemoimmunotherapy in metastatic melanoma–is there a need for the double? Anti-cancer drugs 2000; 11:143-8; PMID:10831272; http://dx.doi.org/10.1097/00001813-200003000-00001
- Vokes EE, Weichselbaum RR, Lippman SM, Hong WK. Head and neck cancer. The New England journal of medicine 1993; 328:184-94; PMID:8417385; http://dx.doi.org/10.1056/NEJM199301213280306
- Ben-Baruch A. Organ selectivity in metastasis: regulation by chemokines and their receptors. Clin Exp Metastas 2008; 25:345-56; http://dx.doi.org/10.1007/s10585-007-9097-3
- Muller A, Homey B, Soto H, Ge NF, Catron D, Buchanan ME, McClanahan T, Murphy E, Yuan W, Wagner SN, et al. Involvement of chemokine receptors in breast cancer metastasis. Nature 2001; 410:50-6; PMID:11242036; http://dx.doi.org/10.1038/35065016
- Payne AS, Cornelius LA. The role of chemokines in melanoma tumor growth and metastasis. J Invest Dermatol 2002; 118:915-22; PMID:12060384; http://dx.doi.org/10.1046/j.1523-1747.2002.01725.x
- Li XX, Lewis MT, Huang J, Gutierrez C, Osborne CK, Wu MF, Hilsenbeck SG, Pavlick A, Zhang X, Chamness GC, et al. Intrinsic resistance of tumorigenic breast cancer cells to chemotherapy. J Natl Cancer I 2008; 100:672-9; http://dx.doi.org/10.1093/jnci/djn123
- Bao SD, Wu QL, McLendon RE, Hao YL, Shi Q, Hjelmeland AB, Dewhirst MW, Bigner DD, Rich JN. Glioma stem cells promote radioresistance by preferential activation of the DNA damage response. Nature 2006; 444:756-60; PMID:17051156; http://dx.doi.org/10.1038/nature05236
- Bertrand G, Maalouf M, Boivin A, Battiston-Montagne P, Beuve M, Levy A, Jalade P, Fournier C, Ardail D, Magné N, et al. Targeting head and neck cancer stem cells to overcome resistance to photon and carbon ion radiation. Stem Cell Rev 2013; 10(1):114-26; PMID:23955575; http://dx.doi.org/10.1007/s12015-013-9467-y
- Phillips TM, McBride WH, Pajonk F. The response of CD24(-/low)/CD44(+) breast cancer-initiating cells to radiation. J Natl Cancer I 2006; 98:1777-85; http://dx.doi.org/10.1093/jnci/djj495
- Teitz-Tennenbaum S, Wicha MS, Chang AE, Li Q. Targeting cancer stem cells via dendritic-cell vaccination. Oncoimmunol 2012; 1:1401-3; PMID:23243607; http://dx.doi.org/10.4161/onci.21026
- Korkaya H, Wicha MS. HER2 and breast cancer stem cells: more than meets the eye. Cancer Res 2013; 73:3489-93; PMID:23740771; http://dx.doi.org/10.1158/0008-5472.CAN-13-0260
- Duarte S, Momier D, Baque P, Casanova V, Loubat A, Samson M, Guigonis JM, Staccini P, Saint-Paul MC, De Lima MP, et al. Preventive cancer stem cell-based vaccination reduces liver metastasis development in a rat colon carcinoma syngeneic model. Stem Cells 2013; 31:423-32; PMID:23193035; http://dx.doi.org/10.1002/stem.1292
- Garcia-Hernandez MD, Gray A, Hubby B, Klinger OJ, Kast WM. Prostate stem cell antigen vaccination induces a long-term protective immune response against prostate cancer in the absence of autoimmunity. Cancer Res 2008; 68:861-9; PMID:18245488; http://dx.doi.org/10.1158/0008-5472.CAN-07-0445
- Pellegatta S, Poliani PL, Corno D, Menghi F, Ghielmetti F, Suarez-Merino B, Caldera V, Nava S, Ravanini M, Facchetti F, et al. Neurospheres enriched in cancer stem-like cells are highly effective in eliciting a dendritic cell-mediated immune response against malignant gliomas. Cancer Res 2006; 66:10247-52; PMID:17079441; http://dx.doi.org/10.1158/0008-5472.CAN-06-2048
- Xu QJ, Liu GT, Yuan XP, Xu ML, Wang HQ, Ji JF, Konda B, Black KL, Yu JS. Antigen-Specific T-Cell Response from Dendritic Cell Vaccination Using Cancer Stem-Like Cell-Associated Antigens. Stem Cells 2009; 27:1734-40; PMID:19536809; http://dx.doi.org/10.1002/stem.102
- Ben-Baruch A. Site-specific metastasis formation Chemokines as regulators of tumor cell adhesion, motility and invasion. Cell Adhes Migr 2009; 3:328-33; http://dx.doi.org/10.4161/cam.3.4.9211
- Dobner BC, Riechardt AI, Joussen AM, Englert S, Bechrakis NE. Expression of haematogenous and lymphogenous chemokine receptors and their ligands on uveal melanoma in association with liver metastasis. Acta Ophthalmol 2012; 90:e638-e44; PMID:23164171; http://dx.doi.org/10.1111/j.1755-3768.2012.02515.x
- Murakami T, Cardones AR, Hwang ST. Chemokine receptors and melanoma metastasis. J Dermatol Sci 2004; 36:71-8; PMID:15519136; http://dx.doi.org/10.1016/j.jdermsci.2004.03.002
- Kai H, Kadono T, Kakinuma T, Tomita M, Ohmatsu H, Asano Y, Tada Y, Sugaya M, Sato S. CCR10 and CCL27 are overexpressed in cutaneous squamous cell carcinoma. Pathol Res Pract 2011; 207:43-8; PMID:21144674; http://dx.doi.org/10.1016/j.prp.2010.10.007
- Simonetti O, Goteri G, Lucarini G, Filosa A, Pieramici T, Rubini C, Biagini G, Offidani A. Potential role of CCL27 and CCR10 expression in melanoma progression and immune escape. Eur J Cancer 2006; 42:1181-7; PMID:16644201; http://dx.doi.org/10.1016/j.ejca.2006.01.043
- Wiley HE, Gonzalez EB, Maki W, Wu MT, Hwang ST. Expression of CC chemokine receptor-7 and regional lymph node metastasis of B16 murine melanoma. J Natl Cancer I 2001; 93:1638-43; http://dx.doi.org/10.1093/jnci/93.21.1638
- Murakami T, Cardones AR, Finkelstein SE, Restifo NP, Klaunberg BA, Nestle FO, Castillo SS, Dennis PA, Hwang ST. Immune evasion by murine melanoma mediated through CC chemokine receptor-10. J Exp Med 2003; 198:1337-47; PMID:14581607; http://dx.doi.org/10.1084/jem.20030593
- Pivarcsi A, Muller A, Hippe A, Rieker J, van Lierop A, Steinhoff M, Seeliger S, Kubitza R, Pippirs U, Meller S, et al. Tumor immune escape by the loss of homeostatic chemokine expression. Proc Natl Acad Sci USA 2007; 104:19055-60; http://dx.doi.org/10.1073/pnas.0705673104
- Chen K, Huang YH, Chen JL. Understanding and targeting cancer stem cells: therapeutic implications and challenges. Acta Pharmacol Sin 2013; 34:732-40; PMID:23685952; http://dx.doi.org/10.1038/aps.2013.27
- Enderling H, Hlatky L, Hahnfeldt P. Cancer Stem Cells: A Minor Cancer Subpopulation that Redefines Global Cancer Features. Front Oncol 2013; 3:00076
- Schatton T, Frank MH. Cancer stem cells and human malignant melanoma. Pigm Cell Melanoma R 2008; 21:39-55; http://dx.doi.org/10.1111/j.1755-148X.2007.00427.x