Abstract
The objective of the present work was to evaluate the impact of the phenotype of both mononuclear inflammatory cells (MICs) and cancer-associated fibroblast (CAFs) in early breast cancer patients, specifically assessed as to their expression of MMP/TIMP relative to their position within the tumor (i.e., localization at the tumor center or invasive front) and the occurrence of distant metastases.. An immunohistochemical study was performed using tissue arrays and specific antibodies against matrix metalloproteinase (MMP)−1, −2, −7, −9, −11, −13 and −14, tissue inhibitors of metalloproteinase (TIMP)−1, −2 and −3, both at tumor center and at invasive front, in 107 patients with primary ductal invasive breast tumors. Data were analyzed by unsupervised hierarchical clustering analysis. Our results indicated that MMP-11 expression by MICs, and TIMP-2 expression by CAFs at either the tumor center or the invasive front, were the most potent independent prognostic factors for predicting the clinical outcome of patients. Using the unsupervised hierarchical clustering analysis, we found well-defined clusters of cases identifying subgroups of tumors showing a high molecular profile of MMPs/TIMPs expression by stromal cells (CAFs and MICs), both at the tumor center and at the invasive front, which were strongly associated with a higher prevalence of distant metastasis. In addition, we found combinations of these clusters defining subpopulations of breast carcinomas differing widely in their clinical outcome. The results presented here identify biologic markers useful to categorize patients into different subgroups based on their tumor stroma, which may contribute to improved understanding of the prognosis of breast cancer patients.
Abbreviations:
- bFGF, fibroblast growth factor
- CAF, cancer-associated fibroblast
- CI, confidence interval
- ECM, extracellular matrix
- EGF, epidermal growth factor
- EMT, epithelial-mesenchymal transition
- EGF, epidermal growth factor
- ER, estrogen receptor
- HER2, human epidermal growth factor receptor 2
- HGF, hepatocyte growth factor
- IGFs, insulin growth factors
- IGFBPs, IGF binding protein
- IL, interleukin
- MIC, mononuclear inflammatory cell
- MMP, matrix metalloprotease
- NFkB, nuclear factor kappa B
- PgR, progesterone receptor, TA, tissue array
- TGFß, transforming growth factor ß
- TIMP, tissue inhibitors of metalloproteases
Introduction
Breast cancer is the most common malignancy in women. Despite early diagnosis, surgery, and adjuvant therapy, a considerable number of patients experience recurrence with metastatic disease. Recent advances in whole-genome technologies have generated an overwhelming amount of molecular data regarding mammary carcinoma.Citation1 However, in spite of all of these data, breast cancer is currently treated on the basis of the status of only 3 clinical markers of cancer cells: estrogen receptor (ER), progesterone receptor (PgR), and human epidermal growth factor receptor 2 (HER2). This fact reflects the complexity and heterogeneity of breast cancer biology and is the principal reason for which a personalized treatment approach remains distant. Thus, we consider the need for finding a new approach to elucidate the mechanisms intimately involved in breast cancer progression.
Tumor initiation is typically conceptualized as the accumulation of genetic and epigenetic mutations in the epithelium that results in the recruitment of reactive stroma. Similar to other solid tumors, breast carcinomas are composed by 2 distinct compartments: the parenchyma and the stroma. There are several lines of evidence indicating that progression of tumors toward a malignant phenotype does not depend exclusively on the cell-autonomous properties of cancer cells themselves but is also deeply influenced by tumor stroma reactivity.Citation2 Two well-studied cellular component of the tumor stroma are mononuclear inflammatory cells (MICs) and cancer-associated fibroblasts (CAFs), both of which may be present within the invasive front or infiltrate the tumor center. In this context, we have found that different subpopulations of MICs and CAFs –characterized on the basis of expression of matrix metalloproteases (MMPs) and tissue inhibitors of metalloproteases (TIMPs)– exist both at the tumor center and invasive front., Citation3-6 These distinct stromal subsets display unique prognostic significance, such that breast carcinomas containing either MICs or CAFs cells with a molecular profile of high MMPs/TIMPs expression had a high rate of development of distant metastases as compared with those tumors with a low expression profile. These are relevant findings considering that MMPs are governed by the tumor stroma and exert powerful influences on the local tissue microenvironment during tumorigenesis and progression. These enzymes are able to impact tumor cell behavior in vivo by several means: i) direct degradation of the stromal connective tissue and basement membrane components, favoring invasion and metastasis of cancer cellsCitation7; ii) cleavage of membrane-bound growth factors or cytokines as well as their receptorsCitation8-10; iii) cleavage of pro-apoptotic factors and induction of a more aggressive phenotype via generation of apoptotic resistant cellsCitation11; iv) regulation of tumor angiogenesis, both positively through the ability of MMPs to mobilize or activate pro-angiogenic factors,Citation12 or negatively via generation of angiogenesis inhibitors, such as angiostatin, endostatin and tumstatin, cleaved from large protein precursors Citation13; or v) cleavage of cell adhesion molecules, such as cadherins, leading to an increased cell motility occurring in epithelial mesenchymal transition (EMT).Citation14-15 On the other hand, the activity of MMPs is specifically inhibited by TIMPs, but it is now assumed that TIMPs are multifactorial proteins also involved in the induction of proliferation and the inhibition of apoptosis.Citation16-17
The objective of the present work is to evaluate the impact of total MICs and CAFs in a population of early breast cancer patients by phenotypic characterization and assessment of their MMPs/TIMPs molecular profile at the tumor center and invasive front in relation to the occurrence of distant metastases. A total of 10 MMPs and TIMPs were analyzed using immunohistochemistry and tissue array (TA) techniques, and the data were analyzed by unsupervised hierarchical cluster analysis by each cellular type and by each tumor location. We found clusters of stromal cell phenotypes in various combinations capable of defining subpopulations of breast carcinomas differing widely in their clinical outcome.
Results
In order to characterize to expression of tumor-associated metalloproteases and their inhibitors, we performed more than 8,000 determinations in arrays of cancer specimens from 107 patients (10 protein expressions in 107 tumors, 2 areas by tumor, 2 cores by tumor area, and 2 fields by core) with primary invasive ductal carcinoma of the breast.
The majority of MMPs and TIMPs were mainly expressed by cancer cells, both at the tumor center (MMP-1: 86.0% of tumors; MMP-2: 32.7%; MMP-7: 84.1%; MMP-9: 77.6%; MMP-11: 86.9%; MMP-13: 77.6%; MMP-14: 91.6%; TIMP-1: 94.4%; TIMP-2: 84.1%; TIMP-3: 86.9%) and at the invasive front (MMP-1: 96.3% of the tumors; MMP-2: 39.3%; MMP-7: 83.7%; MMP-9: 95.2%; MMP-11: 97.1%; MMP-13: 76.7%; MMP-14: 90.2%; TIMP-1: 96.2%; TIMP-2: 93.1%; TIMP-3: 58.7%) in breast carcinomas. However, these proteins were also expressed by stromal cells in a significant percentage of tumors.
shows representative examples of CAFs and MICs expressing MMPs and TIMPs localized to the tumor center and the invasive front in breast carcinomas. Immunostaining for these proteins revealed a cytoplasmic location in cancer cells and tumor-associated stromal cells, including both CAFs and MICs. In neoplasms positive for CAFs and MICs expressing either MMPs or TIMPs, at least 70% of these cells showed a positive immunostaining of each evaluated field.
Figure 1. Human mammary carcinomas contain tumor stromal cells expressing metalloproteases and their inhibitors. Representative pictures of mammary cancer patient tissue array immunostaining for the different matrix metalloproteases (MMPs) and tissue inhibitors of metalloproteases (TIMPs) analyzed in breast cancer patients (200X), both at tumor center and at invasive front. (A) MMP-1, (B) MMP-2, (C) MMP-7, (D) MMP-9, (E) MMP-11, (F) MMP-13, (G) MMP-14, (H) TIMP-1, (I) TIMP-2 and (J) TIMP-3.
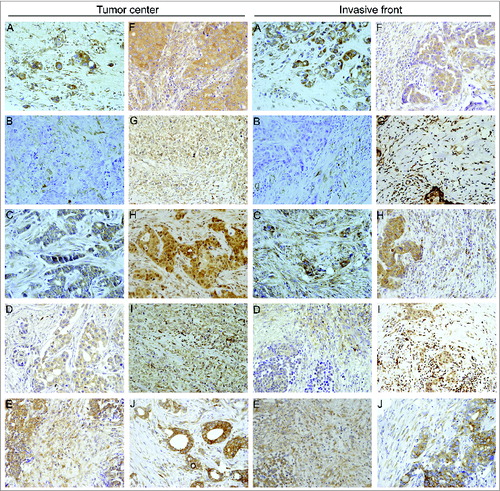
To confirm the expression of these proteins by each stromal cell type, we performed double-immunostaining in the tissue sections using antibodies specific for MMPs/TIMPs and specific markers (CD45 and α smooth muscle actin [α-SMA], respectively) to identify MICs or CAFs in the tumor samples ().
Figure 2. Human mammary carcinomas contain CAFs and MICs expressing metalloproteases and their inhibitors. Representative pictures of double-immunostaining of mammary cancer patient tissue arrays to confirm expression of matrix metalloproteases (MMPs) and tissue inhibitors of metalloproteases (TIMPs) by each type of stromal cell. (A) Positive MMP-11 staining (brown) in mononuclear inflammatory cells (MICs) identified by the CD45 marker (pink) (200X) and (B) Positive TIMP-2 staining (brown) in cancer-associated fibroblasts (CAFs) identified by the α-SMA marker (pink) (200X).
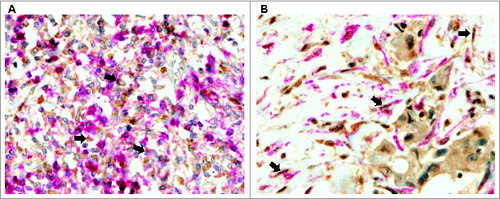
Considering the clinical importance of the stromal phenotypes according to the expression of MMPs and TIMPs, and since there is a practical interest in providing easy prognostic information for clinical use, we prospectively analyzed the possible prognostic value of all of these stromal biological markers. We found that stromal expression of several MMPs and TIMPs, at various tumor locations were associated with prognosis (). Of these, MMP-11 expression by MICs and TIMP-2 expression by CAFs (either at the tumor center or at the invasive front) were the most potent independent prognostic factors for predicting the clinical outcome. As shown in , the expression of these proteins was related to a higher probability of recurrence in the form of distant metastases.
Figure 3. Prognostic significance of stromal expression of metalloproteases and their inhibitors in mammary carcinoma patients. Kaplan–Meier survival curves for relapse-free survival as a function of matrix metalloprotease 11 (MMP-11) expression by mononuclear inflammatory cells (MICs) at the tumor center (A) or at the invasive front (B), or as a function of tissue inhibitors of metalloprotease 2 (TIMP-2) expression by cancer-associated fibroblasts (CAFs) located at the tumor center (C) or at the invasive front (D).
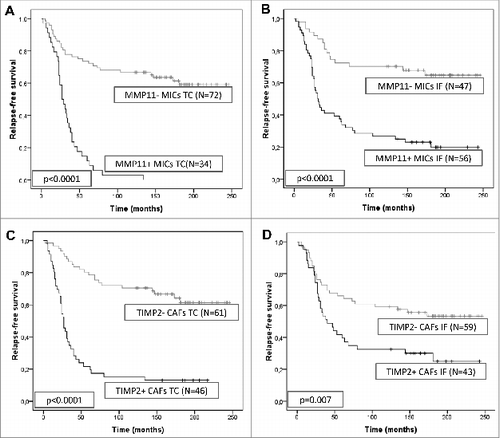
Table 1. Basal demographics and clinical characteristics of 107 patients with invasive ductal carcinoma of the breast included in our study.
To identify specific groups of tumors with distinct MMP/TIMP immunohistochemical expression profiles, the obtained data were subsequently evaluated by unsupervised hierarchical clustering analysis for each cell type. This algorithm places proteins on the horizontal axis and samples on the vertical axis based on similarity of their expression profile, and if appropriate, generates dendrograms with well-defined clusters of cases for each cellular type (). In the case of CAFs, the dendrogram shows a first-order division of the tumors into 2 distinct MMP/TIMP molecular profiles, both at the tumor center (designated group F1A (n = 64) and group F1B (n = 43) and at the invasive front (designated group F2A (n = 51) and group F2B (n = 56) ( and 4B, respectively). Among MICs, we also found a first-order division of tumors into 2 distinct MMP/TIMP molecular profiles at the tumor center (designated group M1A (n = 59) and group M1B (n = 48) (). However, we identified 3 distinct groups for MICs at the invasive front with high, intermediate, and low MMP/TIMP profiles (designated group M2A = 23), group M2B = 57); and group M2C (n = 27), respectively) (). Nevertheless, this method did not produce a dendrogram with well-defined clusters of cases according to MMP/TIMP profiles among cancer cells, either at the tumor center or at the invasive front (Figure S1).
Figure 4. Clustering analysis of human mammary carcinoma patient expression of metalloproteases and their inhibitors. Hierarchical clustering analysis of global expression patterns of matrix metalloproteases (MMPs) and tissue inhibitors of metalloproteases (TIMPs) among different cells types present in breast tumor specimens (n=107) as measured by immunohistochemistry on tissue microarrays from the tumor center and the invasive front. Graphical representation of hierarchical clustering results in cancer-associated fibroblasts (A, B) and mononuclear inflammatory cells (C, D). Rows: tumor samples; columns: MMPs/TIMPs. Protein expressions are depicted according to a color scale: red, positive staining; green, negative staining; gray, missing data. Two major clusters of tumors (F1A and F1B) were shown in fibroblast-like cells both in the tumor center (A), and (F2A and F2B) in the invasive front (B). For mononuclear inflammatory cells (MICs), we found 2 major cluster of tumors (M1A and M1B) in the tumor center (C), whereas 3 distinct clusters of tumors (M2A, M2B and M2C) were found at the invasive front (D).
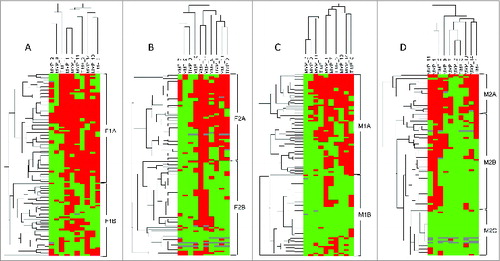
shows MMPs and TIMPs expressions in the different clusters of breast carcinomas with distinct MMP/TIMP molecular profiles in CAFs and in MICs located at either the tumor center or the invasive front. As shown in this table, MMP−1, −11, −13, −14 and TIMP−1 and −2, were identified as the most frequently expressed factors in the groups of tumors with high MMPs/TIMPs molecular profiles relative to those with low expression profiles.
Table 2. Expression of MMPs and TIMPs in 3 different cluster groups resulting from the combination of stromal cells in the tumor center and in the invasive front. Data are expressed as number of positive cases (%).
We also studied the possible relationship between these cluster subgroups and patient outcome, and found that the cluster subgroups with high MMP/TIMP molecular profiles, either CAFs or MICs, located at both the tumor center and the invasive front, were associated with both poor relapse-free and overall survival (). Also, we further investigated the possible impact of the different combination of these molecular MMP/TIMP profiles on prognosis. We found that among our patient data, there was a subgroup of tumors (n = 23) showing the highest molecular profile of MMPs/TIMPs expression by stromal cells (both CAFs and MICs) in both tumor locations, which were strongly associated with recurrence in the form of distant metastases (P < 0.001;). On the contrary, tumors displaying the lowest MMPs/TIMPs profile (n = 11) had an excellent clinical outcome, whereas the third subgroup (n = 73) showed an intermediate molecular profile and an intermediate clinical outcome ().
Figure 5. Survival analysis of human mammary carcinoma patients stratified according to expression of metalloproteases and their inhibitors. Kaplan–Meier survival curves (relapse-free survival and overall survival) as a function of the 2 major clusters of tumors (high and low profile of matrix metalloproteases (MMPs) and tissue inhibitors of metalloproteases (TIMPs) expression) in fibroblast-like cells at the tumor center (A) and at the invasive front (B), or in mononuclear inflammatory cells (MICs) at the tumor center (C) or at the invasive front (D). Survival curves as a function of the combination of the different cluster subgroups both in fibroblast cells and MICs, in tumor center and at the invasive front (E).
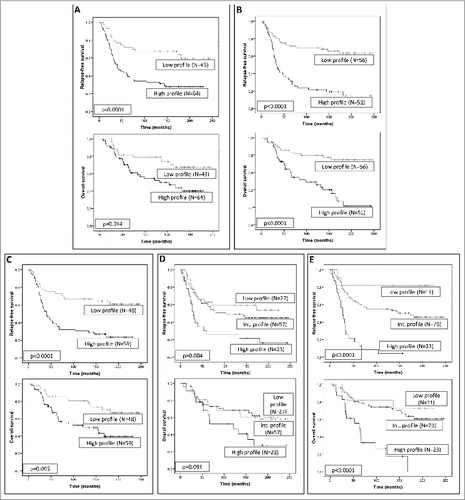
Regarding the possible biological relevance of these combinations of cluster subgroups (low, intermediate and high molecular profile of MMP/TIMP expression in CAFs and MICs, at the tumor center and the invasive front), we investigated their possible relation with the clinico-pathological characteristics from patients and tumors. Our data demonstrated no significant associations between patients or tumors characteristics and MMPs/TIMPs molecular profile ().
Cox's multivariate analysis demonstrated that histological grade (grade II: (relative risk (RR) (confidence interval (CI)) = 1.6 (0.8–3.0); Grade III: 3.8 (1.9–7.6) was the only clinical characteristic significantly and independently associated with distant relapse-free survival. Moreover, the combination of cluster groups of stromal cells at the tumor center and at the invasive front (intermediate expression profile: 2.9 (0.7–12.1); high expression profile: 10.5 (2.4–46)) was the most potent predictive factor significantly and independently associated with distant relapse-free survival.
Discussion
Here, we have found that tumor stroma phenotype varies in concordance with the expression of certain MMPs and TIMPs by CAFs and MICs, both the in tumor center and in the invasive front. These findings led us to identify different subgroups of tumors differing widely in their stromal phenotype and in regards to patient prognosis. There was a subgroup of tumors showing a stromal molecular profile of abundant MMP/TIMP expression in each one of these tumor locations, which was strongly associated with higher recurrence of distant metastases. By contrary, tumors with stromal phenotypes displaying low molecular profiles had an excellent clinical outcome. Thus, these findings suggest a diabolic link between stromal cell phenotypes and disease recurrence, with stromal cells appearing along the tumor locations that ultimately may promote the escape of cancer cells from the primary tumor and favor metastatic spread in breast cancer patients. Although cancer cells also express high levels of MMPs and TIMPs, it seems that the stromal expression of these proteins is particularly crucial to tumor progression. Indeed, mammary cancer cells expressed proteins involved in extracellular matrix degradation, however the response of the stroma appears to depend on their crosstalk with cancer cells, and this reciprocal communication is what opens the door to tumor cell invasion and the development of metastatic disease.
Our results are in agreement with prior studies reporting that stromal characteristics may dictate tumor outcome in many cases of breast cancer.Citation18-23 Normal fibroblasts are the most abundant cell type in the connective tissue and are responsible for the synthesis and turnover of the extracellular matrix. However, CAFs are different from normal fibroblasts, including a more rapid proliferation rate, as well as several actions promoting tumor phenotypes such as survival, proliferation, general metabolic reprogramming, angiogenic shift, ECM remodeling, EMT activation, the acquisition of stem cell traits, metabolic reprogramming toward a reverse Warburg phenotype, or inflammatory cell recruitment.Citation24-27 All of these actions are due to the fact that CAFs produce a repertoire of growth factors and cytokines that influence the behavior of the epithelium, such as hepatocyte growth factor (HGF), epidermal growth factor (EGF), insulin growth factors (IGFs), IGF binding protein (IGFBPs), fibroblast growth factor (bFGF) or transforming growth factor β (TGFβ).Citation2,28,29 In addition, there are data indicating that CAFs contribute to both chemotherapy and endocrine resistance.Citation29,30 It is also known that CAFs are capable of evoking a proinflammatory responseCitation31. After activation, CAFs initiate a pro-inflammatory response including the secretion/expression of interleukin (IL)-1β, IL-6, IL-8, the chemokine SDF-1/CXCL12, and nuclear factor kappa (NFκB), which may induce inflammation by recruiting components of the immune system.Citation32,33 Thus, CAFs may orchestrate a distorted architecture of the host tissue and a functional “corrupted” stroma which in turn helps the metastatic spread.Citation34 Nevertheless, these CAFs are heterogeneous populations and their relative composition greatly differs among tumors. The origin of CAFs is matter of debate and many origins have been proposed, such as resident normal fibroblasts, derivation from mesenchymal stem cells or transdifferentiation of epithelial and endothelial cells.Citation35–36 Possibly due to divergent origins, there is marked heterogeneity in marker expression among CAFs subpopulations, or in CAFs originating from different tumors. We consider that our results identify biological markers of CAFs, which confer tumor aggressiveness and may help define functionally distinct fibroblast-like cell populations in breast carcinomas.
Immune cell infiltration within a solid tumor is a naturally occurring event, which can sometimes lead to blocking of cancer progression and thus limit or even prevent the generation of metastasis.Citation37 In general, this occurs in early stages of tumor progression as a result of host immunosurveillance. However, unfortunately in many cases, the host is unable to generate sufficient antitumor immune responses due to the immunosuppressive abilities of tumors that circumvent or dampen immunity, thus leading to tumor escape from host immunosurveillance. Additionally, it remains a distinct possibility that MICs favor tumor progression. Accumulating evidences indicate that diverse immune cells exert pro-tumor functions, as they secrete cytokines, growth factors, chemokines and proteases that stimulate angiogenesis as well as proliferation, migration and invasive potential of cancer cells.Citation38–40 In this sense, the data from our present study, similarly to our previous reports, indicate the existence of a phenotype of MICs showing a high molecular profile of MMPs/TIMPs in the tumor center that is associated with a high metastatic rateCitation3, 5, Citation41. These results suggest that leukocytes from peripheral blood undergo phenotypic modification to infiltrate tumors from the invasive front to the tumor center. This seems to be a dynamic process in which inflammatory cells and immunomodulatory mediators present in the tumor microenvironment polarize host immune response toward specific phenotypes impacting on tumor progression.
Our results demonstrate the biological heterogeneity among tumors with regard to the molecular profile of MMPs/TIMPs expression by stromal cells, both at the tumor center and at the invasive front. In order to improve clinical prognosis on the basis of stromal functionally, we investigated the outcome impact of expression of each MMPs/TIMPs by each key stromal cellular type and in each tumor location. Thus, we identified several biological markers with strong statistical impact on both relapse-free and overall survival, such as MIC expression of MMP-11 and CAF expression of TIMP-2 at either the tumor center or at the invasive front. The finding that MICs express relatively abundant MMP-11 (stromalysin-3) is a strong indicator of metastatic rate, in accordance with our previous reports,Citation3 an expression previously reported to be associated with a high inflammatory molecular profile (highest intratumoral levels of IL-1, IL-5, IL-6, IL-17, IFNβ and NFκB) in breast carcinomas.Citation42
An interesting question is how both CAFs and MICs maintain their defined functional state over a prolonged period of time. At least, 3 distinct mechanisms are possible: (i) genetic mutations, such as reported in studies describing p53 and PTEN mutations in the stromal compartment of breast cancer patients,Citation43-44 although other studies indicate that the stromal population is genetically stable in breast cancerCitation45; (ii) epigenetic alterations, or (iii) persistent environmental effects due to the presence of signals originating from cancer cells. Therefore, we consider that our findings show different functional stages in the tumor-associated stromal cellular components that influence disease outcome andmay be an excellent platform to assess the nature of these signals. The unsolved question is, if the tumor stromal cells merely respond to signals from the carcinoma cells or respond to autocrine signals to modulate tumor progression. Regardless, in the present study, we identify phenotypes of the more frequent stromal cells broadly influencing tumor prognosis. Therefore, this finding is of great clinical interest and also suggests that these populations of host stromal cells could be possible targets for inhibition of tumor progression and metastasis. This is especially relevant if we consider that the stromal compartment surrounding tumors is generally considered as genetically stable compared to the rapidly dividing carcinoma cells. In addition, there are many pieces of evidence indicating that the tumor microenvironment is fertile ground for the development of novel therapies with the potential to augment existing treatment and prevention options. In fact, some new potential drugs targeting stromal cells, either CAFs or tumor-associated macrophages occurring in breast cancer, have already been developed and are under investigation in pre-clinical and clinical trials.Citation29, 46
It is known that metastasis, which is the leading cause of death in patients with solid tumors, is a highly inefficient process, and it is estimated that less than 1% of disseminated tumor cells are able to form viable metastases. The “seed and soil” hypothesis postulates that an appropriate host microenvironment (the soil) is needed for the optimal growth of tumor cells (the seed).Citation47 The results presented herein contribute to this concept, identifying biological markers to categorize patients into different subtypes of tumor stroma with the power to predict prognosis and therapeutic response.
Materials and Methods
Patient selection
This study comprised 107 women with a histologically confirmed diagnosis of early invasive breast cancer of ductal-type treated between 1990 and 2001, some of which were previously included in our preliminary studies on the expression of MMPs and TIMPs in breast cancer.Citation41,48,49 We selected women with the following inclusion criteria: invasive ductal carcinoma, at least 6 histopathologically-assessed axillary lymph nodes, and a minimum of 10 years of follow-up in those women without tumor recurrence. The exclusion criteria were the following: metastatic disease at presentation, prior history of any type of malignant tumor, bilateral breast cancer at presentation, having received any type of neoadjuvant therapy, development of loco-regional recurrence during the follow-up period, development of a second primary cancer, and absence of sufficient tissue in the paraffin blocks used for manufacturing the tissue arrays.Citation33 We randomly selected a sample size of 107 patients, in accordance to 4 different groups of similar size and stratified with regard to nodal status and the development of metastatic disease, which were key variables in our study. Thus, we include a sufficient number of both node-negative and node-positive patients to comprise each subgroup among patients without and with disease recurrence for securing the statistical power of the survival analysis. Note that approximately half of the cases with distant metastasis during the follow-up period occurred in each of the node-negative and node-positive subgroups. Patients’ characteristics included in the 2 main groups, with or without distant metastases (recurrence), are listed in . Patients underwent either modified radical mastectomy or wide resection with axillary lymphadenectomy. Postoperative radiotherapy was given to 41 patients (38.3%). Data about the criteria for systemic adjuvant therapy of the patients were described elsewhere.Citation48 Overall, 42 patients received chemotherapy, 32 patients received tamoxifen, and 16 patients received both types of systemic therapy.
The median follow-up period in patients without metastasis was of 187 months, and 52 months in patients with metastatic disease. The study adhered to national regulations and was approved by our Institution's Ethics and Investigation Committee.
Tissue arrays
Routinely fixed (overnight in 10% buffered formalin), paraffin embedded tumor samples stored in our pathology laboratory files were used in this study. TAs blocks were obtained by punching a tissue cylinder (core) with a diameter of 1.5 mm through a histologically representative area of each ‘donor’ tumor block, which was then inserted into an empty ‘recipient’ tissue array paraffin block using a manual tissue arrayer (Beecker Instruments, Sun Praerie, Winconsin, USA) as described elsewhere.Citation48 A total of 4 cores were employed for each case. Two of these cores in each case corresponding to tumor center area, and another 2 cores corresponding to invasive front. This method, with 2 cores (double redundancy) of each tumor area has been shown to correlate well with conventional immunohistochemical staining.Citation48 The invasive front was defined as the area within 2 mm surrounding the tumors and which contained cancerous cells. From the 107 tumor samples available, 8 tissue array blocks were prepared, each containing 32 tumor samples maximum.
Immunohistochemistry
Immunohistochemistry was carried out on TA sections 5 μm thick, fixed in 10% buffered formalin and embedded in paraffin using a TechMate TM50 autostainer (Dako, Glostrup, Denmark). Antibodies for MMPs and TIMPs were obtained from Neomarker (Lab Vision Corporation, Fremont, CA, USA). The dilution for each antibody was established based on negative and positive controls (1:50 for MMP−2, −7, −13 and −14; 1:100 for MMP-9, TIMP−1, −2 and −3; 1:200 for MMP-1; and 1:400 for MMP-11). To enhance antigen retrieval, tissue sections were treated in a PT-Link (Dako) at 97≡C for 20 min, in citrate buffer (pH 6.1) for MMP-1, −14, TIMP−1 and −3, or in ethylene diamine tetraacetic acid (EDTA) buffer (pH 9) for MMP-13 and TIMP-2. Antibodies against MMP−2, −7, −9 and −11 do not require antigen retrieval. The negative control was DakoCytomation mouse serum diluted at the same concentration as the primary antibody used. All dilutions were made in Antibody Diluent, (Dako, Glostrup, Denmark) and incubated for 30 min to 2 h at room temperature. Breast tumor samples in which we confirmed the presence of the evaluated proteins by Western blot analysis, were used as positive controls, as described previously.Citation3,5 Endogenous peroxidase activity was blocked by incubating the slides in peroxidase-blocking solution (Dako) for 5 min. The EnVision Detection Kit (Dako) was used as the staining detection system. Sections were counterstained with hematoxylin, dehydrated with ethanol, and permanently coverslipped.
Double-immunostaining methods were performed with the BenchMark ULTRA Staining System (Ventana-Roche, Rotkreuz, Switzerland). Paraffin-embedded tissue sections were pre-treated at 95°C for 52 min in buffer (pH 8), and incubated with the following primary antibodies: MMP-11 (1:1000 at 40°C for 80 min) and the CD45 marker (ready-to-use (Roche), 36 min) specific for MICs, or TIMP-2 (1:100 at 40°C for 60 min) and the α-SMA marker (ready-to-use (Roche), 40 min) specific for fibroblast. For each antibody preparation studied, the location of immunoreactivity in each cell type was determined. In each case, immunoreactivity was classified into 2 categories depending upon the percentage of cells stained (negative: 0–10% positive cells; positive: >10% positive cells) in each cell type (cancer cells, CAFs and MICs). We studied both cores that were carried out for each patient and averaged the results. In the event that no tumor was present in a particular core, then the results of the other core analyzed was given. Two certified pathologists (LOG and NB) blinded to the clinical outcome of the patients performed the histological examination. We distinguished stromal cells from cancer cells on the basis of cell size (the latter cells are larger in size). Stromal cell subsets were distinguished primarily by morphology(CAFs are spindle shaped cells whereas MICs are round cells). Additionally, whereas cancer cells are arranged forming either acinar or trabecullar patterns, stromal cells are scattered throughout the tissue.
Staining for ERs and PgRs was scored according to the method described by Allred et al.Citation50 and HER-2 staining according to the criteria used for the Herceptest. Controls included breast cancer tissue with known immunoreactivity for each antibody. Ki-67 and p53 were scored according to the percentages of stained cells. A case was considered positive if at least 15% or 25% of cells were stained, respectively. In addition, we established the following subtypes: luminal type A (ER+, PgR+, HER2-), luminal type B (ER+, PgR+, HER2+), HER2+ (HER2+, ER-, PgR-), and basal-like (ER-, PgR-, HER2-)51.
Data analysis and statistical methods
The significance of observed differences in percentages were calculated using the χ2 test. For analysis of metastasis-free survival and overall survival analysis, we employed Cox's univariate method. Cox's regression model was used to examine the interactions of different prognostic factors in a multivariate analysis. In the multivariate analysis only the parameters that achieved statistical significance for distant relapse-free survival in the univariate analysis (as well as the type of systemic therapy) were included. Expression profiles were analyzed by unsupervised hierarchical clustering, a method that organizes the patterns of protein expression in a tree structure based on similarity. Data were reformatted as follows: −3 designated negative staining, 3 indicated positive staining, and missing data were left blank. We used the Cluster 3.0 program (average linkage, Pearson's correlation). Results were displayed with the Treeview program. The PASW 18.0 software was used for all calculations.
Disclosure of Potential Conflicts of Interest
No potential conflicts of interest were disclosed.
992222_Supplementary_Materials.zip
Download Zip (157.4 KB)Acknowledgments
We want to thank Begoña Álvarez for technical assistance.
Funding
This study was supported by a grant from Fondo de Investigación Sanitaria del Instituto de Salud Carlos III (FIS PI13/02745).
References
- Balko JM, Stricker TP, Arteaga CL. The genomic map of breast cancer: which roads lead to better targeted therapies? Breast Cancer Res 2013; 15:209; PMID:23905624; http://dx.doi.org/10.1186/bcr3435
- Marsh T, Pietras K, McAllister SS. Fibroblasts as architects of cancer pathogenesis. Biochim Biophys Acta 2013; 1832:1070-8; PMID:23123598; http://dx.doi.org/10.1016/j.bbadis.2012.10.013
- Gonzalez LO, Pidal I, Junquera S, Corte MD, Vazquez J, Rodriguez JC, Lamelas ML, Merino AM, Garcia-Muniz JL, Vizoso FJ. Overexpression of matrix metalloproteinases and their inhibitors in mononuclear inflammatory cells in breast cancer correlates with metastasis-relapse. Br J Cancer 2007; 97:957-63; PMID:17848954; http://dx.doi.org/10.1038/sj.bjc.6603935
- Ben-Batalla I, Seoane S, Garcia-Caballero T, Gallego R, Macia M, Gonzalez LO, Vizoso F, Perez-Fernandez R. Deregulation of the Pit-1 transcription factor in human breast cancer cells promotes tumor growth and metastasis. J Clin Invest 2010; 120:4289-302; PMID:21060149; http://dx.doi.org/10.1172/JCI42015
- Gonzalez LO, Corte MD, Junquera S, Gonzalez-Fernandez R, del Casar JM, Garcia C, Andicoechea A, Vazquez J, Perez-Fernandez R, Vizoso FJ. Expression and prognostic significance of metalloproteases and their inhibitors in luminal A and basal-like phenotypes of breast carcinoma. Hum Pathol 2009; 40:1224-33; PMID:19439346; http://dx.doi.org/10.1016/j.humpath.2008.12.022
- Del Casar JM, Gonzalez-Reyes S, Gonzalez LO, Gonzalez JM, Junquera S, Bongera M, Garcia MF, Andicoechea A, Serra C, Vizoso FJ. Expression of metalloproteases and their inhibitors in different histological types of breast cancer. J Cancer Res Clin Oncol 2010; 136:811-9; PMID:19916023; http://dx.doi.org/10.1007/s00432-009-0721-2
- Nelson AR, Fingleton B, Rothenberg ML, Matrisian LM. Matrix metalloproteinases: biologic activity and clinical implications. J Clin Oncol 2000; 18:1135-49; PMID:10694567
- Noe V, Fingleton B, Jacobs K, Crawford HC, Vermeulen S, Steelant W, Bruyneel E, Matrisian LM, Mareel M. Release of an invasion promoter E-cadherin fragment by matrilysin and stromelysin-1. J Cell Sci 2001; 114:111-8; PMID:11112695
- Sternlicht MD, Werb Z. How matrix metalloproteinases regulate cell behavior. Annu Rev Cell Dev Biol 2001; 17:463-516; PMID:11687497; http://dx.doi.org/10.1146/annurev.cellbio.17.1.463
- Egeblad M, Werb Z. New functions for the matrix metalloproteinases in cancer progression. Nat Rev Cancer 2002; 2:161-74; PMID:11990853; http://dx.doi.org/10.1038/nrc745
- Fingleton B, Vargo-Gogola T, Crawford HC, Matrisian LM. Matrilysin ; MMP-7 expression selects for cells with reduced sensitivity to apoptosis. Neoplasia 2001; 3:459-68; PMID:11774028; http://dx.doi.org/10.1038/sj.neo.7900190
- Stetler-Stevenson WG. Matrix metalloproteinases in angiogenesis: a moving target for therapeutic intervention. J Clin Invest 1999; 103:1237-41; PMID:10225966; http://dx.doi.org/10.1172/JCI6870
- Cornelius LA, Nehring LC, Harding E, Bolanowski M, Welgus HG, Kobayashi DK, Pierce RA, Shapiro SD. Matrix metalloproteinases generate angiostatin: effects on neovascularization. J Immunol 1998; 161:6845-52; PMID:9862716
- Hynes RO. The extracellular matrix: not just pretty fibrils. Science 2009; 326:1216-9; PMID:19965464; http://dx.doi.org/10.1126/science.1176009
- Roy R, Yang J, Moses MA. Matrix metalloproteinases as novel biomarkers and potential therapeutic targets in human cancer. J Clin Oncol 2009; 27:5287-97; PMID:19738110; http://dx.doi.org/10.1200/JCO.2009.23.5556
- Jiang Y, Goldberg ID, Shi YE. Complex roles of tissue inhibitors of metalloproteinases in cancer. Oncogene 2002; 21:2245-52; PMID:11948407; http://dx.doi.org/10.1038/sj.onc.1205291
- Guedez L, McMarlin AJ, Kingma DW, Bennett TA, Stetler-Stevenson M, Stetler-Stevenson WG. Tissue inhibitor of metalloproteinase-1 alters the tumorigenicity of Burkitt's lymphoma via divergent effects on tumor growth and angiogenesis. Am J Pathol 2001; 158:1207-15; PMID:11290537; http://dx.doi.org/10.1016/S0002-9440(10)64070-9
- Bergamaschi A, Tagliabue E, Sorlie T, Naume B, Triulzi T, Orlandi R, Russnes HG, Nesland JM, Tammi R, Auvinen P, et al. Extracellular matrix signature identifies breast cancer subgroups with different clinical outcome. J Pathol 2008; 214:357-67; PMID:18044827; http://dx.doi.org/10.1002/path.2278
- Finak G, Bertos N, Pepin F, Sadekova S, Souleimanova M, Zhao H, Chen H, Omeroglu G, Meterissian S, Omeroglu A, et al. Stromal gene expression predicts clinical outcome in breast cancer. Nat Med 2008; 14:518-27; PMID:18438415; http://dx.doi.org/10.1038/nm1764
- DeNardo DG, Brennan DJ, Rexhepaj E, Ruffell B, Shiao SL, Madden SF, Gallagher WM, Wadhwani N, Keil SD, Junaid SA, et al. Leukocyte complexity predicts breast cancer survival and functionally regulates response to chemotherapy. Cancer Discov 2011; 1:54-67; PMID:22039576; http://dx.doi.org/10.1158/2159-8274.CD-10-0028
- Martinet L, Garrido I, Filleron T, Le Guellec S, Bellard E, Fournie JJ, Rochaix P, Girard JP. Human solid tumors contain high endothelial venules: association with T- and B-lymphocyte infiltration and favorable prognosis in breast cancer. Cancer Res 2011; 71:5678-87; PMID:21846823; http://dx.doi.org/10.1158/0008-5472.CAN-11-0431
- Ahn S, Cho J, Sung J, Lee JE, Nam SJ, Kim KM, Cho EY. The prognostic significance of tumor-associated stroma in invasive breast carcinoma. Tumour Biol 2012; 33:1573-80; PMID:22581521; http://dx.doi.org/10.1007/s13277-012-0411-6
- Min KW, Kim DH, Do SI, Pyo JS, Kim K, Chae SW, Sohn JH, Oh YH, Kim HJ, Choi SH, et al. Diagnostic and prognostic relevance of MMP-11 expression in the stromal fibroblast-like cells adjacent to invasive ductal carcinoma of the breast. Ann Surg Oncol 2013; 20 Suppl 3:S433-42; PMID:23115007; http://dx.doi.org/10.1245/s10434-012-2734-3
- Koontongkaew S. The tumor microenvironment contribution to development, growth, invasion and metastasis of head and neck squamous cell carcinomas. J Cancer 2013; 4:66-83; PMID:23386906; http://dx.doi.org/10.7150/jca.5112
- Madar S, Goldstein I, Rotter V. 'Cancer associated fibroblasts'–more than meets the eye. Trends Mol Med 2013; 19:447-53; PMID:23769623; http://dx.doi.org/10.1016/j.molmed.2013.05.004
- Soon PS, Kim E, Pon CK, Gill AJ, Moore K, Spillane AJ, Benn DE, Baxter RC. Breast cancer-associated fibroblasts induce epithelial-to-mesenchymal transition in breast cancer cells. Endocr Relat Cancer 2013; 20:1-12; PMID:23111755; http://dx.doi.org/10.1530/ERC-12-0227
- Giannoni E, Bianchini F, Calorini L, Chiarugi P. Cancer associated fibroblasts exploit reactive oxygen species through a proinflammatory signature leading to epithelial mesenchymal transition and stemness. Antioxid Redox Signal 2011; 14:2361-71; PMID:21235356; http://dx.doi.org/10.1089/ars.2010.3727
- Kalluri R, Zeisberg M. Fibroblasts in cancer. Nat Rev Cancer 2006; 6:392-401; PMID:16572188; http://dx.doi.org/10.1038/nrc1877
- Mao Y, Keller ET, Garfield DH, Shen K, Wang J. Stromal cells in tumor microenvironment and breast cancer. Cancer Metastasis Rev 2013; 32:303-15; PMID:23114846; http://dx.doi.org/10.1007/s10555-012-9415-3
- Paraiso KH, Smalley KS. Fibroblast-mediated drug resistance in cancer. Biochem Pharmacol 2013; 85:1033-41; PMID:23376122; http://dx.doi.org/10.1016/j.bcp.2013.01.018
- Rasanen K, Virtanen I, Salmenpera P, Grenman R, Vaheri A. Differences in the nemosis response of normal and cancer-associated fibroblasts from patients with oral squamous cell carcinoma. PLoS One 2009; 4:e6879; PMID:19721715; http://dx.doi.org/10.1371/journal.pone.0006879
- Tjomsland V, Spangeus A, Valila J, Sandstrom P, Borch K, Druid H, Falkmer S, Falkmer U, Messmer D, Larsson M. Interleukin 1alpha sustains the expression of inflammatory factors in human pancreatic cancer microenvironment by targeting cancer-associated fibroblasts. Neoplasia 2011; 13:664-75; PMID:21847358
- De Monte L, Reni M, Tassi E, Clavenna D, Papa I, Recalde H, Braga M, Di Carlo V, Doglioni C, Protti MP. Intratumor T helper type 2 cell infiltrate correlates with cancer-associated fibroblast thymic stromal lymphopoietin production and reduced survival in pancreatic cancer. J Exp Med 2011; 208:469-78; PMID:21339327; http://dx.doi.org/10.1084/jem.20101876
- Gerber PA, Hippe A, Buhren BA, Muller A, Homey B. Chemokines in tumor-associated angiogenesis. Biol Chem 2009; 390:1213-23; PMID:19804363; http://dx.doi.org/10.1515/BC.2009.144
- Franco OE, Shaw AK, Strand DW, Hayward SW. Cancer associated fibroblasts in cancer pathogenesis. Semin Cell Dev Biol 2010; 21:33-9; PMID:19896548; http://dx.doi.org/10.1016/j.semcdb.2009.10.010
- Xing F, Saidou J, Watabe K. Cancer associated fibroblasts (CAFs) in tumor microenvironment. Front Biosci (Landmark Ed) 2010; 15:166-79; PMID:20036813; http://dx.doi.org/10.2741/3613
- Vesely MD, Kershaw MH, Schreiber RD, Smyth MJ. Natural innate and adaptive immunity to cancer. Annu Rev Immunol 2011; 29:235-71; PMID:21219185; http://dx.doi.org/10.1146/annurev-immunol-031210-101324
- Adams TE, Alpert S, Hanahan D. Non-tolerance and autoantibodies to a transgenic self antigen expressed in pancreatic beta cells. Nature 1987; 325:223-8; PMID:3543686; http://dx.doi.org/10.1038/325223a0
- Eiro N, Pidal I, Fernandez-Garcia B, Junquera S, Lamelas ML, del Casar JM, Gonzalez LO, Lopez-Muniz A, Vizoso FJ. Impact of CD68/(CD3+CD20) ratio at the invasive front of primary tumors on distant metastasis development in breast cancer. PLoS One 2012; 7:e52796; PMID:23300781; http://dx.doi.org/10.1371/journal.pone.0052796
- Hanahan D, Coussens LM. Accessories to the crime: functions of cells recruited to the tumor microenvironment. Cancer Cell 2012; 21:309-22; PMID:22439926; http://dx.doi.org/10.1016/j.ccr.2012.02.022
- Gonzalez LO, Gonzalez-Reyes S, Marin L, Gonzalez L, Gonzalez JM, Lamelas ML, Merino AM, Rodriguez E, Pidal I, del Casar JM, et al. Comparative analysis and clinical value of the expression of metalloproteases and their inhibitors by intratumour stromal mononuclear inflammatory cells and those at the invasive front of breast carcinomas. Histopathology 2010; 57:862-76; PMID:21166700; http://dx.doi.org/10.1111/j.1365-2559.2010.03723.x
- Eiro N, Gonzalez L, Gonzalez LO, Fernandez-Garcia B, Lamelas ML, Marin L, Gonzalez-Reyes S, del Casar JM, Vizoso FJ. Relationship between the inflammatory molecular profile of breast carcinomas and distant metastasis development. PLoS One 2012; 7:e49047; PMID:23145063; http://dx.doi.org/10.1371/journal.pone.0049047
- Kurose K, Gilley K, Matsumoto S, Watson PH, Zhou XP, Eng C. Frequent somatic mutations in PTEN and TP53 are mutually exclusive in the stroma of breast carcinomas. Nat Genet 2002; 32:355-7; PMID:12379854; http://dx.doi.org/10.1038/ng1013
- Patocs A, Zhang L, Xu Y, Weber F, Caldes T, Mutter GL, Platzer P, Eng C. Breast-cancer stromal cells with TP53 mutations and nodal metastases. N Engl J Med 2007; 357:2543-51; PMID:18094375; http://dx.doi.org/10.1056/NEJMoa071825
- Hosein AN, Wu M, Arcand SL, Lavallee S, Hebert J, Tonin PN, Basik M. Breast carcinoma-associated fibroblasts rarely contain p53 mutations or chromosomal aberrations. Cancer Res 2010; 70:5770-7; PMID:20570891; http://dx.doi.org/10.1158/0008-5472.CAN-10-0673
- Eiró N, Sendon-Lago J, Seoane S, Bermúdez MA, Lamelas ML, Garcia-Caballero T, Schneider J, Perez-Fernandez R, Vizoso FJ. Potential therapeutic effect of the secretome from human uterine cervical stem cells against both cancer and stromal cells compared with adipose tissue stem cells. Oncotarget 2014; 5:10692-708; PMID:25296979
- Paget S. The distribution of secondary growths in cancer of the breast. 1889. Cancer Metastasis Rev 1989; 8:98-101; PMID:2673568
- Vizoso FJ, Gonzalez LO, Corte MD, Rodriguez JC, Vazquez J, Lamelas ML, Junquera S, Merino AM, Garcia-Muniz JL. Study of matrix metalloproteinases and their inhibitors in breast cancer. Br J Cancer 2007; 96:903-11; PMID:17342087; http://dx.doi.org/10.1038/sj.bjc.6603666
- Del Casar JM, Gonzalez LO, Alvarez E, Junquera S, Marin L, Gonzalez L, Bongera M, Vazquez J, Vizoso FJ. Comparative analysis and clinical value of the expression of metalloproteases and their inhibitors by intratumor stromal fibroblasts and those at the invasive front of breast carcinomas. Breast Cancer Res Treat 2009; 116:39-52; PMID:19241156; http://dx.doi.org/10.1007/s10549-009-0351-z
- Allred DC, Harvey JM, Berardo M, Clark GM. Prognostic and predictive factors in breast cancer by immunohistochemical analysis. Mod Pathol 1998; 11:155-68; PMID:9504686
- Nielsen TO, Hsu FD, Jensen K, Cheang M, Karaca G, Hu Z, Hernandez-Boussard T, Livasy C, Cowan D, Dressler L, et al. Immunohistochemical and clinical characterization of the basal-like subtype of invasive breast carcinoma. Clin Cancer Res 2004; 10:5367-74; PMID:15328174; http://dx.doi.org/10.1158/1078-0432.CCR-04-0220