Abstract
While the link between thermoregulation and aging is generally accepted, much further research, reflection, and debate is required to elucidate the physiological and molecular pathways that generate the observed thermal-induced changes in lifespan. Our aim in this review is to present, discuss, and scrutinize the thermoregulatory mechanisms that are implicated in the aging process in endotherms and ectotherms. Our analysis demonstrates that low body temperature benefits lifespan in both endothermic and ectothermic organisms. Research in endotherms has delved deeper into the physiological and molecular mechanisms linking body temperature and longevity. While research in ectotherms has been steadily increasing during the past decades, further mechanistic work is required in order to fully elucidate the underlying phenomena. What is abundantly clear is that both endotherms and ectotherms have a specific temperature zone at which they function optimally. This zone is defended through both physiological and behavioral means and plays a major role on organismal senescence. That low body temperature may be beneficial for lifespan is contrary to conventional medical theory where reduced body temperature is usually considered as a sign of underlying pathology. Regardless, this phenomenon has been targeted by scientists with the expectation that advancements may compress morbidity, as well as lower disease and mortality risk. The available evidence suggests that lowered body temperature may prolong life span, yet finding the key to temperature regulation remains the problem. While we are still far from a complete understanding of the mechanisms linking body temperature and longevity, we are getting closer.
Abbreviations
Tb | = | body temperature |
Introduction
The earliest scientific reference of centenarian longevity comes from the astronomer Hipparchus of Nicea who confirmed that Democritus of Abdera lived 109 y (c. 470/460 – c. 370/360 BC).Citation1 While such extended life spans appear relatively often nowadays, aging and longevity are among of the most rapidly advancing areas in biological research, with an ever-increasing number of physiological, molecular, pharmacological, and dietary factors being implicated in organismal senescence that may serve as targets for treatment. In this light, evidence-based research indicates that the rate of aging is mainly determined by genetics, environmental factors, and lifestyle choices.Citation2,3 Interestingly, while a large number of gene variants are associated with aging, they explain a small fraction of hereditary longevity.Citation4,5 Indeed, a number of studies have shown that only 23–33% of lifespan variation can be attributed to genetic factors, while the rest is determined by behavior and environmental factors ().Citation2,5-7 Therefore, it appears that the accumulation of molecular disorder that contributes to somatic deterioration is subject to considerable plasticity. Among the most important environmental factors known to affect longevity is body temperature (Tb) and the majority of the available evidence suggests that increased Tb leads to shorter lifespan.Citation3 However, while the link between thermoregulation and aging is generally accepted, much further research, reflection, and debate is required to elucidate the physiological and molecular pathways that generate the observed thermal-induced changes in lifespan. Our aim in this review is to present, discuss, and scrutinize the thermoregulatory mechanisms that are implicated in the aging process in endotherms and ectotherms. We hope that this comparative approach will aid our understanding of aging and longevity and will contribute toward future advancements that could compress morbidity and lower disease and mortality risk.
Aging, Physiology, and Functional Integrity
Aging encapsulates all time-induced phenomena occurring during an organism's lifespan. While the term ‘aging’ is often used to describe the accumulation of molecular disorder that contributes to somatic deterioration (), aging is not always associated with a decline in structural or functional integrity. For instance, aging in the first quarter (or more) of an organism's lifetime is associated with significant growth and marked improvements in a broad range of physiological functions. At the other end of the life span, aging in the final quarter (or more) of an organism's lifetime is associated with tissue damage and physical decline, usually linked with underlying pathologies and disease susceptibility (). This latter form of aging will be the principal focus of the current review. Specifically, we present the available state of knowledge in endotherms and ectotherms regarding the link between thermoregulation and different physiological adaptations that lead to loss of functional capability and senescence.
Body Temperature and Longevity
In all organisms, Tb is affected by many factors and exerts pleiotropic effects that influence almost all bodily systems and organs. As such, its impact on aging and longevity has been difficult to isolate. Nevertheless, the effects of reduced Tb on health and longevity have been circulating since 1917 (reviewed in South et al., 1972)Citation8, and it is now unanimously accepted that low Tb benefits lifespan.Citation3,9-12 For instance, men exhibiting Tb below the median demonstrated significantly higher survival rates over 25 y of follow-up in the Baltimore Longitudinal Study of Aging.Citation12 In mice, reducing Tb through genetic manipulation results in 20% increase in life span,Citation9 while male C57B1/6 mice (one of the most common inbred strain of laboratory mice) display a lower Tb and live longer than their female peers.Citation13 Similar results are also observed in ectotherms, where many organisms, including fish, butterflies, and C. elegans, demonstrate increased lifespan at low ambient temperatures.Citation14-18 Interestingly, the general understanding until recently was that Tb is an indicator of metabolic rateCitation19 and, therefore, it merely portrays the effect of altered metabolic rates on longevity. However, recent data revealed that thermosensory neurons involved in thermoregulatory behavior play a key role in determining lifespan.Citation17,20,21 This discovery brought forth the interesting view that thermal sensation serves as a vital input for organismal homeostasis in addition to its role in regulating behavioral and autonomic thermoregulation.
Endothermy and Longevity
Endothermic thermoregulation
The defining characteristic of endotherms is the maintenance of their internal environment at a metabolically favorable temperature achieved primarily through heat released by internal bodily functions (instead of almost complete dependence on ambient heat, as seen in ectotherms). This internal heat release originates primarily from routine metabolism since the fundamental chemical reactions generating ATP are highly inefficient. Indeed, dissipation of the electrochemical gradient produced when electrons are pumped across the mitochondrial inner membrane occurs not only by protons entering the mitochondrial matrix through ATP synthase (in a process that generates ATP) but also by protons bypassing the ATP synthase entrance and returning to the mitochondrial matrix through uncoupling agents (such as thyroxine and uncoupling protein 1). The latter process converts the energy of the electrochemical gradient to heat and represents approximately 70–95% of the energy released through substrate oxidation.
Under conditions of cold exposure or low metabolic activity, endotherms employ heat production mechanisms to maintain Tb at the desired level. These mechanisms include shivering (i.e., increased muscle metabolism leading to heat release as described above) and non-shivering (i.e., uncoupled oxidative metabolism in brown adipose tissue) thermogenesis. The latter depends entirely on the function of uncoupling proteinCitation1,22,23 a mitochondrial inner membrane protein that is expressed exclusively in brown adipose tissue.Citation24,25 The presence of uncoupling protein 1 in brown adipocytes provides an alternative biochemical pathway compared to the rest of eukaryotic cells, which results in the production of heat instead of ATP. Indeed, brown adipocytes express large amounts of uncoupling protein 1 which allows protons to bypass the ATP synthase entry and return to the mitochondrial matrix. Through this process, the energy of the electrochemical gradient is dissipated as heat.Citation22,26,27
In addition to heat production, endotherms employ also heat conservation (sympathetic cutaneous vasoconstriction and blood redistribution), heat loss (cholinergic cutaneous vasodilation and eccrine sweating), and behavioral thermoregulation (conscious decisions directly aiming at Tb regulation) mechanisms (). These powerful mechanisms (reviewed in detail elsewhere)Citation28-31 allow endotherms to maintain their internal environment at a metabolically favorable temperature.
Mechanisms linking low Tb and longevity in endotherms
The beneficial effects of low Tb on longevity are contrary to conventional medical theory where reduced Tb is usually considered as a sign of underlying pathology, particularly in the elderly.Citation32-37 Nevertheless, studies in mice show that low Tb extends life span by exploiting metabolic pathways that are known to attenuate autoimmunity in old age.Citation38 Further contradicting common medical theory, reduced Tb has been shown to augment resistance to environmental stressors such as irradiation.Citation38,39 In addition, low Tb has been shown to favorably influence immune function (reviewed in Walsh and Whitham, 2006).Citation40 Specifically, core temperature reductions of 0.5°CCitation41 and 0.6°CCitation42 result in elevated natural killer cell activity, while further attenuation to 0.8°C in core temperature does not affect total leukocyte numbers,Citation43 and a 1°C core temperature drop during surgery leads to reduced lymphocyte proliferation and interleukin 2 production 24 and 48 hours post surgery.Citation44 More importantly, chronic reductions in Tb, have been shown to either benefitCitation45,46 or not hamperCitation47 immune responsiveness. These results are in line with in vitro experiments showing that monocytes incubated for one hour at 34°C killed a greater number of E. coli compared with incubation for one hour at 37°C.Citation48 Chronic attenuation of Tb in mice via repeated cold exposure induces heat shock proteins in brown adipose tissue, with increased binding of heat shock transcription factors to DNA that may contribute to the development of cold tolerance.Citation49-51 The transcription of heat shock proteins is initiated by norepinephrine after binding to brown adipose tissue adrenergic receptors. In turn, the heat shock proteins facilitate the translocation and activity of the enzymes involved in heat generation and may interfere with the inflammatory response mediated by tumor necrosis factor α.Citation49,50,52 Therefore, it could be cautiously proposed that low Tb may lead to increased lifespan through beneficial effects on immune function. However, the link between low Tb and immune function is not completely understood, while the connections between inflammation and immune function with low Tb appear to depend on the severity of the stress imposed by the extent and duration of the cold exposure, as well as the acclimation level of the individual.Citation53-55 Indeed, cold exposure that is sufficiently severe to induce an unremitting stress leads to augmented cortisol production and, in turn, immunosuppressive response.Citation54 Therefore, while cold acclimation leads to suppressed cortisol responseCitation53 and immune system activation,Citation45 the overall immune response to cold exposure appears to be context-dependent especially in non-acclimated individuals.
In a previous study we showed that being born during the colder seasons of the year is associated with increased birth weight, gestational age, and longevity, as well as with lower risk of fetal growth restriction, and premature birth.Citation56 In contrast, being born during the warmer seasons is negatively associated with birth weight, gestational age, and longevity.Citation56 These findings are supported by European and African data showing that birth weight and longevity are positively associated with being born during the colder seasons but negatively associated with being born during the remaining periods of the year.Citation57-59 These data support the notion that the rate of aging may be affected by low Tb at critical periods of early development. It is well known that the fragile biochemical equilibrium of the mammalian intrauterine environment is significantly associated with environmental factors.Citation60-64 Therefore, it could be proposed that low Tb during pregnancy and early development may be linked to permanent structural, functional, and metabolic changes, which, in turn, can lead to physiological or metabolic ‘programming’ of the newborn with beneficial effects on longevity. One example of early-life programming that may be beneficial for long term health and longevity pertains to brown adipose tissue which is maximally recruited at birth in response to the cold challenge of the extra-uterine environment.Citation65 While the extent to which brown adipose tissue content and function during adulthood are affected by early-life factors remains to be established, the available data in mammals show that the physiology of this tissue during early life is dependent on the exposure of the offspring to the cool temperature challenge of the extra-uterine environment.Citation66,67 At this point, it is important to note that the aforementioned link between rate of aging and low Tb may, in fact, reflect differences in tissue perfusion (i.e., low tissue perfusion) and/or metabolism. Nevertheless, this contention remains to be explored by future studies.
The beneficial effects of low Tb on health outcomes and, in turn, longevity, may be explained, at least in part, by enhanced activity of uncoupling proteins. Uncoupling protein 1 is a transporter located in the inner mitochondrial membrane of brown adipose tissue. Its role is to enhance proton conductivity by uncoupling ATP production from substrate oxidation resulting in heat production when the animal is exposed to a cold environment (reviewed in Rial and Zardoya, 2009).Citation68 When maintained in a standard housing temperature (23°C), uncoupling protein 1 deficient mice develop an age-associated increase in diet-induced obesity.Citation69 Uncoupling protein 2 knock-out mice reveal increased prevalence for autoimmune diabetes characterized by increased macrophage infiltration of islets, enhanced interleukin 1β and nitric oxide production from macrophages, as well as augmented molecular damage induced by reactive oxygen species.Citation70 In humans, obesity has been genetically associated with attenuated uncoupling protein 1 gene expression caused by an uncoupling protein 1 promoter polymorphism.Citation71 Furthermore, acceleration of cellular respiration resulting from uncoupled oxidative phosphorylation for the purpose of heat release leads to attenuated production of reactive oxygen species (reviewed in Rial and Zardoya, 2009).Citation68 Consequently, uncoupling proteins have been recognized as key players in the antioxidant defense system of eukaryotesCitation68 and their activity may explain, at least in part, the improved health and increased longevity associated with a low Tb.
Body mass represents the capacity of the body to store heat and it is linearly associated with Tb in most species. In humans, increased body massCitation72,73 and obesityCitation74 are associated with higher Tb independently of age. A reduction in white adipose tissue content in low Tb animals is a relevant consistency that has significant health implications.Citation3 For example, increased adiposity has been linked to high levels of inflammation which, in turn, have been associated with several chronic diseases.Citation75,76 Moreover, it is worth noting that the link between Tb and obesity has been also assessed through environmental observations, demonstrating that continuous exposure to neutral or high ambient temperatures may partly contribute to the obesity epidemic.Citation77 Based on these findings, it could be argued that the inverse association between Tb and longevity may reflect, at least in part, the well-knownCitation78,79 pathophysiological processes related to obesity. On the same topic, chronic low Tb is also known to result from caloric restriction.Citation80,81 Some have argued, therefore, that part of the longevity conferred by Tb may be due to caloric restriction, or vice versa.Citation11 However, an elegant study by Conti and colleaguesCitation9 has provided solid evidence that Tb has an independent role in determining lifespan. In that study, chronic low Tb in transgenic mice resulted in a 12% and 20% increase in median lifespan from birth, in male and female mice, respectively. Interestingly, these transgenic animals gained body mass during this study without a significant change in food intake, a finding that effectively dissociates the longevity effect of low Tb from that of caloric restriction. Nevertheless, the fact that low Tb may reflect, at least in part, the beneficial effects of caloric restriction on longevity cannot be ruled out.Citation9 The proposed mechanisms linking caloric restriction and Tb as well as their beneficial effects on longevity are illustrated in .
Figure 3. A conceptual model illustrating the mechanisms linking caloric restriction and reduced body temperature (Tb) and their influence on longevity. Adapted from: Carrillo AE, Flouris AD. Caloric restriction and longevity: effects of reduced body temperature. Aging Res Rev 2011; 10:153–62. Note: BAT = brown adipose tissue; UCP1 = uncoupling protein one; ROS = reactive oxygen species.
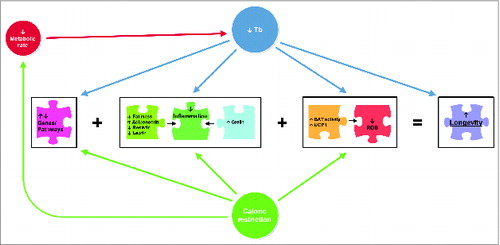
Ectothermy and Longevity
Ectothermic thermoregulation
While Tb in endotherms is mainly determined and controlled by cellular metabolism,Citation82 ectotherms regulate Tb mainly though behavioral mechanisms.Citation83 Thermoregulation in ectothermic organisms is a neuronal process and, interestingly, the pathways that link thermal stimuli to metabolic acclimation in ectotherms are comparable to those associated with thermoregulation in endotherms. Specifically, the afferent signals originated in thermosensory receptors are conducted through the dorsal horn of the medulla, reach the hypothalamus, and are translated as efferent sympathetic outflows that stimulate transcriptional regulators of metabolic processes and behavioral mechanisms to maintain a constant internal environment relative to external environmental variation.Citation82,84-86 In some ectotherms, metabolism is also regulated at organ (e.g. cardiovascular modulation rates) and cellular (e.g., mitochondrial capacities) levels, and it is always subject to environmental temperature.Citation86 For example, short-term exposure to extremely high or low ambient temperature may alter the expression of heat shock genes, thereby protecting proteins from temperature-induced damage.Citation86,87 Long-term exposures to extreme ambient temperature (e.g., acclimatization) may cause greater tolerance to subsequence thermal variation which can alter performance and affect fitness.Citation88-90 Many ectothermic organisms have developed the capacity for thermal acclimatization and can adjust their metabolism to tolerate extreme temperatures or seasonal variation.Citation91-93 For instance, some reptiles, including the Alligator mississipiensis, present acclimatization which, combined with maximized sun exposure, leads to higher Tb's in winter than in summer.Citation94
Ectotherms can maintain their Tb within a relatively narrow temperature range despite the temporal and geographic variation in the thermal habitats they occupy.Citation95 Nevertheless, Tb during inactivity and the amount of hours of activity exposed to a selected ambient temperature may vary due to constrains of the specific thermal environment.Citation96 Heat exchange with the environment occurs via radiation, convection, and conduction and varies in relation to body composition and size ().Citation97,98 For example, evaporative cooling in reptiles occurs via panting which minimizes dehydration. In amphibians, evaporative cooling can lead to dehydration if the access to water is not available. These mechanisms of heat exchange are illustrated in . With respect to body size, a higher surface to volume ratio translates into higher rates of heat exchange and, in amphibians, also generates higher rates of water loss. Most invertebrates, fish, anurans and reptiles present an inverse relationship between ambient temperature and body size (the “temperature size rule,” Atkinson, 1994; Atkinson & Sibly 1997) which is commonly associated to a higher survival in cold environments and lower predation risk (Ray, 1960; Ashton et al, 2000; Ashton and Feldman, 2003; Heinze et al, 2003). On the other hand, body size of some diatoms (e.g., Phaeodaetylum trieornutum), copepods (e.g., Salmineola salmoneus) mayflis of the genus Ephemeroptera, salamanders and squamates (lizards and snakes) generally decrease with latitude and elevation (Atkinson, 1995; Laugen et al, 2005; Pincheira-Donoso et al, 2008; Vitt and Caldwell, 2009). The lack of a common pattern for ectotherms indicates a complex undetermined relationship between size and thermoregulation and general statements cannot be made (Angiletta and Dunham, 2003). Variation in body size in ectotherms determine differences in the total time of exposure (e.g., to predators, to solar radiation) and time of activity (e.g., foraging, mating) among populations that share the same preferred temperature and may result in variations in life-history traits such as aging and longevity.Citation99 Metabolic rate increases with activity time (e.g., pursuing prey and mating) together with an increase in energy utilization that is maximized at or near the peak Tb.Citation100-104 The utilized energy that is not expended will be allocated to reproduction, growth and storage.Citation96 Following maturity, a pronounced reallocation of energy from maintenance and growth to reproduction is observed; shaped by natural selection, the investments in each of these functions result in a broad variety of life history traits and phenology (e.g., age at maturity and longevity).Citation105
Ectothermic mechanisms linking low Tb and longevity
Among ectotherms, variation in body size and life-history traits – such as age at maturity and longevity – are usually highly geographically variable and are heavily dependent on the local climate.Citation106-108 The effects of reduced Tb on ectotherm health and longevity have been circulating since 1917.Citation8 In poikilotherms, early studies showed that fish living in 15°C water lived significantly longer than fish living in 20°C water.Citation15 Similarly, Drosophila melanogaster was shown to live approximately twice as long at 21°C than at 27°C,Citation109 while the lifespan of C. elegans was increased by 75% by reducing temperature by 5°C.Citation110
In 1960, RayCitation111 observed that plants and animals at low ambient temperatures grew slowly and delayed sexual maturation long enough to attain maturation at larger sizes than at high ambient temperatures. One probable explanation for these findings is that, since reproductive events are relatively less frequent in colder environments, larger body size would be favored by natural selection to enhance fecundity. On the other hand, in colder environments a larger body size at maturity is adaptive only if it coincides with a low survivorship of adults.Citation112 Finally, in colder environments larger females produce larger offspring or offer better parental care when mating with larger males.Citation113,114 Living in hot regions and/or having longer activity seasons allow ectotherms to spend more time at high Tb and this usually results in fast growth and an early sexual maturity.Citation99,115-118 For example, growth rates of juvenile Lacerta vivipara, Sceloporus occidentalis, and S. graciosus increase with daily activity time due to high Tb via radiant heat,Citation104,119120 while maturity may be accelerated in laboratory animals that are maintained under optimal thermal conditions.Citation103,121 These mechanisms can have different effects in individual animals within a species or population and further research will help us understand the relationships among temperature, growth rate and body size.
Growth rates and ages at metamorphosis, sexual maturation, and death in ectotherms are associated traits that depend on metabolic rate.Citation122 Due to their dependence on ambient temperature, ectotherms exhibit significant differences between the chronological and physiological growth and longevity. These are combined in different ways and applied in diverse environments and are, ultimately, linked to adaptive and evolutionary processes imposed by natural selection.Citation123 For instance, reproductive fitness is negatively affected when delayed maturity is associated with a short lifespan.Citation18,112,124 In ectotherms, especially in thermoconformers, cold and warm ambient temperatures attenuate and increase metabolic rate, respectively. Thermoregulatory ectotherms (e.g., lizards) may maintain a Tb within a narrow range but they are subjected to seasonal and daily variations that determine their hours of activity and affect their overall growth and lifespan. Therefore, populations of the same species may exhibit different growth rates and longevity when inhabiting or exposed to different environments.Citation18,125 Nevertheless, the relationships between Tb and metabolic rate, as well as low Tb-increase in lifespan and high Tb-premature mortality, are commonly observed in ectothermsCitation17 and – to date – have been generally attributed to the link between Tb and metabolic rate.Citation20,126
Different thermoregulatory mechanisms enable ectotherms to influence the effect of ambient temperature over their physiological processes (e.g., temperature-sensitive circadian mechanisms).Citation127,128 Behavioral thermoregulation is the main mechanism by which ectotherms maintain their Tb as close as possible to an independently defined target range at which their performance tends to be maximal.Citation129-134 Given that behavioral thermoregulation functions through conscious decisions, it can have a significant impact on lifespan. For instance, in lizards and fish, neurons in the preoptic hypothalamus function as feedback reflex that direct thermoregulatory behaviorCitation135 encompassing changes of microhabitat,Citation136-141 site and retreat selection,Citation134,141-144 shifts in activity hours,Citation100-102,145-148 variations in the frequency of exposure and duration of stays in full sun,Citation148-151 as well as modifying posture.Citation140,143,152 Importantly, the most common thermoregulatory behavior consists in shuttling between sun and shade or hot and cold microenvironments.Citation153-155 For example, thermoregulatory diurnal lizards would maintain their Tb within the preferred range especially during the activity hours, basking in the sun until they reach near-critical high Tb and shuttling to the shade to retreat until achieving cooler Tb.Citation90,130 Also, nocturnal geckos are active after sunset when ambient temperature is low but they retreat in refuge that will offer appropriate temperatures for optimal physiological functioning during the day.Citation156-158 These examples exemplify that, despite the obvious physiological benefits of thermoregulation for ectotherms, there are also many associated costs that ultimately affect aging and longevity.Citation101,102,159-161 For example, when shuttling from sun to shade a lizard spends energy for locomotion and the risk of being predated is increased, thus indirectly affecting lifespan.Citation116 Moreover, the necessity to thermoregulate may interfere not only in finding mates but also in foraging and acquiring the food required for growth.Citation161 Costs and physiological benefits are independent and may vary in different environments. The physiological optimal temperature of an organism corresponds usually to the tissue or cellular systemsCitation162 and it is generally inferred from the animal's preferred temperature. However, the latter is not necessarily near the ecologically optimal temperature, which is more relevant when trying to understand the whole-animal system.Citation161,16,164 Thus, the maximal performance of ectotherms is affected by how distant its ecologically optimal temperature is from its operative temperature: whole-organismal activities, like healing and surviving rates, predation success, egg production, metabolic scope, reproductive output, individual growth and longevity, depend directly not only on the environmental temperature but also on the efficiency of the animal's thermoregulatory behavior.
Physiological adjustments associated with thermal regulation in ectothermic vertebrates, such as cutaneous losses of water, panting, salivation, and urination which are all considered evaporative cooling processes, have been described since the early 1940sCitation153,165 but little is known about the thermoregulatory pathways that are involved in these processes as well as the way they may affect aging and longevity. Several studies have demonstrated similarities between mammalian and crocodilian afferent and efferent pathways (e.g., similar cardiovascular response to peripheral temperature variation).Citation166,167 Cardiovascular responses in other reptiles have been reported as principally mediated by autonomic mechanisms in snakes,Citation168 countercurrent heat exchangers in desert lizards (Phrynosoma sp., and Holbrookia sp)Citation169 and marine turtles (Chelonia mydas, and Caretta caretta),Citation170 as well as stimulated locally by prostaglandins as well as nitric oxide in lizards (Pogona vitticeps)Citation94 and crocodiles (Crocodylus porosus).Citation171 At the cellular level, variations in mitochondrial capacity and density are observed within species that occupy broad territories and diverse thermal environments.Citation172,173 This mechanism was also reported for several aquatic vertebrates like Anguilla Anguilla,Citation174 Champsocephalus esox and Eleginops maclovinus,Citation172 as well as Zoarces viviparusCitation175 and Fundulus heteroclitusCitation173 in response to changes in the thermal environment.
In the summer, the cellular capacity to oxidize piruvate and palmitoyl carnitine, associated with lower enzyme activities, in the red muscle of Oncorhynchus mykiss is reducedCitation176 and, consequently, reaction rates and mitochondrial capacity are maintained constant throughout the year despite the difference of 15°C in Tb found between summer and winter.Citation177 A complex network of sensory, neural, hormonal, and effector systems provide the means by which ectotherms sense and respond to environmental factors that may impose upon their thermal biology and longevity.Citation178 Experiments in the soil nematode Caenorhabditis elegans suggested that thermosensory neurons evolved in order to prevent lifespan reductions in warm environments by means of inhibiting thermosensory neurons through mutations or laser ablation.Citation20 At high ambient temperatures the amphid neurons with finger-like ciliated endings promote the transcription of the daf-9 gene, responsible for increasing the production of steroid hormone. The activation of the steroid signaling pathway blocks the activity of the DAF-12 nuclear hormone receptor and, thus, contributes to the observed anti-aging effects.Citation17 Another example is the positive effect of corticosterone on metabolic rate and Tb. In stressful situations the hypothalamus-pituitary-adrenal axis is activated and corticosterone is secreted in the blood stream, which promotes behavioral and physiological processes associated with survival mechanisms (e.g., appetite, cardiovascular functions).Citation179,180 In some reptiles, this hormone is also found in the plasma in benign environments, yet the reasons for this phenomenon remain unclear.Citation181 In the New Zealand common gecko, Hoplodactylus maculatus, the variation in non-stress-induced corticosterone is correlated with the variation in Tb.Citation182,183 Similar corticosterone-Tb correlations were found in green turtles (Chelonia mydas),Citation184 in male tautara (Sphenodon punctatus),Citation185 and in the marine iguana (Amblyrhynchus cristatus).Citation186 Geckos implanted with corticosterone would consume oxygen at a rate of 50% higher that the placebo geckos, display heat-seeking behaviors, present a higher Tb in their terraria and selected higher ambient temperature when in thermal gradients.Citation187 This mechanism might be of significance for the survival and lifespan of ectotherms inhabiting non stable environments with ample thermal daily and seasonal variations.Citation187 In the last decade, studies focusing on specific thermoregulatory mechanisms and innate factors that may be directly associated with longevity in ectotherms have flourished, yet many of the interactions between the physiological and ecological processes involved remain unclear.
Thermal plasticity and the efficiency of thermoregulation through physiological and behavioral mechanisms may compensate for environmental extreme conditions that could affect growth, aging and longevity, and subsequently fitness. Natural selection may favor changes in the physiology that determine the efficiency with which resources are assimilated and used for growth, therefore, promoting faster growth through thermal specialization.Citation188 To date, our knowledge on the interaction of these factors within the populations of ectothermic vertebrates remains limited and encourages us to pursue ways to further understand the natural framework in which the temperature-size relationships have evolved.
Comparative Remarks
The mechanisms through which thermoregulation may be implicated in the aging process in endotherms and ectotherms are illustrated in . In endotherms, low Tb has been shown to extend life span by augmenting resistance to environmental stressorsCitation38,39 and by exploiting metabolic pathways that are known to attenuate autoimmunity in old age.Citation38 In addition, low Tb has been shown to favorably influence immune function,Citation40 but this link is not completely understood, while the connections between inflammation and/or immune function with low Tb appear to depend on the severity of the stress imposed as well as the acclimation level of the individual.Citation53-55 The beneficial effects of low Tb may be also exerted during pregnancy and early development which are known to generate structural, functional, and metabolic changes in adult life.Citation56 One example of early-life programming that may be beneficial for long-term health and longevity pertains to brown adipose tissue which is maximally recruited at birth in response to the cold challenge of the extra-uterine environment.Citation65 While the link between early life Tb and later life brown adipose tissue function requires further research, enhanced activity of uncoupling proteins (particularly uncoupling protein 1 that is expressed in brown adipose tissue) are known to attenuate production of reactive oxygen species which, in turn, benefit longevity.Citation68 Finally, increased body massCitation72,73 and obesityCitation74 are associated with higher Tb independently of age, while reduced white adipose tissue content in low Tb animals is a relevant consistency that has significant life-prolonging implications.Citation3
Figure 5. The mechanisms through which thermoregulation may be implicated in the aging process in endotherms (A) and ectotherms (B).
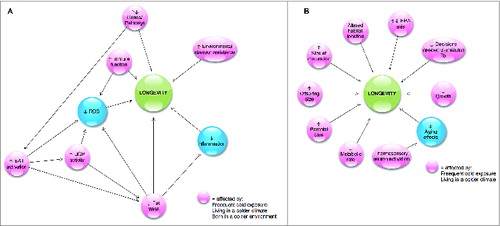
Among ectotherms, variation in body size and life-history traits – such as age at maturity and longevity – are usually highly geographically variable and are heavily dependent on local climate.Citation106-108 Ectothermic plants and animals at low ambient temperatures grow slowly and delay sexual maturation long enough to attain maturation at larger sizes than at high ambient temperatures.Citation111 Moreover, larger body size is favored by natural selection to enhance fecundity.Citation111 On the other hand, living in hot regions and/or having longer activity seasons allow ectotherms to spend more time at high Tb and this generally results in fast growth and an early sexual maturity.Citation99,115-118 These processes as well as the relationships between temperature and metabolic rate, low temperature-increase in lifespan and high temperature-premature mortality are generally attributed to the link between Tb and metabolic rate.Citation17,20,122,126 Another link between thermoregulation and aging in ectotherms relates to the fact that behavioral thermoregulation functions through conscious decisions that, ultimately, affect aging and longevity.Citation101,102,159-161 For example, when shuttling from sun to shade a lizard spends energy for locomotion and the risk of being predated is increased, thus indirectly affecting lifespan.Citation116 In this light, the maximal performance of ectotherms is affected by how distant its ecologically optimal temperature is from its operative temperature: whole-organismal activities including healing and surviving rates, predation success, egg production, metabolic scope, reproductive output, individual growth and longevity, depend directly not only on the environmental temperature but also on the efficiency of the animal's thermoregulatory behavior. Finally, recent studies in C. elegans showed that the sensation of heat via thermosensory neurons may affect lifespan. This suggests that thermal sensation serves as a vital input for organismal homeostasis, in addition to its role in regulating behavioral and autonomic thermoregulation.
Concluding Remarks
Our aim in this review is to present, discuss, and scrutinize the thermoregulatory mechanisms that are implicated in the aging process in endotherms and ectotherms. Our analysis demonstrates that low Tb benefits lifespan in both endothermicCitation3,9-13 and ectothermicCitation14-18 organisms. Research in endotherms has delved deeper into the physiological and molecular mechanisms linking Tb and longevity. Our knowledge on the physiological and molecular pathways linking Tb and longevity in ectotherms has been steadily increasing during the past decades, yet further mechanistic work is required in order to fully elucidate the underlying phenomena. What is abundantly clear is that both endotherms and ectotherms have a specific temperature zone at which they function optimally.Citation18,189-192 This zone is defended through both physiologicalCitation166,167,193,194 and behavioralCitation135-141,195,196 means and plays a major role on organismal senescence.Citation3,17,20,21
That low Tb may be beneficial for lifespan is contrary to conventional medical theory where reduced Tb is usually considered as a sign of underlying pathology.Citation32-37 Regardless, this phenomenon has been targeted by scientists with the expectation that advancements may compress morbidity, as well as lower disease and mortality risk. In his 1978 articleCitation10 in Geriatrics, Saul Kent wrote that “it is possible that lowered body temperature may prolong the human life span; finding the key to temperature regulation remains the problem, but the search is on.” Thirty-seven years later we are still far from a complete understanding of the mechanisms linking Tb and longevity, but we are getting closer.
Disclosure of Potential Conflicts of Interest
No potential conflicts of interest were disclosed.
Funding
A.D.F. was supported by funding from the European Union 7th Framework Program (FP7-PEOPLE-2012-IRSES; grant no. 319010; FP7-PEOPLE-2013-IRSES; grant no. 612547).
Additional information
Notes on contributors
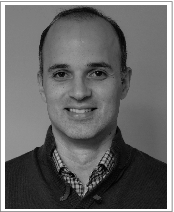
Andreas D Flouris
Andreas D Flouris is an Assistant Professor in Human Physiology at the University of Thessaly Department of Physical Education and Sport Science (Greece) and an Adjunct Professor in Environmental Medicine with the School of Human Kinetics, at the University of Ottawa (Canada). He is a Section Editor in Temperature, and an Associate Editor for the journals BMC Pulmonary Medicine and BMC Research Notes.

Carla Piantoni
Carla Piantoni is an ecophysiologist focused patterns of variation in performance, thermal sensitivity and growth in ectotherms across different environments and time. Her current projects at the Physiology Department of Bioscience Institute of the University of São Paulo, Brazil address the effects of global warming on the thermophysiology, performance and growth of a species of lizard, Tropidurus torquatus along a latitudinal and temporal gradient in the Cerrado Biome. She is also a Research Collaborator at the Smithsonian Institution (Washington, DC).
References
- Laertius D. The lives and opinions of eminent philosophers. Translated by Charles D. Yonge. London: Bohn H. G., 1853
- vB Hjelmborg J, Iachine I, Skytthe A, Vaupel JW, McGue M, Koskenvuo M, Kaprio J, Pedersen NL, Christensen K. Genetic influence on human lifespan and longevity. Hum Genet 2006; 119:312-21; PMID:16463022; http://dx.doi.org/10.1007/s00439-006-0144-y
- Carrillo AE, Flouris AD. Caloric restriction and longevity: effects of reduced body temperature. Ageing Res Rev 2011; 10:153-62; PMID:20969980; http://dx.doi.org/10.1016/j.arr.2010.10.001
- Budovsky A, Craig T, Wang J, Tacutu R, Csordas A, Lourenco J, Fraifeld VE, de Magalhaes JP. LongevityMap: a database of human genetic variants associated with longevity. Trends Genet 2013; 29:559-60; PMID:23998809; http://dx.doi.org/10.1016/j.tig.2013.08.003
- Wheeler HE, Kim SK. Genetics and genomics of human ageing. Philos Trans R Soc Lond B Biol Sci 2011; 366:43-50; PMID:21115529; http://dx.doi.org/10.1098/rstb.2010.0259
- Ljungquist B, Berg S, Lanke J, McClearn GE, Pedersen NL. The effect of genetic factors for longevity: a comparison of identical and fraternal twins in the Swedish twin registry. J Gerontol A Biol Sci Med Sci 1998; 53:M441-6; PMID:9823748; http://dx.doi.org/10.1093/gerona/53A.6.M441
- McGue M, Vaupel JW, Holm N, Harvald B. Longevity is moderately heritable in a sample of Danish twins born 1870-1880. J Gerontol 1993; 48:B237-44; PMID:8227991; http://dx.doi.org/10.1093/geronj/48.6.B237
- South FE, Hannon JP, Willis JR. Hibernation and Hypothermia: Perspectives and Challenges. New York: Elsevier Scientific Publishing Co., 1972
- Conti B, Sanchez-Alavez M, Winsky-Sommerer R, Morale MC, Lucero J, Brownell S, Fabre V, Huitron-Resendiz S, Henriksen S, Zorrilla EP, et al. Transgenic mice with a reduced core body temperature have an increased life span. Science 2006; 314:825-8; PMID:17082459; http://dx.doi.org/10.1126/science.1132191
- Kent S. Body temperature and life span. Geriatrics 1978; 33:109-12, 16; PMID:355058
- Tabarean I, Morrison B, Marcondes MC, Bartfai T, Conti B. Hypothalamic and dietary control of temperature-mediated longevity. Ageing Res Rev 2010; 9:41-50; PMID:19631766; http://dx.doi.org/10.1016/j.arr.2009.07.004
- Roth GS, Lane MA, Ingram DK, Mattison JA, Elahi D, Tobin JD, Muller D, Metter EJ. Biomarkers of caloric restriction may predict longevity in humans. Science 2002; 297:811; PMID:12161648; http://dx.doi.org/10.1126/science.1071851
- Sanchez-Alavez M, Alboni S, Conti B. Sex- and age-specific differences in core body temperature of C57Bl/6 mice. Age (Dordr) 2011; 33:89-99; PMID:20635153; http://dx.doi.org/10.1007/s11357-010-9164-6
- Klass MR. Aging in the nematode Caenorhabditis elegans: major biological and environmental factors influencing life span. Mech Ageing Dev 1977; 6:413-29; PMID:926867; http://dx.doi.org/10.1016/0047-6374(77)90043-4
- Liu RK, Walford RL. Increased growth and life-span with lowered ambient temperature in the annual fish, cynolebias adloffi. Nature 1966; 212:1277-8; http://dx.doi.org/10.1038/2121277a0
- Munch SB, Salinas S. Latitudinal variation in lifespan within species is explained by the metabolic theory of ecology. Proc Natl Acad Sci U S A 2009; 106:13860-4; PMID:19666552; http://dx.doi.org/10.1073/pnas.0900300106
- Mori I, Sasakura H. Aging: shall we take the high road? Curr Biol 2009; 19:R363-4; PMID:19439256; http://dx.doi.org/10.1016/j.cub.2009.03.040
- Karlsson B, Wiklund C. Butterfly life history and temperature adaptations; dry open habitats select for increased fecundity and longevity. J Anim Ecol 2005; 74:99-104; http://dx.doi.org/10.1111/j.1365-2656.2004.00902.x
- Gillooly JF, Brown JH, West GB, Savage VM, Charnov EL. Effects of size and temperature on metabolic rate. Science 2001; 293:2248-51; PMID:11567137; http://dx.doi.org/10.1126/science.1061967
- Lee SJ, Kenyon C. Regulation of the longevity response to temperature by thermosensory neurons in Caenorhabditis elegans. Curr Biol 2009; 19:715-22; PMID:19375320; http://dx.doi.org/10.1016/j.cub.2009.03.041
- Xiao R, Zhang B, Dong Y, Gong J, Xu T, Liu J, Xu XZ. A genetic program promotes C. elegans longevity at cold temperatures via a thermosensitive TRP channel. Cell 2013; 152:806-17; PMID:23415228; http://dx.doi.org/10.1016/j.cell.2013.01.020
- Mattson MP. Perspective: does brown fat protect against diseases of aging? Ageing Res Rev 2010; 9:69-76; PMID:19969105; http://dx.doi.org/10.1016/j.arr.2009.11.004
- Golozoubova V, Hohtola E, Matthias A, Jacobsson A, Cannon B, Nedergaard J. Only UCP1 can mediate adaptive nonshivering thermogenesis in the cold. FASEB J 2001; 15:2048-50; PMID:11511509
- Tsuboyama-Kasaoka N, Tsunoda N, Maruyama K, Takahashi M, Kim H, Ikemoto S, Ezaki O. Up-regulation of uncoupling protein 3 (UCP3) mRNA by exercise training and down-regulation of UCP3 by denervation in skeletal muscles. Biochem Biophys Res Commun 1998; 247:498-503; PMID:9642158; http://dx.doi.org/10.1006/bbrc.1998.8818
- Valente A, Jamurtas AZ, Koutedakis Y, Flouris AD. Molecular pathways linking non-shivering thermogenesis and obesity: focusing on brown adipose tissue development. Biol Rev Camb Philos Soc 2015; 90(1):77–88; PMID:24708171; http://dx.doi.org/10.1111/brv.12099
- Rousset S, Alves-Guerra MC, Mozo J, Miroux B, Cassard-Doulcier AM, Bouillaud F, Ricquier D. The biology of mitochondrial uncoupling proteins. Diabetes 2004; 53 Suppl 1:S130-5; http://dx.doi.org/10.2337/diabetes.53.2007.S130
- Ducharme NA, Bickel PE. Lipid droplets in lipogenesis and lipolysis. Endocrinology 2008; 149:942-9; PMID:18202123; http://dx.doi.org/10.1210/en.2007-1713
- Kenny GP, Flouris AD. The human thermoregulatory system and its response to thermal stress. Protective Clothing: Managing Thermal Stress. In: Wang F, Gao C, eds. Cambridge, UK: Woodhead Publishing Ltd. in Association with The Textile Institute, 2014:319-49
- Kellogg DL Jr. In vivo mechanisms of cutaneous vasodilation and vasoconstriction in humans during thermoregulatory challenges. J Appl Physiol 2006; 100:1709-18; PMID:16614368; http://dx.doi.org/10.1152/japplphysiol.01071.2005
- Shibasaki M, Wilson TE, Crandall CG. Neural control and mechanisms of eccrine sweating during heat stress and exercise. J Appl Physiol 2006; 100:1692-701; PMID:16614366; http://dx.doi.org/10.1152/japplphysiol.01124.2005
- Flouris AD. Functional architecture of behavioural thermoregulation. Eur J Appl Physiol 2011; 111:1-8; PMID:20711785; http://dx.doi.org/10.1007/s00421-010-1602-8
- Kroetz FW, Leon DF, Leonard JJ. The diagnosis of acute circulatory failure: shock and syncope. Prog Cardiovasc Dis 1967; 10:262-82; PMID:4867786; http://dx.doi.org/10.1016/0033-0620(67)90013-8
- Fox RH, Woodward PM, Exton-Smith AN, Green MF, Donnison DV, Wicks MH. Body temperatures in the elderly: a national study of physiological, social, and environmental conditions. Br Med J 1973; 1:200-6; PMID:4686555; http://dx.doi.org/10.1136/bmj.1.5847.200
- Fox RH, MacGibbon R, Davies L, Woodward PM. Problem of the old and the cold. Br Med J 1973; 1:21-4; PMID:4683633; http://dx.doi.org/10.1136/bmj.1.5844.21
- Kvistad CE, Thomassen L, Waje-Andreassen U, Naess H. Low body temperature associated with severe ischemic stroke within 6 hours of onset: the Bergen NORSTROKE Study. Vasc Health Risk Manag 2012; 8:333-8; PMID:22701327; http://dx.doi.org/10.2147/VHRM.S31614
- Kvistad CE, Oygarden H, Thomassen L, Waje-Andreassen U, Naess H. Persistent middle cerebral artery occlusion associated with lower body temperature on admission. Vasc Health Risk Manag 2013; 9:297-302; PMID:23807851; http://dx.doi.org/10.2147/VHRM.S44570
- Sund-Levander M, Wahren LK. The impact of ADL status, dementia and body mass index on normal body temperature in elderly nursing home residents. Arch Gerontol Geriatr 2002; 35:161-9; PMID:14764354; http://dx.doi.org/10.1016/S0167-4943(02)00019-5
- Rikke BA, Johnson TE. Lower body temperature as a potential mechanism of life extension in homeotherms. Exp Gerontol 2004; 39:927-30; PMID:15217694; http://dx.doi.org/10.1016/j.exger.2004.03.020
- Liu RK, Walford RL. The effect of lowered body temperature on lifespan and immune and non-immune processes. Gerontologia 1972; 18:363-88; PMID:4618218; http://dx.doi.org/10.1159/000211944
- Walsh NP, Whitham M. Exercising in environmental extremes : a greater threat to immune function? Sports Med 2006; 36:941-76; PMID:17052132; http://dx.doi.org/10.2165/00007256-200636110-00003
- Lackovic V, Borecky L, Vigas M, Rovensky J. Activation of NK cells in subjects exposed to mild hyper- or hypothermic load. J Interferon Res 1988; 8:393-402; PMID:2457640; http://dx.doi.org/10.1089/jir.1988.8.393
- Brenner IK, Castellani JW, Gabaree C, Young AJ, Zamecnik J, Shephard RJ, Shek PN. Immune changes in humans during cold exposure: effects of prior heating and exercise. J Appl Physiol 1999; 87:699-710; PMID:10444630
- Cross MC, Radomski MW, VanHelder WP, Rhind SG, Shephard RJ. Endurance exercise with and without a thermal clamp: effects on leukocytes and leukocyte subsets. J Appl Physiol 1996; 81:822-9; PMID:8872652
- Beilin B, Shavit Y, Razumovsky J, Wolloch Y, Zeidel A, Bessler H. Effects of mild perioperative hypothermia on cellular immune responses. Anesthesiology 1998; 89:1133-40; PMID:9822001; http://dx.doi.org/10.1097/00000542-199811000-00013
- Jansky L, Pospisilova D, Honzova S, Ulicny B, Sramek P, Zeman V, Kaminkova J. Immune system of cold-exposed and cold-adapted humans. Eur J Appl Physiol Occup Physiol 1996; 72:445-50; PMID:8925815; http://dx.doi.org/10.1007/BF00242274
- Kim K, Suzuki K, Peake J, Ahn N, Ogawa K, Hong C, Kim S, Lee I, Park J. Physiological and leukocyte subset responses to exercise and cold exposure in cold-acclimatized skaters. Biol Sport 2014; 31:39-48; PMID:24917688; http://dx.doi.org/10.5604/20831862.1086731
- Castellani JW, M Brenner IK, Rhind SG. Cold exposure: human immune responses and intracellular cytokine expression. Med Sci Sports Exerc 2002; 34:2013-20; PMID:12471310; http://dx.doi.org/10.1097/00005768-200212000-00023
- Roberts NJ Jr., Steigbigel RT. Hyperthermia and human leukocyte functions: effects on response of lymphocytes to mitogen and antigen and bactericidal capacity of monocytes and neutrophils. Infect Immun 1977; 18:673-9; PMID:412788
- Matz JM, Blake MJ, Tatelman HM, Lavoi KP, Holbrook NJ. Characterization and regulation of cold-induced heat shock protein expression in mouse brown adipose tissue. Am J Physiol 1995; 269:R38-47; PMID:7631901
- Matz JM, LaVoi KP, Epstein PN, Blake MJ. Thermoregulatory and heat-shock protein response deficits in cold-exposed diabetic mice. Am J Physiol 1996; 270:R525-32; PMID:8780216
- Shephard RJ, Shek PN. Cold exposure and immune function. Can J Physiol Pharmacol 1998; 76:828-36; PMID:10066131; http://dx.doi.org/10.1139/y98-097
- Jaattela M. Overexpression of major heat shock protein hsp70 inhibits tumor necrosis factor-induced activation of phospholipase A2. J Immunol 1993; 151:4286-94; PMID:8409402
- Izawa S, Kim K, Akimoto T, Ahn N, Lee H, Suzuki K. Effects of cold environment exposure and cold acclimatization on exercise-induced salivary cortisol response. Wilderness Environ Med 2009; 20:239-43; PMID:19737029; http://dx.doi.org/10.1580/07-WEME-OR-123R2.1
- Heroux O, Campbell JS. A study of the pathology and life span of 6 degree C- and 30 degree C.-acclimated rats. Lab Invest 1960; 9:305-15; PMID:14401326
- Holloszy JO, Smith EK. Longevity of cold-exposed rats: a reevaluation of the “rate-of-living theory.” J Appl Physiol 1986; 61:1656-60; PMID:3781978
- Flouris AD, Spiropoulos Y, Sakellariou GJ, Koutedakis Y. Effect of seasonal programming on fetal development and longevity: links with environmental temperature. Am J Hum Biol 2009; 21:214-6; PMID:19194861; http://dx.doi.org/10.1002/ajhb.20818
- Doblhammer G, Vaupel JW. Lifespan depends on month of birth. Proc Natl Acad Sci U S A 2001; 98:2934-9; PMID:11226344; http://dx.doi.org/10.1073/pnas.041431898
- Moore SE, Cole TJ, Poskitt EM, Sonko BJ, Whitehead RG, McGregor IA, Prentice AM. Season of birth predicts mortality in rural Gambia. Nature 1997; 388:434; PMID:9242401; http://dx.doi.org/10.1038/41245
- Vaiserman AM, Collinson AC, Koshel NM, Belaja, II, Voitenko VP. Seasonal programming of adult longevity in Ukraine. Int J Biometeorol 2002; 47:49-52; PMID:12461609; http://dx.doi.org/10.1007/s00484-002-0144-0
- Hille ET, Weisglas-Kuperus N, van Goudoever JB, Jacobusse GW, Ens-Dokkum MH, de Groot L, Wit JM, Geven WB, Kok JH, de Kleine MJ, et al. Functional outcomes and participation in young adulthood for very preterm and very low birth weight infants: the Dutch Project on Preterm and Small for Gestational Age Infants at 19 years of age. Pediatrics 2007; 120:e587-95; PMID:17766499; http://dx.doi.org/10.1542/peds.2006-2407
- Carrillo AE, Koutedakis Y, Flouris AD. Early life mammalian biology and later life physical performance: maximising physiological adaptation. Br J Sports Med 2011; 45:1000-1; PMID:21798868; http://dx.doi.org/10.1136/bjsports-2011-090198
- Flouris AD, Carrillo AE. Influence of early life factors on elite performance. J Appl Physiol 2011; 110:284; discussion 94; PMID:21542161
- Julian CG, Wilson MJ, Moore LG. Evolutionary adaptation to high altitude: a view from in utero. Am J Hum Biol 2009; 21:614-22; PMID:19367578; http://dx.doi.org/10.1002/ajhb.20900
- Buchowicz B, Yu T, Nance DM, Zaldivar FP, Cooper DM, Adams GR. Increased rat neonatal activity influences adult cytokine levels and relative muscle mass. Pediatr Res 2010; 68:399-404; PMID:20657345
- Symonds ME, Bird JA, Clarke L, Gate JJ, Lomax MA. Nutrition, temperature and homeostasis during perinatal development. Exp Physiol 1995; 80:907-40; PMID:8962708
- Clarke L, Heasman L, Firth K, Symonds ME. Influence of route of delivery and ambient temperature on thermoregulation in newborn lambs. Am J Physiol 1997; 272:R1931-9; PMID:9227610
- Clarke L, Buss DS, Juniper DT, Lomax MA, Symonds ME. Adipose tissue development during early postnatal life in ewe-reared lambs. Exp Physiol 1997; 82:1015-27; PMID:9413733; http://dx.doi.org/10.1017/S095806709701645X
- Rial E, Zardoya R. Oxidative stress, thermogenesis and evolution of uncoupling proteins. J Biol 2009; 8:58; PMID:19589183; http://dx.doi.org/10.1186/jbiol155
- Kontani Y, Wang Y, Kimura K, Inokuma KI, Saito M, Suzuki-Miura T, Wang Z, Sato Y, Mori N, Yamashita H. UCP1 deficiency increases susceptibility to diet-induced obesity with age. Aging Cell 2005; 4:147-55; PMID:15924571; http://dx.doi.org/10.1111/j.1474-9726.2005.00157.x
- Emre Y, Hurtaud C, Karaca M, Nubel T, Zavala F, Ricquier D. Role of uncoupling protein UCP2 in cell-mediated immunity: how macrophage-mediated insulitis is accelerated in a model of autoimmune diabetes. Proc Natl Acad Sci U S A 2007; 104:19085-90; PMID:18006654; http://dx.doi.org/10.1073/pnas.0709557104
- Sramkova D, Krejbichova S, Vcelak J, Vankova M, Samalikova P, Hill M, Kvasnickova H, Dvorakova K, Vondra K, Hainer V, et al. The UCP1 gene polymorphism A-3826G in relation to DM2 and body composition in Czech population. Exp Clin Endocrinol Diabetes 2007; 115:303-7; PMID:17516293; http://dx.doi.org/10.1055/s-2007-977732
- Eriksson H, Svardsudd K, Larsson B, Welin L, Ohlson LO, Wilhelmsen L. Body temperature in general population samples. The study of men born in 1913 and 1923. Acta Med Scand 1985; 217:347-52; PMID:4013825; http://dx.doi.org/10.1111/j.0954-6820.1985.tb02708.x
- Rising R, Fontvieille AM, Larson DE, Spraul M, Bogardus C, Ravussin E. Racial difference in body core temperature between Pima Indian and Caucasian men. Int J Obes Relat Metab Disord 1995; 19:1-5; PMID:7719384
- Waalen J, Buxbaum JN. Is older colder or colder older? The association of age with body temperature in 18,630 individuals. J Gerontol A Biol Sci Med Sci 2011; 66:487-92; PMID:21324956; http://dx.doi.org/10.1093/gerona/glr001
- Schlitt A, Heine GH, Blankenberg S, Espinola-Klein C, Dopheide JF, Bickel C, Lackner KJ, Iz M, Meyer J, Darius H, et al. CD14+CD16+ monocytes in coronary artery disease and their relationship to serum TNF-α levels. Thromb Haemost 2004; 92:419-24; PMID:15269840
- Vachharajani V, Granger DN. Adipose tissue: a motor for the inflammation associated with obesity. IUBMB Life 2009; 61:424-30; PMID:19319966; http://dx.doi.org/10.1002/iub.169
- Hansen JC, Gilman AP, Odland JO. Is thermogenesis a significant causal factor in preventing the "globesity" epidemic? Med Hypotheses 2010; 75:250-6; PMID:20363565; http://dx.doi.org/10.1016/j.mehy.2010.02.033
- Koutedakis Y, Bouziotas C, Flouris AD, Nelson PN. Longitudinal modeling of adiposity in periadolescent Greek schoolchildren. Med Sci Sports Exerc 2005; 37:2070-4; PMID:16331131; http://dx.doi.org/10.1249/01.mss.0000178099.80388.15
- Douketis JD, Sharma AM. Obesity and cardiovascular disease: pathogenic mechanisms and potential benefits of weight reduction. Semin Vasc Med 2005; 5:25-33; PMID:15968577; http://dx.doi.org/10.1055/s-2005-871739
- Duffy PH, Feuers RJ, Hart RW. Effect of chronic caloric restriction on the circadian regulation of physiological and behavioral variables in old male B6C3F1 mice. Chronobiol Int 1990; 7:291-303; PMID:2085870; http://dx.doi.org/10.3109/07420529009064635
- Lane MA, Baer DJ, Rumpler WV, Weindruch R, Ingram DK, Tilmont EM, Cutler RG, Roth GS. Calorie restriction lowers body temperature in rhesus monkeys, consistent with a postulated anti-aging mechanism in rodents. Proc Natl Acad Sci U S A 1996; 93:4159-64; PMID:8633033; http://dx.doi.org/10.1073/pnas.93.9.4159
- Seebacher F. Responses to temperature variation: integration of thermoregulation and metabolism in vertebrates. J Exp Biol 2009; 212:2885-91; PMID:19717669; http://dx.doi.org/10.1242/jeb.024430
- Angilletta J, Michael J, Sears MW, Winters RS. Seasonal variation in reproductive effort and its effect on offspring size in the lizard Sceloporus undulatus. Herpetologica 2001; 57:365-75
- Stevenson RD. The relative importance of behavioral and physiological adjustments controlling body temperature in terrestrial ectotherms. Am Nat 1985; 126:362-86; http://dx.doi.org/10.1086/284423
- Patapoutian A, Peier AM, Story GM, Viswanath V. ThermoTRP channels and beyond: mechanisms of temperature sensation. Nat Rev Neurosci 2003; 4:529-39; PMID:12838328; http://dx.doi.org/10.1038/nrn1141
- Seebacher F, Brand MD, Else PL, Guderley H, Hulbert AJ, Moyes CD. Plasticity of oxidative metabolism in variable climates: molecular mechanisms. Physiol Biochem Zool 2010; 83:721-32; PMID:20586603; http://dx.doi.org/10.1086/649964
- Feder ME, Hofmann GE. Heat-shock proteins, molecular chaperones, and the stress response: evolutionary and ecological physiology. Annu Rev Physiol 1999; 61:243-82; PMID:10099689; http://dx.doi.org/10.1146/annurev.physiol.61.1.243
- Bouchard P, Guderley H. Time course of the response of mitochondria from oxidative muscle during thermal acclimation of rainbow trout, Onchorhynchus mykiss. J Exp Biol 2003; 206:3455-65; PMID:12939376; http://dx.doi.org/10.1242/jeb.00578
- Rogers K, Seebacher F, Thompson MB. Biochemical acclimation of metabolic enzymes in response to lowered temperatures in tadpoles of Limnodynastes peronii. Comp Biochem Physiol A 2004; 137:731-8; http://dx.doi.org/10.1016/j.cbpb.2004.02.008
- Angilletta J, J. M. Thermal Adaptation. A Theoretical and Empirical Synthesis. Oxford: Oxford University Press, 2009
- Tomanek L, Somero GN. Evolutionary and acclimation induced variation in the heat-shock responses of congeneric marine snails (genus Tegula) from different thermal habitats: implications for limits of thermotolerance and biogeography. J Exp Biol 1999; 202:2925-36; PMID:10518474
- Wakeling JM, Cole NJ, Kemp KM, Johnston IA. The biomechanics and evolutionary significance of thermal acclimation in the common carp Cyprinus carpio. Am J Physiol Regul Integr Comp Physiol 2000; 279:657-65
- Narum SR, Campbell RM, Meyer KA, Miller MR, Hardy RW. Thermal adaptation and acclimation of ectotherms from differing aquatic climates. Mol Ecol 2013; 22(11):3090-7
- Seebacher F, Franklin CE. Prostaglandins are important in thermoregulation of a lizard (Pogona vitticeps). Proc R Soc Lond B Biol Sci Suppl 2003; 270:S50-S3; http://dx.doi.org/10.1098/rsbl.2003.0007
- Bogert CM. Thermoregulation in reptiles, a factor in evolution. Evolution 1949; 3:195-211; PMID:18138377; http://dx.doi.org/10.2307/2405558
- Adolph SC, Porter WP. Temperature, activity, and lizard life histories. Am Nat 1993; 142:273-95; PMID:19425979; http://dx.doi.org/10.1086/285538
- Brattstrom BH. Amphibian temperature regulation studies in the field and laboratory. Am Zool 1979; 19: 345-56
- Vitt LJ, Janalee P. Caldwell. Herpetology. An introductiory biology of amphibians and reptiles. London, UK: Academic Press, Elsevier, 2009
- Grant BW, Dunham AE. Elevational covariation in environmental constraints and life histories of the desert lizard Sceloporus. Ecology 1990; 71:1765-76; http://dx.doi.org/10.2307/1937584
- Porter WP, Mitchell JW, Beckman WA, DeWitt CB. Behavioral implications of mechanistic ecology. Thermal and behavioral modeling of desert ectotherms and their microenvironment. Oecologia 1973; 13:1-54; http://dx.doi.org/10.1007/BF00379617
- Huey RB. Behavioral thermoregulation in lizards: importance of associated costs. Science 1974; 184:1001-3; PMID:4826166; http://dx.doi.org/10.1126/science.184.4140.1001
- Huey RB. Winter thermal ecology of the Iguanid lizard Tropidurus peruvianus. Copeia 1974:149-55; http://dx.doi.org/10.2307/1443017
- Porter WP, Tracy R. Biophysical analyses of energetics, time-space utilization, and distributional limits. Lizard ecology: Studies of a model organism. In: Huey RB, Pianka ER, Schoener TW, eds. Cambridge, Massachusetts, and London, England: Harvard University Press, 1983:55-83
- Sinervo B, Adolph SC. Thermal sensitivity of growth-rate in hatchling Sceloporus lizards - Environmental, behavioral and genetic-aspects. Oecologia 1989; 78:411-9; http://dx.doi.org/10.1007/BF00379118
- Dunham AE, Miles DB, Reznick DN. Life History patterns in squamate reptiles. Biology of the Reptilia. In: Huey CGaRB, ed. New York: Alan R. Liss, 1988:441-522
- Van Damme R, Bauwens D, Verheyen RF. Selected body temperatures in lizard Lacerta vivipara: variation within and between populations. J Therm Biol 1986; 11:219-22; http://dx.doi.org/10.1016/0306-4565(86)90006-9
- Bauwens D, Verheyen RF. Variation of reproductive traits in a population of the lizard Lacerta vivipara. Holar Ecol 1987; 10:120-7
- Sorci G, Clobert J, Belichon S. Phenotypic plasticity of growth and survival in the common lizard Lacerta vivipara. J Ani Ecol 1996; 65:781-90; http://dx.doi.org/10.2307/5676
- Miquel J, Lundgren PR, Bensch KG, Atlan H. Effects of temperature on the life span, vitality and fine structure of Drosophila melanogaster. Mech Ageing Dev 1976; 5:347-70; PMID:823384; http://dx.doi.org/10.1016/0047-6374(76)90034-8
- Van Voorhies WA, Ward S. Genetic and environmental conditions that increase longevity in Caenorhabditis elegans decrease metabolic rate. Proc Natl Acad Sci U S A 1999; 96:11399-403; PMID:10500188; http://dx.doi.org/10.1073/pnas.96.20.11399
- Ray C. The application of Bergmann's and Allen's Rules to the poikilotherms. J Morphol 1960; 106:85-108; PMID:14436612; http://dx.doi.org/10.1002/jmor.1051060104
- Stearns SC, Koella JC. The evolution of phenotypic plasticity in life-history traits: predictions of reaction norms for age and size at maturity. Int J Org Evolution 1986; 40:893-913
- Perrin N. Why are offspring born larger when it is colder? Phenotypic plasticity for offspring size in the cladoceran Simocephalus vetulus (Müller). Funct Ecol 1988; 2:283-8; http://dx.doi.org/10.2307/2389399
- Yampolsky LY, Scheiner SM. Why larger offspring at lower temperatures? A Demographic Approach The Am Naturalist 1996; 147:86-100; http://dx.doi.org/10.1086/285841
- Pianka ER. Comparative autecology of the lizard Cnemidophorus tigris in different parts of its geographic range. Ecology 1970; 51:703-20; http://dx.doi.org/10.2307/1934053
- Pianka ER, Pianka HD. The ecology of Moloch horridus (Lacertilia: Agamidae) in Western Australia. Copeia 1970; 1970:90-103; http://dx.doi.org/10.2307/1441978
- Tinkle DW. The role of environment in the evolution of life history differences within and between lizard species. A symposium on Ecosystematics, In: Allen RT, James FC, eds. 1972:77-100
- Ballinger RE. Life-history variations. Lizard ecology: Studies of a model organism. In: Huey RB, Eric R. Pianka, and Thomas W. Schoener, ed. Cambridge, Massachusetts, and London, England: Harvard University Press, 1983:241-60
- Sinervo B. The evolution of maternal investment in lizards: an experimental and comparative analysis of egg size and its effects on offspring performance. Evolution 1990; 44:279-94; http://dx.doi.org/10.2307/2409407
- Avery RA. Physiological aspects of Iizard growth: the role of thermoregulation. Symp Zool Soc Land 1984; 52:407-24
- Ferguson GW, Talent LG. Life-history traits of the lizard Sceloporus undulatus from two populations raised in a common laboratory environment. Oecologia 1993; 93:88-94
- Sacher GA. Longevity and ageing in vertebrate evolution. BioScience 1978; 28:497-501; http://dx.doi.org/10.2307/1307295
- Castanet J. Age estimation and longevity in reptiles. Gerontology 1994; 40:174-92; PMID:7926855; http://dx.doi.org/10.1159/000213586
- Steams SC. Life history tactics: a review of the ideas. Q Rev Biol 1976; 51:3-47; PMID:778893; http://dx.doi.org/10.1086/409052
- Castanet J, Naulleau G. La squelettochronologie chez les Reptiles II. Resultats experimentaux sur la signification des marques de croissance squelettiques chez les Serpents. Remarques sur la croissance et la longevite de la Vipere Aspic. (I). Annales des Sciences Naturelles, Zoologie, Paris - 13c Serie 1985; 7:41-62
- Speakman JR. Body size, energy metabolism and lifespan. J Exp Biol 2005; 208:1717-30; PMID:15855403; http://dx.doi.org/10.1242/jeb.01556
- Glaser FT, Stanewsky R. Temperature synchronization of the Drosophila circadian clock. Curr Biol 2005; 15:1352-63; PMID:16085487; http://dx.doi.org/10.1016/j.cub.2005.06.056
- Boothroyd CE, Wijnen H, Naef F, Saez L, Young MW. Integration of light and temperature in the regulation of circadian gene expression in Drosophila. PLoS Genet 2007; 3:e54; PMID:17411344
- Bauwens D, Garland Jr. T, Castilla AM, van Damme R. Evolution of sprint speed in lacertid lizards: Morphological, physiological, and behavioral covariation. Evolution 1995; 49:848-63; http://dx.doi.org/10.2307/2410408
- Hertz PE, Huey RB, Stevenson RD. Evaluating temperature regulation by field-active ectotherms: the fallacy of the inappropriate question. Am Nat 1993; 142:796-818; PMID:19425957; http://dx.doi.org/10.1086/285573
- Brattstrom BH. Body temperatures of reptiles. Am Midl Nat 1965; 73:376-422; http://dx.doi.org/10.2307/2423461
- Heath JE. Behavioral thermoregulation of body temperature in poikilotherms. Physiologist 1970; 13:399-410; PMID:4920512
- Licht P, Dawson WP, Shoemaker VH, Main AR. Observations on the thermal relations of western Australian lizards. Copeia 1966; 1966:97-110; http://dx.doi.org/10.2307/1440766
- Huey RE. Temperature, physiology, and the ecology of reptiles. Biology of the Reptilia Physiology C In: Gans C, Pough HF, eds. London: Academic Press, 1982:25-91
- Cabanac M, Hammel HT, Hardy JD. Tiliqua scincoides. Temperature sensitive units in lizard brain. Science 1967; 158:1050-1; PMID:6054482; http://dx.doi.org/10.1126/science.158.3804.1050
- Hertz PE. Adaptation to altitude in two West Indian anoles (Reptilia: Iguanidae): field thermal biology and physiological ecology. J Zool, London 1981; 195:25-37; http://dx.doi.org/10.1111/j.1469-7998.1981.tb01891.x
- Hertz PE, Huey RB. Compensation for altitudinal changes in the thermal environment by some Anolis lizards on Hispaniola. Ecology 1981; 62:515-21; http://dx.doi.org/10.2307/1937714
- Christian KA, Tracy CR, Porter WP. Seasonal shifts in body temperature and use of microhabitats by Galapagos land iguanas (Conolophus pallidus). Ecology 1983; 64:463-8; http://dx.doi.org/10.2307/1939965
- Adolph SC. Influence of behavioral thermoregulation on microhabitat use by two Sceloporus lizards. Ecology 1990; 71:315-27; http://dx.doi.org/10.2307/1940271
- Bauwens D, Hertz PE, Castilla AM. Thermoregulation in a lacertid lizard: the relative contributions of distinct behavioral mechanisms. Ecology 1996; 77:1818-30; http://dx.doi.org/10.2307/2265786
- Webb JK, Shine R. Using thermal ecology to predict retreat-site selection by an endangered snake species. Biol Conserv 1998; 86:233-42; http://dx.doi.org/10.1016/S0006-3207(97)00180-8
- Huey RB, Peterson CR, Arnold SJ, Porter WP. Hot rocks and not-so-hot rocks: Retreat-site selection by garter snakes and its thermal consequences. Ecology 1989; 70:931-44; http://dx.doi.org/10.2307/1941360
- Kearney M, Predavec M. Do nocturnal ectotherms thermoregulate? A study of the temperate gecko Christinus marmoratus. Ecology 2000; 81:2984-96 ; http://dx.doi.org/10.1890/0012-9658(2000)081%5b2984:DNETAS%5d2.0.CO;2
- Kearney M. Hot rocks and much-too-hot rocks: seasonal patterns of retreat-site selection by a nocturnal ectotherm. J Ther Biol 2002; 27 205-18; http://dx.doi.org/10.1016/S0306-4565(01)00085-7
- Schmidt-Nielsen K, Dawson WR. Terrestrial animals in dry heat: desert reptiles. Handbook of Physiology Section 4: Adaptation to the Environment. In: Field J, ed. Washington, DC: American Physiology Society, 1964:467-80
- Heatwole H, Lin TH, Villalón E, Muñíz A, Matta A. Some aspects of the thermal ecology of Puerto Rican Anoline lizards. J Herpetol 1969; 3:65-77; http://dx.doi.org/10.2307/1563225
- Huey RB, Pianka ER. Seasonal variation in thermoregulatory behavior and body temperature of diurnal Kalahari lizards. Ecology 1977; 58:1066-75; http://dx.doi.org/10.2307/1936926
- Carrascal LM, Díaz JA. Thermal ecology and spatio-temporal distribution of the Mediterranean lizard Psammodromus algirus. Holarctic Ecol 1989; 12:137-43
- Pearson OP. Habits of the lizard Liolaemus multiformis multiformis at high altitudes in southern Peru. Copeia 1954; 1954:111-6; http://dx.doi.org/10.2307/1440329
- Van Damme R, Bauwens D, Castilla AM, Verheyen RF. Altitudinal variation of the thermal biology and running performance in the lizard Podarcis tiliguerta. Oecologia 1989; 80:516-24; http://dx.doi.org/10.1007/BF00380076
- Díaz JA, Cabezas-Días S. Seasonal variation in the contribution of different behavioural mechanisms to lizard thermoregulation. Funct Ecol 2004; 18:867-75; http://dx.doi.org/10.1111/j.0269-8463.2004.00916.x
- Martín J, López P, Carrascal LM, Salvador A. Adjustment of basking postures in the high-altitude Iberian rock lizard (Lacerta monticola). Can J Zool 1995; 73:1065-8; http://dx.doi.org/10.1139/z95-126
- Heath JE. Temperature regulation and diurnal activity in horned lizards. Univ Calif Publ Zool 1965; 64:97-136
- Hammel HT, Caldwell FT, Jr., Abrams RM. Regulation of body temperature in the blue-tongued lizard. Science 1967; 156:1260-2; PMID:6025552; http://dx.doi.org/10.1126/science.156.3779.1260
- Spellerberg IF. Thermal ecology of allopatric lizards (Sphenomorphus) in Southeast Australia. I. The Environ Lizard Critical Temperatures Oecologia 1972; 9:371-83
- Autumn K, De Nardo DF. Behavioral thermoregulation increases growth rate in a nocturnal lizard. J Herpetol 1995; 29:157-62; http://dx.doi.org/10.2307/1564552
- Angilletta MJ Jr., Werner YL. Australian geckos do not display diel variation in thermoregulatory behavior. Copeia 1998; 1998:736-42; http://dx.doi.org/10.2307/1447806
- Piantoni C, Ibargüengoytía NR, Cussac VE. Growth and age of the southernmost distributed gecko of the world (Homonota darwini) studied by skeletochronology. Amphibia-Reptilia 2006; 27:393-400; http://dx.doi.org/10.1163/156853806778190060
- Parker WS, Pianka ER. Further ecological observations on the western banded gecko, Coleonyx variegatus. Copeia 1973; 1974:528-31; http://dx.doi.org/10.2307/1442544
- Huey RB, Webster TP. Thermal biology of a solitary lizard: Anolis marmoratus of Guadeloupe, Lesser antilles. Ecology 1975; 56:445-52; http://dx.doi.org/10.2307/1934975
- Huey RB, Slatkin M. Cost and benefits of lizard thermoregulation. Q Rev Biol 1976; 51:363-84; PMID:981504; http://dx.doi.org/10.1086/409470
- Dawson WR. On the physiological significance of the preferred body temperatures of reptiles. Perspectives of biophysical ecology. In: Gates DM, Schmerl RB, ed. New York: Springer, 1975
- Bartholomew GA. The role of physiology in the distribution of terrestrial vertebrates. Zoogeography. In: CL H, ed. Washington, DC: AAAS, 1958:81-95
- Huey RB, Stevenson RD. Integrating thermal physiology and ecology of ectotherms: A discussion of approaches. Am Zool 1979; 19:357-66
- Cowles RB, Bogert CM. A preliminary study of the thermal requirements of desert reptiles. Bull Am Museum Nat His 1944; 83:261-96
- Seebacher F, Franklin CE. Physiological mechanisms of thermoregulation in reptiles: a review. J Comp Physiol B 2005; 175:533-41; PMID:16047177; http://dx.doi.org/10.1007/s00360-005-0007-1
- Seebacher F, Murray SA. Transient receptor potential ion channels control thermoregulatory behaviour in reptiles. PLoS One 2007; 2:e281; PMID:17356692; http://dx.doi.org/10.1371/journal.pone.0000281
- Galli GLJ, Skovgaard N, Abe AS, Taylor EW, Wang T. The adrenergic regulation of the cardiovascular system in the South American rattlesnake, Crotalis durissus. Comp Biochem Physiol A 2007; 148:510-20; http://dx.doi.org/10.1016/j.cbpa.2007.06.420
- Ganslosser U. Temperature, physiology, and the ecology of reptiles. Encyclopedia of Ecology. In: Jorgensen SE, Fath BD, eds. London: Elsevier, 2008:3550-7
- Hochscheid S, Bentivegna F, Speakman JR. Regional blood flow in sea turtles: Implications for heat exchange in an aquatic ectotherm. Physiol Biochem Zool 2002; 75:66-76; PMID:11880979; http://dx.doi.org/10.1086/339050
- Seebacher F, Franklin CE. Integration of autonomic and local mechanisms in regulating cardiovascular responses to heating and cooling in a reptile (Crocodylus porosus). J Comp Physiol B 2004; 174:205-10; PMID:14722721; http://dx.doi.org/10.1007/s00360-003-0331-2
- Johnston IA, Calvo J, Guderley H, Fernandez D, Palmer L. Latitudinal variation in the abundance and oxidative capacities of muscle mitochondria in perciform fishes. J Exp Biol 1998; 201:1-12; PMID:9390931
- Fangue NA, Richards JG, Schulte PM. Do mitochondrial properties explain intraspecific variation in thermal tolerance? J Exp Biol 2009; 212:514-22; PMID:19181899; http://dx.doi.org/10.1242/jeb.024034
- Wodke E. Effects of acclimation temperature on oxidative metabolism of eel (Anguilla anguilla). One. Liver and red muscle - changes in mitochondrial content and in oxidative capacity of isolated coupled mitochondria. J Comp Physiol B 1974; 91:309-32; http://dx.doi.org/10.1007/BF00698060
- Lucassen M, Schmidt A, Eckerle LG, Pörtner HO. Mitochondrial proliferation in the permanent vs. temporary cold: enzyme activities and mRNA levels in Antarctic and temperate zoarcid fish. Am J Physiol 2003; 285:R1410-R20
- St-Pierre J, Charest P, Guderley H. Relative contribution of quantitative and qualitative changes in mitochondria to metabolic compensation during seasonal acclimatization of rainbow trout Oncorhynchus mykiss. J Exp Biol 1998; 201:2961-70
- Guderley H, St-Pierre J. Seasonal cycles of mitochondrial ADP sensitivity and oxidative capacities in trout oxidative muscle. J Comp Physiol B 1999; 169:474-80; PMID:10595316; http://dx.doi.org/10.1007/s003600050244
- Firth BT, Turner SJ. Sensory, neural, and hormonal aspects of thermoregulation. Biology of the Reptilia Physiology D. In: Gans C, Pough HF, eds. London: Academic Press, 1982:229-74
- Greenberg GN, Wingfield JC. Stress and reproduction: reciprocal relationships. Hormones and Reproduction in Fishes, Amphibians, and Reptiles. In: Norris DO, Jones RE, eds. New York: Plenum, 1987:461-503
- Astheimer LB, Buttemer WA, Wingfield JC. Gender and seasonal differences in the adrenocortical response to ACTH challenge in an Arctic passerine, Zonotrichia leucophrys gambelii. Gen Comp Endocrinol 1994; 94:33-43; PMID:8045366; http://dx.doi.org/10.1006/gcen.1994.1057
- Guillette LJ Jr., Cree A, Rooney AA. Biology of stress: interactions with reproduction, immunology and intermediary metabolism. Health and Welfare of Captive Reptiles. In: Warwick C, Frye FL, Murphy JB, eds. London: Chapman and Hall, 1995:32-81
- Girling JE, Cree A. Plasma corticosterone levels are not significantly related to reproductive stage in female common geckos (Hoplodactylus maculatus). Gen Comp Endocrinol 1995; 100:273-81; PMID:8775054; http://dx.doi.org/10.1006/gcen.1995.1158
- Cree A, Tyrrell CL, Preest MR, Thorburn D, Guillette J. Protecting embryos from stress: corticosterone effects and the corticosterone response to capture and confinement during pregnancy in a live-bearing lizard (Hoplodactylus maculatus). Gen Comp Endocrinol 2003; 134:316-29; PMID:14636639; http://dx.doi.org/10.1016/S0016-6480(03)00282-X
- Jessop TS, Hamann M, Read MA, Limpus CJ. Evidence for a hormonal tactic maximizing green turtle reproduction in response to a pervasive ecological stressor. Gen Comp Endocrinol 2000; 118:407-17; PMID:10843792; http://dx.doi.org/10.1006/gcen.2000.7473
- Tyrrell CL, Cree A. Relationships between corticosterone concentration and season, time of day and confinement in a wild reptile (Tuatara, Sphenodon punctatus). Gen Comp Endocrinol 1998; 11:97-108; http://dx.doi.org/10.1006/gcen.1997.7051
- Woodly SK, Painter DL, Moore MC, Wikelski M, Romero LM. Effect of tidal cycle and food intake on the bseline plasma corticosterone rhytm in intertidally oforaging marine iguanas. Gen Comp Endocrinol 2003; 132:216-22; PMID:12812768; http://dx.doi.org/10.1016/S0016-6480(03)00085-6
- Preest M, Cree AT. Hermoregulatory behavior and raises metabolic rate in the New Zealand common Gecko, Hoplodactylus maculatus. Physiol Biochem Zool 2008; 81:641-50; PMID:18752421; http://dx.doi.org/10.1086/590371
- Angilletta J, Michael J, Todd D. Steury and Michael W. Sears. Temperature, growth rate, and body size in ectotherms: Fitting pieces of a life-history puzzle. Integr Comp Biol 2004; 44:498-509; PMID:21676736; http://dx.doi.org/10.1093/icb/44.6.498
- Baccini M, Biggeri A, Accetta G, Kosatsky T, Katsouyanni K, Analitis A, Anderson HR, Bisanti L, D'Ippoliti D, Danova J, et al. Heat effects on mortality in 15 European cities. Epidemiology 2008; 19:711-9; PMID:18520615; http://dx.doi.org/10.1097/EDE.0b013e318176bfcd
- Morabito M, Crisci A, Moriondo M, Profili F, Francesconi P, Trombi G, Bindi M, Gensini GF, Orlandini S. Air temperature-related human health outcomes: current impact and estimations of future risks in Central Italy. Sci Total Environ 2012; 441:28-40; PMID:23134767; http://dx.doi.org/10.1016/j.scitotenv.2012.09.056
- Montero JC, Miron IJ, Criado-Alvarez JJ, Linares C, Diaz J. Influence of local factors in the relationship between mortality and heat waves: Castile-La Mancha (1975-2003). Sci Total Environ 2012; 414:73-80; PMID:22154213; http://dx.doi.org/10.1016/j.scitotenv.2011.10.009
- Ballester J, Robine JM, Herrmann FR, Rodo X. Long-term projections and acclimatization scenarios of temperature-related mortality in Europe. Nat Commun 2011; 2:358; PMID:21694706; http://dx.doi.org/10.1038/ncomms1360
- Flouris AD, Cheung SS, Fowles JR, Kruisselbrink LD, Westwood DA, Carrillo AE, Murphy RJ. Influence of body heat content on hand function during prolonged cold exposures. J Appl Physiol 2006; 101:802-8; PMID:16709657; http://dx.doi.org/10.1152/japplphysiol.00197.2006
- Flouris AD, Westwood DA, Cheung SS. Thermal balance effects on vigilance during 2-hour exposures to −20°C. Aviat Space Environ Med 2007; 78:679-79
- Flouris AD, Cheung SS. Human conscious response to thermal input is adjusted to changes in mean body temperature. Br J Sports Med 2008; 43:199-203; PMID:18216157; http://dx.doi.org/10.1136/bjsm.2007.044552
- Cheung S, Flouris AD. Maximal oxygen uptake regulation as a behavioral mechanism. J Appl Physiol 2009; 106:345; PMID:19202592