Abstract
In tumors, cross talk between malignant and non-malignant cells (stroma) influences tumor growth, angiogenesis and metastasis. Stromal cells in tumors typically include vascular cells, fibroblasts and a heterogeneous population of inflammatory cells. Adipocytes may also be present. Adipose tissue is perhaps the least studied stromal cell “compartment” despite the fact that some tumors, particularly breast tumors, grow in close proximity to or physically interact with adipocytes. Apart from adipocytes and numerous blood vessels, adipose tissue harbors macrophages, which increase in proportion to adipose tissue mass. While circulating or bone marrow-derived macrophages play a well-defined role in tumor growth, it is less understood how resident adipose tissue-associated macrophages contribute to tumor progression. Here, we will review the role of adipose tissue in tumor growth and angiogenesis with emphasis on the specific functions of adipose tissue macrophages in these processes.
Introduction
Stromal cells in tumors including fibroblasts, inflammatory cells and vascular cells are well-studied for their role in tumor growth.Citation1 On the other hand, little attention has been given to the role of adipose tissue (AT) in the development or progression of solid tumors. The putative causal relationship between excess adiposity (obesity) and cancer has ignited interest in the relationship between AT and cancer. Epidemiological studies sponsored by The National Cancer Institute have revealed that 34,000 new cases of cancer in men and 50,500 in women could be linked to obesity. These cancers are of diverse origin and include the breast, prostate, kidney, thyroid, colon, rectum, endometrium, esophagus and gallbladder.Citation2
Adipocytes secrete numerous growth factors, hormones and other bioactive molecules that can directly influence the growth of cancer cells. For example, adipocytes produce estrogen, which may stimulate the growth of breast and endometrial cancers.Citation3 The satiety hormone leptin, also produced by adipocytes, acts as a mitogenic factor for colon, ovarian, breast and prostate cancer cells.Citation4-Citation6 In contrast, adipocyte-derived adiponectin, which regulates fatty acid oxidation, inhibits breast cancer cell proliferation.Citation7 Adipocytes may also fuel tumor growth by providing a source for free fatty acids (FFAs). The β-oxidation of FFAs may offer an alternative to glycolysis and fulfill the energy requirements of rapidly proliferating malignant cells.Citation8 Moreover, FFAs might be used for the generation of building blocks incorporated into newly synthesized membranes or converted into lipid-based signaling mediators.Citation8
Other than the direct effects of adipocyte-derived factors on tumor growth, subacute inflammation associated with increased adiposity may create a permissive microenvironment for the growth of cancer cells. Associations between cancer and inflammation are now well-established.Citation9,Citation10 Inflammatory cells may stimulate the growth of cancer cells directly, or influence tumor angiogenesis by providing soluble growth and survival factors, matrix remodeling enzymes and other bioactive molecules that promote the growth and migration of vascular endothelial cells. For example, macrophages secrete many proangiogenic factors including vascular endothelial growth factor (VEGF), tumor necrosis factor α (TNF-α), granulocyte macrophage colony-stimulating factor (GM-CSF) and interleukins-1 and -6 (IL-1 and IL-6).Citation11
In this mini-review, we will briefly synthesize what is known about the role of adipocytes and tumor progression. We will focus on the role of AT macrophages and mechanistic pathways involved in macrophage mobilization and polarization. We will explore how adipocytes, frequently overlooked as a central player in the tumor microenvironment, could play a prevailing role in driving processes related to tumor-associated inflammation and stimulation of tumor angiogenesis.
Adipose Tissue
AT is located beneath the skin (subcutaneous AT), surrounding internal organs (visceral AT) and within the bone marrow. There are two types of AT: white adipose tissue (WAT) and brown adipose tissue (BAT). Whereas WAT is used for energy storage in the form of lipids, BAT is highly specialized for nonshivering thermogenesis due to high mitochondrial content and expression of the mitochondrial uncoupling protein-1 (UCP-1). Interestingly, recent data indicate existence of “beige” adipocytes possessing features of both white and brown AT and a distinct gene expression signature.Citation12
Apart from its energy storage functions, AT is an important endocrine and immunologically active “organ.” For example, AT secretes leptin, adiponectin, resistin, visfatin, TNF-α, IL-6, monocyte chemoattractant protein (MCP-1) [also referred to as chemokine (C-C motif) ligand 2 (CCL2)], plasminogen activator inhibitor-1 (PAI-1) and many other factors.Citation13 Whereas leptin and adiponectin are primarily produced by adipocytes, resistin, visfatin, TNF-α, IL-6, MCP-1 and PAI-1 are expressed by both adipocytes and AT-resident immune cells, in particular macrophages.Citation14
In addition to adipocytes, AT harbors the stromal vascular fraction (SVF) composed of preadipocytes, fibroblasts, vascular endothelial cells and a heterogeneous population of immune cells. Among the AT-associated immune cells are mast cells, eosinophils, B cells, T cells and macrophages. Macrophages comprise the largest group of immune cells within AT and they are uniquely activated or polarized in obese AT depots.Citation15 In addition to clearance of dead or dying adipocytes, AT macrophages (ATMs) regulate matrix remodeling, angiogenesis and proliferation or differentiation of adipocyte precursors. In lean subjects, macrophages maintain adipocyte insulin sensitivity by secreting IL-10 which inhibits inflammatory mediators such as TNF-α.Citation15 In obesity, however, impaired cross talk between adipocytes and macrophages plays an important role in the pathophysiology of numerous co-morbidities, for example, insulin resistance.Citation16 Pro-inflammatory macrophages block insulin action in adipocytes by downregulating expression of the glucose transporter type-4 (GLUT4).Citation17 Notably, a compensatory rise in insulin release may be associated with cancers of the breast, colon, endometrium, kidney and pancreas.Citation3 This may be due to the well-known ability of insulin to induce the intracellular PI3K/Akt signaling pathway involved in cell proliferation and survival.Citation18
Obesity and Adipose Tissue Macrophages
Macrophage infiltration is a common feature in inflamed or obese AT. In obese mice, macrophage numbers increase 3- to 5-fold compared with lean mice and can comprise up to 50% of all cells within a visceral AT depot.Citation19 The same is true in humans where a 3-fold increase in the number of ATMs has been observed in AT from obese subjects.Citation19 The cellular and molecular mechanisms leading to macrophage recruitment in AT of obese subjects are becoming clear (for an excellent review, see Dalmas et al.).Citation20 For example, adipocyte hypertrophy causes adipocyte dysfunction and FFA release. FFAs serve as ligands for the Toll-like receptor-4 (TLR-4) expressed by macrophages and adipocytes.Citation21 Macrophages might therefore be mobilized in order to scavenge released FFAs. Indeed, it has been shown that deficiency of myeloid TLR-4 can result in reduced macrophage infiltration.Citation22
In obese subjects, adipocytes increase in size by hypertrophy. Adipocyte hypertrophy can lead to adipocyte death, which may be an early stimulus for macrophage recruitment even though the cause of adipocyte death is not entirely clear.Citation21 One possibility is that hypertrophy causes rupture of the fragile adipocyte membrane leading to spillage of lipids.Citation23 Macrophages are then recruited to scavenge dead or dying cells and free lipid droplets. In good accord with this possibility, approximately 90% of all macrophages found in obese AT are localized to sites of necrotic-like death.Citation24 Crown-like structures (CLS) consisting of single or fused macrophages (i.e., multi-nucleated giant cells) may form in these necrotic areas. CLS are associated with obesity and are a signature of adipocyte dysfunction and a chronic inflammatory reaction.Citation24 Furthermore, hypoxia associated with necrotic areas within AT may directly stimulate adipocytes to upregulate inflammatory mediators including macrophage inhibitory factor (MIF), matrix metalloproteinases (MMP-2 and MMP-9), IL-6, Angplt4, PAI-1, VEGF and leptin.Citation25
Prompted by the observation that monocytes/macrophages express the leptin receptor, Gruen et al. demonstrated that leptin acts as a potent chemoattractant for monocytes/macrophages in vitro.Citation26 Moreover, Lepob/ob and LepRdb/db mice, when compared with models of diet-induced obesity, had decreased numbers of macrophages.Citation21 In contrast, a recent study by Gutierrez and HastyCitation27 demonstrated that hematopoietic deficiency of the leptin receptor did not affect macrophage accumulation in AT. It is possible that leptin affects macrophage recruitment into AT indirectly by upregulating endothelial cell adhesion molecules. For example, adipocyte-conditioned medium, as well as recombinant human leptin, increased platelet endothelial cell adhesion molecule (PECAM-1) and intercellular adhesion molecule (ICAM-1) expression by human AT endothelial cells which in turn increased chemotaxis of blood monocytes.Citation28 This paradigm is supported by the observed increase in AT expression of ICAM-1 within three weeks of high-fat feeding in mice.Citation29
Chemokines also regulate macrophage recruitment to AT, particularly in inflamed AT associated with obesity. For example the level of MCP-1 produced by AT is increased during obesity; however, it is still controversial whether MCP-1 and its receptor CCR2 play significant role in macrophage recruitment into AT.Citation21 Whereas MCP-1 overexpression, under control of the adipocyte P2 (aP2) promoter increases the number of macrophages within AT and leads to insulin resistance and glucose intolerance,Citation30 experiments with MCP-1−/− and CCR2−/− mice yielded inconsistent results.Citation21
M1 vs. M2 Macrophage Polarization
Obesity leads to an increase in macrophage numbers and phenotypic/morphological changes in ATMs. In general, tissue-resident macrophages are relatively quiescent but continuously “sample” the microenvironment through phagocytosis. However, following perturbations in the local microenvironment, macrophages can assume a range of different phenotypes.Citation31 After stimulation with interferon gamma (IFN-γ) alone or in combination with TLR ligands (e.g., LPS), macrophages acquire the M1, classically activated phenotype and secrete pro-inflammatory cytokines (e.g., TNF-α, IL-6 and IL-1β) that contribute to insulin resistance. They also generate reactive oxygen species such as nitric oxide via activation of inducible oxide synthase (iNOS). On the other hand, alternatively activated M2 macrophages, induced by exposure to IL-4 and IL-13, produce arginase that blocks iNOS activity and stimulates production of ornithine, a precursor of hydroxyproline and polyamines. M2 macrophages also secrete anti-inflammatory cytokines including IL-10, TGF-β, IL-1 receptor agonist-a (IL-1Ra) and IL-4.Citation32 Using CD11c expression as an M1 marker, Lumeng et al.Citation33 found that high fat diet-induced obesity in lean mice caused a switch in ATMs from the M2 phenotype to the proinflammatory M1 phenotype. Moreover, conditional bone marrow depletion of CD11c+ cells in obese mice led to normalization of insulin sensitivity indicating a link between inflammation and insulin resistance.Citation34
In addition to attracting macrophages, FFAs released from hypertrophied adipocytes might drive M1 polarization of ATMs. Is has been shown that FFAs induce inflammatory changes in macrophages through NFκB activation in vitro.Citation35 Interestingly, Cani et al. found increased circulating levels of LPS in obese mice.Citation36 A high-fat diet led to significant modulation of dominant bacterial populations within the intestinal microbiota by increasing the number of Gram-negative bacteria following LPS translocation from the gut. Additionally, LPS-infused lean mice displayed a metabolic phenotype similar to one from high-fat fed mice with increased numbers of ATMs, AT inflammation and insulin resistance.Citation36 Decreased adiponectin secretion in obesity might also contribute to M1 polarization of ATMs.Citation37 For example, peritoneal and AT-associated macrophages isolated from adiponectin knockout mice displayed increased M1 and decreased M2 markers. Moreover, adiponectin increased expression of M2 markers in stimulated human macrophages.Citation37
A “phenotypic switch” model proposed by Lumeng et al.Citation33 has been challenged by several studies in which macrophages with mixed M1/M2 phenotypes were found in obese adipose tissue of both mice and human.Citation38,Citation39 For example, human ATMs from obese AT were found to express markers of M2 macrophages including the mannose receptor (MR), CD163, integrin αvβ5 and anti-inflammatory cytokines (IL-10 and IL-1Ra) together with markers of M1 macrophages such as pro-inflammatory cytokines TNF-α, IL-6, IL-1, MCP-1 and macrophage inflammatory protein-1 (MIP-1a).Citation38 Given the recognized tumor-promoting function of macrophages, Mayi et al. investigated whether ATMs might contribute to the link between obesity and cancer.Citation40 Indeed, as revealed by comparable gene expression profiles, ATMs resembled human tumor-associated macrophages (TAMs). Expression of angiogenic factors, chemokines, cytokines, proteases and growth factors, which are also actively secreted by TAMs, was increased in ATMs vs. control monocyte-derived macrophages (MDMs).Citation40
Tumor-Associated Adipose Tissue and Macrophages
Solid tumors harbor a higher number of macrophages compared with normal, counterpart tissues. TAMs may comprise up to 50% of the tumor cell mass.Citation41 The majority of TAMs are suggested to resemble M2 macrophages, which create a permissive microenvironment for tumor growth and metastasis. TAM infiltration correlates with poor prognosis in cancers of the breast, cervix, bladder and brain.Citation42
Most TAMs are derived from monocytes in the blood and are recruited by a diverse repertoire of chemokines and growth factors including MCP-1 and colony-stimulating factor-1 (CSF-1). Once recruited to tumors, monocytes differentiate into macrophages in response to factors such as M-CSF and GM-CSF and accumulate in avascular and necrotic areas.Citation43 Although it was initially thought that malignant cells were the predominant source of pro-angiogenic mediators, it is now recognized that TAMs and other pro-inflammatory cells express abundant angiogenic factors including VEGF, GM-CSF, IL-1, IL-6 and TNF-α.Citation11 Thus, TAMs play major role in the regulation of tumor angiogenesis by stimulating the growth and migration of vascular endothelial cells.
Cross-talk between adipocytes and cancer cells has been understudied compared with other stromal cells within the tumor microenvironment. To date most knowledge about adipocytes has been derived from studies of breast cancer.Citation44,Citation45 Human breast tumor biopsies are most often totally devoid of adipocytes in the tumor center. The ones present at the invasive front of the tumor are however morphologically changed. They exhibit reduction in size and changes in shape from polyhedral cells to more rounded.Citation46 As shown by Dirat et al., cancer cells strikingly alter adipocyte function and vice versa.Citation47 So called “cancer-associated adipocytes” (CAAs) exhibited a modified phenotype such as delipidation, decreased adipocyte marker expression and overexpression of proteases and pro-inflammatory cytokines. Interestingly, CAAs stimulated tumor cell invasiveness as shown by increased numbers of lung metastases after cancer cell incubation with CAAs followed by intravenous injection. Kushiro et al. found that adipocyte-conditioned medium increased the invasive ability of B16BL6 melanoma cells by promoting epithelial-to-mesenchymal transition (EMT) and downregulating the adhesive protein E-cadherin and metastasis suppressor Kiss1.Citation48
Recently, we found that AT juxtaposed to implanted tumors exhibited reduced adipocyte size, extensive fibrosis, multiple blood vessels and a dense macrophage infiltrate.Citation49 Using syngeneic B16F10 mouse melanoma as a model, we isolated and characterized the tumor-associated adipose tissue (). Histological analysis revealed striking changes in adipocyte morphology and numerous CD11b+ inflammatory cells (). We isolated these CD11b+ cells from tumor-associated AT and found they were characterized as “mixed” based on the expression of M1 (e.g., NOS2 and MCP-1) and M2 (e.g., arginase, VEGF, CD163 and CD301) markers. Most CD11b+ cells were clustered within fibrotic areas and crowded near the periphery of blood vessels. Tumors implanted directly into a subcutaneous WAT depot grew more rapidly and were more vascularized compared with tumors implanted in an anatomical site devoid of a WAT depot. We performed protein expression arrays of tumor-associated AT and found that IL-6 was strikingly upregulated and stimulated endothelial cell proliferation. TIMP-1 and the chemotactic factors CXCL1, MCP-1 and MIP-2 were also highly overexpressed in tumor-associated AT. The mixed M1/M2 macrophage phenotype we observed in tumor-associated AT may not be surprising. As shown by Willenborg et al.,Citation50 macrophages and their monocytic progenitors present during the early stages of repair after excision skin injury were found to overexpress both mediators associated with M1 (i.e., IL-6, NOS2 and IL-1β) and M2 (i.e., arginase, VEGF, PIGF and Tie2) macrophage activation. Later stages of repair skew macrophages toward the M2 phenotype as revealed by expression of anti-inflammatory IL-10 and scavenger receptors CD163 and CD301.
Figure 1. Characteristics of tumor-associated adipose tissue. Representative photograph of adipose tissue (AT) juxtaposed to subcutaneously-implanted B16F10 mouse melanoma (Aa). Hematoxylin-eosin histological examination of normal AT (Ab) and tumor-associated AT (Ac) revealed reduced adipocyte size in tumor-associated AT together with numerous, dilated blood vessels and extensive fibrosis. Yellow arrowheads identify blood vessels. Size bar, 50 µm (Ab–c). Immunohistochemical analysis revealed massive infiltration of CD11b+ cells surrounding blood vessels in tumor-associated AT. Yellow arrowhead identifies a blood vessel. Size bar, 25 µm (Ad–e). Proposed model of interactions between adipocytes, macrophages and vascular endothelial cells within tumor-associated AT using vertical growth-phase melanoma as a model (B). The mass of tumor cells may cause rupture of fragile adipocyte membrane leading to cell death and release of lipids. Free lipids are scavenged by macrophages. Furthermore, in the presence of tumor-derived factors, adipocytes may dedifferentiate into fibroblast-like cells and preadipocytes are mobilized to replace dead or dying adipocytes. The lipid-laden, “activated” macrophages instigate an inflammatory response, which further contributes to fibrosis and extracellular matrix (ECM) deposition. New ECM creates scaffolding for blood vessels and factors such as MCP-1, VEGF and IL-6, secreted by activated macrophages, stimulate angiogenesis and further macrophage recruitment.
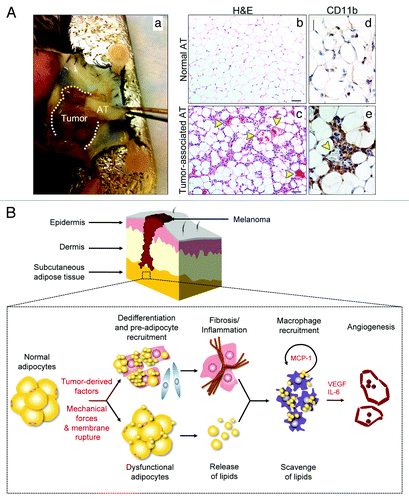
Recent evidence suggests that metabolism might also contribute to the control of macrophage activation in response to cues derived from the tissue microenvironment (for an excellent review, see Biswas and Mantovani).Citation51 For example, M1 macrophages, upon IFNγ activation, express high levels of Ferritin (involved in iron storage) and low levels of Ferroportin (involved in iron export). In contrast, M2 macrophages demonstrate a FerritinlowFerroportinhigh phenotype. Thus, M1 macrophages by sequestering iron, support tumorstatic activity, since iron is indispensable for DNA synthesis. Conversely, M2 macrophages would favor tumor growth.Citation51
Taken together, a model of the tumor microenvironment that includes input and cross-talk between cancer cells, adipocytes, macrophages and vascular cells is emerging. It has been proposed that subcutaneous AT may contribute to the vertical growth phase of primary melanomas by the production of growth factors.Citation52 Moreover, the presence of fat cells within melanomas correlates with both increased metastatic potential and poor prognosis.Citation53 Using vertical growth phase melanoma as a paradigm, in one scenario, recruitment of macrophages into tumor-associated AT may be stimulated by dysfunctional, dead, or dying adipocytes (). Once recruited, macrophages involved in inflammatory processes resembling foam-cell formation in atherogenic plaques are trapped and become resident macrophages that locally promote growth, angiogenesis and tumor progression by releasing inflammatory cytokines and pro-angiogenic factors.
Perspective
While cancer cells are primarily dependent on cell-autonomous mutations resulting in their immortality, tumor growth beyond a few millimeters requires collaboration with stromal cells. For example, fibroblasts, pro-inflammatory cells and vascular cells coalesce to create a permissive microenvironment that allows tumor cells to grow and metastasize. Adipocytes have been largely over-looked as a contributor to these processes, but several recent studies have begun to elucidate how adipocytes might contribute to tumor progression. Particularly in breast tumors which growth within or in close proximity to adipocytes or lymph node metastases, which are surrounded by adipose tissue, tumor cell-to-adipocyte interactions may play important roles in angiogenesis and inflammation. Collectively, recent studies suggest that AT is a “non-trivial” dynamic partner in tumor biology that should be explored in more detail to better understand processes related to tumor progression, inflammation and angiogenesis.
From our perspective, there are multiple prevailing and unanswered questions regarding the role of adipocytes in different tumor microenvironments. First, how do different types of adipocytes (WAT vs. BAT) affect tumor progression? The recent revelation that BAT persists into adult life at least raises the possibility that tumor cells could interact with BAT in a tissue-specific context that is different from WAT.Citation54 Second, how does fatty acid composition affect macrophage recruitment/polarization? Polyunsaturated fatty acids have been shown to reduce macrophage recruitment to AT whereas saturated fatty acids mediate inflammatory cytokine production.Citation21 Thus, the well-established link between inflammation and cancer highlights the potential importance of including dietary interventions targeted at reducing inflammation into therapeutic strategies. Third, what is the nature of adipocyte-derived chemo-attractant signals that promote recruitment and polarization of monocytes into TAMs or ATMs? While stimuli such as TNF and hypoxia are well-known to “educate” macrophages, it is less-understood if free lipids or FFAs in tumor-associated adipose tissue can affect macrophage polarization or recruitment. Finally, how might alterations in tumor-associated adipocytes directly affect the functions of nearby vascular endothelial cells? Adipose tissue is one of the most vascularized tissues in the body and there is an intimate association between vascular cells and adipocytes. In tumors, changes in the expression of adipocyte-derived adipokines or dysfunctional adipocyte metabolism might directly affect the associated vasculature. Thus, the partnership between adipocytes and vascular cells could substantially contribute to processes related to inflammation and tumor development, particularly in breast cancer. A better understanding of the complex alliance between cancer cells, adipocytes, inflammatory cells and vascular cells will further elucidate how the tumor microenvironment fuels tumor growth and how obesity and cancer are linked.
Acknowledgments
M.W. is supported by the Helse-Vest, Haukeland University Hospital. A.C.D. is supported by a K99/ROO award (CA140708) from the National Cancer Institute and National Institutes of Health. We apologize to our colleagues for not citing their excellent work due to space limitations.
Disclosure of Potential Conflicts of Interest
No potential conflicts of interest were disclosed.
References
- Egeblad M, Nakasone ES, Werb Z. Tumors as organs: complex tissues that interface with the entire organism. Dev Cell 2010; 18:884 - 901; http://dx.doi.org/10.1016/j.devcel.2010.05.012; PMID: 20627072
- Available from. . http://www.cancer.gov/cancertopics/factsheet/Risk/obesity.
- Calle EE, Kaaks R. Overweight, obesity and cancer: epidemiological evidence and proposed mechanisms. Nat Rev Cancer 2004; 4:579 - 91; http://dx.doi.org/10.1038/nrc1408; PMID: 15286738
- Hoda MR, Keely SJ, Bertelsen LS, Junger WG, Dharmasena D, Barrett KE. Leptin acts as a mitogenic and antiapoptotic factor for colonic cancer cells. Br J Surg 2007; 94:346 - 54; http://dx.doi.org/10.1002/bjs.5530; PMID: 17212381
- Choi JH, Park SH, Leung PC, Choi KC. Expression of leptin receptors and potential effects of leptin on the cell growth and activation of mitogen-activated protein kinases in ovarian cancer cells. J Clin Endocrinol Metab 2005; 90:207 - 10; http://dx.doi.org/10.1210/jc.2004-0297; PMID: 15522945
- Laud K, Gourdou I, Pessemesse L, Peyrat JP, Djiane J. Identification of leptin receptors in human breast cancer: functional activity in the T47-D breast cancer cell line. Mol Cell Endocrinol 2002; 188:219 - 26; http://dx.doi.org/10.1016/S0303-7207(01)00678-5; PMID: 11911959
- Körner A, Pazaitou-Panayiotou K, Kelesidis T, Kelesidis I, Williams CJ, Kaprara A, et al. Total and high-molecular-weight adiponectin in breast cancer: in vitro and in vivo studies. J Clin Endocrinol Metab 2007; 92:1041 - 8; http://dx.doi.org/10.1210/jc.2006-1858; PMID: 17192291
- Nomura DK, Long JZ, Niessen S, Hoover HS, Ng SW, Cravatt BF. Monoacylglycerol lipase regulates a fatty acid network that promotes cancer pathogenesis. Cell 2010; 140:49 - 61; http://dx.doi.org/10.1016/j.cell.2009.11.027; PMID: 20079333
- Balkwill F, Charles KA, Mantovani A. Smoldering and polarized inflammation in the initiation and promotion of malignant disease. Cancer Cell 2005; 7:211 - 7; http://dx.doi.org/10.1016/j.ccr.2005.02.013; PMID: 15766659
- Hanahan D, Weinberg RA. Hallmarks of cancer: the next generation. Cell 2011; 144:646 - 74; http://dx.doi.org/10.1016/j.cell.2011.02.013; PMID: 21376230
- Lin EY, Li JF, Gnatovskiy L, Deng Y, Zhu L, Grzesik DA, et al. Macrophages regulate the angiogenic switch in a mouse model of breast cancer. Cancer Res 2006; 66:11238 - 46; http://dx.doi.org/10.1158/0008-5472.CAN-06-1278; PMID: 17114237
- Wu J, Boström P, Sparks LM, Ye L, Choi JH, Giang AH, et al. Beige adipocytes are a distinct type of thermogenic fat cell in mouse and human. Cell 2012; 150:366 - 76; http://dx.doi.org/10.1016/j.cell.2012.05.016; PMID: 22796012
- Tilg H, Moschen AR. Adipocytokines: mediators linking adipose tissue, inflammation and immunity. Nat Rev Immunol 2006; 6:772 - 83; http://dx.doi.org/10.1038/nri1937; PMID: 16998510
- Shoelson SE, Herrero L, Naaz A. Obesity, inflammation, and insulin resistance. Gastroenterology 2007; 132:2169 - 80; http://dx.doi.org/10.1053/j.gastro.2007.03.059; PMID: 17498510
- Schipper HS, Prakken B, Kalkhoven E, Boes M. Adipose tissue-resident immune cells: key players in immunometabolism. Trends Endocrinol Metab 2012; 23:407 - 15; http://dx.doi.org/10.1016/j.tem.2012.05.011; PMID: 22795937
- Wentworth JM, Naselli G, Brown WA, Doyle L, Phipson B, Smyth GK, et al. Pro-inflammatory CD11c+CD206+ adipose tissue macrophages are associated with insulin resistance in human obesity. Diabetes 2010; 59:1648 - 56; http://dx.doi.org/10.2337/db09-0287; PMID: 20357360
- Lumeng CN, Deyoung SM, Saltiel AR. Macrophages block insulin action in adipocytes by altering expression of signaling and glucose transport proteins. Am J Physiol Endocrinol Metab 2007; 292:E166 - 74; http://dx.doi.org/10.1152/ajpendo.00284.2006; PMID: 16926380
- Wang Y, Hua S, Tian W, Zhang L, Zhao J, Zhang H, et al. Mitogenic and anti-apoptotic effects of insulin in endometrial cancer are phosphatidylinositol 3-kinase/Akt dependent. Gynecol Oncol 2012; 125:734 - 41; http://dx.doi.org/10.1016/j.ygyno.2012.03.012; PMID: 22426488
- Weisberg SP, McCann D, Desai M, Rosenbaum M, Leibel RL, Ferrante AW Jr.. Obesity is associated with macrophage accumulation in adipose tissue. J Clin Invest 2003; 112:1796 - 808; PMID: 14679176
- Dalmas E, Clément K, Guerre-Millo M. Defining macrophage phenotype and function in adipose tissue. Trends Immunol 2011; 32:307 - 14; http://dx.doi.org/10.1016/j.it.2011.04.008; PMID: 21616718
- Surmi BK, Hasty AH. Macrophage infiltration into adipose tissue: initiation, propagation and remodeling. Future Lipidol 2008; 3:545 - 56; http://dx.doi.org/10.2217/17460875.3.5.545; PMID: 18978945
- Coenen KR, Gruen ML, Lee-Young RS, Puglisi MJ, Wasserman DH, Hasty AH. Impact of macrophage toll-like receptor 4 deficiency on macrophage infiltration into adipose tissue and the artery wall in mice. Diabetologia 2009; 52:318 - 28; http://dx.doi.org/10.1007/s00125-008-1221-7; PMID: 19052722
- Monteiro R, de Castro PM, Calhau C, Azevedo I. Adipocyte size and liability to cell death. Obes Surg 2006; 16:804 - 6; http://dx.doi.org/10.1381/096089206777346600; PMID: 16756747
- Cinti S, Mitchell G, Barbatelli G, Murano I, Ceresi E, Faloia E, et al. Adipocyte death defines macrophage localization and function in adipose tissue of obese mice and humans. J Lipid Res 2005; 46:2347 - 55; http://dx.doi.org/10.1194/jlr.M500294-JLR200; PMID: 16150820
- Sun K, Kusminski CM, Scherer PE. Adipose tissue remodeling and obesity. J Clin Invest 2011; 121:2094 - 101; http://dx.doi.org/10.1172/JCI45887; PMID: 21633177
- Gruen ML, Hao M, Piston DW, Hasty AH. Leptin requires canonical migratory signaling pathways for induction of monocyte and macrophage chemotaxis. Am J Physiol Cell Physiol 2007; 293:C1481 - 8; http://dx.doi.org/10.1152/ajpcell.00062.2007; PMID: 17728393
- Gutierrez DA, Hasty AH. Haematopoietic leptin receptor deficiency does not affect macrophage accumulation in adipose tissue or systemic insulin sensitivity. J Endocrinol 2012; 212:343 - 51; http://dx.doi.org/10.1530/JOE-11-0338; PMID: 22194312
- Curat CA, Miranville A, Sengenès C, Diehl M, Tonus C, Busse R, et al. From blood monocytes to adipose tissue-resident macrophages: induction of diapedesis by human mature adipocytes. Diabetes 2004; 53:1285 - 92; http://dx.doi.org/10.2337/diabetes.53.5.1285; PMID: 15111498
- Brake DK, Smith EO, Mersmann H, Smith CW, Robker RL. ICAM-1 expression in adipose tissue: effects of diet-induced obesity in mice. Am J Physiol Cell Physiol 2006; 291:C1232 - 9; http://dx.doi.org/10.1152/ajpcell.00008.2006; PMID: 16807303
- Kamei N, Tobe K, Suzuki R, Ohsugi M, Watanabe T, Kubota N, et al. Overexpression of monocyte chemoattractant protein-1 in adipose tissues causes macrophage recruitment and insulin resistance. J Biol Chem 2006; 281:26602 - 14; http://dx.doi.org/10.1074/jbc.M601284200; PMID: 16809344
- Mosser DM, Edwards JP. Exploring the full spectrum of macrophage activation. Nat Rev Immunol 2008; 8:958 - 69; http://dx.doi.org/10.1038/nri2448; PMID: 19029990
- Mantovani A, Sica A, Sozzani S, Allavena P, Vecchi A, Locati M. The chemokine system in diverse forms of macrophage activation and polarization. Trends Immunol 2004; 25:677 - 86; http://dx.doi.org/10.1016/j.it.2004.09.015; PMID: 15530839
- Lumeng CN, Bodzin JL, Saltiel AR. Obesity induces a phenotypic switch in adipose tissue macrophage polarization. J Clin Invest 2007; 117:175 - 84; http://dx.doi.org/10.1172/JCI29881; PMID: 17200717
- Patsouris D, Li PP, Thapar D, Chapman J, Olefsky JM, Neels JG. Ablation of CD11c-positive cells normalizes insulin sensitivity in obese insulin resistant animals. Cell Metab 2008; 8:301 - 9; http://dx.doi.org/10.1016/j.cmet.2008.08.015; PMID: 18840360
- Suganami T, Tanimoto-Koyama K, Nishida J, Itoh M, Yuan XM, Mizuarai S, et al. Role of the Toll-like receptor 4/NF-kappa B pathway in saturated fatty acid-induced inflammatory changes in the interaction between adipocytes and macrophages. Arterioscl Throm Vas 2007; 27:84 - 91; http://dx.doi.org/10.1161/01.ATV.0000251608.09329.9a
- Cani PD, Amar J, Iglesias MA, Poggi M, Knauf C, Bastelica D, et al. Metabolic endotoxemia initiates obesity and insulin resistance. Diabetes 2007; 56:1761 - 72; http://dx.doi.org/10.2337/db06-1491; PMID: 17456850
- Ohashi K, Parker JL, Ouchi N, Higuchi A, Vita JA, Gokce N, et al. Adiponectin promotes macrophage polarization toward an anti-inflammatory phenotype. J Biol Chem 2010; 285:6153 - 60; http://dx.doi.org/10.1074/jbc.M109.088708; PMID: 20028977
- Zeyda M, Farmer D, Todoric J, Aszmann O, Speiser M, Györi G, et al. Human adipose tissue macrophages are of an anti-inflammatory phenotype but capable of excessive pro-inflammatory mediator production. Int J Obes (Lond) 2007; 31:1420 - 8; http://dx.doi.org/10.1038/sj.ijo.0803632; PMID: 17593905
- Shaul ME, Bennett G, Strissel KJ, Greenberg AS, Obin MS. Dynamic, M2-like remodeling phenotypes of CD11c+ adipose tissue macrophages during high-fat diet--induced obesity in mice. Diabetes 2010; 59:1171 - 81; http://dx.doi.org/10.2337/db09-1402; PMID: 20185806
- Mayi TH, Daoudi M, Derudas B, Gross B, Bories G, Wouters K, et al. Human adipose tissue macrophages display activation of cancer-related pathways. J Biol Chem 2012; 287:21904 - 13; http://dx.doi.org/10.1074/jbc.M111.315200; PMID: 22511784
- Kelly PMA, Davison RS, Bliss E, McGee JO. Macrophages in human breast disease: a quantitative immunohistochemical study. Br J Cancer 1988; 57:174 - 7; http://dx.doi.org/10.1038/bjc.1988.36; PMID: 2833921
- Bingle L, Brown NJ, Lewis CE. The role of tumour-associated macrophages in tumour progression: implications for new anticancer therapies. J Pathol 2002; 196:254 - 65; http://dx.doi.org/10.1002/path.1027; PMID: 11857487
- Murdoch C, Giannoudis A, Lewis CE. Mechanisms regulating the recruitment of macrophages into hypoxic areas of tumors and other ischemic tissues. Blood 2004; 104:2224 - 34; http://dx.doi.org/10.1182/blood-2004-03-1109; PMID: 15231578
- Tan JX, Buache E, Chenard MP, Dali-Youcef N, Rio MC. Adipocyte is a non-trivial, dynamic partner of breast cancer cells. Int J Dev Biol 2011; 55:851 - 9; http://dx.doi.org/10.1387/ijdb.113365jt; PMID: 21948738
- Wang YY, Lehuédé C, Laurent V, Dirat B, Dauvillier S, Bochet L, et al. Adipose tissue and breast epithelial cells: a dangerous dynamic duo in breast cancer. Cancer Lett 2012; 324:142 - 51; http://dx.doi.org/10.1016/j.canlet.2012.05.019; PMID: 22643115
- Andarawewa KL, Motrescu ER, Chenard MP, Gansmuller A, Stoll I, Tomasetto C, et al. Stromelysin-3 is a potent negative regulator of adipogenesis participating to cancer cell-adipocyte interaction/crosstalk at the tumor invasive front. Cancer Res 2005; 65:10862 - 71; http://dx.doi.org/10.1158/0008-5472.CAN-05-1231; PMID: 16322233
- Dirat B, Bochet L, Dabek M, Daviaud D, Dauvillier S, Majed B, et al. Cancer-associated adipocytes exhibit an activated phenotype and contribute to breast cancer invasion. Cancer Res 2011; 71:2455 - 65; http://dx.doi.org/10.1158/0008-5472.CAN-10-3323; PMID: 21459803
- Kushiro K, Chu RA, Verma A, Núñez NP. Adipocytes Promote B16BL6 Melanoma Cell Invasion and the Epithelial-to-Mesenchymal Transition. Cancer Microenviron 2012; 5:73 - 82; http://dx.doi.org/10.1007/s12307-011-0087-2; PMID: 21892698
- Wagner M, Bjerkvig R, Wiig H, Melero-Martin JM, Lin RZ, Klagsbrun M, et al. Inflamed tumor-associated adipose tissue is a depot for macrophages that stimulate tumor growth and angiogenesis. Angiogenesis 2012; 15:481 - 95; http://dx.doi.org/10.1007/s10456-012-9276-y; PMID: 22614697
- Willenborg S, Lucas T, van Loo G, Knipper JA, Krieg T, Haase I, et al. CCR2 recruits an inflammatory macrophage subpopulation critical for angiogenesis in tissue repair. Blood 2012; 120:613 - 25; http://dx.doi.org/10.1182/blood-2012-01-403386; PMID: 22577176
- Biswas SK, Mantovani A. Orchestration of metabolism by macrophages. Cell Metab 2012; 15:432 - 7; http://dx.doi.org/10.1016/j.cmet.2011.11.013; PMID: 22482726
- Klein J, Permana PA, Owecki M, Chaldakov GN, Böhm M, Hausman G, et al. What are subcutaneous adipocytes really good for?. Exp Dermatol 2007; 16:45 - 70; PMID: 17181636
- Smolle J, Hofmann-Wellenhof R, Woltsche-Kahr I, Haas J, Kerl H. Quantitative assessment of fat cells in subcutaneous metastatic melanoma. Correlation with outcome. Am J Dermatopathol 1995; 17:555 - 9; http://dx.doi.org/10.1097/00000372-199512000-00005; PMID: 8599468
- Cypess AM, Lehman S, Williams G, Tal I, Rodman D, Goldfine AB, et al. Identification and importance of brown adipose tissue in adult humans. N Engl J Med 2009; 360:1509 - 17; http://dx.doi.org/10.1056/NEJMoa0810780; PMID: 19357406