Abstract
All aerobic organisms have developed sophisticated mechanisms to prevent, detect and respond to cell damage caused by the unavoidable production of reactive oxygen species (ROS). Plants and algae are able to synthesize specific pigments in the chloroplast called carotenoids to prevent photo-oxidative damage caused by highly reactive by-products of photosynthesis. In this study we used the unicellular green alga Chlamydomonas reinhardtii to demonstrate that defects in carotenoid biosynthesis lead to the activation of autophagy, a membrane-trafficking process that participates in the recycling and degradation of damaged or toxic cellular components. Carotenoid depletion caused by either the mutation of phytoene synthase or the inhibition of phytoene desaturase by the herbicide norflurazon, resulted in a strong induction of autophagy. We found that high light transiently activates autophagy in wild-type Chlamydomonas cells as part of an adaptation response to this stress. Our results showed that a Chlamydomonas mutant defective in the synthesis of specific carotenoids that accumulate during high light stress exhibits constitutive autophagy. Moreover, inhibition of the ROS-generating NADPH oxidase partially reduced the autophagy induction associated to carotenoid deficiency, which revealed a link between photo-oxidative damage, ROS accumulation and autophagy activation in Chlamydomonas cells with a reduced carotenoid content.
Introduction
Photosynthetic reactions require the orchestrated participation of multi-subunit protein complexes and cofactors, including metals, lipids and pigments such as chlorophyll and carotenoids.Citation1-Citation3 Like chlorophyll, carotenoids are essential constituents of all photosynthetic organisms due to their antioxidant and protective properties, although some nonphotosynthetic organisms including fungi and bacteria are able to synthesize these pigments.Citation4 Carotenoids are also present in many animals as visual pigments, antioxidants or colorants, although metazoans are not capable of de novo synthesis of these molecules. Two types of carotenoids can be distinguished: carotenes, consisting exclusively of hydrogen and carbon atoms and xanthophylls, which contain one or more oxygen functions.Citation4,Citation5 In plants and algae, carotenoids localize to the chloroplast, where they are synthesized,Citation4,Citation5 although extraplastidic carotenoids are frequently encountered among green algae under certain stress conditions.Citation6,Citation7 Perhaps the most important function of carotenoids in photosynthetic organisms is to protect cells against excessive light and toxic reactive oxygen species (ROS) generated as by-products of photosynthesis. Carotenoids aid in light harvesting, prevent photo-oxidative damage of the thylakoid membrane through quenching of excited intermediates like superoxide anion (1O2) or triplet chlorophyll (3Chl) molecules, and participate in energy dissipation when light absorption exceeds the photosynthetic capacity in a process known as nonphotochemical quenching (NPQ).Citation8-Citation11
Our current knowledge on the biosynthesis of carotenoids has been obtained mostly from studies in plants and carotenogenic bacteria.Citation12-Citation15 Nevertheless, early works with carotenoid-deficient mutants of the unicellular green alga Chlamydomonas reinhardtii were among the first of this type of mutants characterized in plants and algae.Citation16 Chlamydomonas has been widely used as a powerful model system for the study of photosynthesis and the biogenesis of the photosynthetic apparatus. Mutants deficient in photosynthetic activity were originally isolated in Chlamydomonas based on its ability to grow using acetate from the medium as carbon source.Citation17 In the presence of acetate, the photosynthetic function of Chlamydomonas is dispensable and mutants of essential photosynthetic genes are viable and can be readily isolated. An additional important feature of Chlamydomonas is its capacity to synthesize chlorophyll and fully assemble the photosynthetic apparatus in the dark. This is in contrast to higher plants, which are not able to maintain normal chloroplast structure and function in the absence of light.Citation18 These physiological features of Chlamydomonas together with available molecular tools for the analysis and efficient transformation of the nuclear and chloroplast genomes of Chlamydomonas allowed for the identification of central components in photosynthesis, such as the Rieske protein of the cytochrome b6f complex.Citation17
Functional studies performed in Chlamydomonas have shown that some mutants deficient in carotenoid biosynthesis are light sensitive and need to be grown in the dark on acetate-containing medium.Citation19 It has been also reported that Chlamydomonas mutant cells unable to synthesize photo-protective xanthophylls such as zeaxanthin and lutein bleach and accumulate ROS when exposed to high light.Citation9 The molecular mechanisms and signaling pathways participating in the cellular response to this damage are unclear. In plants, oxidative stress mediated by H2O2 or methyl viologen (MV) generates ROS and induces the degradation of oxidized proteins in the vacuole via autophagy (also known as macroautophagy).Citation20 During autophagy, cytosolic components, including entire organelles, organelle fragments, membranes and protein complexes, are enclosed in bulk within a double-membrane vesicle, called an autophagosome, and delivered to the vacuole to recycle needed nutrients or degrade toxic components.Citation21,Citation22 Autophagy is conserved from yeast to plants and mammals, indicating that components of this catabolic process are evolutionarily conserved. Pioneer mutagenesis studies in yeast identified autophagy-related (ATG) genes, which mediate structural and regulatory functions of autophagy.Citation23 Homologs to yeast ATG genes have been identified in mammals and more recently in plants and algae.Citation24-Citation26 The analysis of atg mutants in plants has revealed that autophagy is active at a basal level under normal growth and is required for the adequate adaptation and response of the plant to different stresses such as nutrient limitation, oxidative stress, salt and drought conditions and pathogen attack.Citation27
The development of specific autophagy markers has been decisive to increase our knowledge about this catabolic process. The highly conserved ATG8 protein tightly associates with the autophagosome membrane and this stable binding has been widely used to monitor autophagy in different systems. A conserved C-terminal Gly residue of ATG8 covalently binds to the lipid phosphatidylethanolamine (PE), and this modified form firmly binds to the autophagosome.Citation28 In plants, two markers for autophagosomes have been reported, the monodansylcadaverine dye and GFP-ATG8 fusion protein.Citation29-Citation31 ATG8 is functionally conserved in Chlamydomonas and as in other organisms this protein has been exploited as an autophagy marker to investigate this process in algae.Citation32,Citation33 Chlamydomonas ATG8 (CrATG8) is cleaved at the C-terminal conserved Gly and is associated with membranes. Stationary growth phase or different stress conditions such as nutrient limitation, oxidative stress or the accumulation of misfolded proteins in the endoplasmic reticulum caused the activation of autophagy in Chlamydomonas.Citation33 Immunofluorescence (IF) microscopy studies showed that CrATG8 is localized to punctate structures and a single spot could be detected in many exponentially growing cells whereas autophagy activation resulted in a pronounced detection of CrATG8 in more spots.Citation33
The ultrastructure analysis of a Chlamydomonas mutant strain (lts1-204) deficient in phytoene synthase,Citation19 one of the first enzymes in the carotenoid biosynthetic pathway (), revealed the presence of cytoplasmic compartments that resembled autophagous vacuoles filled with membranous material.Citation34 However, the unavailability at that time of a specific autophagy marker made impossible to test the activation of this process in lts1-204 cells. In this study, we took advantage of the development of a molecular marker of autophagy in Chlamydomonas to establish a link between carotenoid biosynthesis and control of autophagy in a photosynthetic organism.
Figure 1. Schematic representation of carotenoid biosynthesis in Chlamydomonas. Mutations in lts1-204 and npq1 lor1 strains are indicated. Norflurazon blocks carotenoid biosynthesis by inhibiting the phytoene desaturase enzyme, the first enzyme of the pathway that converts the colorless phytoene in colored carotenoids in Chlamydomonas.
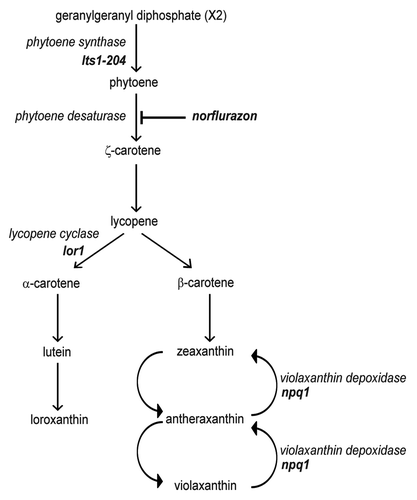
Results
Mutation of phytoene synthase triggers autophagy
The Chlamydomonas lts1-204 mutant is unable to synthesize carotenoidsCitation19 and accumulates cytoplasmic vacuoles.Citation34 To investigate whether the catabolic process of autophagy is induced in lts1-204 cells, the abundance and the modification state of the CrATG8 protein were examined. Compared with wild-type cells grown under similar conditions, CrATG8 was more abundant in lts1-204 cells (). Moreover, modified forms of the protein that specifically appear upon autophagy activation,Citation33 were detected (), demonstrating that autophagy is upregulated in cells lacking phytoene synthase. Since lts1-204 mutant cells cannot grow in the presence of light due to the absence on protective carotenoids,Citation19 we next studied the effect of light on autophagy in this mutant. lts1-204 cells were grown in the dark and then shifted to standard light conditions. The level of unmodified and modified forms of CrATG8 increased in lts1-204 cells upon exposure to light compared with dark conditions (), which indicates that the damage caused by the light in these cells led to a further induction of autophagy. No significant change in CrATG8 abundance was detected in wild-type cells shifted from dark to standard light (). Autophagy activation in plants leads to the mobilization of Rubisco, the most abundant chloroplast protein, and other stroma-localized proteins to the vacuole through an ATG gene-dependent mechanism.Citation35-Citation37 We examined the abundance of the Rubisco large subunit (RbcL) in lts1-204 cells exposed to light and no decrease was observed despite autophagy activation under these conditions ().
Figure 2. Constitutive autophagic activity in the lts1-204 mutant. (A) Induction and modification of CrATG8 in the lts1-204 mutant. Cells were grown in acetate medium (TAP) to 2 × 106 cells/ml (exponential phase) under dark conditions and soluble extracts were obtained as described in Materials and Methods. Fifteen micrograms of total extracts of Chlamydomonas cells were resolved by 15% SDS-PAGE followed by western blotting with anti-CrATG8 and anti-CrFKBP12 antibodies. The asterisk denotes modified forms of the CrATG8 protein. (B) CrATG8 modification upon dark-to-light transition. Chlamydomonas cells growing exponentially (1 × 106 cells/ml) in TAP under dark conditions were transferred to standard light illumination (20 µE) and samples were taken at different times for its analysis. Cells maintained in dark conditions were used as control. Cell-free extracts were resolved by SDS-PAGE and immunoblotted with anti-CrATG8 and anti-RbcL. Western blot analyses with anti-CrFKBP12 were used as loading controls. (C) Immunolocalization of CrATG8 in wild-type and lts1-204 Chlamydomonas cells. Cells growing exponentially in TAP medium without light were transferred to standard light for 6 h or maintained in dark conditions as a control. Cells were collected and processed for IF microscopy analysis. Scale bar, 8 µm. (D) ROS levels in wild-type and lts1-204 Chlamydomonas strains. Log phase cells growing in TAP medium in the dark were either transferred to standard light for 6 h or maintained in the dark. ROS production was determined as described in Materials and Methods. Values are the means of five independent determinations.
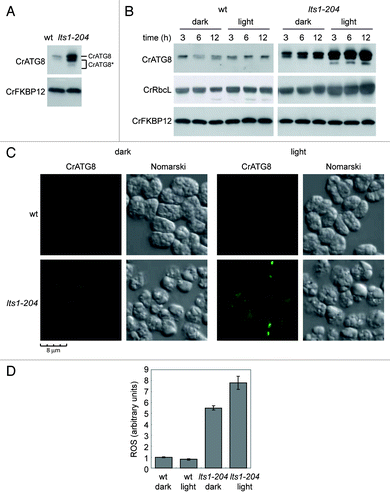
In addition to CrATG8 abundance and modification, the cellular distribution of this protein can also be used to monitor autophagy in Chlamydomonas.Citation33 In wild-type cells grown in the dark, the CrATG8 signal was very weak and localized in a single spot in some cells (). Under similar conditions, the spots corresponding to CrATG8 were more intense and easily detected in lts1-204 cells (), in consonance with the increased abundance of this protein and the detection of its modified forms by western blot (). In agreement with the increased autophagy observed in lts1-204 cells exposed to light (), the overall CrATG8 signal was sharper and several spots per cell could be detected in carotenoid-deficient but not in wild-type cells shifted to light ().
Given the critical role that carotenoids play in preventing photo-oxidative damage in plants and algae, we determined the level of ROS in wild-type and lts1-204 cells grown in the dark or exposed to light. Despite the absence of light, the basal level of ROS was higher in carotenoid-deficient cells (), indicating that these pigments are also important to maintain low ROS levels in complete darkness. As expected from the protective role of carotenoids, exposure of lts1-204 cells to light resulted in an increase of ROS whereas no significant effect was observed in wild-type cells (). Taken together, these results strongly suggest that autophagy is induced in lts1-204 cells as a consequence of ROS accumulation.
Inhibition of phytoene desaturase by norflurazon activates autophagy
The former results indicate that autophagy is induced in carotenoid-deficient lts1-204 cells. However, carotenoids can never be synthesized in these cells due to the absence of phytoene synthase, and autophagic activity might be upregulated due to the adaptation of cells to the lack of carotenoids. To strengthen the correlation between carotenoid deficiency and autophagy activation, we used the bleaching herbicide norflurazon (NF), which specifically inhibits phytoene desaturase activityCitation38 to block the biosynthesis of carotenoids in wild-type cells. Log phase cultures were treated with 20 μM NF and autophagy was monitored by western blot and IF assays of the CrATG8 protein. The abundance of CrATG8 increased and modified forms of the protein could be detected after 24 h of treatment whereas no effect was observed in untreated cells (). These effects were even more pronounced after 48 h (). Lower concentrations of NF were tested for autophagy induction and our results indicated that 100–500 nM was sufficient to significantly activate autophagy (Fig. S1). IF assays also revealed a strong induction of autophagy in NF-treated cells, since the CrATG8 signal was much more intense and several spots per cell could be detected compared with untreated cells (). To confirm that NF is indeed inhibiting carotenoid biosynthesis, the level of prominent carotenoids in ChlamydomonasCitation5 was determined. NF drastically reduced the level of violaxanthin, lutein and β-carotene (). In contrast, the concentration of zeaxanthin, a specific carotenoid synthesized during high light stress,Citation39 increased in NF-treated cells (). Downregulation of prominent carotenoids by NF was fast since a clear reduction was observed after 6 h and about 80–90% of these carotenoids were depleted within 12 h (Fig. S2).
Figure 3. Inhibition of the carotenoid biosynthetic pathway leads to autophagy activation in Chlamydomonas. (A) Induction and modification of CrATG8 by norflurazon (NF) treatment in light or dark growth conditions. Wild-type Chlamydomonas cells in exponential growth phase were treated with 20 µM NF during 6, 12, 24 or 48 h under standard light (left panel) or dark (right panel) conditions. Samples of nontreated cells were taken at the same times and used as control. Fifteen µg of total extracts were resolved by 15% SDS-PAGE followed by western blotting with anti-CrATG8, anti-RbcL and anti-CrFKBP12 antibodies. (B) Immunolocalization of CrATG8 in wild-type cells treated with NF. Chlamydomonas cells growing exponentially under light (right) or dark (left) conditions were treated with NF for 48 h. Nontreated cells were used as control. Cells were collected and processed for IF microscopy analysis. Scale bar, 8 µm. (C) Changes in carotenoid contents in response to NF treatment in light and dark growth conditions. Prominent carotenoids present in wild-type Chlamydomonas cells treated or not with NF for 24 h were determined as described in Materials and Methods. Results are means of three independent experiments. (D) Induction of ROS in response to NF treatment. The content of ROS in TAP-grown cells under light or dark conditions and treated with NF for 24 or 48 h was determined. Untreated cells were used as control. Values are the means of four independent experiments.
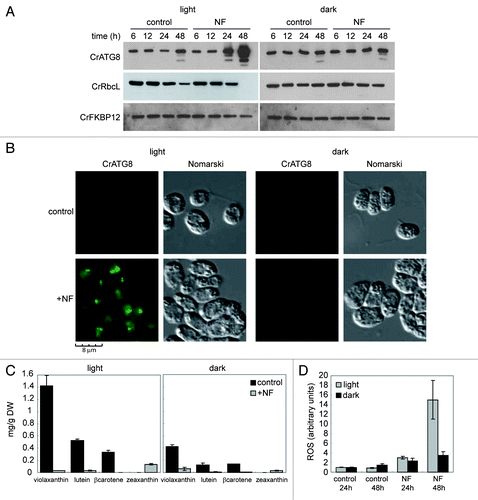
Given that carotenoid-deficient cells have reduced protection from light, we also investigated the effect of NF in the dark. Interestingly, NF had no effect on autophagy in the absence of light () despite carotenoids were effectively depleted (). The analysis of RbcL abundance upon NF treatment in light- or dark-grown cells revealed that this protein is not primarily degraded via autophagy since RbcL is still detected after 24 h of treatment in the light when autophagy is already induced (). However, a prolonged treatment resulted in a further induction of autophagy and the degradation of RbcL (), suggesting that these two processes might be linked when cells are subjected to a severe stress. Supporting this model, we found that NF-mediated degradation of RbcL was not observed in the dark when autophagy is not induced (). ROS levels were also determined in NF-treated cells in the presence or in the absence of light. Inhibition of carotenoid biosynthesis by NF led to a dramatic increase of ROS in the light but not in the dark (). Thus, these results link autophagy activation to ROS production due to a reduced protection of carotenoid-deficient cells from light.
Activation of autophagy in Chlamydomonas cells lacking specific xanthophylls
In addition to studying autophagy in cells fully depleted of carotenoids, we investigated whether the absence of specific xanthophylls may play a role in the control of autophagy in Chlamydomonas. The npq1 lor1 double mutant is unable to synthesize α-carotene, lutein and loroxanthin or to convert violaxanthin to antheraxanthin and zeaxanthinCitation9 (). Despite the absence of these specific carotenoids, npq1 lor1 mutant cells display a similar rate of growth in low light to wild-type cells.Citation9 Our results indicated that autophagy is upregulated in the npq1 lor1 mutant grown under dim light conditions compared with wild-type cells. High levels of CrATG8 and modified forms of the protein were observed in mutant cells compared with wild-type cells (). Moreover, IF assays showed that spots corresponding to CrATG8 could be readily detected and the overall signal was more intense in the npq1 lor1 mutant than in wild-type cells under similar growth conditions ().
Figure 4. Autophagy is constitutively induced in the npq1 lor1 mutant. (A) Induction and modification of CrATG8 in the npq1 lor1 mutant. Wild-type and npq1 lor1 strains were grown to log phase in TAP medium in dim light conditions. The abundance of CrATG8 was analyzed by western blot using CrFKBP12 as loading control. Fifteen micrograms of total extracts were loaded per lane. (B) High light stress activates autophagy. Wild-type, npq1, lor1 and npq1 lor1 strains were grown in TAP medium to exponential growth phase under dim light and then shifted to high light (1200 µE m−2 s−1). Samples were taken at the indicated times and processed for western blot analysis with the indicated antibodies. Cells maintained in dim light were used as control. (C) Immunolocalization of CrATG8 in wild-type and npq1 lor1 cells under dim light or exposed to high light stress for 6 h. Scale bar, 8 µm. (D) ROS levels in wild-type and npq1 lor1 cells grown to log phase in dim light or shifted to high light for 6 h. Values are the means of four independent determinations.
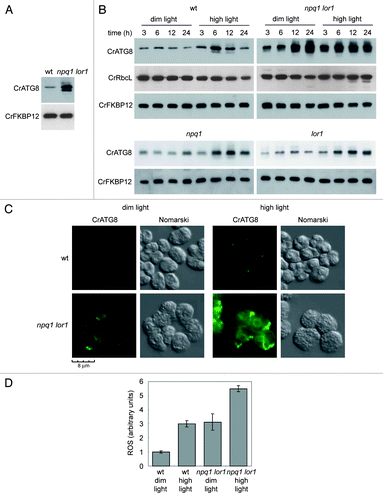
Exposure of npq1 lor1 cells to high light results in impaired growth and rapid bleaching due to reduced photo-protection.Citation9 We therefore investigated whether the lack of specific xanthophylls may affect the regulation of autophagy in response to high light (1200 µE m−2 s−1). Wild-type and npq1 lor1 mutant cells were grown in dim light and then shifted to high light, and autophagy was monitored at different times. In wild-type cells, increased levels of CrATG8 were detected after 6 h under high light conditions although CrATG8 abundance recovered the level observed in untreated cells after 24 h (). These results indicate that autophagy is transiently induced in wild-type cells in response to high light and strongly suggest that this catabolic process participates in the adaptation of the cell to this stress in Chlamydomonas. IF assays also showed an induction of autophagy in wild-type cells subjected to high light stress (). In contrast to wild-type cells, CrATG8 levels were higher in the npq1 lor1 mutant grown in dim light (). In addition to the overall increased CrATG8 abundance, we found that CrATG8 is upregulated in npq1 lor1 cells after 12 h of growth in dim light (). We have previously reported induction and modification of CrATG8 when wild-type Chlamydomonas cells reach the stationary growth phaseCitation33 (). Our present results indicate that the induction of CrATG8 associated to growth phase developed earlier in npq1 lor1 mutant cells, suggesting that the absence of specific carotenoids may alter the regulation of autophagy during cell growth. Exposure of npq1 lor1 mutant cells to high light also led to a further activation of autophagy although this induction occurred earlier than in wild-type cells and was not transient (). In agreement with these results, the cellular distribution of CrATG8 strongly differed in wild-type and mutant cells in response to high light. The CrATG8 signal was detected as intense spots as well as membranous-like structures in npq1 lor1 mutant cells exposed to high light (). No RbcL degradation was observed in wild-type and npq1 lor1 mutant cells at different light intensities ().
Unlike the npq1 lor1 double mutant, npq1 and lor1 single mutants display a growth rate similar to wild-type cells at low and high light conditions due to the redundant protective effect of lutein/loroxanthin and zeaxanthin/antheraxanthin against photo-oxidative damage.Citation9 To further investigate the possible link between autophagy activation and reduced carotenoid-mediated photo-protection, CrATG8 activation was monitored in npq1 and lor1 single mutants. In contrast to npq1 lor1 cells, the single mutants exhibited autophagic activity similar to the one observed in wild-type cells under dim or high light conditions (). These results support the activation of autophagy only when the photo-protection mediated by carotenoids is compromised.
It is well known that high light enhances the production of ROS in photosynthetic organisms. Accordingly, we found an increase of ROS in wild-type cells shifted from dim light to high light for 6 h (), which correlated with the induction of autophagy observed in these cells (). We also determined the abundance of ROS in the npq1 lor1 mutant grown in dim light or shifted to high light. In agreement with the increased autophagy detected in npq1 lor1 mutant cells in dim light (), ROS levels were higher compared with wild-type cells under these conditions (). Moreover, exposure to high light further induced ROS production in the npq1 lor1 mutant (). Taken together, these results established a clear correlation between ROS production and autophagy activation in response to high light and demonstrate that specific xanthophylls are required for the proper regulation of autophagy under this stress.
Inhibition of photosynthetic electron transport does not trigger autophagy
As integral components of LHCI, LHCII and cytochrome b6f complex, carotenoids play an essential role in the assembly of photosynthetic complexes and are required for an efficient electron transport in the chloroplast.Citation4 To investigate whether the induction of autophagy associated to carotenoid deficiency might be due to the inhibition of photosynthetic electron transport, autophagy was monitored in Chlamydomonas cells treated with the photosynthetic inhibitors DCMU or DBMIB. DCMU inhibits the flow of electrons from PSII to plastoquinone whereas DBMIB blocks the flow after plastoquinone by binding to the cytochrome b6f complex.Citation40 None of these inhibitors had a significant effect on autophagy (), strongly suggesting that the induction of this degradative process in carotenoid-deficient cells is not due to the inhibition of photosynthetic electron transport.
Figure 5. Inhibition of photosynthetic electron flow does not activate autophagy in Chlamydomonas. (A) Wild-type Chlamydomonas cells were grown in TAP medium to log phase under standard light conditions. The photosynthetic inhibitors DCMU (10 µM) or DBMIB (2 µM) were added and samples were taken at the indicated times and processed for western blot analysis. (B) Effect of MV on photosynthesis. Exponentially growing cells were treated with MV (1 µM) in the presence or in the absence of light and samples were collected at different times of treatment. Untreated cells were used as control. The abundance of CrATG8 and RbcL proteins in total extracts was analyzed by western blotting using CrFKBP12 as loading control. (C) Immunofluorescence analysis of CrATG8 in wild-type cells treated with 1 µM MV for 8 h under light and dark conditions. Scale bar, 8 µm. (D) ROS levels in MV treated cells. Cells growing exponentially under light or dark conditions were treated with 1 µM MV for 8 h and ROS levels were determined. Values are the means of five independent experiments. (E) Activation of autophagy by H2O2. CrATG8 abundance was analyzed in light (L) and dark (D) grown cells treated or not (control) with 1 mM H2O2 for 8 h.
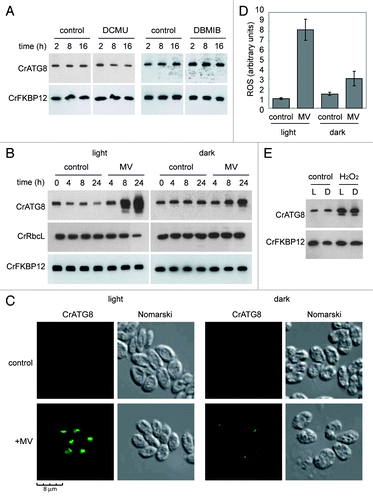
In plants, it has been shown that the herbicide MV, also known as paraquat, triggers autophagy.Citation20 MV has a double effect as inhibitor of photosynthesis and generator of ROS. In the chloroplast, MV intercepts electrons from PSI and then reacts with oxygen to produce superoxide anion (O2-), which in turn is rapidly converted to H2O2.Citation41 We found that MV potently induced autophagy in Chlamydomonas in the presence of light but has also a moderate effect in the dark (), indicating that the action of MV on autophagy is associated with the generation of ROS in the chloroplast through the disruption of the photosynthetic electron flow. As expected from these results, ROS production in the chloroplast was enhanced in Chlamydomonas cells treated with MV in the light whereas only a mild increase was detected in the dark (). Activation of autophagy by MV-induced chloroplast damage did not directly result in RbcL degradation (; 8 h treatment with light) but a prolonged stress significantly reduced RbcL abundance (; 24 h treatment under light conditions). Nevertheless, downregulation of RbcL was not detected under dark conditions when autophagy is not induced (), revealing that autophagy might indirectly participate in RbcL degradation upon severe oxidative stress. Unlike MV, H2O2 can cause oxidative damage directly to cellular components such as proteins or lipids, and we found accordingly that H2O2 treatment similarly activates autophagy in both light and dark (). Together, these results demonstrate that inhibition of photosynthetic electron flow has no effect on autophagy unless it is coupled to the generation of ROS.
NADPH oxidase activity partially regulates autophagy induction in response to carotenoid deficiency
NADPH oxidases are ROS-producing enzymes localized at the plasma membrane and are highly conserved in plants.Citation42 In a recent study, it has been shown that inhibition of NADPH oxidase activity by diphenylene iodinium (DPI) suppressed autophagy induction upon nutrient starvation and salt stress but not during osmotic stress.Citation43 These findings assigned an important role to ROS generated by NADPH oxidases to the regulation of autophagy in plants and revealed that this process can be activated by NADPH oxidase-dependent and -independent pathways. ROS-producing NADPH oxidase gene family seems to be conserved in algal genomes,Citation44 and accordingly two putative NADPH oxidase homologs could be found in the C. reinhardtii genome (http://genome.jgi-psf.org/Chlre4/Chlre4.home.html), RBOL1 (au5.g10913_t1) and RBOL2 (au5.g10915_t1). Thus, we investigated whether inhibition of NADPH oxidase by DPI may have an effect on autophagy induction by carotenoid depletion. Chlamydomonas cells were treated first with NF to inhibit carotenoid biosynthesis and then with DPI or drug vehicle as control. Interestingly, the modified forms of CrATG8 detected in NF-treated cells were strongly reduced with DPI, although the overall increase in CrATG8 abundance, a landmark of autophagy activation, was not significantly affected. As expected, DPI had no effect on autophagy induction mediated by MV or H2O2 treatment since these two compounds are direct generators of ROS. Thus, the partial reduction of autophagy activation by DPI revealed a functional link between ROS production and autophagy regulation in response to photo-oxidative damage.
Discussion
Electron microscopy studies have shown that the carotenoid-deficient mutant lts1-204 accumulates cytoplasmic compartments that appeared to be autophagous vacuoles filled with membranous material.Citation34 Our current knowledge about the function of vacuoles in Chlamydomonas is limited and the presence of double-membrane autophagosomes has not been reported so far. Most Chlamydomonas species have two contractile vacuoles at the apical region of the cell involved in maintenance of osmotic balance.Citation45 However, additional small (about 1 μm in diameter) vacuoles located between the chloroplast and nucleus can be detected in wild-type Chlamydomonas cells under optimal growth.Citation46,Citation47 These vacuoles contain electron dense, polymorphic material and may provide a secretory function as well as an intracellular digestive compartment.Citation48
Using a specific molecular marker of autophagy, the CrATG8 protein, we demonstrate here that this catabolic process is upregulated in the highly vacuolated mutant lts1-204 under heterotrophic growth (). The question arises as to why autophagy is increased in this carotenoid-less mutant in the absence of light. We found that ROS production is enhanced in lts1-204 cells grown in the dark, which connects autophagy activation to the level of ROS in carotenoid-depleted cells. Additional experimental evidence strongly supported this model. First, exposure of lts1-204 cells to light, a lethal condition for this mutant,Citation19 resulted in a further increase in ROS and autophagy () due to the absence of protective carotenoids. Second, treatment of Chlamydomonas cells with norflurazon efficiently reduced the level of major carotenoids both in light and dark conditions although the herbicide had a stimulatory effect on autophagy only in light, when ROS are strongly generated (). It has been reported that norflurazon at low concentration stimulates the formation of lipid hydroperoxides and leads to growth arrest and cell death in Chlamydomonas cells exposed to high light.Citation49,Citation50 Our results show that norflurazon at low or high concentrations trigger autophagy in standard light (and Fig. S1), revealing the activation of prosurvival cellular mechanisms to reduce the negative effects of this stress. Third, experiments performed with wild-type and npq1 lor1 mutant cells in excess light also sustained a link between photo-oxidative damage, ROS production and autophagy activation. High light stress is associated with the generation of ROS and promotes the conversion of violaxanthin to zeaxanthin by de-epoxidation in the xanthophyll cycleCitation39 (). Zeaxanthin accumulates in the LHCs and prevents photo-oxidative damage to thylakoids through thermal dissipation of part of the excess excitation energy in plants and algae.Citation9,Citation51 In this study, a transient induction of autophagy was detected when wild-type cells were shifted from dim light to high light, which indicates that this process participates in the cellular adaptation to this stress (). Accordingly, we found that ROS were more abundant under excess light conditions (). The npq1 lor1 mutant lacks the protective xanthophylls zeaxanthin and lutein, and as a result of this deficiency these cells show a severe lack of NPQ, undergo irreversible bleaching and accumulate ROS upon exposure to high light.Citation9,Citation52 In agreement with these findings, we found in our study that npq1 lor1 mutant cells exhibited increased constitutive autophagy in dim light and a stronger and permanent induction in response to high light (). This is in contrast to npq1 and lor1 mutants where autophagy responds to light stress as in wild-type cells () likely due to the redundant role of lutein/loroxanthin (absent in lor1 mutant) and antheraxanthin/zeaxanthin (absent in npq1 mutant) in protecting Chlamydomonas cells from photo-oxidative damage.Citation9 Finally, MV triggered autophagy associated to ROS production in Chlamydomonas (). During illumination, MV intercepts electrons from PSI and donates them to oxygen, yielding different types of ROS.Citation41 Our results strongly suggest that, as in carotenoid-deficient cells, autophagy activation occurred to overcome the toxic effect of ROS generated in the chloroplast but not as a direct consequence of photosynthesis inhibition. Induction of autophagy by MV is thus strongly dependent on light as demonstrated by the reduced effect of the herbicide in dark. However, a moderate upregulation of autophagy could be observed when Chlamydomonas cells were treated with MV in the dark. In plants, it has been shown that MV activates autophagy.Citation20 Nevertheless, these experiments were performed in the light since darkness results in nutrient limitation and hence autophagy induction in plants.Citation30,Citation53 In an early study, it was proposed that MV may also accept electrons in the absence of light from the respiratory electron transport chain in the plant mitochondria, and hence forming superoxide anions within this organelle.Citation54 It is therefore likely that MV has a similar effect on the mitochondria of Chlamydomonas cells and that the mild induction of autophagy detected in the dark is due to the generation of ROS at this site of the cell.
Uncontrolled accumulation of ROS in the chloroplast caused by carotenoid deficiency has negative consequences on the functionality and structure of the organelle. Photosynthetic reaction centers fail to accumulate in the absence of carotenoids and consequently chlorophyll is degraded and thylakoid synthesis is aborted.Citation34,Citation55 The lts1-204 mutant is apparently unable to form a pyrenoid body and Rubisco assumes a diffuse localization throughout the plastid in these cells.Citation34 Autophagy might be induced in carotenoid-depleted cells as a primary response to prevent further damage caused by toxic products. In consonance with this hypothesis, it has been proposed that the large number of vacuoles in lts1-204 cells may arise as an attempt to recycle components of aberrant thylakoids.Citation34 Our present study demonstrates that autophagy is constitutively active in this mutant and establishes a strong correlation between increased vacuole content and autophagy induction in Chlamydomonas. Transfer of stromal material to a class of vacuoles distinct to contractile vacuoles has been reported in Chlamydomonas cells grown in autotrophic conditions.Citation46 Ultrastructure of these cells revealed a continuity of the outer membrane of the chloroplast envelope and vacuolar membranes, suggesting that relocation of stromal material to vacuoles is a pathway for degradation.Citation46 Partial mobilization of chloroplasts to the vacuole by autophagy has been demonstrated in plants under carbon-limited conditions caused by darkness.Citation35-Citation37 Chloroplast degradation occurs via Rubisco-containing bodies (RCBs), a kind of autophagic body containing chloroplast stroma.Citation35 However, it is unknown whether a specific stress in the chloroplast caused by reduced carotenoid contents may trigger autophagy and chloroplast degradation in plants via RCBs. Unlike plants, Chlamydomonas cells have a single chloroplast and its degradation would lead to cell death. Our results do not support a direct correlation between autophagy activation by chloroplast oxidative damage and the degradation of chloroplast proteins. Carotenoid-deficient lts1-204 cells displayed constitutive autophagy but RbcL abundance was similar to the one observed in wild-type cells (). Similarly, high light stress in wild-type or npq1 lor1 cells did not cause a significant reduction in RbcL levels despite autophagy is induced under these conditions (and). However, severe oxidative stress caused by prolonged NF or MV treatments led to a pronounced decrease in RbcL abundance in the light but not in the dark (and). These findings indicated that autophagy might be involved in chloroplast protein remobilization but it is not the only mechanism for Rubisco degradation, in strong agreement with previous observations in plants reporting degradation of Rubisco subunits in autophagy deficient mutants.Citation30,Citation37 Alternatively, it is also possible that the increased autophagy found in carotenoid-depleted Chlamydomonas cells mainly participates in the bulk remobilization of cytoplasmic components that become damaged by excess ROS generated in the chloroplast instead of the direct degradation of this organelle.
ROS-generating NADPH oxidases have been involved in autophagy regulation upon nutrient and salt stress in plants, although other conditions such as osmotic stress cause autophagy induction in a NADPH oxidase-independent manner, indicating that not all autophagic processes are regulated through ROS generated by these enzymes.Citation43 Our study shows that carotenoid deficiency resulted in ROS production in the chloroplast of Chlamydomonas cells, which in turn activate autophagy. Experiments performed with the NADPH oxidase inhibitor DPI established a functional link between ROS and autophagy induction, and revealed that these enzymes might participate in autophagy activation upon chloroplast photo-oxidative damage but are not main components of this signaling pathway (). These results support different regulatory pathways for autophagy activation in response to different stimuli in Chlamydomonas, and are in consonance with the finding that lack of AtRbohD (the most highly expressed Arabidopsis NADPH oxidaseCitation42) or AtRbohF NADPH oxidases had little or no impact on chloroplast ROS accumulation in plants.Citation56 ROS play a multitude of signaling roles in different organisms including plantsCitation57 and efficiently transmit signals within and across different cells. It is thus tempting to speculate that chloroplast ROS generated in carotenoid-deficient cells may signal to plasma membrane NADPH oxidases to regulate autophagy induction although this is at present unknown.
Figure 6. Inhibition of NADPH activity partially suppressed autophagy induction upon carotenoid deficiency. (A) Wild-type Chlamydomonas cells were grown in TAP medium to log phase under standard light conditions and treated with 20 µM NF for 24 h to inhibit carotenoid deficiency. DPI (20 µM) or drug vehicle (C) was then added for 4 h to inhibit NADPH oxidase activity. Autophagy was monitored by western blot analysis with anti-CrATG8 antibody. (B) Oxidative stress was induced in wild-type Chlamydomonas cells with 1 µM MV or 1 mM H2O2 for 8 h, and then DPI (20 µM) or drug vehicle (C) was added to inhibit NADPH oxidase activity. Cells were collected and processed for western blot analysis.
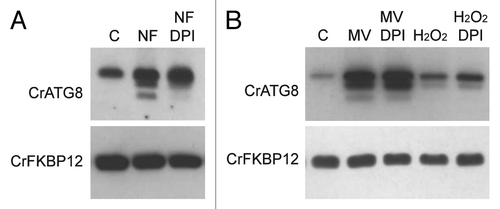
Accumulating evidence indicates that ROS play an important role in the activation of autophagy in mammalian cells.Citation58,Citation59 As the main source of ROS in nonphotosynthetic organisms, the mitochondria can be damaged by the accumulation of ROS. In this case, nonfunctional mitochondria are targeted for degradation via a specific form of autophagy known as mitophagy.Citation60 ROS can also act directly on components of the autophagy pathway to control this catabolic process. The cysteine protease ATG4, which catalyzes both the maturation of ATG8 (LC3 in mammals) at its C-terminus and its delipidation and subsequent release from the autophagosome membrane,Citation61,Citation62 has been identified as a direct target for oxidation by H2O2 in humans.Citation63 ROS may also promote autophagy by stimulating the activation of signaling pathways that control the initial steps of this process, such as the AMPK-TSC1/2-mTOR cascade.Citation64 TOR is a major regulator of autophagy and is widely conserved from yeast to plants and mammals.Citation26 The recent identification of TOR as an upstream regulator of autophagy in plants and algaeCitation33,Citation65 might suggest a role of this signaling pathway in the activation of autophagy by specific stresses generated in the chloroplast. Alternatively, damages in the chloroplast could be transmitted to the autophagic machinery through a different pathway, and future studies should concentrate to explore this hypothesis.
Materials and Methods
Strains and growth conditions
Chlamydomonas reinhardtii lts1-204, npq1, lor1 and npq1 lor1 mutants and the isogenic wild-type 4A+ strain were obtained from the Chlamydomonas Center culture collection (www.chlamy.org/strains.html). Cells were grown as described by HarrisCitation66 under continuous illumination at 25°C. According to experimental designs, cells were grown under dark conditions, standard light illumination (20–30 µE m−2 s−1) or high light illumination (1200 µE m−2 s−1). The npq1 lor1 mutant was maintained and grown at dim light (5–10 µE m−2 s−1) to prevent any light damage. If required, TRIS-acetate phosphate medium (TAP) was solidified with 1.2% Bacto agar (Difco, 214010). When specified, cells were treated with 20 µM norflurazon (Sigma, PS1044) from a 10 mM stock in methanol, 1 mM H2O2 (Panreac, 141077.1410) from a 1M stock in water, 1 µM methyl viologen (Sigma, 85,617-7) from a 10 mM stock in water or 20 µM DPI from a 25 mM stock in DMSO (Sigma, D2926). To inhibit photosynthesis, cells were treated with 10 µM DCMU (Sigma, D7763) or 2 µM DBMIB (Sigma, 271993).
Protein preparation
Chlamydomonas cells from liquid cultures were collected by centrifugation (4000 × g, 5 min), washed twice in lysis buffer [50 mM TRIS-HCl (pH 7.5)] and resuspended in a minimal volume of the same solution. Cells were lysed by two cycles of slow freezing to -80°C followed by thawing to room temperature. The soluble cell extract was separated from the insoluble fraction by centrifugation (15000 × g, 15 min) at 4°C, and subsequently prepared for its analysis. Proteins were quantified with the Coomassie dye binding method (BioRad, 500-0006).
Western blotting
Total protein extracts were resolved by SDS-PAGE electrophoresis and transferred to nitrocellulose membrane for antibody binding. Immunoblots were visualized using horseradish peroxidase-conjugated secondary antibodies (Sigma, A6154) and the ECL Plus chemiluminescencent system (GE Healthcare, RPN2132). The Chlamydomonas anti-CrATG8 and anti-CrFKBP12 antibodies have been described previouslyCitation33,Citation67 and they were used to 1:2500 and 1:3000 dilutions, respectively. RbcL antibody was purchased from Agrisera (AS03 037) and used to 1:10000 dilution.
Fluorescence microscopy
Chlamydomonas cells were fixed and stained for IF microscopy as previously described.Citation68,Citation69 The primary antibody used was affinity-purified rabbit polyclonal anti-CrATG8 (1:500). For signal detection, a fluorescein isothiocyanate-labeled goat anti-rabbit antibody (1:500; Sigma, F4890) was used. Preparations were photographed on a DM6000B microscope (Leica) with an ORCA-ER camera (Hamamatsu) and processed with the Leica Application Suite Advanced Fluorescence software package (Leica). For the comparative analysis of the fluorescein isothiocyanate signal from different samples, the same acquisition time was fixed.
Carotenoid extraction and HPLC analysis
Samples from Chlamydomonas cultures were 30-fold concentrated and used for the carotenoids extraction with 80% of acetone (v/v). This step was repeated twice each sample and the extracts were finally dried with nitrogen gas and dissolved in 1ml of acetone. Then, the samples were centrifuged and analyzed by HPLC using a Waters Spherisorb ODS2 column (4.6 × 250 mm, 5 μm particle size) (Waters, PSS831915). The chromatographic method used was previously reportedCitation70 with some modifications. Pigments were eluted at a flow rate of 1.0 ml min−1 with a linear gradient from 100% solvent A [acetonitrile:methanol:0.1mM TRIS-HCl pH 8.0 (84:2:14)] to 100% solvent B [methanol:ethyl acetate (68:32)] for 20 min, followed by 7 min of 100% solvent B, then 1 min with a linear gradient from 100% solvent B to 100% solvent A, and finally 6 min in 100% solvent A. Pigments were detected at 450 nm using a Waters 2996 photodiode array detector. Identification of carotenoids was achieved by the comparison of the individual characteristic absorption spectrum and the retention time with known standards. The quantification was performed using a calibration curve generated with commercially available carotenoids standards from Sigma and DHI.
Dry weight determinations
Dry weight (DW) was determined by filtering an exact volume of microalgae culture (5 ml) on pretared glass-fiber filters GF/F (Whatman, 1822-915). The filters were washed three times with 0.5 M ammonium formate solution to remove salts and dried at 80°C for 24 h. The dried filters were weighed in an analytical balance and the dry weight calculated by the difference.
ROS determination
The analysis of ROS was performed following the protocol previously described.Citation71 Total protein extracts from Chlamydomonas cells growing under different conditions were prepared as described above. 200–400 μg of total protein were used for ROS quantification in each sample. Each measurement was performed on two equal aliquots, one of them containing 100 mM ascorbate (Sigma, A5960) used as background signal. Samples were incubated for 15 min at 25°C. Then, 2′,7′-dichlorodihydrofluorescein diacetate (H2DCFDA) (Invitrogen, D-399) in dimethyl sulfoxide (DMSO) was added to a final concentration of 25 µM and incubated for 30 min at 30°C. Fluorescence was measured using a Cary Eclipse fluorescence spectrophotometer (Varian) with excitation/emission wavelengths set up to 485 and 525 nm, respectively. The ascorbate-intensive fluorescence background was subtracted from each experimental sample. Six independent measurements were performed for each sample, and the obtained values were expressed as relative fluorescence units per microgram of protein. Each experiment was performed at least four independent times.
Abbreviations: | ||
Chl | = | chlorophyll |
DBMIB | = | 2,5-dibromo-3-methyl-6-isopropyl-p-benzoquinone |
DCMU | = | 3-(3′,4′-dichlorophenyl)-1,1-dimethylurea |
DPI | = | diphenylene iodinium |
IF | = | immunofluorescence |
LHC | = | light harvesting complex |
MV | = | methyl viologen |
NF | = | norflurazon |
NPQ | = | nonphotochemical quenching |
PE | = | phosphatidylethanolamine |
PS | = | photosystem |
RCB | = | Rubisco-containing body |
ROS | = | reactive oxygen species |
TAP | = | tris-acetate-phosphate |
Additional material
Download Zip (79.4 KB)Acknowledgments
We thank the Chlamydomonas Center culture collection for providing Chlamydomonas strains and in particular to Krishna Niyogi and Arthur Grossman for making carotenoid mutant strains available to the research community. This work was supported by the Spanish Ministry of Science and Innovation (grant BFU2009-07368 to J.L.C.).
Disclosure of Potential Conflicts of Interest
No potential conflicts of interest were disclosed.
References
- Murchie EH, Niyogi KK. Manipulation of photoprotection to improve plant photosynthesis. Plant Physiol 2011; 155:86 - 92; http://dx.doi.org/10.1104/pp.110.168831; PMID: 21084435
- Rochaix JD. Assembly of the photosynthetic apparatus. Plant Physiol 2011; 155:1493 - 500; http://dx.doi.org/10.1104/pp.110.169839; PMID: 21239619
- Rochaix JD. Regulation of photosynthetic electron transport. Biochim Biophys Acta 2011; 1807:375 - 83; http://dx.doi.org/10.1016/j.bbabio.2010.11.010; PMID: 21118674
- Grossman AR, Lohr M, Im CS. Chlamydomonas reinhardtii in the landscape of pigments. Annu Rev Genet 2004; 38:119 - 73; http://dx.doi.org/10.1146/annurev.genet.38.072902.092328; PMID: 15568974
- Lohr M. Carotenoids. In: Stern DB, ed. The Chlamydomonas Sourcebook Organellar and Metabolic Processes. Amsterdam: Academic Press, 2009:799-817.
- Boussiba S. Carotenogenesisin the green alga Haematococcus pluvialis: cellular physiology and stress response. Physiol Plant 2000; 108:111 - 7; http://dx.doi.org/10.1034/j.1399-3054.2000.108002111.x
- Del Campo JA, Garcia-Gonzalez M, Guerrero MG. Outdoor cultivation of microalgae for carotenoid production: current state and perspectives. Appl Microbiol Biotechnol 2007; 74:1163 - 74; http://dx.doi.org/10.1007/s00253-007-0844-9; PMID: 17277962
- Havaux M, Niyogi KK. The violaxanthin cycle protects plants from photooxidative damage by more than one mechanism. Proc Natl Acad Sci USA 1999; 96:8762 - 7; http://dx.doi.org/10.1073/pnas.96.15.8762; PMID: 10411949
- Niyogi KK, Bjorkman O, Grossman AR. The roles of specific xanthophylls in photoprotection. Proc Natl Acad Sci USA 1997; 94:14162 - 7; http://dx.doi.org/10.1073/pnas.94.25.14162; PMID: 9391170
- Peterman EJ, Gradinaru CC, Calkoen F, Borst JC, van Grondelle R, van Amerongen H. Xanthophylls in light-harvesting complex II of higher plants: light harvesting and triplet quenching. Biochemistry 1997; 36:12208 - 15; http://dx.doi.org/10.1021/bi9711689; PMID: 9315858
- Sarry JE, Montillet JL, Sauvaire Y, Havaux M. The protective function of the xanthophyll cycle in photosynthesis. FEBS Lett 1994; 353:147 - 50; http://dx.doi.org/10.1016/0014-5793(94)01028-5; PMID: 7926040
- Armstrong GA, Hearst JE. Carotenoids 2: Genetics and molecular biology of carotenoid pigment biosynthesis. FASEB J 1996; 10:228 - 37; PMID: 8641556
- Cazzonelli CI, Pogson BJ. Source to sink: regulation of carotenoid biosynthesis in plants. Trends Plant Sci 2010; 15:266 - 74; http://dx.doi.org/10.1016/j.tplants.2010.02.003; PMID: 20303820
- Cunningham FX, Gantt E. Genes and Enzymes of Carotenoid Biosynthesis in Plants. Annu Rev Plant Physiol Plant Mol Biol 1998; 49:557 - 83; http://dx.doi.org/10.1146/annurev.arplant.49.1.557; PMID: 15012246
- Hirschberg J. Carotenoid biosynthesis in flowering plants. Curr Opin Plant Biol 2001; 4:210 - 8; http://dx.doi.org/10.1016/S1369-5266(00)00163-1; PMID: 11312131
- Sager R, Zalokar M. Pigments and photosynthesis in a carotenoid-deficient mutant of Chlamydomonas. Nature 1958; 182:98 - 100; http://dx.doi.org/10.1038/182098a0; PMID: 13566193
- Harris EH. Chlamydomonas as a Model Organism. Annu Rev Plant Physiol Plant Mol Biol 2001; 52:363 - 406; http://dx.doi.org/10.1146/annurev.arplant.52.1.363; PMID: 11337403
- Waters MT, Langdale JA. The making of a chloroplast. EMBO J 2009; 28:2861 - 73; http://dx.doi.org/10.1038/emboj.2009.264; PMID: 19745808
- McCarthy SS, Kobayashi MC, Niyogi KK. White mutants of Chlamydomonas reinhardtii are defective in phytoene synthase. Genetics 2004; 168:1249 - 57; http://dx.doi.org/10.1534/genetics.104.030635; PMID: 15579683
- Xiong Y, Contento AL, Nguyen PQ, Bassham DC. Degradation of oxidized proteins by autophagy during oxidative stress in Arabidopsis. Plant Physiol 2007; 143:291 - 9; http://dx.doi.org/10.1104/pp.106.092106; PMID: 17098847
- Nakatogawa H, Suzuki K, Kamada Y, Ohsumi Y. Dynamics and diversity in autophagy mechanisms: lessons from yeast. Nat Rev Mol Cell Biol 2009; 10:458 - 67; http://dx.doi.org/10.1038/nrm2708; PMID: 19491929
- Xie Z, Klionsky DJ. Autophagosome formation: core machinery and adaptations. Nat Cell Biol 2007; 9:1102 - 9; http://dx.doi.org/10.1038/ncb1007-1102; PMID: 17909521
- Tsukada M, Ohsumi Y. Isolation and characterization of autophagy-defective mutants of Saccharomyces cerevisiae.. FEBS Lett 1993; 333:169 - 74; http://dx.doi.org/10.1016/0014-5793(93)80398-E; PMID: 8224160
- Bassham DC. Plant autophagy–more than a starvation response. Curr Opin Plant Biol 2007; 10:587 - 93; http://dx.doi.org/10.1016/j.pbi.2007.06.006; PMID: 17702643
- Bassham DC, Laporte M, Marty F, Moriyasu Y, Ohsumi Y, Olsen LJ, et al. Autophagy in development and stress responses of plants. Autophagy 2006; 2:2 - 11; PMID: 16874030
- Díaz-Troya S, Perez-Perez ME, Florencio FJ, Crespo JL. The role of TOR in autophagy regulation from yeast to plants and mammals. Autophagy 2008; 4:851 - 65; PMID: 18670193
- Bassham DC. Function and regulation of macroautophagy in plants. Biochim Biophys Acta 2009; 1793:1397 - 403; http://dx.doi.org/10.1016/j.bbamcr.2009.01.001; PMID: 19272302
- Ichimura Y, Kirisako T, Takao T, Satomi Y, Shimonishi Y, Ishihara N, et al. A ubiquitin-like system mediates protein lipidation. Nature 2000; 408:488 - 92; http://dx.doi.org/10.1038/35044114; PMID: 11100732
- Contento AL, Xiong Y, Bassham DC. Visualization of autophagy in Arabidopsis using the fluorescent dye monodansylcadaverine and a GFP-AtATG8e fusion protein. Plant J 2005; 42:598 - 608; http://dx.doi.org/10.1111/j.1365-313X.2005.02396.x; PMID: 15860017
- Thompson AR, Doelling JH, Suttangkakul A, Vierstra RD. Autophagic nutrient recycling in Arabidopsis directed by the ATG8 and ATG12 conjugation pathways. Plant Physiol 2005; 138:2097 - 110; http://dx.doi.org/10.1104/pp.105.060673; PMID: 16040659
- Yoshimoto K, Hanaoka H, Sato S, Kato T, Tabata S, Noda T, et al. Processing of ATG8s, ubiquitin-like proteins, and their deconjugation by ATG4s are essential for plant autophagy. Plant Cell 2004; 16:2967 - 83; http://dx.doi.org/10.1105/tpc.104.025395; PMID: 15494556
- Pérez-Pérez ME, Crespo JL. Autophagy in the model alga Chlamydomonas reinhardtii.. Autophagy 2010; 6:562 - 3; http://dx.doi.org/10.4161/auto.6.4.11822; PMID: 20404489
- Pérez-Pérez ME, Florencio FJ, Crespo JL. Inhibition of target of rapamycin signaling and stress activate autophagy in Chlamydomonas reinhardtii.. Plant Physiol 2010; 152:1874 - 88; http://dx.doi.org/10.1104/pp.109.152520; PMID: 20107021
- Inwood W, Yoshihara C, Zalpuri R, Kim KS, Kustu S. The ultrastructure of a Chlamydomonas reinhardtii mutant strain lacking phytoene synthase resembles that of a colorless alga. Mol Plant 2008; 1:925 - 37; http://dx.doi.org/10.1093/mp/ssn046; PMID: 19825593
- Ishida H, Yoshimoto K, Izumi M, Reisen D, Yano Y, Makino A, et al. Mobilization of rubisco and stroma-localized fluorescent proteins of chloroplasts to the vacuole by an ATG gene-dependent autophagic process. Plant Physiol 2008; 148:142 - 55; http://dx.doi.org/10.1104/pp.108.122770; PMID: 18614709
- Izumi M, Wada S, Makino A, Ishida H. The autophagic degradation of chloroplasts via rubisco-containing bodies is specifically linked to leaf carbon status but not nitrogen status in Arabidopsis. Plant Physiol 2010; 154:1196 - 209; http://dx.doi.org/10.1104/pp.110.158519; PMID: 20807997
- Wada S, Ishida H, Izumi M, Yoshimoto K, Ohsumi Y, Mae T, et al. Autophagy plays a role in chloroplast degradation during senescence in individually darkened leaves. Plant Physiol 2009; 149:885 - 93; http://dx.doi.org/10.1104/pp.108.130013; PMID: 19074627
- Sandmann G, Albrecht M. Accumulation of colorless carotenes and derivatives during interaction of bleaching herbicides with phytoene desaturation. Z Naturforsch C 1990; 45:487 - 91
- Li Z, Wakao S, Fischer BB, Niyogi KK. Sensing and responding to excess light. Annu Rev Plant Biol 2009; 60:239 - 60; http://dx.doi.org/10.1146/annurev.arplant.58.032806.103844; PMID: 19575582
- Finazzi G, Furia A, Barbagallo RP, Forti G. State transitions, cyclic and linear electron transport and photophosphorylation in Chlamydomonas reinhardtii.. Biochim Biophys Acta 1999; 1413:117 - 29; http://dx.doi.org/10.1016/S0005-2728(99)00089-4; PMID: 10556624
- Babbs CF, Pham JA, Coolbaugh RC. Lethal hydroxyl radical production in paraquat-treated plants. Plant Physiol 1989; 90:1267 - 70; http://dx.doi.org/10.1104/pp.90.4.1267; PMID: 16666920
- Suzuki N, Miller G, Morales J, Shulaev V, Torres MA, Mittler R. Respiratory burst oxidases: the engines of ROS signaling. Curr Opin Plant Biol 2011; http://dx.doi.org/10.1016/j.pbi.2011.07.014; PMID: 21862390
- Liu Y, Xiong Y, Bassham DC. Autophagy is required for tolerance of drought and salt stress in plants. Autophagy 2009; 5:954 - 63; http://dx.doi.org/10.4161/auto.5.7.9290; PMID: 19587533
- Anderson A, Bothwell JH, Laohavisit A, Smith AG, Davies JM. NOX or not? Evidence for algal NADPH oxidases. Trends Plant Sci 2011; 16:579 - 81; http://dx.doi.org/10.1016/j.tplants.2011.09.003; PMID: 22000495
- Luykx P, Hoppenrath M, Robinson D. Structure and behaviour of contractile vacuoles in Chlamydomonas reinhardtii.. Protoplasma 1997; 198:73 - 84; http://dx.doi.org/10.1007/BF01282133
- Park H, Eggink L, Roberson R, Hoober J. Transfer of proteins from the chloroplast to vacuoles in Chlamydomonas reinhardtii (Chlorophyta): a pathway for degradation. J Phycol 1999; 35:528 - 38; http://dx.doi.org/10.1046/j.1529-8817.1999.3530528.x
- Sager R, Palade GE. Structure and development of the chloroplast in Chlamydomonas. I. The normal green cell. J Biophys Biochem Cytol 1957; 3:463 - 88; http://dx.doi.org/10.1083/jcb.3.3.463; PMID: 13438931
- Komine Y, Eggink LL, Park H, Hoober JK. Vacuolar granules in Chlamydomonas reinhardtii: polyphosphate and a 70-kDa polypeptide as major components. Planta 2000; 210:897 - 905; http://dx.doi.org/10.1007/s004250050695; PMID: 10872220
- Fischer BB, Krieger-Liszkay A, Hideg E, Snyrychova I, Wiesendanger M, Eggen RI. Role of singlet oxygen in chloroplast to nucleus retrograde signaling in Chlamydomonas reinhardtii.. FEBS Lett 2007; 581:5555 - 60; http://dx.doi.org/10.1016/j.febslet.2007.11.003; PMID: 17997989
- Fischer BB, Rufenacht K, Dannenhauer K, Wiesendanger M, Eggen RI. Multiple stressor effects of high light irradiance and photosynthetic herbicides on growth and survival of the green alga Chlamydomonas reinhardtii.. Environ Toxicol Chem 2010; 29:2211 - 9; http://dx.doi.org/10.1002/etc.264; PMID: 20872684
- Niyogi KK, Grossman AR, Bjorkman O. Arabidopsis mutants define a central role for the xanthophyll cycle in the regulation of photosynthetic energy conversion. Plant Cell 1998; 10:1121 - 34; PMID: 9668132
- Baroli I, Gutman BL, Ledford HK, Shin JW, Chin BL, Havaux M, et al. Photo-oxidative stress in a xanthophyll-deficient mutant of Chlamydomonas. J Biol Chem 2004; 279:6337 - 44; http://dx.doi.org/10.1074/jbc.M312919200; PMID: 14665619
- Chung T, Suttangkakul A, Vierstra RD. The ATG autophagic conjugation system in maize: ATG transcripts and abundance of the ATG8-lipid adduct are regulated by development and nutrient availability. Plant Physiol 2009; 149:220 - 34; http://dx.doi.org/10.1104/pp.108.126714; PMID: 18790996
- Bowler C, Slooten L, Vandenbranden S, De Rycke R, Botterman J, Sybesma C, et al. Manganese superoxide dismutase can reduce cellular damage mediated by oxygen radicals in transgenic plants. EMBO J 1991; 10:1723 - 32; PMID: 2050109
- Herrin DL, Battey JF, Greer K, Schmidt GW. Regulation of chlorophyll apoprotein expression and accumulation. Requirements for carotenoids and chlorophyll. J Biol Chem 1992; 267:8260 - 9; PMID: 1373722
- Miura E, Kato Y, Sakamoto W. Reactive oxygen species derived from impaired quality control of photosystem II are irrelevant to plasma-membrane NADPH oxidases. Plant Signal Behav 2010; 5:264 - 6; http://dx.doi.org/10.4161/psb.5.3.10604; PMID: 20023407
- Mittler R, Vanderauwera S, Suzuki N, Miller G, Tognetti VB, Vandepoele K, et al. ROS signaling: the new wave?. Trends Plant Sci 2011; 16:300 - 9; http://dx.doi.org/10.1016/j.tplants.2011.03.007; PMID: 21482172
- Filomeni G, Desideri E, Cardaci S, Rotilio G, Ciriolo MR. Under the ROS...thiol network is the principal suspect for autophagy commitment. Autophagy 2010; 6:999 - 1005; http://dx.doi.org/10.4161/auto.6.7.12754; PMID: 20639698
- Scherz-Shouval R, Elazar Z. Regulation of autophagy by ROS: physiology and pathology. Trends Biochem Sci 2011; 36:30 - 8; http://dx.doi.org/10.1016/j.tibs.2010.07.007; PMID: 20728362
- Wang K, Klionsky DJ. Mitochondria removal by autophagy. Autophagy 2011; 7:297 - 300; http://dx.doi.org/10.4161/auto.7.3.14502; PMID: 21252623
- Kim J, Huang WP, Klionsky DJ. Membrane recruitment of Aut7p in the autophagy and cytoplasm to vacuole targeting pathways requires Aut1p, Aut2p, and the autophagy conjugation complex. J Cell Biol 2001; 152:51 - 64; http://dx.doi.org/10.1083/jcb.152.1.51; PMID: 11149920
- Kirisako T, Ichimura Y, Okada H, Kabeya Y, Mizushima N, Yoshimori T, et al. The reversible modification regulates the membrane-binding state of Apg8/Aut7 essential for autophagy and the cytoplasm to vacuole targeting pathway. J Cell Biol 2000; 151:263 - 76; http://dx.doi.org/10.1083/jcb.151.2.263; PMID: 11038174
- Scherz-Shouval R, Shvets E, Fass E, Shorer H, Gil L, Elazar Z. Reactive oxygen species are essential for autophagy and specifically regulate the activity of Atg4. EMBO J 2007; 26:1749 - 60; http://dx.doi.org/10.1038/sj.emboj.7601623; PMID: 17347651
- Alexander A, Cai SL, Kim J, Nanez A, Sahin M, MacLean KH, et al. ATM signals to TSC2 in the cytoplasm to regulate mTORC1 in response to ROS. Proc Natl Acad Sci USA 2010; 107:4153 - 8; http://dx.doi.org/10.1073/pnas.0913860107; PMID: 20160076
- Liu Y, Bassham DC. TOR is a negative regulator of autophagy in Arabidopsis thaliana.. PLoS ONE 2010; 5:e11883; http://dx.doi.org/10.1371/journal.pone.0011883; PMID: 20686696
- Harris EH. The Chlamydomonas Sourcebook. San Diego: Academic Press, 1989.
- Crespo JL, Diaz-Troya S, Florencio FJ. Inhibition of target of rapamycin signaling by rapamycin in the unicellular green alga Chlamydomonas reinhardtii.. Plant Physiol 2005; 139:1736 - 49; http://dx.doi.org/10.1104/pp.105.070847; PMID: 16299168
- Cole DG, Diener DR, Himelblau AL, Beech PL, Fuster JC, Rosenbaum JL. Chlamydomonas kinesin-II-dependent intraflagellar transport (IFT): IFT particles contain proteins required for ciliary assembly in Caenorhabditis elegans sensory neurons. J Cell Biol 1998; 141:993 - 1008; http://dx.doi.org/10.1083/jcb.141.4.993; PMID: 9585417
- Díaz-Troya S, Florencio FJ, Crespo JL. Target of rapamycin and LST8 proteins associate with membranes from the endoplasmic reticulum in the unicellular green alga Chlamydomonas reinhardtii.. Eukaryot Cell 2008; 7:212 - 22; http://dx.doi.org/10.1128/EC.00361-07; PMID: 18039939
- Baroli I, Do AD, Yamane T, Niyogi KK. Zeaxanthin accumulation in the absence of a functional xanthophyll cycle protects Chlamydomonas reinhardtii from photooxidative stress. Plant Cell 2003; 15:992 - 1008; http://dx.doi.org/10.1105/tpc.010405; PMID: 12671093
- Joo JH, Wang S, Chen JG, Jones AM, Fedoroff NV. Different signaling and cell death roles of heterotrimeric G protein alpha and beta subunits in the Arabidopsis oxidative stress response to ozone. Plant Cell 2005; 17:957 - 70; http://dx.doi.org/10.1105/tpc.104.029603; PMID: 15705948