Abstract
In early pregnancy, trophoblasts and the fetus experience hypoxic and low-nutrient conditions; nevertheless, trophoblasts invade the uterine myometrium up to one third of its depth and migrate along the lumina of spiral arterioles, replacing the maternal endothelial lining. Here, we showed that autophagy, an intracellular bulk degradation system, occurred in extravillous trophoblast (EVT) cells under hypoxia in vitro and in vivo. An enhancement of autophagy was observed in EVTs in early placental tissues, which suffer from physiological hypoxia. The invasion and vascular remodeling under hypoxia were significantly reduced in autophagy-deficient EVT cells compared with wild-type EVT cells. Interestingly, soluble endoglin (sENG), which increased in sera in preeclamptic cases, suppressed EVT invasion by inhibiting autophagy. The sENG-inhibited EVT invasion was recovered by TGFB1 treatment in a dose-dependent manner. A high dose of sENG inhibited the vascular construction by EVT cells and human umbilical vein endothelial cells (HUVECs), meanwhile a low dose of sENG inhibited the replacement of HUVECs by EVT cells. A protein selectively degraded by autophagy, SQSTM1, accumulated in EVT cells in preeclamptic placental biopsy samples showing impaired autophagy. This is the first report showing that impaired autophagy in EVT contributes to the pathophysiology of preeclampsia.
Introduction
Preeclampsia, characterized by hypertension and proteinuria after 20 weeks of gestation, complicates 3% to 5% of all pregnancies worldwide, and is the leading cause of maternal, fetal and neonatal mortality. Preeclampsia remains a major cause of maternal mortality (15% to 46%) and is responsible for a 5-fold increase in perinatal mortality.Citation1,Citation2 The World Health Organization has recognized the importance of preeclampsia by launching a global program to conquer preeclampsia-eclampsia.Citation3 Additionally, women with a history of preeclampsia and their offspring are at greater risk of developing cardiovascular disease later in life.Citation4
The current hypothesis regarding the etiology of preeclampsia is focused on inadequate trophoblast invasion and placentation.Citation2 Trophoblast stem cells differentiate into two cell types, villous trophoblasts and extravillous trophoblasts (EVTs) in humans. Invading trophoblasts called interstitial EVTs migrate into the decidualized endometrium and endovascular EVTs migrate along the lumina of spiral arterioles.Citation5 The invasion of EVTs into spiral arteries starts earlier in pregnancy and the endovascular trophoblastic cells aggregate in the lumen of the vessel forming the “trophoblastic plug,” to allow the growth of the embryo and placenta in a low-oxygen environment in the first stage of pregnancy.Citation6 This EVT-invasion anchors the fetus to the mother and creates the large-diameter and low-resistant vessels that carry blood to the intervillous space.Citation7 EVT invasion in humans is also much deeper than that in other mammalian species. Trophoblasts can invade into the maternal decidua under harsh conditions, such as low oxygen (2–5% O2) and low glucose concentrations (1 mM) until 11 weeks of gestation (Fig. S1a).Citation8-Citation11 As trophoblast invasion of the uterus proceeds, the hypoxia inducible factor (HIF) system plays a critical role in EVT functions.Citation7 After 12 weeks, endovascular EVTs invade the uterine spiral arteries, replace the endothelial cells, and participate in the degradation of tunica media smooth muscle cells. This remodeling of the spiral arteries is essential for proper placental perfusion to sustain fetal growth (Fig. S1b). Preeclampsia is considered to be caused by poor placentation, and a two-step process has been proposed.Citation2 The first preclinical stage, hypoplastic placentation, can be explained by the shallow invasion by trophoblast until 20 weeks of gestation. This impairment is closely related with a failure of vascular remodeling and decreased circulation in the early placenta, resulting in continuous placental ischemia after 20 weeks of gestation. The initial events leading to these changes remain little understood. At the second stage, a growing body of evidence is accumulating to show that releasing and genetic factors, soluble fms-related tyrosine kinase 1 (sFLT1), soluble endoglin (sENG), angiotensin II receptor, type 1 receptor agonistic autoantibody (AGTR1-AA) and catechol-O-methyltransferase (COMT) among others, are responsible for maternal endothelial dysfunction, causing the maternal signs of hypertension and proteinuria.Citation12-Citation17
Autophagy is a process for degrading cellular components under stress to maintain cellular homeostasis. In addition to its well-understood physiological role in recycling intracellular materials under starvation, there is growing evidence for the participation of autophagy in other cellular processes including cellular differentiation, tissue remodeling, growth control, adaptation to adverse environments and cellular immunity.Citation18-Citation21 Additionally, autophagy is essential for preimplantation development beyond the four- and eight-cell stages and blastocyst survival in mammals.Citation22,Citation23 Apart from the placental energy supply, autophagy helps neonates with the production of amino acids by autophagic degradation of ‘self’ proteins.Citation24 Some papers reported the expression of autophagy-related proteins in placentas; there is, however, no direct evidence that autophagy is involved in human placentation.Citation25,Citation26
We now report that autophagy was essential for EVT functions, invasion and vascular remodeling, under physiological hypoxia, which is necessary for the first stage of placentation, in vitro and in vivo. Furthermore, we first report that sENG, an exacerbation factor in the second stage of preeclampsia, is a physiologically active substance that inhibited autophagy in EVTs under hypoxia, resulting in a disruption of EVT functions. Additionally, the expression of SQSTM1, a hallmark of autophagic inhibition, was observed in EVTs in preeclampsia cases. These results suggested that autophagy may be essential in elucidating the pathophysiology of preeclampsia.
Results
Detection of autophagy induced in primary extravillous trophoblasts (EVTs) or EVT cell lines under hypoxia
To evaluate autophagy in EVTs, microtubule-associated protein 1 light chain 3 β (MAP1LC3B) staining was performed under hypoxia or normoxia. MAP1LC3B puncta were observed in the cytoplasm of primary EVT cells under hypoxia (2% O2; physiological O2 concentration in early pregnancy period); on the other hand, few MAP1LC3B puncta were observed in EVT under normoxia (). MAP1LC3B puncta were not seen in EVTs in early placental tissues at a shallower site from the fetus at the implantation site (), but were clearly seen at a deeper site from the fetus side () and around the spiral arteries (, arrows) in the implantation site. No such cells were seen at the villous trophoblast region (, arrowheads).
Figure 1. Detection of autophagy in EVTs from human subjects in early pregnancy. (a–d) Early chorionic and decidual tissues were obtained from patients who had undergone elective termination at 8 weeks of gestation, and villous explant cultures were performed on Matrigel under hypoxia, 2% oxygen tension (a), and normoxia, 20% oxygen tension (b). HLA-G, one of the markers for EVTs, was expressed on primary cultured trophoblasts in (c). DIC is shown in (d). Arrows indicate MAP1LC3B (LC3) dot formation, a marker of autophagic activation. (e–l) MAP1LC3B staining in normal pregnant subjects at 8 weeks of gestation (e). MAP1LC3B dot formation (g and h, arrows) was detected in the maternal decidua basalis, the implantation site, at a deeper site from the fetus side in square DEC (g), but not at a shallower site from the fetus in the implantation site (f) or in the villous trophoblast region (h, arrowheads). (f–h) Enlarged images of the white squares. (i–l) Double immunohistochemical staining for cytokeratin 7 (KRT7), an EVT marker, (k) and MAP1LC3B (j) revealed that cells with punctated MAP1LC3B were KRT7-positive EVTs (l). (m) Enlarged image of the KRT7-positive EVTs with MAP1LC3B puncta in (l). Hoechst33342 staining, nuclei staining, is shown in (i). Scale bar: 50 µm. STB, syncytiotrophoblasts; CTB, cytotrophoblasts; COL, cell column; DEC, decidua basalis. Electron microscopic images of autophagic structures in HTR8/SVneo cells under hypoxia (n and o). HTR8/SVneo cells, which were cultured under hypoxia for 4 h, were fixed and observed by electron microscopy (×20,000). The smooth endoplasmic reticulum, some destroyed organelles and autophagosomes (Ap) were present (n, arrow). The autophagosome (Ap) had come into contact with multiple vesicle bodies (Mb) (o, arrow). AL, autolysosome; M, mitochondria.
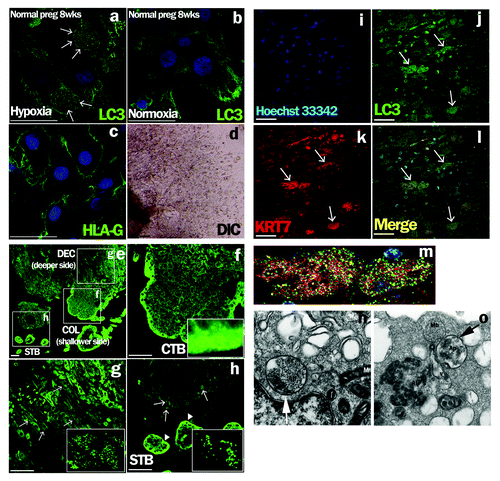
To confirm the occurrence of autophagy in the deeper invading EVT cells, double immunohistochemical staining was performed. MAP1LC3B puncta () were present in cytokeratin 7 (KRT7)-positive EVT cells () in the maternal decidua basalis at a deeper site from the fetus side at the implantation site (, arrows). An enlarged image of KRT7-positive EVT cells with MAP1LC3B puncta is shown in .
An electron microscopic analysis showed the accumulation of autophagosomes 0.5–1.5 μm in size, which are double-membrane structures containing undigested cytoplasmic materials including organelles (), in HTR8/SVneo cells under hypoxia for 4 h. The number of MAP1LC3B puncta was significantly increased under hypoxia as well as starvation in HTR8/SVneo cells at 24 h, compared with that under normoxia (, p = 0.005). The proportion of autophagic cells was increased over 50% from 4–24 h under hypoxia, and then gradually decreased (). In the western blot analysis, the amount of MAP1LC3B-II was increased under hypoxia, which induced HIF1A expression, and the band of MAP1LC3B-II was further augmented in the presence of E64d and pepstatin, protease inhibitors, under hypoxia (). Additionally, SQSTM1 expression, a biomarker for autophagy flux, was decreased in HTR8/SVneo cells under hypoxia, suggesting the activation of autophagy. Flow cytometry showed that the total amount of MAP1LC3B decreased in a time-dependent manner under hypoxia (). These results directly showed the enhancement of autophagy in EVT cells under hypoxia.
Figure 2. Activation of autophagy in EVT cells under hypoxia. (A) The number of MAP1LC3B (LC3) puncta in HTR8/SVneo cells, infected with the GFP-MAP1LC3B adenovirus vector, in the presence of E64d and pepstatin under 20% (Cont), starvation (Starved) or 2% oxygen tension (hypoxia) for 24 h. (B) The proportion of autophagic cells, identified as cells containing more than 5 MAP1LC3B puncta, among HTR8/SVneo cells in the presence of E64d and pepstatin under 2% oxygen tension for 48 h. (C) Western blots in HTR8/SVneo cells under normoxia and hypoxia (2% oxygen tension) are shown as follows: MAP1LC3B (LC3), HIF1A, SQSTM1 and TUBA1A. Expression of MAP1LC3B-II was examined in the presence or absence of E64d and pepstatin under normoxia and hypoxia (2% oxygen tension). Nor, normoxia; Hyp, hypoxia. (D) Intracellular MAP1LC3B levels determined by flow cytometry. The amount of MAP1LC3B was estimated at 4 and 24 h under 2% oxygen tension (hypoxia) in HTR8/SVneo cells.
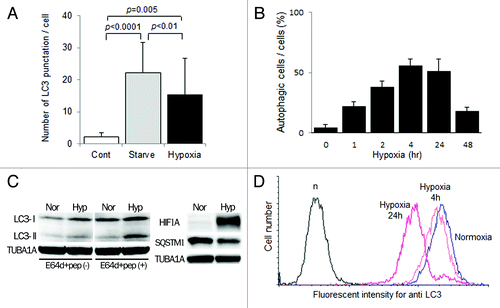
The role of autophagy in EVT cell functions under hypoxia
To clarify the specific role of autophagy in trophoblast functions, we constructed autophagy-deficient cells by stably transfecting ATG4BC74A, an inactive mutant of ATG4B, which inhibits autophagic degradation and lipidation of MAP1LC3B paralogs (see Materials and Methods).Citation27 As shown in and Figure S2a, no MAP1LC3B puncta were observed in the HTR8-ATG4BC74A cells and HchEpC1b-ATG4BC74A cells under hypoxia. Western blot analysis also showed a complete MAP1LC3B-II deficiency in the HTR8-ATG4BC74A cells (). The expression of SQSTM1, a protein selectively degraded by autophagy, in HTR8-mStrawberry, a control cell line that expressed only monomeric red fluorescent protein, showed a large decline under hypoxia, whereas no clear decline in SQSTM1 expression in HTR8-ATG4BC74A cells was observed (). Enzyme-linked immunosorbent assays showed a significant decrease of SQSTM1 expression under hypoxia in the HTR8-mStrawberry cells, but not in the HTR8-ATG4BC74A cells (). Flow cytometric analysis showed that the total amount of cytoplasmic MAP1LC3B did not decrease under hypoxia in the HchEpC1b-ATG4BC74A cells (Fig. S2b). In regard to cell growth and cell death, there were no differences between HchEpC1b-mStrawberry cells and HchEpC1b-ATG4BC74A cells (Fig. S2C and S2D).
Figure 3. Confirmation of autophagy-deficient cells. (A) Representative panels show mStrawberry (red), anti-MAP1LC3B (LC3) (green) and merged images (lower panels) in HTR8-ATG4BC74A (ATG4BC74A: left panels), an autophagy-deficient EVT cell line, and HTR8-mStrawberry cells (mStrawberry: right panels) under hypoxia for 24 h. (B) Western blots in HTR8-ATG4BC74A and -mStrawberry cells under normoxia and hypoxia (2% oxygen tension) are shown as follows: MAP1LC3B (LC3), SQSTM1, HIF1A and TUBA1A. (C) SQSTM1 expression in HTR8-ATG4BC74A and -mStrawberry cells under normoxia (white bars) and hypoxia (black bars) estimated by ELISA. Results were normalized per milligram of protein from cell lysate. (D) An in vitro invasion assay model before 12 weeks of gestation. Hypoxia (2% oxygen tension) was similar to the placental pO2 level before 12 weeks of gestation. Invasion assays were performed with HchEpC1b-ATG4BC74A or -mStrawberry cells under normoxia (gray bars) or hypoxia (black bars) for 48 h. The Y-axis indicates the number of invading cells. Data were normalized to 1 for normoxia at 24 h. (E) Three-dimensional invasion assays were performed with HchEpC1b-ATG4BC74A or -mStrawberry cells under normoxia (gray bars) and hypoxia (black bars) for 48 h. Photographs show the invading cells (mStrawberry) at the incision face of the gel, in which cells were seeded. The Y-axis indicates the depth of invasion from the surface of the gel. Scale bar: 300 μm.
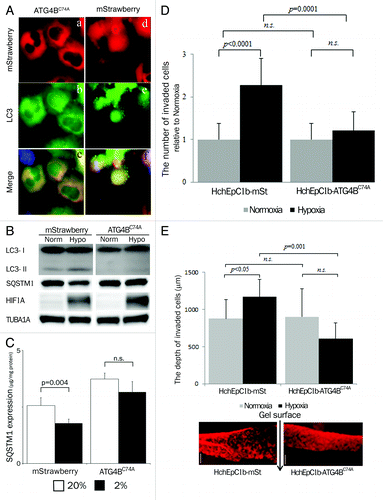
EVT invades the maternal decidua under 2% O2 for intact placentation before 12 weeks of gestation. To clarify the role of autophagy in trophoblast invasion, conventional invasion assays were performed under 2% O2, mimicking the physiological hypoxia in the early stages of pregnancy before 12 weeks of gestation (Fig. S1a). Under hypoxia the number of invading HchEpC1b-ATG4BC74A cells, an autophagy-deficient EVT cell line, was significantly decreased (p = 0.0001), compared with that of HchEpC1b-mStrawberry-expressing cells (). Similar results were obtained in HTR8-ATG4BC74A cells under hypoxia (Fig. S2f). In three-dimensional culture assays, the depth of HchEpC1b-ATG4BC74A cells was significantly shallower than that of HchEpC1b-mStrawberry cells under normoxia (, p = 0.001). In the next stage, endovascular EVTs invade the uterine spiral arteries and replace spiral artery endothelial cells after 12 weeks of gestation. Tube formation assays with EVT cells and human umbilical vascular endothelial cells (HUVECs), an in vitro model of vascular remodeling by EVT cells after 12 weeks of gestation, were performed under 8% oxygen tension (Fig. S1b).Citation2,Citation28,Citation29 This level of oxygen tension is thought to match the placental conditions after 12 weeks of gestation, the so-called “second wave,” in which vascular remodeling takes place.Citation11 Both HTR8-mStrawberry cells and HTR8-ATG4BC74A cells formed a tube structure with HUVECs at 12 h (), but they did not form a tube structure without HUVECs (). The tube structure was predominantly formed by HUVECs (green) in both settings at 6 h (), and there was no significant difference in the area occupied by HTR8 cells (red) in the total tube area formed by dual cells at 6 h (). In the culture with HTR8-mStrawberry cells and HUVECs, the tubes were mostly occupied by HTR8-mStrawberry cells (red) at 12 h or later (, blue line); meanwhile, the tubes were occupied by HUVECs (green) when HTR8-ATG4BC74A cells were cocultured with HUVECs (, red line). The proportion of HTR8 (red) in the total tube area was significantly higher in HTR8-mStrawberry cells than in HTR8-ATG4BC74A cells (, blue and red lines), suggesting that replacement of endothelial cells by EVT cells required autophagy. These results indicated that autophagy plays an important role in the endovascular interaction between EVT and endothelial cells.
Figure 4. Tube formation assay in the HUVEC and EVT coculture system. The tube formation assay was used as an in vitro model of vascular remodeling after 12 weeks of gestation. Hypoxia (8% oxygen tension) was similar to the placental pO2 level after 12 weeks of gestation. Tube formation assays with human umbilical vein endothelial cells (HUVECs, b, e, h, k, labeled with green) with HTR8-ATG4BC74A (g and j, labeled with red), an autophagy-deficient EVT cell line, or HTR8-mStrawberry cells (a and d, labeled with red) were performed under 8% oxygen tension for 6 or 12 h. Representative merged images are shown from cultures for 6 h (c and i) or 12 h (f and l). Representative figures in the lower panels show single cell cultures, HTR8-mStrawberry cells (m), HTR8-ATG4BC74A cells (n) or HUVECs (o). (p) The graph shows the HTR8 (red) area as a proportion of the total area (red and green). *p < 0.001: the proportion in HTR8-mStrawberry and HUVECs (blue line) was significantly higher than that in HTR8-ATG4BC74A and HUVECs (red line). Data are shown as the mean ± S. E. for three independent experiments. Scale bar: 300 μm.
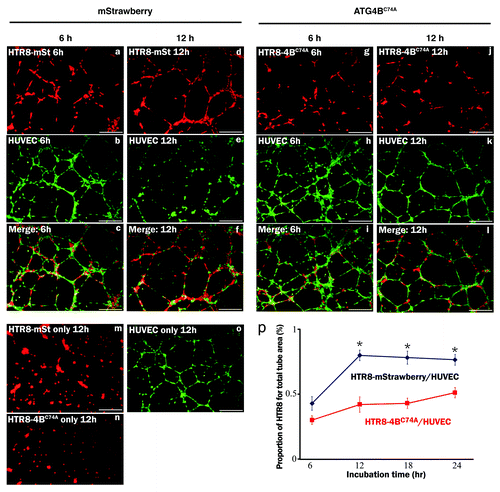
Soluble endoglin inhibits hypoxia-induced autophagy in EVT cells
Soluble endoglin (sENG) is known to cooperate with sFLT1 in the induction of severe preeclampsia in humans as well as a rat model.Citation16,Citation30 We examined whether preeclampsia-related substances such as sENG, sFLT1, transforming growth factor, β 1 (TGFB1) and tumor necrosis factor-α (TNF) affect autophagy in EVT under hypoxia (2% O2) (). Only sENG significantly inhibited the number of MAP1LC3B puncta in HchEpC1b-mStrawberry cells, the control cell line, under hypoxia at 24 h in the presence of E64d and pepstatin (, p = 0.007) or even in the absence of E64d and pepstatin (, p < 0.0001). sENG repressed the MAP1LC3B-II conversion under hypoxia in western blotting (), and flow cytometric analysis showed that hypoxia-induced MAP1LC3B degradation was diminished by sENG (). These results indicated that sENG inhibited the induction of autophagy in EVTs under hypoxia.
Figure 5. sENG inhibited autophagy in EVT cells under hypoxia. (A) The numbers of MAP1LC3B (LC3) puncta in HchEpC1b-mStrawberry cells in the presence of 5 ng/ml TNF, 5 ng/ml TGFB1, 100 ng/ml sENG or 5 μg/ml sFLT1 with E64d and pepstatin under 2% oxygen tension for 24 h. (B) The numbers of MAP1LC3B puncta in HchEpC1b-mStrawberry cells in the presence of 100 ng/ml sENG without E64d and pepstatin under 20% or 2% oxygen tension for 24 h. Representative figures show MAP1LC3B staining in HchEpC1b-mStrawberry cells in the presence or absence of 100 ng/ml sENG under 2% oxygen tension. (C) Western blot analysis showed that MAP1LC3B-II expression was not enhanced in hypoxic conditions in the presence of 100 ng/ml sENG. (D) The amount of MAP1LC3B in HchEpC1b-mStrawberry cells under 20% oxygen tension (normoxia), 2% oxygen tension (hypoxia) or 2% oxygen tension in the presence of 100 ng/ml sENG (hypoxia with sENG). n, negative control. Data are represented as means ± S.E. n.s., not significant. Statistical significance was calculated using the Mann-Whitney U test.
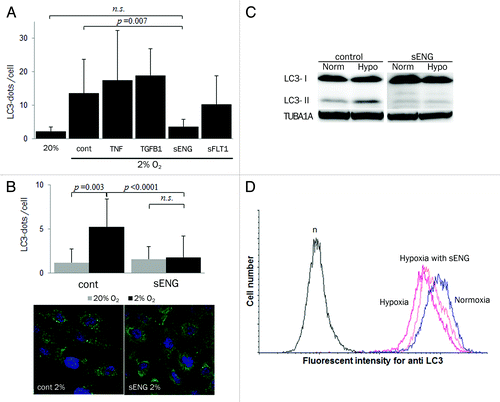
Disruption of EVT by soluble endoglin
To assess the effects of sENG-induced inhibition of autophagy on EVT functions, invasion and vascular remodeling assays were performed. sENG significantly decreased the number of invading HchEpC1b-mStrawberry cells, a control cell line, but not HchEpC1b-ATG4BC74A cells, an autophagy-deficient EVT cell line, under hypoxia (2% O2) at 48 h (, p = 0.0006). On the other hand, the number of HchEpC1b-mStrawberry cells under normoxia was not affected by sENG (Fig. S3a). The inhibition of HchEpC1b-mStrawberry cells by 100 ng/ml sENG under hypoxia was recovered by TGFB1 in a dose-dependent manner and recovered to the control level under hypoxia by an additional 20 ng/ml of TGFB1 (). In regard to vascular remodeling, a dose of more than 250 ng/ml of sENG significantly inhibited the formation of tubes compared with that in the control under 8% O2 tension, but there were no significant differences in the total tube area formed by HchEpC1b-mStrawberry cells with HUVECs in the presence of 125 ng/ml sENG (; Fig. S3c). HTR8-mStrawberry cells (red) replaced HUVECs (green) in the absence of sENG at 12 h (Fig. 6De and Di, blue solid line). On the other hand, sENG (100 ng/ml) impaired the replacement of HUVECs (green) by HTR8-mStrawberry cells (red) at 12 h (Fig. 6Df and Di, blue dotted line); however, 100 ng/ml sENG did not affect the proportion of HTR8-ATG4BC74A cells (red) in the total tube area at 12 h (Fig. 6Dg, Dh and Dj, red solid and dotted lines), suggesting that replacement of endothelial cells by EVT cells (a model for vascular remodeling) was impaired by a low dose (100 ng/ml) of sENG in the HTR8-mStrawberry cells. These results suggested that sENG inhibited the EVT functions, such as invasion and tube formation, through inhibition of autophagy.
Figure 6. sENG inhibited EVT invasion and vascular remodeling. (A) Invasion assays were performed with HchEpC1b-ATG4BC74A, an autophagy-deficient EVT cell line, or HchEpC1b-mStrawberry cells in the presence or absence of 100 ng/ml sENG under 2% oxygen tension for 48 h. The Y-axis indicates the number of invading cells. Data were normalized to 1 for the control at 48 h. (B) HchEpC1b-mStrawberry cells were treated with 100 ng/ml sENG and several doses of TGFB1 under 2% oxygen tension for 48 h. Data were normalized to 1 for the control at 48 h. (C) Tube formation assays by HUVECs with HchEpC1b-mStrawberry cells were performed under 8% oxygen tension for 24 h in the presence of 0, 125, 250 or 500 ng/ml sENG. The quantification of total tube area formed with dual cell lines was evaluated at 24 h. (D) Representative figures of tube formation by HUVECs (green) with HTR8-ATG4BC74A (red) or HTR8-mStrawberry cells (red) in the presence (f and h) or absence (e and g) of 100 ng/ml sENG under 8% oxygen tension for 12 h. (i and j) The graphs show the HTR8 (red) area as a proportion of the total area in the HTR8-mStrawberry cells and HUVECs (i) or HTR8-ATG4BC74A cells and HUVECs (j). *p < 0.001: the proportion in HTR8-mStrawberry and HUVECs control (blue solid line) is significantly higher than that in HTR8-mStrawberry and HUVECs with 100 ng/ml sENG (blue dotted line). There was no significant difference between the HTR8-ATG4BC74A and HUVECs control (red solid line) and the HTR8-ATG4BC74A and HUVECs with 100 ng/ml sENG (red dotted line). Data are shown as the mean ± S.E. for three independent experiments. Scale bar: 300 μm. n.s., not significant.
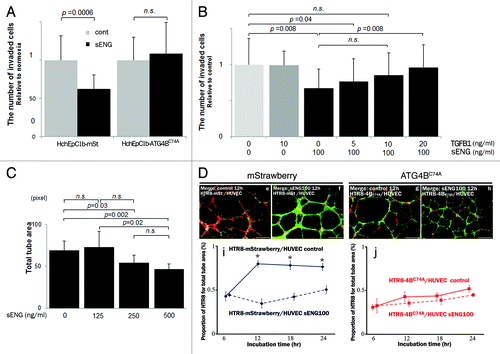
Accumulation of SQSTM1 in EVTs in preeclampsia
Finally, to evaluate the autophagy in EVTs of preeclamptic cases in vivo, the expression of SQSTM1 was assessed as a marker of autophagy inhibition in placental bed biopsy samples. It has been reported that hypoxic activation of autophagy induces clearance of the SQSTM1 protein in carcinoma cells.Citation31 Our results showed that SQSTM1 expression was significantly decreased under hypoxia in HTR8-mStrawberry or HchEpC1b-mStrawberry cells, control cell lines, although it was not significantly decreased under hypoxia in HTR8-ATG4BC74A or HchEpC1b-ATG4BC74A cells, autophagy-deficient EVT cell lines (; Fig. S2e). SQSTM1 expression in interstitial EVT cells was not found in normal pregnancy (), but was markedly found in preeclampsia (). Similar results were obtained in endovascular EVT cells (). Little expression of SQSTM1 was, however, seen in villous trophoblasts
Figure 7. The expression of SQSTM1 in EVTs in preeclamptic placentas. Representative panels show the expression of cytokeratin 7 (KRT7) and SQSTM1 in placental bed biopsies in normal placenta (NP) and preeclamptic placenta (PE) by immunohistochemistry. Four panels on the left show the interstitial EVTs (a–d), four panels in the center show the endovascular EVT (e–h) and two panels on the right show the villous region (I and j). (d and h) Enlarged images of the SQSTM1-positive cells. KRT7 staining, indicating EVTs, was performed in (a, c, e and g). SQSTM1 staining, a marker of autophagic inhibition, was performed in (b, d, f, and h–j). KRT7-positive cells in the myometrium around the vessels were counted as endovascular EVTs, and those in the myometrium without vessels, as interstitial EVTs. (K and L) The graphs show ratios of SQSTM1-positive cells to KRT7-positive cells in the interstitial EVTs (K), in the endovascular EVTs (L) and in the villous trophoblast (M) between preeclampsia (PE, n = 9) and normal pregnancy (NP, n = 17). Dots indicate the ratios of individuals, and bars indicate the median values. Square, PE; Circles, NP and triangles, placenta previa. Scale bar: 50 µm.
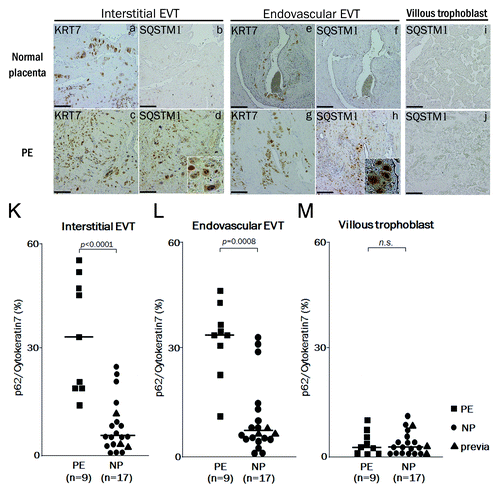
in preeclampsia (). Additionally, the distribution of SQSTM1 was similar to that of KRT7, which indicates EVTs, in the serial sections of preeclampsia placental bed biopsy samples. The ratio of SQSTM1 to KRT7 expression was significantly higher in preeclampsia than in normal pregnancy in both interstitial and endovascular EVTs (, p < 0.0001, p = 0.0008). Patients with placenta previa, who all delivered preterm, did not show significantly high expression of SQSTM1 in EVTs (, triangle marks). On the other hand, there were no significant differences in SQSTM1 expression in villous trophoblasts between the preeclampsia and normal pregnancy samples (). These results suggested that disruption of autophagy occurred in EVTs, but not villous trophoblasts, in preeclampsia.
Discussion
We first showed the enhancement of autophagy even under physiological hypoxia in EVTs, suggesting that autophagy has physiological functions during early placentation in vivo. As shown in Figure S1, the oxygen concentrations, at which EVT functions, were known to be different before and after 12 weeks of gestation. As for EVT functions, autophagy was an indispensable mechanism to promote EVT-invasion as well as crosstalk between EVTs and the endothelium at the feto-maternal interface under physiological hypoxia during early pregnancy. On the other hand, many reports have shown that a disruption of EVT functions contributes to the pathophysiology of preeclampsia during early pregnancy.Citation2,Citation6,Citation28,Citation29 Our results showed that the failure of EVT invasion and vascular remodeling by EVTs were induced by autophagic inhibition. Paradoxically, there might be a correlation between the inhibition of autophagy and onset of preeclampsia. Therefore, we hypothesized that impairment of autophagy may be involved in poor placentation. Maternal endothelial dysfunction induced by sENG in concert with sFLT1 is recognized as one of the major causes of preeclampsia.Citation16,Citation30 Thus, this study first showed that sENG inhibited the autophagy in EVTs under hypoxia, resulting in a failure of vascular remodeling by EVTs as well as failure of EVT invasion. Our data also showed the accumulation of SQSTM1 in EVTs in preeclampsia cases, suggesting that autophagy in EVTs is impaired in preeclampsia. Our results are the first to show the importance of autophagy to the pathophysiology of preeclampsia.
In regard to the pathophysiology of preeclampsia, a placental hypoxic model in rats clearly showed that placental hypoxia itself led to increased plasma and placental expression of sENG, resulting in decreased fetal weight and increased blood pressure in dams.Citation32 This animal model mimicked the two stages of preeclampsia, hypoplastic placentation and endothelial dysfunction. The release of sENG under 2% O2 tension was also increased in primary human trophoblasts obtained from placentas of preeclampsia patients, compared with those from normal pregnant women.Citation33 Thus, hypoxia initiates the induction of preeclampsia and then augments sENG production from syncytiotrophoblasts or endothelial cells in the placenta. Our results suggested that inhibition of autophagy in EVTs, which induces shallow trophoblast invasion, could cause preeclampsia, by emphasizing hypoxia at the feto-maternal interface.
Recent data show that mRNAs for sENG and TGFB1 were already elevated in chorionic villous samples at 11 weeks of gestation from women who later developed preeclampsia.Citation34 The sENG concentration in sera was already elevated at 17–20 weeks of gestation before the onset of early-onset preeclampsia.Citation30,Citation35 The sENG concentration in the intervillous space could be higher than that in maternal sera; therefore, vascular remodeling by EVTs might be disrupted by sENG in the first or second trimester of pregnancy, resulting in shallow trophoblast invasion and inadequate vascular remodeling in preeclampsia. Mano et al. report that membrane-bound ENG negatively regulates the invasion of normal HTR8/SVneo cells under normoxia, but sENG does not affect invasion in HTR8/SVneo cells under normoxia.Citation36 In our study, sENG diminished EVT functions by inhibiting autophagy in hypoxia, but not normoxia. Additionally, the suppressive effect by sENG on EVT invasion under hypoxia was removed by the addition of TGFB1 (). Hypoxia also enhances the release of sENG in syncytiotrophoblasts.Citation33 If autophagy, which is indispensable for EVT invasion under hypoxia in the first trimester, is impaired in EVTs, hypoxia in the placenta caused by shallow invasion might enhance sENG production in the second trimester, resulting in insufficient vascular remodeling.
Not only EVT invasion but also vascular formation between EVTs and HUVECs was impaired by a disruption of autophagy in this study. High concentrations of sENG (250 and 500 ng/ml) inhibited the tube formation by HUVECs and HchEpC1b-mStrawberry cells (; Fig. S3c); on the other hand, a low concentration of sENG, 100 ng/ml, which is close to the serum level in preeclampsia patients, suppressed the vascular remodeling by EVT cells like autophagy-deficient cells. Although this is indirect evidence, inadequate vascular remodeling of EVTs might be, in part, related to the disruption of autophagy in EVTs during the second stage of preeclampsia.
Impaired selective turnover of SQSTM1 by autophagy corresponds to the pathophysiological conditions seen in human hepatocellular carcinoma.Citation37 A recent study shows that a failure of autophagy enhances oxidative stress, accompanied by SQSTM1 accumulation, in a mouse kidney cell line under metabolic stress, low glucose and 1% O2 tension.Citation38 Mice lacking ATG16L1 in hematopoietic cells, which accumulate SQSTM1, are also highly susceptible to endotoxin-induced inflammatory immune responses, resulting in Crohn disease like colitis.Citation39 In our study, a decline of SQSTM1 expression under hypoxia was observed in autophagy-normal EVT cells but not in autophagy-deficient EVT cells (; Fig. S2e). Marked SQSTM1 expression in EVTs in placental bed biopsies in preeclampsia was found in vivo, suggesting the disruption of autophagy by sENG in EVT cells in preeclamptic cases. Preeclampsia is an inflammatory disease in pregnant women, and the level of TNF is increased in the plasma of preeclampsia women.Citation40-Citation42 EVT cells which express SQSTM1 at high levels in preeclampsia women might be susceptible to inflammatory cytokines.
In regard to the correlation between autophagy and placenta, a few papers reported the expression of autophagy-related proteins in placentas following vaginal delivery and caesarean section, or normal placenta and preeclampsia.Citation25,Citation26 Oh et. al. showed that the expression of MAP1LC3B mRNA was significantly increased in placentas from patients with preeclampsia, compared with normal pregnancies. Immunostaining for MAP1LC3B in placental villi was significantly increased in preeclamptic placentas, suggesting that autophagy is activated in syncytiotrophoblasts.Citation25 Additionally, Hung T et al. reported that autophagy is activated in the villous trophoblasts in preeclampsia with intrauterine growth restriction (PE+IUGR) or IUGR placentas, compared with that in normal human pregnancy.Citation43 In our study, we did not detect the accumulation of SQSTM1, a marker of autophagic inhibition, in placental villi in either preeclamptic placentas or normal placentas, suggesting that autophagy was not inhibited in syncytiotrophoblasts. Taken together, autophagy was activated in syncytiotrophoblasts, and inhibited in EVTs in preeclamptic placentas. Preeclamptic placentas are well known to suffer from severe hypoxia. The differences in SQSTM1 expression between EVTs and syncytiotrophoblasts in preeclamptic placentas may indicate a difference of susceptibility to hypoxia dependent on autophagic activation. In other words, these findings suggested that hypoxia induced-autophagy activation maintains cellular homeostasis in syncytiotrophoblasts in preeclamptic placentas, and the disruption of autophagy in EVTs might contribute to hypoplastic placentation.
As for trophoblast invasion, there are differences between mouse and human placentation. Human EVTs invade into the uterine myometrium up to one third of its depth. In mice, the invasion is shallower. Additionally, ATG5−/− mice, an autophagy-deficient model, are born with minimal abnormalities at birth and do not show IUGR.Citation24 Furthermore, Kumasawa et al. reported that the physiological excessive expression of human sFLT1 in placenta induced symptoms of preeclampsia in pregnant mice.Citation13 But mouse sFLT1 in placenta did not induce preeclampsia (personal communication). There might be quite a difference in function between humans and mice in regard to anti-angiogenic factors as well as EVT-invasion. As autophagy is well conserved from yeast to humans, it may be developmentally involved in the pathophysiology of preeclampsia, a feature of human pregnancy.
In conclusion, we showed for the first time that autophagy played an important role in trophoblast functions under physiologically hypoxic conditions, suggesting a contribution of autophagy to normal placentation. On the other hand, the activation of autophagy in EVTs under hypoxia was inhibited by sENG, and autophagic inhibition in EVTs was observed in preeclampsia cases. Therefore, identification of the disruption of EVT due to inhibition of autophagy by sENG will break new ground for studying the pathophysiology of preeclampsia.
Materials and Methods
Reagents
Recombinant human sENG (R&D, 1097-EN), human sFLT1 (R&D, 321-FL/CF), human TNF (R&D, 210-TA/CF) and human TGFB1 (R&D, 240-B) were purchased from R&D Systems. The following antibodies (Ab) were used: rabbit polyclonal Ab for MAP1LC3B (MBL, PM036), rabbit polyclonal Ab for MAP1LC3B (ABGENT, AP1802a), mouse monoclonal Ab for SQSTM1 (MBL, 5F2), mouse monoclonal Ab for HIF1A (BD PharMingen, H72320), mouse monoclonal Ab for TUBA1A (Sigma-Aldrich, T8203), mouse monoclonal Ab for KRT7 (NOVUS Biological, NB120-17069) and mouse monoclonal Ab for HLA-G (Abcam, Ab7759). Protease inhibitors, E64d (Peptide institute, 4321-v) and pepstatin A (Peptide institute, 4397), were purchased from Peptide Institute, Inc.
Subjects
All clinical studies were approved by the University of Toyama Ethics Committee, and informed consent was obtained from all patients. Clinical samples were obtained in early or late pregnancy. Normal early placental tissues in the early pregnant period were obtained from patients (four cases, median maternal age 27 y, range 23–32 y; median gestational age 8 weeks, range 7–10 weeks) who had undergone elective termination by vaginal curettage. The termination was done legally at the patient’s wish. The women had received no medication, or had infectious, autoimmune, or other systemic diseases. Karyotype was not analyzed in the abortive specimens. These specimens were treated as normal early pregnant specimens. All placental bed biopsy samples, late placental tissue samples, were obtained when caesarean section was performed. Preeclampsia was defined by the American College of Obstetricians and Gynecologists criteria. Clinical information is described in Table S1. The normal pregnancy group (NP) contained healthy pregnant women who received a caesarean section due to breech presentation or previous caesarean section. Additionally, three patients with placenta previa, which were all preterm deliveries, are shown in . They are clearly shown in the normal pregnancy groups in .
Construction of an autophagy-deficient cell line
To establish autophagy-deficient HTR8/SVneo cells or autophagy-deficient HchEpC1b cells, we used pMRX-IRES-puro-mStrawberry-ATG4BC74A, an ATG4BC74A mutant expression vector that inhibits MAP1LC3B-II formation, and pMRX-IRES-puro-mStrawberry, a control vector encoding monomeric red fluorescent protein. The procedures for constructing the vectors were reported previously.Citation44 After infection with these vectors, the cells were incubated in DMEM (Sigma, D5671) supplemented with 10% FBS (Cell culture technologies, CC3008-504) containing 0.3 µg/ml puromycin (Sigma, P8833). Growing cells were collected, and the expression of mStrawberry was confirmed by fluorescence microscopy. The constructed autophagy-deficient cell lines and the control vector-infected cell lines were named HTR-ATG4BC74A cells and HchEpC1b-ATG4BC74A cells and HTR8-mStrawberry cells and HchEpC1b-mStrawberry cells, respectively.
Cell culture
EVT cell lines, HTR8/SVneo (a gift from Dr. Charles H. Graham, Department of Anatomy and Cell Biology, Queen’s University) and HchEpC1b, were used in this study. HchEpC1b is an HPV E6 and hTERT-transfected immortalized EVT cell line.Citation45 HTR8/SVneo cells were cultured in DMEM supplemented with 10% FBS, 100 U/ml penicillin and 100 µg/ml streptomycin (GIBCO, 15140) at 37°C in a 5% CO2 atmosphere, and HchEpC1b cells with RPMI1640 medium (Sigma, R8758) supplemented with 10% FBS, 100 U/ml penicillin and 100 µg/ml streptomycin at 37°C in a 5% CO2 atmosphere. HUVECs (Lonza, CC-2517) were cultured in EGM-2 Bullet Kit (Lonza, CC-3162) according to the manufacturer’s instructions. For hypoxic cultures, the cells were plated on a 35-mm dish at 2 × 105 cells/dish. After 24 h, the cells were transferred to an incubator under a 2% O2 and 5% CO2 atmosphere at 37°C. For starved cultures, the culture medium was exchanged for Hanks’ solution (Nacalai Tesque Inc., 17460).
Small pieces of tissue (2 to 3 mm) from the placental villi, which were obtained from 1st trimester placental tissues, were dissected (usually 8 to 16 villus tips per sample). For each explant, 5 μg/cm2 of FibronectinTM (BD Biosciences, 356008) was placed in the center of an 8-well culture slide (Thermo Fisher Scientific, Inc., 177402). After the formation of gels (30 min at 37°C), the dissected tissue pieces were carefully placed at the center of each chamber, covered with 200 μl of medium, and incubated for 24 h at 37°C with 5% CO2 and 20% O2. After an initial period of 24 h, villi with good attachment and outgrowth on the gel were selected for the hypoxic culture. Before the experiment, the outgrown cells were characterized by immunocytochemical analysis for KRT7 and HLA-G to confirm that they were EVTs.
Quantitative analysis of GFP-LC3 puncta
For the quantitative analysis of MAP1LC3B, the cells were stained with MAP1LC3B or transfected with a green fluorescent protein-microtubule-associated protein 1 light chain 3 protein (GFP-MAP1LC3B) adenovirus vector (5 × 109 pfu/ml) and incubated for 24 h. The cells were pretreated with lysosomal protease inhibitors, E64d (10 ng/ml) and pepstatin A (10 ng/ml), for 2 h to distinguish cytoplasmic MAP1LC3B puncta, and then fixed with 4% paraformaldehyde-PBS.Citation46 The incidence of autophagy was estimated by quantifying the number of MAP1LC3B puncta within MAP1LC3B-stained cells by manual counting in five independent visual fields using a fluorescence microscope (KEYENCE, BZ-8000) or a confocal microscope (Carl Zeiss, LSM700). Cells containing more than 5 GFP-MAP1LC3B punta in cytoplasm were counted as autophagic. These experiments were independently performed at least three times.
Invasion assays and three-dimensional invasion assay
A conventional invasion assay was performed using a BD BioCoat Growth Factor Reduced Matrigel Invasion Chamber (BD Biosciences, 354483) according to the manufacturer’s instructions. Cells were plated in the upper insert at 5 × 104 /well and incubated under normoxia (20% O2) or hypoxia (2% O2). These cells were incubated for 48 h, and then the upper surface of the membrane in each insert was gently scrubbed with a cotton swab to remove all of the noninvading cells, and the cells on the under surface of the membranes were fixed in 100% methanol (Wako Pure Chemical Industries, Ltd., 131-01826), stained with 0.05% Toluidine Blue Solution (Wako, 206-14555), and then the number of invading cells was counted by microscopy.
The three-dimensional invasion assay was performed as described previously.Citation47 Collagen matrix, Cellmatrix® (Kurabo Industries, Ltd., KP-2020), was prepared according to the manufacturer’s instructions. Cells at 2 × 105 /ml were then placed on the top of the matrix and incubated under normoxia (20% O2) or hypoxia (2% O2) for 48 h. The collagen matrix was fixed with 4% paraformaldehyde (Wako, 163-20145) and cut for observation. The invasion distance was measured by fluorescence microscopy.
Tube formation assay using an EVT cell and endothelial cell coculture system
This assay was from a previous report.Citation48 This three-dimensional dual cell culture model is suitable for evaluating vascular remodeling by trophoblasts. Briefly, EVT cells (1 × 105 /ml) or HUVECs (1 × 105 /ml), labeled with cell tracker green CMFDA (5-chloromethylfluorescein diacetate, Molecular Probes, C-2925) or cell tracker red CMTMR [5-(and-6)-(((4-chloromethyl)benzoyl)amino)tetramethylrhodamine, Molecular Probes, C-2927], respectively, were cocultured on thick Matrigel (BD, 356234)-coated plates in the presence or absence of sENG. The cells were then incubated in an 8% O2 and 5% CO2 atmosphere at 37°C for 24 h. The images were imported as TIFF files into the National Institutes of Health Image J software (http://rsbweb.nih.gov/ij/). The total area of the tube-like structures per image was quantified using Image J. The proportion, HTR8/HUVECs, was calculated by dividing the area of HTR8 by the area of HUVECs. The averages of these factors were calculated from the data for five independent visual fields. These experiments were independently performed at least three times. HUVECs, for which passages were performed 3 times, were used in this assay.
Immunohistochemistry
Immunohistochemistry was performed as described previously.Citation49 The sections were incubated overnight at 4°C, with the primary antibodies, anti-KRT7 (1:250) and anti-MAP1LC3B (1:100; ABGENT), diluted with 5% BSA/DW. After extensive washing with TBS-Tween solution, the sections were stained with the secondary antibodies, Alexa Fluor 594 donkey anti-mouse IgG (1:2000; Molecular Probes, A-21203) and Alexa Fluor 488 donkey anti-rabbit IgG (1:2000; Molecular Probes, A-21206), and with Hoechst33342 (1:500; Molecular Probes, H-3570) for nuclear staining overnight at 4°C and then observed by confocal microscopy. Confocal images of fluorescent materials were collected using a confocal microscope (Carl Zeiss).
Immunocytochemistry
Primary EVT cells, HTR8/SVneo or HchEpC1b cells, were fixed in 4% paraformaldehyde/PBS for 15 min and labeled with the primary antibody, anti-MAP1LC3B (MBL), at a dilution of 1:500. Negative controls were produced by replacing the primary antibody with normal rabbit serum and then were secondarily stained with Alexa Fluor 488 donkey anti-rabbit IgG. They were finally stained with Hoechst33342 for 10 min, washed with PBS and observed under a fluorescence microscope or a confocal microscope.
Electron microscopy
Electron microscopy was performed as described previously.Citation50 Cells were fixed with 0.1 M phosphate buffer containing 2.5% glutaraldehyde and 2% paraformaldehyde at 4°C for 1 h. The cells were washed with the same buffer three times and post-fixed with 1% osmium tetroxide (OsO4) in 0.1 M phosphate buffer for 1 h. After washing, cells were incubated with a 3% uranyl solution, further dehydrated with a graded series of ethanol and propylene oxide solutions, and embedded. Areas containing cells were block-mounted and cut into 50-nm sections. The sections were stained with uranyl acetate and lead citrate and observed under an electron microscope.
Flow cytometry
Cells were harvested, washed once and then fixed in 4% paraformaldehyde-PBS for 15 min at room temperature. After being permeabilized with 100 μg/ml digitonin (Sigma-Aldrich, D141) for 15 min at room temperature, the cells were resuspended in PBS solution containing anti-MAP1LC3B antibody (1:200, MBL) for 30 min at room temperature. Subsequently, the cells were stained with FITC goat anti-rabbit IgG (BD PharMingen, 554020) and incubated for 30 min at room temperature. Samples were then analyzed with a FACS Calibur flow cytometer (BD Biosciences) using the Cell Quest software as described previously (BD Biosciences).Citation51 Negative controls were performed by replacing the primary antibody with normal rabbit serum. To estimate the proportion of dead cells, the harvested cells were suspended in PBS solution containing 5 µg/ml propidium iodide (Molecular Probes, P3566), and incubated for 30 min at room temperature. Samples were then analyzed with a FACS Calibur flow cytometer using the Cell Quest software (BD Bioscience).
Cell proliferation assay
To estimate cell proliferation, we used two methods: the first was manual counting and the second involved use of the cell proliferation reagent WST-1 (Roche, 5015944) according to the manufacturer’s instructions.
Enzyme-linked immunosorbent assay (ELISA)
SQSTM1 expression was measured with a p62 ELISA kit (Enzo Life Sciences, ADI-900-212-0001) according to the manufacturer's instructions. The cells were cultured under 2% or 20% oxygen tension for 24 h. The cells were lysed with Radio-Immunoprecipitation Assay (RIPA) buffer containing protease inhibitors. The range of the standard curve was 625 to 40,000 pg/ml. For each assay, all samples were assayed in duplicate in the same plate. Results were normalized per milligram of protein from cell lysate.
Statistical analysis
Results are presented as the mean ± S. E. and comparisons between multiple groups were made using ANOVA. Values of p < 0.05 were considered statistically significant.
Abbreviations: | ||
AGTR1-AA | = | angiotensin II receptor, type 1 receptor agonistic autoantibody |
ATG4B | = | autophagy-related 4B |
COMT | = | catechol-O-methyltransferase |
EVT | = | extravillous trophoblast |
HIF1A | = | hypoxia inducible factor 1, alpha subunit |
HLA-G | = | major histocompatibility complex, class I, G |
HUVECs | = | human umbilical vein endothelial cells |
KRT7 | = | cytokeratin 7 |
MAP1LC3B | = | microtubule-associated protein 1 light chain 3 beta |
NP | = | normal pregnancy |
PE | = | preeclampsia |
sENG | = | soluble endoglin |
sFLT1 | = | soluble fms-related tyrosine kinase 1 |
TGFB1 | = | transforming growth factor, beta 1 |
TNF | = | tumor necrosis factor-alpha |
Additional material
Download Zip (4 MB)Acknowledgments
We thank all the staff at the Department of Obstetrics, University of Toyama, for help with patient identification and recruitment. We thank Dr. E. Morita for providing advice and M. Suzuki, Y. Shimizu and A. Ushijima for technical assistance with some assays. This research was supported by grants from the Ministry of Education, Culture, Sports, Science and Technology, Japan [Grant-in-Aid for Scientific Research (B)-23390386 and Grant-in-Aid for Young Scientists (B)-23791817].
Disclosure of Potential Conflicts of Interest
No potential conflicts of interest were disclosed.
Supplemental Materials
Supplemental materials may be found here: www.landesbioscience.com/journals/autophagy/article/XXXX
References
- Duley L. The global impact of pre-eclampsia and eclampsia. Semin Perinatol 2009; 33:130 - 7; http://dx.doi.org/10.1053/j.semperi.2009.02.010; PMID: 19464502
- Steegers EA, von Dadelszen P, Duvekot JJ, Pijnenborg R. Pre-eclampsia. Lancet 2010; 376:631 - 44; http://dx.doi.org/10.1016/S0140-6736(10)60279-6; PMID: 20598363
- World Health Organization. Policy and Coordination Comittee 16th meeting. Department of Reproductive Health and Research. Summary Medium-term Programme of Work 2004-2009 HRP/PCC(16)/2003/8.1.
- Smith GC, Pell JP, Walsh D. Pregnancy complications and maternal risk of ischaemic heart disease: a retrospective cohort study of 129,290 births. Lancet 2001; 357:2002 - 6; http://dx.doi.org/10.1016/S0140-6736(00)05112-6; PMID: 11438131
- Zhou Y, Fisher SJ, Janatpour M, Genbacev O, Dejana E, Wheelock M, et al. Human cytotrophoblasts adopt a vascular phenotype as they differentiate. A strategy for successful endovascular invasion?. J Clin Invest 1997; 99:2139 - 51; http://dx.doi.org/10.1172/JCI119387; PMID: 9151786
- Huppertz B. The feto-maternal interface: setting the stage for potential immune interactions. Semin Immunopathol 2007; 29:83 - 94; http://dx.doi.org/10.1007/s00281-007-0070-7; PMID: 17621696
- Red-Horse K, Zhou Y, Genbacev O, Prakobphol A, Foulk R, McMaster M, et al. Trophoblast differentiation during embryo implantation and formation of the maternal-fetal interface. J Clin Invest 2004; 114:744 - 54; PMID: 15372095
- Genbacev O, Zhou Y, Ludlow JW, Fisher SJ. Regulation of human placental development by oxygen tension. Science 1997; 277:1669 - 72; http://dx.doi.org/10.1126/science.277.5332.1669; PMID: 9287221
- Jauniaux E, Hempstock J, Teng C, Battaglia FC, Burton GJ. Polyol concentrations in the fluid compartments of the human conceptus during the first trimester of pregnancy: maintenance of redox potential in a low oxygen environment. J Clin Endocrinol Metab 2005; 90:1171 - 5; http://dx.doi.org/10.1210/jc.2004-1513; PMID: 15562012
- Jauniaux E, Watson A, Burton G. Evaluation of respiratory gases and acid-base gradients in human fetal fluids and uteroplacental tissue between 7 and 16 weeks’ gestation. Am J Obstet Gynecol 2001; 184:998 - 1003; http://dx.doi.org/10.1067/mob.2001.111935; PMID: 11303211
- Tuuli MG, Longtine MS, Nelson DM. Review: Oxygen and trophoblast biology--a source of controversy. Placenta 2011; 32:Suppl 2 S109 - 18; http://dx.doi.org/10.1016/j.placenta.2010.12.013; PMID: 21216006
- Kanasaki K, Palmsten K, Sugimoto H, Ahmad S, Hamano Y, Xie L, et al. Deficiency in catechol-O-methyltransferase and 2-methoxyoestradiol is associated with pre-eclampsia. Nature 2008; 453:1117 - 21; http://dx.doi.org/10.1038/nature06951; PMID: 18469803
- Kumasawa K, Ikawa M, Kidoya H, Hasuwa H, Saito-Fujita T, Morioka Y, et al. Pravastatin induces placental growth factor (PGF) and ameliorates preeclampsia in a mouse model. Proc Natl Acad Sci U S A 2011; 108:1451 - 5; http://dx.doi.org/10.1073/pnas.1011293108; PMID: 21187414
- Levine RJ, Maynard SE, Qian C, Lim KH, England LJ, Yu KF, et al. Circulating angiogenic factors and the risk of preeclampsia. N Engl J Med 2004; 350:672 - 83; http://dx.doi.org/10.1056/NEJMoa031884; PMID: 14764923
- Maynard SE, Min JY, Merchan J, Lim KH, Li J, Mondal S, et al. Excess placental soluble fms-like tyrosine kinase 1 (sFlt1) may contribute to endothelial dysfunction, hypertension, and proteinuria in preeclampsia. J Clin Invest 2003; 111:649 - 58; PMID: 12618519
- Venkatesha S, Toporsian M, Lam C, Hanai J, Mammoto T, Kim YM, et al. Soluble endoglin contributes to the pathogenesis of preeclampsia. Nat Med 2006; 12:642 - 9; http://dx.doi.org/10.1038/nm1429; PMID: 16751767
- Zhou CC, Zhang Y, Irani RA, Zhang H, Mi T, Popek EJ, et al. Angiotensin receptor agonistic autoantibodies induce pre-eclampsia in pregnant mice. Nat Med 2008; 14:855 - 62; http://dx.doi.org/10.1038/nm.1856; PMID: 18660815
- Cuervo AM. Autophagy: in sickness and in health. Trends Cell Biol 2004; 14:70 - 7; http://dx.doi.org/10.1016/j.tcb.2003.12.002; PMID: 15102438
- Levine B, Klionsky DJ. Development by self-digestion: molecular mechanisms and biological functions of autophagy. Dev Cell 2004; 6:463 - 77; http://dx.doi.org/10.1016/S1534-5807(04)00099-1; PMID: 15068787
- Mizushima N. Autophagy: process and function. Genes Dev 2007; 21:2861 - 73; http://dx.doi.org/10.1101/gad.1599207; PMID: 18006683
- Yoshimori T. Autophagy: a regulated bulk degradation process inside cells. Biochem Biophys Res Commun 2004; 313:453 - 8; http://dx.doi.org/10.1016/j.bbrc.2003.07.023; PMID: 14684184
- Tsukamoto S, Kuma A, Murakami M, Kishi C, Yamamoto A, Mizushima N. Autophagy is essential for preimplantation development of mouse embryos. Science 2008; 321:117 - 20; http://dx.doi.org/10.1126/science.1154822; PMID: 18599786
- Lee JE, Oh HA, Song H, Jun JH, Roh CR, Xie H, et al. Autophagy regulates embryonic survival during delayed implantation. Endocrinology 2011; 152:2067 - 75; http://dx.doi.org/10.1210/en.2010-1456; PMID: 21363932
- Kuma A, Hatano M, Matsui M, Yamamoto A, Nakaya H, Yoshimori T, et al. The role of autophagy during the early neonatal starvation period. Nature 2004; 432:1032 - 6; http://dx.doi.org/10.1038/nature03029; PMID: 15525940
- Oh SY, Choi SJ, Kim KH, Cho EY, Kim JH, Roh CR. Autophagy-related proteins, LC3 and Beclin-1, in placentas from pregnancies complicated by preeclampsia. Reprod Sci 2008; 15:912 - 20; http://dx.doi.org/10.1177/1933719108319159; PMID: 19050324
- Signorelli P, Avagliano L, Virgili E, Gagliostro V, Doi P, Braidotti P, et al. Autophagy in term normal human placentas. Placenta 2011; 32:482 - 5; http://dx.doi.org/10.1016/j.placenta.2011.03.005; PMID: 21459442
- Fujita N, Noda T, Yoshimori T. Atg4B(C74A) hampers autophagosome closure: a useful protein for inhibiting autophagy. Autophagy 2009; 5:88 - 9; http://dx.doi.org/10.4161/auto.5.1.7183; PMID: 19104152
- Jauniaux E, Watson AL, Hempstock J, Bao YP, Skepper JN, Burton GJ. Onset of maternal arterial blood flow and placental oxidative stress. A possible factor in human early pregnancy failure. Am J Pathol 2000; 157:2111 - 22; http://dx.doi.org/10.1016/S0002-9440(10)64849-3; PMID: 11106583
- Kalkunte S, Boij R, Norris W, Friedman J, Lai Z, Kurtis J, et al. Sera from preeclampsia patients elicit symptoms of human disease in mice and provide a basis for an in vitro predictive assay. Am J Pathol 2010; 177:2387 - 98; http://dx.doi.org/10.2353/ajpath.2010.100475; PMID: 20889559
- Levine RJ, Lam C, Qian C, Yu KF, Maynard SE, Sachs BP, et al, CPEP Study Group. Soluble endoglin and other circulating antiangiogenic factors in preeclampsia. N Engl J Med 2006; 355:992 - 1005; http://dx.doi.org/10.1056/NEJMoa055352; PMID: 16957146
- Pursiheimo JP, Rantanen K, Heikkinen PT, Johansen T, Jaakkola PM. Hypoxia-activated autophagy accelerates degradation of SQSTM1/p62. Oncogene 2009; 28:334 - 44; http://dx.doi.org/10.1038/onc.2008.392; PMID: 18931699
- Gilbert JS, Gilbert SA, Arany M, Granger JP. Hypertension produced by placental ischemia in pregnant rats is associated with increased soluble endoglin expression. Hypertension 2009; 53:399 - 403; http://dx.doi.org/10.1161/HYPERTENSIONAHA.108.123513; PMID: 19075097
- Gu Y, Lewis DF, Wang Y. Placental productions and expressions of soluble endoglin, soluble fms-like tyrosine kinase receptor-1, and placental growth factor in normal and preeclamptic pregnancies. J Clin Endocrinol Metab 2008; 93:260 - 6; http://dx.doi.org/10.1210/jc.2007-1550; PMID: 17956952
- Farina A, Sekizawa A, De Sanctis P, Purwosunu Y, Okai T, Cha DH, et al. Gene expression in chorionic villous samples at 11 weeks’ gestation from women destined to develop preeclampsia. Prenat Diagn 2008; 28:956 - 61; http://dx.doi.org/10.1002/pd.2109; PMID: 18792924
- Noori M, Donald AE, Angelakopoulou A, Hingorani AD, Williams DJ. Prospective study of placental angiogenic factors and maternal vascular function before and after preeclampsia and gestational hypertension. Circulation 2010; 122:478 - 87; http://dx.doi.org/10.1161/CIRCULATIONAHA.109.895458; PMID: 20644016
- Mano Y, Kotani T, Shibata K, Matsumura H, Tsuda H, Sumigama S, et al. The loss of endoglin promotes the invasion of extravillous trophoblasts. Endocrinology 2011; 152:4386 - 94; http://dx.doi.org/10.1210/en.2011-1088; PMID: 21914777
- Inami Y, Waguri S, Sakamoto A, Kouno T, Nakada K, Hino O, et al. Persistent activation of Nrf2 through p62 in hepatocellular carcinoma cells. J Cell Biol 2011; 193:275 - 84; http://dx.doi.org/10.1083/jcb.201102031; PMID: 21482715
- Mathew R, Karp CM, Beaudoin B, Vuong N, Chen G, Chen HY, et al. Autophagy suppresses tumorigenesis through elimination of p62. Cell 2009; 137:1062 - 75; http://dx.doi.org/10.1016/j.cell.2009.03.048; PMID: 19524509
- Saitoh T, Fujita N, Jang MH, Uematsu S, Yang BG, Satoh T, et al. Loss of the autophagy protein Atg16L1 enhances endotoxin-induced IL-1beta production. Nature 2008; 456:264 - 8; http://dx.doi.org/10.1038/nature07383; PMID: 18849965
- Conrad KP, Miles TM, Benyo DF. Circulating levels of immunoreactive cytokines in women with preeclampsia. Am J Reprod Immunol 1998; 40:102 - 11; http://dx.doi.org/10.1111/j.1600-0897.1998.tb00398.x; PMID: 9764352
- Vince GS, Starkey PM, Austgulen R, Kwiatkowski D, Redman CW. Interleukin-6, tumour necrosis factor and soluble tumour necrosis factor receptors in women with pre-eclampsia. Br J Obstet Gynaecol 1995; 102:20 - 5; http://dx.doi.org/10.1111/j.1471-0528.1995.tb09020.x; PMID: 7833306
- Visser W, Beckmann I, Bremer HA, Lim HL, Wallenburg HC. Bioactive tumour necrosis factor alpha in pre-eclamptic patients with and without the HELLP syndrome. Br J Obstet Gynaecol 1994; 101:1081 - 2; http://dx.doi.org/10.1111/j.1471-0528.1994.tb13587.x; PMID: 7826964
- Hung TH, Chen SF, Lo LM, Li MJ, Yeh YL, Hsieh TT. Increased autophagy in placentas of intrauterine growth-restricted pregnancies. PLoS One 2012; 7:e40957; http://dx.doi.org/10.1371/journal.pone.0040957; PMID: 22815878
- Fujita N, Hayashi-Nishino M, Fukumoto H, Omori H, Yamamoto A, Noda T, et al. An Atg4B mutant hampers the lipidation of LC3 paralogues and causes defects in autophagosome closure. Mol Biol Cell 2008; 19:4651 - 9; http://dx.doi.org/10.1091/mbc.E08-03-0312; PMID: 18768752
- Omi H, Okamoto A, Nikaido T, Urashima M, Kawaguchi R, Umehara N, et al. Establishment of an immortalized human extravillous trophoblast cell line by retroviral infection of E6/E7/hTERT and its transcriptional profile during hypoxia and reoxygenation. Int J Mol Med 2009; 23:229 - 36; PMID: 19148547
- Tanida I, Minematsu-Ikeguchi N, Ueno T, Kominami E. Lysosomal turnover, but not a cellular level, of endogenous LC3 is a marker for autophagy. Autophagy 2005; 1:84 - 91; http://dx.doi.org/10.4161/auto.1.2.1697; PMID: 16874052
- Bayless KJ, Kwak HI, Su SC. Investigating endothelial invasion and sprouting behavior in three-dimensional collagen matrices. Nat Protoc 2009; 4:1888 - 98; http://dx.doi.org/10.1038/nprot.2009.221; PMID: 20010936
- Kalkunte S, Lai Z, Tewari N, Chichester C, Romero R, Padbury J, et al. In vitro and in vivo evidence for lack of endovascular remodeling by third trimester trophoblasts. Placenta 2008; 29:871 - 8; http://dx.doi.org/10.1016/j.placenta.2008.07.009; PMID: 18775564
- Nakashima A, Shiozaki A, Myojo S, Ito M, Tatematsu M, Sakai M, et al. Granulysin produced by uterine natural killer cells induces apoptosis of extravillous trophoblasts in spontaneous abortion. Am J Pathol 2008; 173:653 - 64; http://dx.doi.org/10.2353/ajpath.2008.071169; PMID: 18688023
- Yoshimori T, Yamagata F, Yamamoto A, Mizushima N, Kabeya Y, Nara A, et al. The mouse SKD1, a homologue of yeast Vps4p, is required for normal endosomal trafficking and morphology in mammalian cells. Mol Biol Cell 2000; 11:747 - 63; PMID: 10679028
- Nakashima A, Ito M, Yoneda S, Shiozaki A, Hidaka T, Saito S. Circulating and decidual Th17 cell levels in healthy pregnancy. Am J Reprod Immunol 2010; 63:104 - 9; http://dx.doi.org/10.1111/j.1600-0897.2009.00771.x; PMID: 20015328