Abstract
During the immune response, the cytokine interleukin 8 (IL-8, CXCL8) functions as a strong chemoattractant for polymorphonuclear leukocytes helping to direct these cells to infected/injured sites. This review focuses on the interaction of IL-8 with sulfated glycosaminoglycans expressed on cell surfaces and the extracellular matrix. This interaction contributes to the recruitment of polymorphonuclear cells from blood, penetration of these cells through the vessel wall, and their directed migration to inflammatory sites. Regulatory aspects of the interplay between IL-8 and heparan sulfate, the most abundant glycosaminoglycan, are highlighted. In this field, the large natural heterogeneity of glycosaminoglycans represents a great challenge that impedes the modeling of IL-8 functions. The interaction of IL-8 with newly developed artificial sulfated hyaluronan derivatives is also considered as these artificial substrates are an important tool for development of new materials in regenerative medicine.
Introduction
Chemokines are small proteins participating as important players of the immune system in recruitment of leukocytes, cell communication and activation. In humans, more than 40 different chemokines are known for their specific functions in innate and acquired immunity. They are divided into four main classes (C, CC, CXC and CX3C chemokines) according to position and spacing of cysteine(s) near the N-terminus. The cytokine interleukin-8 (IL-8, also called CXCL8 according to the chemokine nomenclature) released from fibroblasts, monocytes/macrophages, endothelial and epithelial cells at inflammatory sites is a strong chemoattractant agent mainly toward polymorphonuclear leukocytes (PMNs).Citation1 These cells are rapidly recruited to infected and/or injured tissue and represent the first line of immune response in our body. In addition to IL-8, the infiltration of PMNs to inflammatory sites is also highly regulated and supported by adhesion molecules, other cytokines, and extracellular matrix components in both blood vessel wall and adjacent tissue.Citation2,Citation3 Polymorphonuclear leukocytes contribute to pathogen defense, regulation of the inflammatory process and to tissue injury by releasing special proteins and generating reactive metabolites. The interplay between apoptotic and necrotic PMNs as well as macrophages at infected/injured sites mainly determines the outcome of inflammatory response.Citation4-Citation9 However, fine mechanisms important to switch from a pro- to an anti-inflammatory state including the termination of IL-8 responses are only scarcely known and remain to be further evaluated.
Here we briefly review the physiological functions of IL-8 in the stepwise recruitment of PMNs with special consideration of the interaction of IL-8 with polysaccharides of the extracellular matrix. The binding of IL-8 to sulfated glycosaminoglycans (GAGs) is a crucial step for the regulation of cell adhesion, migration and inflammation. Due to the great structural diversity of GAGs, a number of important questions concerning the matrix interaction of IL-8 remain unsolved. Answers to these questions will help to better understand regulation of inflammation, but also to develop new therapeutic strategies to treat conditions of dysregulated innate immune functions.
Structure of IL-8 and Isoforms
The three-dimensional structure of IL-8, composed of 72 amino acids, is well described.Citation10 The monomer consists of a conformational flexible N-terminus, which passes through a loop region and a three stranded antiparallel β-sheet, arranged in Greek key manner, into a C-terminal α-helix (residues 57−72).Citation11-Citation14 Two disulfide bridges are necessary for the anchorage of the N-terminus to the protein core and for the maintenance of the tertiary structure.Citation12,Citation15 Important structural details of human IL-8 are given in .
Figure 1. Three-dimensional structure of human IL-8 in solution as determined by NMR spectroscopy. The figure was constructed using the coordinates deposited in the Protein Data Bank (accession code pdb1IL8) by the pymol software (version 0.99). Due to the high concentrations used in structural studies IL-8 is present as a dimer, where the two α-helices are arranged in antiparallel fashion on top of a six stranded β-sheet. The receptor binding site, including the N-terminal receptor binding motif ELR (Glu-Leu-Arg) and the loop region from residue 30–36, is shown in yellow. GAG-binding regions, shown in red, comprise the residues 18–23 in the proximal loop and the α-helical domain. Of note, receptor- and GAG-binding sites are spatially separated. Further details are given in the text.
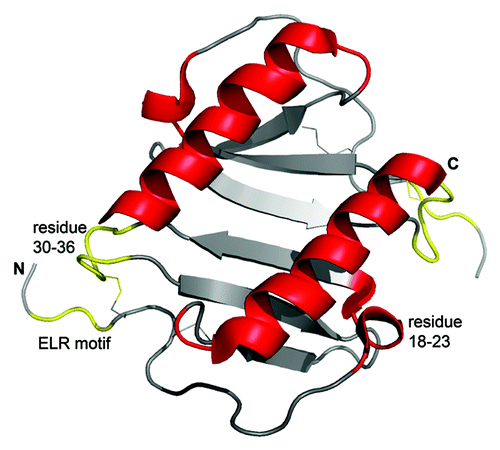
The short, more disordered N-terminus contains cleavage sites for proteolytic enzymes. After secretion as a monomeric protein, IL-8 is processed at the N-terminal end by extracellular proteinases to yield several active forms. The most prominent forms consist of 77 or 72 amino acid residues.Citation16 Proteinase 3, thrombin, plasmin, CD13, cathepsin L and several matrix metalloproteinases are known to be involved in the processing of IL-8. Elongation and deimination are further modification procedures of natural occurring IL-8.Citation17,Citation18
As many other cytokines, IL-8 monomers tend to form dimers. Apparently, the monomeric form dominates at nano- and low micromolar concentrations, i.e., the concentration range commonly used in receptor binding studies. At higher concentrations, important for structural studies, the dimer is preferred.Citation19 However, little is known about the existence of these forms, their functions and the equilibrium between them in vivo. Genetic engineering allows the production of stable IL-8 monomers and dimers that can be used to evaluate the role of this cytokine in inflammatory models.Citation20
The receptor binding motif ELR (GluCitation4-LeuCitation5-ArgCitation6 of the 72 amino acid form of IL-8) is localized near the N-terminus and arranged in a conformation favorable for receptor binding by interaction with the residues 30–36 of the neighboring loop region.Citation21-Citation24 Apparently, this ELR motif is also sterically protected from proteolytic attack. Most, but not all, posttranslational modifications of IL-8 are accompanied by a higher activity of this cytokine in receptor-mediated responses in PMNs or receptor-transfected cells.Citation18,Citation25 In IL-8, the receptor binding ELR motif is immediately adjacent to the first cysteine of the CXC motif that is an important structure characteristic of CXC chemokines.
On the surface of IL-8, there are several positively charged epitopes involved in binding of sulfated glycosaminoglycans.Citation26-Citation28 The two main binding regions for heparin and heparan sulfate are localized on the C-terminal α-helix and the proximal loop around residues 18–23.
Thus, binding sites for receptors and GAGs are well separated allowing IL-8 to interact with both components at the same time. While the removal of the entire C-terminus reduces partially the biological activity of IL-8, the elimination of the N-terminus with the receptor-binding motif completely prevents cytokine binding to the receptor.Citation12,Citation21,Citation29,Citation30 Citrullination of the N-terminal arginine in IL-8 has only small effects on the receptor-binding capacity, but weakens considerably binding to heparan sulfate.Citation17
Receptors for IL-8
Neutrophils as well as other leukocytes are equipped with two kinds of G protein-coupled receptors for IL-8 called CXCR1 and CXCR2.Citation31,Citation32 Both integral proteins have seven transmembrane domains. They bind IL-8 with high affinity as indicated by low interaction constants.Citation1 While CXCR1 is highly specific to IL-8, CXCR2 is known to interact with a number of other cytokines bearing an ELR group.Citation33
Receptor activation by IL-8 causes phosphorylation of protein kinase B, calcium influx, formation of F-actin, and cytoskeletal rearrangement. These events are very important for directed chemotactic movement of PMNs.
The duffy antigen receptor for chemokines (DARC) is also able to bind IL-8 and other chemokines with high affinity. This receptor is involved in transepithelial transport of IL-8.Citation34
In addition to proteolytic processing, several other factors are known to modulate the binding of IL-8 to its receptors. Alpha-1 antitrypsin and IL-8 form a complex that is unable to interact with CXCR1.Citation35 Oligomerization of chemokines affects also their interaction with receptors. While both monomeric and dimeric IL-8 forms are capable of inducing cell recruitment, the dimeric form seems to induce a stronger initial migration of neutrophils.Citation20 Binding to sulfated glycosaminoglycans promotes oligomerization of IL-8.Citation36
Biochemistry of the IL-8/Glycosaminoglycan Interaction
Structural diversity of glycosaminoglycans
Glycosaminoglycans are complex, linear polysaccharides of the extracellular matrix.Citation37,Citation38 They are composed of repeating disaccharide units that can be additionally modified by epimerization and/or sulfation. Most important GAGs in the extracellular matrix are the sulfated polysaccharides heparan sulfate, chondroitin sulfate, dermatan sulfate and keratan sulfate as well as the non-sulfated hyaluronan.Citation3 Mast cells contain and release heparin, a polysaccharide closely related to heparan sulfate. Basic structural properties of these GAGs and potential sites for postsynthetic modifications are listed in .
Table 1. Glycosaminoglycans of the extracellular matrix
Different kinds of postsynthetic modifications result in a great structural diversity of polymers especially in the sulfated forms. For example, 48 different disaccharide units can principally be formed in heparin/heparan sulfate. Even though only 23 of these variants really exist due to steric restrictions,Citation39 the resulting large variety of these polysaccharides is enormous. Moreover, there are also large differences in the degree of modifications in repeating units along the polysaccharide chain. In heparan sulfate, highly N-sulfated regions are alternating with unmodified N-acetyl-rich domains.Citation40 The latter domains contain a considerable fraction of 6-O-sulfate residues.Citation41 This large structural heterogeneity limits considerably our understanding of interacting mechanisms between cytokines and glycosaminoglycans.
Sulfated GAGs (most of all heparan sulfate) are often attached to proteins forming proteoglycans. contains important proteoglycans of the cell surface and the extracellular matrix. Syndecans and glypicans are expressed on the surface of endothelial cell and leukocytes. Agrin, perlecan and type XVIII collagen are components of the subendothelial basement membrane.Citation42 Several other proteoglycans on the cell surface acquire heparan sulfate chains only upon activation of the cell. Important members of this group are the hyaluronan receptor CD44, the type III transforming growth factor-β receptor betaglycan, and testican.Citation42-Citation44
Table 2. Important proteoglycans of cell surfaces and extracellular matrices
Together with different collagens, proteoglycans form a tight network stabilizing tissues and contributing to various tissue-specific functions. In case of infection or injury, immune cells have to penetrate from surrounding blood vessels to inflammatory sites through this network to fulfill their specific immune functions. Interleukin-8, like other cytokines and a number of small proteins such as growth factors, lipases and proteases, is known to interact closely with sulfated proteoglycans.Citation45-Citation49
Structural aspects of the interaction of IL-8 with sulfated glycosaminoglycans
An obvious important question is the significance of particular postsynthetic modifications of sulfated GAGs in interaction with IL-8. Measurement of the tryptophan fluorescence of recombinant human IL-8 (human IL-8 contains one tryptophan residue at position 57)Citation11 in interaction with selected glycosaminoglycan hexasaccharides revealed high affinity binding for both chondroitin-6-sulfate (KD = 1.4 ± 0.4 µM) and heparin (KD = 2.0 ± 0.4 µM).Citation28,Citation50 Binding constants for chondroitin-4-sulfate, dermatan sulfate and hyaluronan are considerably higher.Citation28 These data indicate the importance of 6-O-sulfate groups in these saccharides, which are only found in chondroitin-6-sulfate and heparin/heparan sulfate. The significance of other modifications still remains unknown.
Based on site-directed mutagenesis,Citation51,Citation52 molecular modelingCitation52,Citation53 and NMR spectroscopy,Citation26,Citation50 H18, K20, R60, K64, K67 and R68 were identified as the essential residues in IL-8, participating in the interaction with heparin and heparan sulfate. These basic amino acids, located in the proximal loop and the C-terminal α-helix, are the driving force for electrostatic interaction with negatively charged carboxylic and sulfate groups of the GAGs. It is therefore not surprising that IL-8 possess the same binding regions for heparin and monosulfated GAGs like chondroitin sulfate and dermatan sulfate. Nevertheless, depending on the structure of the GAGs not only different binding constants were determined, but also differences in the involved IL-8 amino acid residues were observed. For example, using NMR spectroscopy, an interaction of chondroitin-6-sulfate with the residues R60, K67, R68 of IL-8 was demonstrated, while this was not the case in the interaction of IL-8 with chondroitin-4-sulfate.Citation28
Although an interaction of hyaluronan with IL-8 was demonstrated in diverse binding studies,Citation28,Citation54 no specific binding sites could be determined by means of NMR spectroscopy.Citation28
According to computational prognoses, it is assumed that in case of monomeric IL-8, binding of GAGs occurs parallel to the C-terminal α-helix under involvement of residues 18–23 of the N-terminal loop.Citation28,Citation52 However, the orientation of GAG molecules in an IL-8 dimer is controversial. For instance, it is supposed that, by a perpendicular orientation of GAGs to the helix axis, the two IL-8 monomers are bridged with each other.Citation27,Citation53 This conflicts with the assumption that longer GAGs bind dimeric IL-8 in a horseshoe fashion, in which respective GAG residues are again aligned in parallel to the α-helix.Citation28,Citation52,Citation55
Published studies have shown that the interaction of GAGs with a protein can be improved by sulfation.Citation27,Citation28,Citation50 However, in addition to the overall number of sulfate groups, the relative positions of sulfate groups also play an essential role.Citation50,Citation55 Hence, the interaction between GAGs and IL-8 is not only a purely electrostatically driven process, but steric interactions as well as the formation of hydrogen bonds are also crucial for its specificity.
Interaction of IL-8 with artificially modified hyaluronan
Hyaluronan is released in large amounts from different cells at infected and/or injured sites in response to various pro-inflammatory stimuli. This long, unbranched and non-sulfated polysaccharide enlarges the space between cells and matrix components in tissues. This provides better conditions for penetration of immune cells, and pathogenic and injured material will be fixed for a given time allowing their removal by phagocytes. However, high molecular weight hyaluronan features predominantly immunosuppressive and anti-inflammatory activities even at sites of inflammation and was shown to promote healing.Citation56-Citation58
Although hyaluronan does not contain sulfated residues, the future application of artificially modified hyaluronan species in three-dimensional scaffolds, in novel tissue replacement approaches and in pads for treatment of chronic wounds is an important tool in regenerative medicine. A crucial chemical modification of hyaluronan is the incorporation of sulfate groups providing the molecule with cytokine, chemokine and growth factor binding capabilities. Specific interaction of sulfated hyaluronan with human bone morphogenetic protein-4 and transforming growth factor-β1 has been demonstrated.Citation59,Citation60 We tested the adsorption of IL-8 to sulfated hyaluronans that were immobilized on a collagen matrix. Indeed, we detected substantial adsorption of IL-8 to the matrices only when they contained sulfated hyaluronan derivatives (). In our assay we used two different hyaluronan derivatives (), one sulfated in the C-6′ position (low sulfated hyaluronan) and one sulfated in the C-6′ position and additionally in the C-4′ and/or C-2 position (highly sulfated hyaluronan).Citation59,Citation61 In these samples, average degree of sulfation per dianhydro sugar unit was 1.0 and 3.1 for the low and high sulfated hyaluronan, respectively. Intriguingly, IL-8 adsorption to highly sulfated hyaluronan was significantly higher than to the low sulfated form indicating a role for 4-O-sulfation and/or 2-O-sulfation in the binding of IL-8 to artificially modified hyaluronan.
Figure 2. (A) Adsorption of human macrophage-derived IL-8 to modified hyaluronan containing different levels of sulfation. For adsorption of IL-8, native and differentially sulfated hyaluronan (kindly provided by Dr Moeller, INNOVENT e.V. Jena, Germany) immobilized on a collagen matrix were incubated with supernatants of LPS stimulated human monocyte-derived macrophages containing 138 ± 46 ng/ml IL-8. Tissue culture polystyrene was used as control substrate. After 24 h at 37°C, the supernatants were harvested and amounts of IL-8 determined by ELISA. Level of adsorbed IL-8 was calculated from the difference of the IL-8 amount determined in supernatants from the control substrate and of the IL-8 amount determined in supernatants from the different hyaluronan matrices, respectively. All data are mean (± SD) of three independent experiments. *p < 0.05; **p < 0.005 (t-test); (B) Chemical structures of native HA and sulfated HA derivatives.Citation59 coll I, collagen I; HA, hyaluronan; lsHA, low sulfated HA; hsHA, high sulfated HA
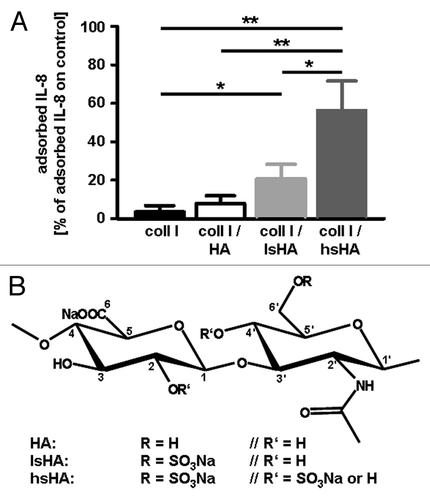
Evidence for Biological Relevance of the IL-8/glycosaminoglycan Interactions
Internalization of IL-8
At the site of infection, IL-8 is produced by extravascular cells as well as stimulated endothelial cells. After binding to heparin sulfate chains and to DARC at the abluminal surface of the endothelium, this cytokine is internalized and transcytozed to the vessel lumen.Citation34,Citation62 The immobilization of IL-8 on the cellular glycocalyx modulates the PMN recruitment.Citation63 However, the relevance of this immobilization is still a matter of controversy.
Effects of glycosaminoglycans on IL-8-mediated chemotaxis of PMNs
Several studies support the hypothesis that binding of IL-8 to surface endothelial GAGs is a crucial prerequisite for PMN attraction. A shortened IL-8 mutant, where the C-terminal GAG-binding domain has been deleted, failed to attract PMNs with the same extent as native IL-8 in vitro and in vivo.Citation62 In another study, IL-8 variants containing alanine mutations in GAG binding domains were instilled into the lungs of mice that led to higher plasma concentrations accompanied by an increased PMN infiltration.Citation64 The high plasma levels of this mutant IL-8 may be caused by its inability to bind to matrix components.
Divergent results were also obtained when chemotaxis of PMNs was assessed in vitro in Boyden chamber assays where two medium-filled chambers are separated by a porous filter membrane. In general, cells are allowed to migrate from the upper to the lower compartment containing chemotactic stimuli. In this experimental setting, the chemotactic activity of PMNs toward IL-8 was determined to occur at low nanomolar concentrations (ED50 = 0.4 ± 0.1 nM).Citation21,Citation50 To achieve a more physiological situation, GAGs have to be included into the experimental setup. Two different strategies can be followed: GAGs can be added either to the cell suspension in the upper chamber or to IL-8 in the lower chamber. Co-incubation of IL-8 with heparin or dextran sulfate decreased the chemotaxis of PMNs,Citation50,Citation65 while co-incubation with heparan sulfate either enhanced chemotaxisCitation66 or did not exert any effect.Citation65 When heparin, chondroitin sulfate or hyaluronan were co-incubated with PMNs, the migratory activity was unaffected.Citation50 While native hyaluronan had no impact on IL-8-induced PMN migration, both, low and high sulfated hyaluronan markedly reduced the chemotactic activity of PMNs upon co-incubation with IL-8 in the Boyden chamber assay (data not shown). These reports imply that investigating chemotaxis in vitro and interpreting the data demands great caution. Addition of GAGs to IL-8 potentially induces the formation of protein-GAG-aggregates that reduce the available amount of IL-8 to build up a chemotactic gradient and thereby hampers migration.Citation28 However, in vivo all three components (PMNs, IL-8 and GAGs) are present together and, thus, IL-8 may still stimulate chemotaxis. Moreover, due to dynamic equilibria between GAG-bound and soluble IL-8 as well as between monomers and dimers, different forms of IL-8 exist that may modulate PMN recruitment profiles spatiotemporal.Citation20
Effects of IL-8 on integrin functions
During the recruitment of PMNs from blood to inflammatory sites, IL-8 acts in concert with selectins and integrins. The ligation of IL-8 to its G-protein coupled receptors CXCR1 and CXCR2 initiates a signaling cascade in PMNs that passes, among others, through the activation of phospholipase C and an inositol 1,4,5-triphosphate-mediated calcium release from internal stores.Citation67 For instance, IL-8 can induce the recruitment of secretory vesicles and to a minor extent of gelatinase granules to the plasma membrane, which both contain the integrin αMβ2 (CD11b/CD18).Citation68 In this way, the cell surface is furnished with additional adhesive proteins required for a firm attachment to the vessel wall.Citation63,Citation69
Interleukin-8 is also involved in integrin activation.Citation70 In addition to CD11b/CD18, the integrin αLβ2 (CD11a/CD18) plays a central role in neutrophil migration. During IL-8-stimulated chemotaxis, CD11a/CD18 is bound to the lamellipodium, while CD11b/CD18 is redistributed to the rear of activated neutrophils.Citation71 This suggests that clustering is a possible mechanism by which IL-8 induces enhanced integrin activity. Another study indicates that CD11a/CD18 is required for the directional migration, whereas CD11b/CD18 mediates cell movement during IL-8 activation.Citation72
Effects of IL-8 on oxidative activity of PMNs
Upon reaching the site of infection, PMNs use several mechanisms to exterminate invading pathogens. Phagocytosis, secretion of hydrolyzing enzymes, microbicidal proteins and myeloperoxidase from azurophilic granules into the phagosoms as well as the production of reactive oxygen species (ROS) belong to their cytotoxic arsenal. To minimize severe collateral damage, those mechanisms have to be activated temporally and spatially restricted.
Interleukin-8 mediates substantial production of ROS in PMNs only at micromolar concentrations as evaluated by conversion of the non-fluorescent dihydrorhodamine 123 into a fluorescent product.Citation50 This concentration range is about three orders of magnitude higher than that of IL-8-induced chemotactic movement. Thus, the main function of IL-8 is to direct PMNs to inflammatory loci and to cause a priming of these cells. Subsequently, full activation of PMNs performing microbicidal functions is achieved by other stimulating agents such as bacterial products (e.g., formylated tripeptides or lipopolysaccharides), components of the complement system and others.
With respect to the influence of GAGs on the ROS production induced by IL-8 only a few information are available. We recently published a study that investigated the relevance of heparin, chondroitin sulfate and hyaluronan for this mechanism. Intriguingly, heparin and chondroitin sulfate enhanced the respiratory activity of PMNs when used in combination with IL-8, while hyaluronan was ineffective.Citation50 Hence, IL-8-mediated stimulation of PMN defense mechanisms necessitates reconsideration, whereby the influence of IL-8/GAG binding has to be taken into account for a proper understanding of the system.
Conclusions
Interleukin-8 is an important chemokine directing polymorphonuclear leukocytes to inflammatory sites. Thereby it interacts closely with sulfated polysaccharides of proteoglycans of cell surfaces and the extracellular matrix, whereby this interaction may be disturbed by enzymatic digestion of polysaccharides.Citation26,Citation28,Citation36,Citation73-Citation75 Although many details of the interplay between IL-8 with its receptors and proteoglycans are well known, we are far from understanding this role of interaction in biological processes in vivo. This is, among others, caused by the complex set of modifications that modify IL-8 and GAGs. On the one hand, different forms of IL-8 exist due to processing and citrullination. On the other hand, the large, natural heterogeneity of postsynthetic modifications in heparan sulfate chains yields an enormous diversity of sulfation and charge distribution in the proteoglycan layer already at short distances. Proteoglycan digesting enzymes that are present during inflammation further modulate the characteristics of the layer. Taken together, this makes it problematic to evaluate the significance of the IL-8-GAG-interaction in vivo.
In vitro experiments revealed the importance of 6-O-sulfation for binding of IL-8 to GAGs. However, it remains unknown which particular patterns of sulfation and dynamic alterations in these patterns contribute to the regulation of IL-8 functions in vivo.
The creation of artificially modified matrices is a fundamental tool in regenerative medicine to improve wound healing and immunological safety of graft materials. Thus, it is highly important to know how these materials interact with cytokines and immune cells. As shown on selected examples, the introduction of sulfate groups into a hyaluronan matrix significantly improves its binding of IL-8.
Thus, in immune responses IL-8 is a necessary component to direct immune cells to infected/injured sites. Hence, it is a high challenge to gain insight into further unknown details of functional and regulatory aspects of this chemokine.
Abbreviations: | ||
DARC | = | duffy antigen receptor for chemokines |
GAGs | = | glycosaminoglycans |
Gal | = | D-galactose |
GalNAc | = | N-acetyl-D-galactosamine |
GlcA | = | D-glucuronic acid |
GlcNAc | = | N-acetyl-D-glucosamine |
IdoA | = | L-iduronic acid |
IL-8 | = | interleukin-8 |
PMNs | = | polymorphonuclear leukocytes |
ROS | = | reactive oxygen species |
Acknowledgments
This work was supported by the German Research Foundation (SFB-TRR67, A6, B3) and the German Federal Ministry of Education and Research (BMBF, PtJ-Bio, 0315883).
References
- Rajarathnam K, Sykes BD, Kay CM, Dewald B, Geiser T, Baggiolini M, et al. Neutrophil activation by monomeric interleukin-8. Science 1994; 264:90 - 2; http://dx.doi.org/10.1126/science.8140420; PMID: 8140420
- Muller WA. Leukocyte-endothelial cell interactions in the inflammatory response. Lab Invest 2002; 82:521 - 33; http://dx.doi.org/10.1038/labinvest.3780446; PMID: 12003992
- Taylor KR, Gallo RL. Glycosaminoglycans and their proteoglycans: host-associated molecular patterns for initiation and modulation of inflammation. FASEB J 2006; 20:9 - 22; http://dx.doi.org/10.1096/fj.05-4682rev; PMID: 16394262
- Walker A, Ward C, Taylor EL, Dransfield I, Hart SP, Haslett C, et al. Regulation of neutrophil apoptosis and removal of apoptotic cells. Curr Drug Targets Inflamm Allergy 2005; 4:447 - 54; http://dx.doi.org/10.2174/1568010054526278; PMID: 16101521
- Vandivier RW, Henson PM, Douglas IS. Burying the dead: the impact of failed apoptotic cell removal (efferocytosis) on chronic inflammatory lung disease. Chest 2006; 129:1673 - 82; http://dx.doi.org/10.1378/chest.129.6.1673; PMID: 16778289
- Krysko DV, D’Herde K, Vandenabeele P. Clearance of apoptotic and necrotic cells and its immunological consequences. Apoptosis 2006; 11:1709 - 26; http://dx.doi.org/10.1007/s10495-006-9527-8; PMID: 16951923
- Erwig LP, Henson PM. Immunological consequences of apoptotic cell phagocytosis. Am J Pathol 2007; 171:2 - 8; http://dx.doi.org/10.2353/ajpath.2007.070135; PMID: 17591947
- Franz S, Hoeve MA, Wickert S, Janko C, Dransfield I. Clearance of apo Nph induces an immunosuppressive response in pro-inflammatory type-1 and anti-inflammatory type-2 MPhi. Autoimmunity 2009; 42:275 - 7; http://dx.doi.org/10.1080/08916930902828080; PMID: 19811275
- Arnhold J, Flemmig J. Human myeloperoxidase in innate and acquired immunity. Arch Biochem Biophys 2010; 500:92 - 106; http://dx.doi.org/10.1016/j.abb.2010.04.008; PMID: 20399194
- Clore GM, Appella E, Yamada M, Matsushima K, Gronenborn AM. Three-dimensional structure of interleukin 8 in solution. Biochemistry 1990; 29:1689 - 96; http://dx.doi.org/10.1021/bi00459a004; PMID: 2184886
- Clore GM, Appella E, Yamada M, Matsushima K, Gronenborn AM. Determination of the secondary structure of interleukin-8 by nuclear magnetic resonance spectroscopy. J Biol Chem 1989; 264:18907 - 11; PMID: 2681204
- Baggiolini M, Clark-Lewis I. Interleukin-8, a chemotactic and inflammatory cytokine. FEBS Lett 1992; 307:97 - 101; http://dx.doi.org/10.1016/0014-5793(92)80909-Z; PMID: 1639201
- Baldwin ET, Weber IT, St Charles R, Xuan JC, Appella E, Yamada M, et al. Crystal structure of interleukin 8: symbiosis of NMR and crystallography. Proc Natl Acad Sci U S A 1991; 88:502 - 6; http://dx.doi.org/10.1073/pnas.88.2.502; PMID: 1988949
- Grasberger BL, Gronenborn AM, Clore GM. Analysis of the backbone dynamics of interleukin-8 by 15N relaxation measurements. J Mol Biol 1993; 230:364 - 72; http://dx.doi.org/10.1006/jmbi.1993.1152; PMID: 8464050
- Rajarathnam K, Clark-Lewis I, Dewald B, Baggiolini M, Sykes BD. 1H NMR evidence that Glu-38 interacts with the N-terminal functional domain in interleukin-8. FEBS Lett 1996; 399:43 - 6; http://dx.doi.org/10.1016/S0014-5793(96)01277-X; PMID: 8980116
- Padrines M, Wolf M, Walz A, Baggiolini M. Interleukin-8 processing by neutrophil elastase, cathepsin G and proteinase-3. FEBS Lett 1994; 352:231 - 5; http://dx.doi.org/10.1016/0014-5793(94)00952-X; PMID: 7925979
- Proost P, Loos T, Mortier A, Schutyser E, Gouwy M, Noppen S, et al. Citrullination of CXCL8 by peptidylarginine deiminase alters receptor usage, prevents proteolysis, and dampens tissue inflammation. J Exp Med 2008; 205:2085 - 97; http://dx.doi.org/10.1084/jem.20080305; PMID: 18710930
- Mortier A, Gouwy M, Van Damme J, Proost P. Effect of posttranslational processing on the in vitro and in vivo activity of chemokines. Exp Cell Res 2011; 317:642 - 54; http://dx.doi.org/10.1016/j.yexcr.2010.11.016; PMID: 21146523
- Burrows SD, Doyle ML, Murphy KP, Franklin SG, White JR, Brooks I, et al. Determination of the monomer-dimer equilibrium of interleukin-8 reveals it is a monomer at physiological concentrations. Biochemistry 1994; 33:12741 - 5; http://dx.doi.org/10.1021/bi00209a002; PMID: 7947677
- Das ST, Rajagopalan L, Guerrero-Plata A, Sai J, Richmond A, Garofalo RP, et al. Monomeric and dimeric CXCL8 are both essential for in vivo neutrophil recruitment. PLoS One 2010; 5:e11754; http://dx.doi.org/10.1371/journal.pone.0011754; PMID: 20668677
- Clark-Lewis I, Schumacher C, Baggiolini M, Moser B. Structure-activity relationships of interleukin-8 determined using chemically synthesized analogs. Critical role of NH2-terminal residues and evidence for uncoupling of neutrophil chemotaxis, exocytosis, and receptor binding activities. J Biol Chem 1991; 266:23128 - 34; PMID: 1744111
- Rajarathnam K, Clark-Lewis I, Sykes BD. 1H NMR studies of interleukin 8 analogs: characterization of the domains essential for function. Biochemistry 1994; 33:6623 - 30; http://dx.doi.org/10.1021/bi00187a032; PMID: 8204599
- Clark-Lewis I, Dewald B, Loetscher M, Moser B, Baggiolini M. Structural requirements for interleukin-8 function identified by design of analogs and CXC chemokine hybrids. J Biol Chem 1994; 269:16075 - 81; PMID: 8206907
- Gerber N, Lowman H, Artis DR, Eigenbrot C. Receptor-binding conformation of the “ELR” motif of IL-8: X-ray structure of the L5C/H33C variant at 2.35 A resolution. Proteins 2000; 38:361 - 7; http://dx.doi.org/10.1002/(SICI)1097-0134(20000301)38:4<361::AID-PROT2>3.0.CO;2-0; PMID: 10707023
- Mortier A, Van Damme J, Proost P. Regulation of chemokine activity by posttranslational modification. Pharmacol Ther 2008; 120:197 - 217; http://dx.doi.org/10.1016/j.pharmthera.2008.08.006; PMID: 18793669
- Kuschert GSV, Coulin F, Power CA, Proudfoot AEI, Hubbard RE, Hoogewerf AJ, et al. Glycosaminoglycans interact selectively with chemokines and modulate receptor binding and cellular responses. Biochemistry 1999; 38:12959 - 68; http://dx.doi.org/10.1021/bi990711d; PMID: 10504268
- Lortat-Jacob H, Grosdidier A, Imberty A. Structural diversity of heparan sulfate binding domains in chemokines. Proc Natl Acad Sci U S A 2002; 99:1229 - 34; http://dx.doi.org/10.1073/pnas.032497699; PMID: 11830659
- Pichert A, Samsonov SA, Theisgen S, Thomas L, Baumann L, Schiller J, et al. Characterization of the interaction of interleukin-8 with hyaluronan, chondroitin sulfate, dermatan sulfate and their sulfated derivatives by spectroscopy and molecular modeling. Glycobiology 2012; 22:134 - 45; http://dx.doi.org/10.1093/glycob/cwr120; PMID: 21873605
- Hébert CA, Vitangcol RV, Baker JB. Scanning mutagenesis of interleukin-8 identifies a cluster of residues required for receptor binding. J Biol Chem 1991; 266:18989 - 94; PMID: 1918013
- Clark-Lewis I, Kim KS, Rajarathnam K, Gong JH, Dewald B, Moser B, et al. Structure-activity relationships of chemokines. J Leukoc Biol 1995; 57:703 - 11; PMID: 7759949
- Holmes WE, Lee J, Kuang WJ, Rice GC, Wood WI. Structure and functional expression of a human interleukin-8 receptor. Science 1991; 253:1278 - 80; http://dx.doi.org/10.1126/science.1840701; PMID: 1840701
- Morohashi H, Miyawaki T, Nomura H, Kuno K, Murakami S, Matsushima K, et al. Expression of both types of human interleukin-8 receptors on mature neutrophils, monocytes, and natural killer cells. J Leukoc Biol 1995; 57:180 - 7; PMID: 7829970
- Murphy PM, Baggiolini M, Charo IF, Hébert CA, Horuk R, Matsushima K, et al. International union of pharmacology. XXII. Nomenclature for chemokine receptors. Pharmacol Rev 2000; 52:145 - 76; PMID: 10699158
- Lee JS, Frevert CW, Wurfel MM, Peiper SC, Wong VA, Ballman KK, et al. Duffy antigen facilitates movement of chemokine across the endothelium in vitro and promotes neutrophil transmigration in vitro and in vivo. J Immunol 2003; 170:5244 - 51; PMID: 12734373
- Bergin DA, Reeves EP, Meleady P, Henry M, McElvaney OJ, Carroll TP, et al. α-1 Antitrypsin regulates human neutrophil chemotaxis induced by soluble immune complexes and IL-8. J Clin Invest 2010; 120:4236 - 50; http://dx.doi.org/10.1172/JCI41196; PMID: 21060150
- Hoogewerf AJ, Kuschert GSV, Proudfoot AEI, Borlat F, Clark-Lewis I, Power CA, et al. Glycosaminoglycans mediate cell surface oligomerization of chemokines. Biochemistry 1997; 36:13570 - 8; http://dx.doi.org/10.1021/bi971125s; PMID: 9354625
- Kjellén L, Lindahl U. Proteoglycans: structures and interactions. Annu Rev Biochem 1991; 60:443 - 75; http://dx.doi.org/10.1146/annurev.bi.60.070191.002303; PMID: 1883201
- Iozzo RV. Matrix proteoglycans: from molecular design to cellular function. Annu Rev Biochem 1998; 67:609 - 52; http://dx.doi.org/10.1146/annurev.biochem.67.1.609; PMID: 9759499
- Esko JD, Selleck SB. Order out of chaos: assembly of ligand binding sites in heparan sulfate. Annu Rev Biochem 2002; 71:435 - 71; http://dx.doi.org/10.1146/annurev.biochem.71.110601.135458; PMID: 12045103
- Park PW, Reizes O, Bernfield M. Cell surface heparan sulfate proteoglycans: selective regulators of ligand-receptor encounters. J Biol Chem 2000; 275:29923 - 6; http://dx.doi.org/10.1074/jbc.R000008200; PMID: 10931855
- Uchimura K, Morimoto-Tomita M, Bistrup A, Li J, Lyon M, Gallagher J, et al. HSulf-2, an extracellular endoglucosamine-6-sulfatase, selectively mobilizes heparin-bound growth factors and chemokines: effects on VEGF, FGF-1, and SDF-1. BMC Biochem 2006; 7:2; http://dx.doi.org/10.1186/1471-2091-7-2; PMID: 16417632
- Parish CR. The role of heparan sulphate in inflammation. Nat Rev Immunol 2006; 6:633 - 43; http://dx.doi.org/10.1038/nri1918; PMID: 16917509
- Iozzo RV. Heparan sulfate proteoglycans: intricate molecules with intriguing functions. J Clin Invest 2001; 108:165 - 7; PMID: 11457866
- Rops AL, van der Vlag J, Lensen JF, Wijnhoven TJ, van den Heuvel LP, van Kuppevelt TH, et al. Heparan sulfate proteoglycans in glomerular inflammation. Kidney Int 2004; 65:768 - 85; http://dx.doi.org/10.1111/j.1523-1755.2004.00451.x; PMID: 14871397
- Jackson RL, Busch SJ, Cardin AD. Glycosaminoglycans: molecular properties, protein interactions, and role in physiological processes. Physiol Rev 1991; 71:481 - 539; PMID: 2006221
- Couchman JR, Woods A. Syndecans, signaling, and cell adhesion. J Cell Biochem 1996; 61:578 - 84; http://dx.doi.org/10.1002/(SICI)1097-4644(19960616)61:4<578::AID-JCB11>3.0.CO;2-C; PMID: 8806081
- Stringer SE, Gallagher JT. Heparan sulphate. Int J Biochem Cell Biol 1997; 29:709 - 14; http://dx.doi.org/10.1016/S1357-2725(96)00170-7; PMID: 9251237
- Perrimon N, Bernfield M. Specificities of heparan sulphate proteoglycans in developmental processes. Nature 2000; 404:725 - 8; http://dx.doi.org/10.1038/35008000; PMID: 10783877
- Gandhi NS, Mancera RL. The structure of glycosaminoglycans and their interactions with proteins. Chem Biol Drug Des 2008; 72:455 - 82; http://dx.doi.org/10.1111/j.1747-0285.2008.00741.x; PMID: 19090915
- Schlorke D, Thomas L, Samsonov SA, Huster D, Arnhold J, Pichert A. The influence of glycosaminoglycans on IL-8-mediated functions of neutrophils. Carbohydr Res 2012; 356:196 - 203; http://dx.doi.org/10.1016/j.carres.2012.02.025; PMID: 22444322
- Kuschert GS, Hoogewerf AJ, Proudfoot AE, Chung CW, Cooke RM, Hubbard RE, et al. Identification of a glycosaminoglycan binding surface on human interleukin-8. Biochemistry 1998; 37:11193 - 201; http://dx.doi.org/10.1021/bi972867o; PMID: 9698365
- Krieger E, Geretti E, Brandner B, Goger B, Wells TN, Kungl AJ. A structural and dynamic model for the interaction of interleukin-8 and glycosaminoglycans: support from isothermal fluorescence titrations. Proteins 2004; 54:768 - 75; http://dx.doi.org/10.1002/prot.10590; PMID: 14997572
- Bitomsky W, Wade RC. Docking of glycosaminoglycans to heparin-binding proteins: validation for aFGF, bFGF, and antithrombin and application to IL-8. J Am Chem Soc 1999; 121:3004 - 13; http://dx.doi.org/10.1021/ja983319g
- David R, Günther R, Baumann L, Lühmann T, Seebach D, Hofmann HJ, et al. Artificial chemokines: combining chemistry and molecular biology for the elucidation of interleukin-8 functionality. J Am Chem Soc 2008; 130:15311 - 7; http://dx.doi.org/10.1021/ja802453x; PMID: 18942784
- Spillmann D, Witt D, Lindahl U. Defining the interleukin-8-binding domain of heparan sulfate. J Biol Chem 1998; 273:15487 - 93; http://dx.doi.org/10.1074/jbc.273.25.15487; PMID: 9624135
- Day AJ, de la Motte CA. Hyaluronan cross-linking: a protective mechanism in inflammation?. Trends Immunol 2005; 26:637 - 43; http://dx.doi.org/10.1016/j.it.2005.09.009; PMID: 16214414
- Jiang D, Liang J, Fan J, Yu S, Chen S, Luo Y, et al. Regulation of lung injury and repair by Toll-like receptors and hyaluronan. Nat Med 2005; 11:1173 - 9; http://dx.doi.org/10.1038/nm1315; PMID: 16244651
- Bollyky PL, Falk BA, Wu RP, Buckner JH, Wight TN, Nepom GT. Intact extracellular matrix and the maintenance of immune tolerance: high molecular weight hyaluronan promotes persistence of induced CD4+CD25+ regulatory T cells. J Leukoc Biol 2009; 86:567 - 72; http://dx.doi.org/10.1189/jlb.0109001; PMID: 19401397
- Hintze V, Moeller S, Schnabelrauch M, Bierbaum S, Viola M, Worch H, et al. Modifications of hyaluronan influence the interaction with human bone morphogenetic protein-4 (hBMP-4). Biomacromolecules 2009; 10:3290 - 7; http://dx.doi.org/10.1021/bm9008827; PMID: 19894734
- Hempel U, Hintze V, Möller S, Schnabelrauch M, Scharnweber D, Dieter P. Artificial extracellular matrices composed of collagen I and sulfated hyaluronan with adsorbed transforming growth factor β1 promote collagen synthesis of human mesenchymal stromal cells. Acta Biomater 2012; 8:659 - 66; http://dx.doi.org/10.1016/j.actbio.2011.10.026; PMID: 22061106
- Kunze R, Rösler M, Möller S, Schnabelrauch M, Riemer T, Hempel U, et al. Sulfated hyaluronan derivatives reduce the proliferation rate of primary rat calvarial osteoblasts. Glycoconj J 2010; 27:151 - 8; http://dx.doi.org/10.1007/s10719-009-9270-9; PMID: 19941065
- Middleton J, Neil S, Wintle J, Clark-Lewis I, Moore H, Lam C, et al. Transcytosis and surface presentation of IL-8 by venular endothelial cells. Cell 1997; 91:385 - 95; http://dx.doi.org/10.1016/S0092-8674(00)80422-5; PMID: 9363947
- Carveth HJ, Bohnsack JF, McIntyre TM, Baggiolini M, Prescott SM, Zimmerman GA. Neutrophil activating factor (NAF) induces polymorphonuclear leukocyte adherence to endothelial cells and to subendothelial matrix proteins. Biochem Biophys Res Commun 1989; 162:387 - 93; http://dx.doi.org/10.1016/0006-291X(89)92009-3; PMID: 2665743
- Tanino Y, Coombe DR, Gill SE, Kett WC, Kajikawa O, Proudfoot AE, et al. Kinetics of chemokine-glycosaminoglycan interactions control neutrophil migration into the airspaces of the lungs. J Immunol 2010; 184:2677 - 85; http://dx.doi.org/10.4049/jimmunol.0903274; PMID: 20124102
- Ramdin L, Perks B, Sheron N, Shute JK. Regulation of interleukin-8 binding and function by heparin and alpha2-macroglobulin. Clin Exp Allergy 1998; 28:616 - 24; http://dx.doi.org/10.1046/j.1365-2222.1998.00283.x; PMID: 9645599
- Webb LMC, Ehrengruber MU, Clark-Lewis I, Baggiolini M, Rot A. Binding to heparan sulfate or heparin enhances neutrophil responses to interleukin 8. Proc Natl Acad Sci U S A 1993; 90:7158 - 62; http://dx.doi.org/10.1073/pnas.90.15.7158; PMID: 8346230
- Lomakina EB, Waugh RE. Signaling and dynamics of activation of LFA-1 and Mac-1 by immobilized IL-8. Cell Mol Bioeng 2010; 3:106 - 16; http://dx.doi.org/10.1007/s12195-009-0099-x; PMID: 21532911
- Sengeløv H, Kjeldsen L, Diamond MS, Springer TA, Borregaard N. Subcellular localization and dynamics of Mac-1 (α m β 2) in human neutrophils. J Clin Invest 1993; 92:1467 - 76; http://dx.doi.org/10.1172/JCI116724; PMID: 8376598
- Detmers PA, Lo SK, Olsen-Egbert E, Walz A, Baggiolini M, Cohn ZA. Neutrophil-activating protein 1/interleukin 8 stimulates the binding activity of the leukocyte adhesion receptor CD11b/CD18 on human neutrophils. J Exp Med 1990; 171:1155 - 62; http://dx.doi.org/10.1084/jem.171.4.1155; PMID: 1969919
- Ma YQ, Plow EF, Geng JG. P-selectin binding to P-selectin glycoprotein ligand-1 induces an intermediate state of alphaMbeta2 activation and acts cooperatively with extracellular stimuli to support maximal adhesion of human neutrophils. Blood 2004; 104:2549 - 56; http://dx.doi.org/10.1182/blood-2004-03-1108; PMID: 15217824
- Seo SM, McIntire LV, Smith CW. Effects of IL-8, Gro-α, and LTB(4) on the adhesive kinetics of LFA-1 and Mac-1 on human neutrophils. Am J Physiol Cell Physiol 2001; 281:C1568 - 78; PMID: 11600420
- Heit B, Colarusso P, Kubes P. Fundamentally different roles for LFA-1, Mac-1 and α4-integrin in neutrophil chemotaxis. J Cell Sci 2005; 118:5205 - 20; http://dx.doi.org/10.1242/jcs.02632; PMID: 16249234
- Goger B, Halden Y, Rek A, Mösl R, Pye D, Gallagher J, et al. Different affinities of glycosaminoglycan oligosaccharides for monomeric and dimeric interleukin-8: a model for chemokine regulation at inflammatory sites. Biochemistry 2002; 41:1640 - 6; http://dx.doi.org/10.1021/bi011944j; PMID: 11814358
- Witt DP, Lander AD. Differential binding of chemokines to glycosaminoglycan subpopulations. Curr Biol 1994; 4:394 - 400; http://dx.doi.org/10.1016/S0960-9822(00)00088-9; PMID: 7922353
- Frevert CW, Kinsella MG, Vathanaprida C, Goodman RB, Baskin DG, Proudfoot A, et al. Binding of interleukin-8 to heparan sulfate and chondroitin sulfate in lung tissue. Am J Respir Cell Mol Biol 2003; 28:464 - 72; http://dx.doi.org/10.1165/rcmb.2002-0084OC; PMID: 12654635