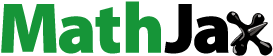
Abstract
The objective in this study was to develop an osteoconductive, biodegradable and rifampicin releasing bone filling composite material for the treatment of osteomyelitis, a bacterial infection of bone that is very difficult and expensive to treat. The composite material will be used together with a ciprofloxacin releasing composite, because of the rapid development of resistant bacteria when rifampicin is used alone. Three composites were manufactured by twin-screw extrusion. The polymer matrix for the composites was poly(L-lactide-co-ε-caprolactone) 70/30 and all the composites contained 8 wt% (weight percent) of rifampicin antibiotic. The β-TCP contents of the composites were 0 wt%, 50 wt% and 60 wt%. The composites were sterilized by gamma irradiation before in vitro degradation and drug release tests. The hydrolytical degradation of the studied composites proceeded quickly and the molecular weight of the polymer component of the composites decreased rapidly. Rifampicin release occurred in four phases in which the high β-TCP content of the samples, polymer degradation and mass loss all played a role in determining the phases. The ceramic component was seen to have a positive effect on the drug release. The composite with 50 wt% of β-TCP showed the most promising rifampicin release profile and it also showed activity against a common osteomyelitis causing bacteria Pseudomonas aeruginosa. A clear inhibition zone was formed in 16 h incubation. Overall, the tested materials showed great potential to be developed into a bone filler material for the treatment of osteomyelitis or other bone related infections in combination with the ciprofloxacin releasing materials.
Introduction
This paper presents the in vitro degradation and drug release results of three rifampicin releasing composite materials as well as the effect of the most promising rifampicin releasing composite against a common osteomyelitis causing bacteria Pseudomonas aeruginosa. The materials are intended for use in the treatment of osteomyelitis or other bone related infections together with the ciprofloxacin releasing composite materials presented in our accompanying study.Citation1 The composites are bioabsorbable and osteoconductive due to the degradation ability of the polymer matrix (Poly-L-lactide-co-ε-caprolactone) (PLCL) and the osteocoductive properties of the ceramic filler, β-tricalcium phosphate (β-TCP). It is envisaged that these materials will enable the simultaneous use of bone fillers that contain different antibiotics. The surgeon applying the fillers will be able to decide which antibiotics and in which ratio to use based on the condition of the patient and the pathogen in question. Such materials have also been requested in the literature.Citation2
Osteomyelitis, which is very challenging and expensive to treat, is a bone infection that is caused by bacteria, commonly Staphylococcus aureus, Pseudomonas aeruginosa or Staphylococcus epidermidis.Citation3 Traditional treatment includes surgical debridement of the infected tissue followed by long courses intravenously or orally administered antibiotics.Citation4 Poor blood circulation in the infected bone tissue can prevent adequate antibiotic concentrations in the infection site being achieved. To overcome this problem, local antibiotic delivery has been applied with commercially available gentamycin releasing Septopal® beads. The problem with this method of antibiotic delivery is that the beads are not biodegradable and require surgical removal followed by bone grafting.Citation5,Citation6
Rifampicin has been used together with ciprofloxacin and other fluoroquinolones in the treatment of osteomyelitis or other bone related infections. The use of rifampicin together with other antibiotics in the treatment of Staphylococcus aureus infections has been reviewed by Perlroth et al.Citation7 In general, in in vivo and human studies, the combination of ciprofloxacin with rifampicin was more effective than monotherapy especially in prosthetic device infections and osteomyelitis.Citation8 Rifampicin has also been studied together with fluoroquinolones in the treatment of deep sternal wound infections and studies have shown that using rifampicin together with fluoroquinolones improves the outcome.Citation9 The fact that rifampicin is effective against bacterial biofilms encourages the use of rifampicin together with other antibiotics.Citation7,Citation10 Rifampicin should never be used alone because resistant bacterial strains develop quite rapidly as a result.Citation7,Citation10
In most of the studies reported about the combination therapy of rifampicin with other antibiotics, the delivery route of the antibiotics has been either intravenous or oral. With local antibiotic treatment, bone tissue that lacks adequate blood circulation can be effectively treated and the pathogens eradicated.Citation11-Citation19 Previous studies have also shown that with local treatment, the drug concentrations in the blood or other tissues are low, at least a decade lower than in the surrounding tissues,Citation20-Citation22 which naturally leads to decreased side effects like nausea which has been reported often as the cause for discontinuation of the therapy or continuing the therapy with lower dose.Citation8,Citation10
In this study, the potential of the materials to release rifampicin in adequate concentrations and the degradation were only tested in vitro. There is still a need for in vivo and clinical testing of the materials. The materials do, however, show great potential for use in the treatment of osteomyelitis and other bone related infections and are now ready to be tested further in vivo.
Results and Discussion
The effect of processing and sterilization on the materials
The processing method used for the composite materials was twin-screw extrusion and it can be assumed that the ceramic and drug particles were evenly distributed due to the efficient mixing in the extrusion process. The composites are denoted PLCL + R [Poly(L-lactide-co-ε-caprolactone) (PLCL) with 8 wt% of rifampicin in feed], PLCL + TCP50 + R [PLCL with 50 wt% of β-tricalcium phosphate (β-TCP) and 8 wt% of rifampicin in feed] and PLCL + TCP60 + R (PLCL with 50 wt% β-TCP and 8 wt% of rifampicin in feed). Processing did not cause degradation detectable with SEC measurements. However, sterilization using gamma irradiation with a measured dose of 29–35 kGy caused significant degradation, as was expected.Citation23 The average molecular weight (Mw) of the raw material was measured as 246,000 g/mol and the number average molecular weight (Mn) 150,000 g/mol. The Mw of the PLCL + R decreased 30% during the sterilization stage and the Mw of the PLCL + TCP50 + R and PLCL + TCP60 + R decreased 40% and 30%, respectively. The Mn decreased 40% for all the composites during the sterilization stage. The polydispersity of PLCL + R did not change during processing but increased from 1.6 to 2.0 during sterilization. For PLCL + TCP50 + R and PLCL + TCP60 + R, the PD decreased from 1.6 to 1.5 during processing and increased slightly to 1.8 for PLCL + TCP50 + R and 1.6 for PLCL + TCP60 + R.
The residual monomer content of the raw material measured by gas chromatography was 0.08 wt% for L-lactide monomer and below detection limit (< 0.02 wt%) for ε-caprolactone monomer. The L-lactide and ε-caprolactone monomer contents of the processed samples were analyzed from two points in the processing batch and there were two parallel samples in both. The L-lactide monomer content decreased slightly during processing. It was 0.04–0.07 mol% and the caprolactone monomer content was below 0.02 mol% for all tested samples. Because there were no significant differences in the monomer contents of the manufactured materials, it can be assumed that the monomers did not cause differences in the hydrolytic degradation behavior of the studied composites.Citation24
UV measurements utilizing the isosbestic point
The UV-measurements of rifampicin proved challenging due to the oxidation of rifampicin to rifampicin quinone in aqueous solutions and in the presence of atmospheric oxygen. This can be seen as a change in the UV-spectrum of a rifampicin solution as well as a change in the color of the solution.Citation25 Rifampicin quinone has a UV-spectrum partly similar to rifampicin and also has antibacterial properties.Citation25 The fact that rifampicin quinone degrades further to other compounds that do not have absorbance in the UV/V is area naturally affects the accuracy of this method. Part of the rifampicin is undetected if it has already degraded. The accuracy is highest when the measurements are made at short intervals, not letting the dissolution medium stay unchanged for long periods. However, as the release test period in this study was long, part of the rifampicin that had oxidized to rifampicin quinone, had time to degrade to other compounds that do not have absorptivity in the UV/V is area, even if the measurements were performed in short intervals. It has also been reported that rifampicin degrades more in solutions with low rifampicin concentration.Citation26 On the other hand, Le Guellec et al. suggest possible in vivo stabilization of the molecule.Citation27
In the later stages of the release test period, it was noticed that the degradation products of the polymer matrix induce a broad UV-peak at the beginning of the scanned area (200–220 nm). This broad peak, however, did not significantly interfere with the use of the isosbestic point at 226 nm.
Rifampicin release from the materials
The measured initial rifampicin contents were 6.5 wt% for PLCL + R, 7.9 wt% for PLCL + TCP50 + R and 7.8 wt% for PLCL + TCP60 + R.
The cumulative release of rifampicin from the studied materials is presented in . It can be seen that the release occurred in four phases and that the β-TCP content of the composites had a significant effect on the rifampicin release. Rifampicin release from the PLCL + TCP60 + R was faster than from the PLCL + TCP50 + R and reached 80% in 130 d. Rifampicin release from the PLCL + TCP50 + R was slower but closer to zero order release that is the desirable release profile in this case. After 150 d, the release slowed down. The rifampicin release from PLCL + R showed a lag phase at the beginning of the test series that lasted about 50 d, during which time the release of the drug was very slow. When compared with the ciprofloxacin release,Citation1 the lag phase lasted longer. This is likely due to the fact that the rifampicin molecule is larger than ciprofloxacin, and this causes slower diffusion through the polymer matrix and the polymer degradation and mass loss have a stronger effect on the release.
Figure 1. The cumulative (A) and daily (B) release of rifampicin (R) from composites of poly(L-lactide-co-ε-caprolactone) (PLCL) and β-tricalcium phosphate (TCP) with initial TCP contents of 0 wt%, 50 wt% and 60 wt% and rifampicin content of 8 wt%. Results shown as averages with standard deviations (n = 5).
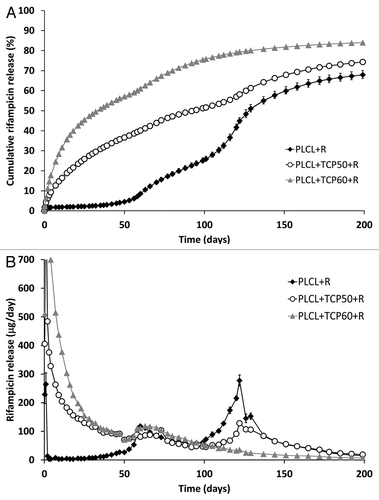
Because of the complex nature of the rifampicin release, the phases of the release should be considered separately. There are several factors affecting the drug release in this case including: the geometry of the device (cylindrical with a rough surface), high β-TCP content that introduces porosity to the matrix, the properties of the polymer change as it degrades and mass loss. The acceleration of the drug release around the inflection points in the cumulative release curves () can be easily seen in , where the daily release of rifampicin is presented. The presence of the inflection points in the cumulative release profile is characteristic for a release mechanism that is a combination of diffusion and erosion mechanisms.Citation28
The first phase of the release was the burst at the beginning of the release. It was during this phase that the rifampicin molecules near the surface were released. The burst in the release was larger than what was observed for ciprofloxacin releasing pellets.Citation1 Rifampicin is more soluble in water than ciprofloxacin which may be the reason for the larger burst. The second phase of the release lasted approximately until the time point of 53 d. During this phase, the release was mainly governed by diffusion and the release from PLCL + R showed a lag phase with almost negligible release. From these observations, it can be concluded that the release of rifampicin could not occur solely by diffusion through the plain polymer matrix. The addition of β-TCP to the polymer matrix accelerated the release markedly. β-TCP was likely to introduce porosity to the composite, and thus the rifampicin release could occur via pore diffusion in the time period up to 53 d. The composites containing both rifampicin and β-TCP can be classified as complex monolithic dispersions because of the high amount of filler in the composite. In these cases, the release of the drug is often proportional to the square root of time, but the rate of the release is higher than what is predicted by the Higuchi model.Citation28 The second phase of the rifampicin release from the composites was proportional to t1/2 with R2 having values of 0.99 for PLCL+TCP50+R, and 0.98 for PLCL+TCP60+R. The results were also fitted to the to the power law kinetics equation (Eqn. 1):Citation28,Citation29
where Mt/M∞ is the fractional drug release, k is the release rate constant, t is time and n the release exponent. The rifampicin release from PLCL + TCP50 + R and PLCL + TCP60 + R had n values of 0.53 and 0.44 respectively. For cylindrical geometry and Fickian diffusion, the diffusion release exponent n would have a value of 0.45. For Case II diffusion (or anomalous diffusion), the n has values of 0.45 < n < 1. The result suggests that the rifampicin release occurs by Case II diffusionCitation28 from the PLCL + TCP50 and by Fickian diffusion from PLCL + TCP60 + R during the second phase of the release.
In the third phase, the drug release was accelerated due to the increased permeability of the polymer caused by degradation. Additionally, the polymer erosion started to play a role in increasing the release rate. With a non-eroding system, the drug release would slowly decrease because it is often proportional to the drug concentration in the device. In the case of a biodegradable system, the increased permeability and polymer erosion accelerate the release.Citation28 Here, the third phase of the release of PLCL + TCP50 + R obeyed again the t1/2 kinetics very well (R2 = 0.99). The release from PLCL + TCP60 + R had at this point reached 60% of the total cumulative release.
There was also a fourth phase to be seen in the release of PLCL + R and PLCL + TCP50 + R but not in the PLCL + TCP60 + R. The fourth phase of the release was also not seen in the ciprofloxacin release results.Citation1 The inflection point in the release curves was at the time point of 116 d. It coincided well with the acceleration of mass loss from the composites. The mass loss was already notable at that time and the Mw of the polymer had decreased to the level of 9,000–14,000 g/mol. From the PLCL + TCP60 + R, the available drug for release had apparently been released already at this time point and thus no phase change was seen there.
Rifampicin remaining in the samples after the in vitro test series
At the time point of 250 d, the drug release had decreased to an almost negligible level and 70–85% of the total rifampicin loaded in the composites had been released. The test series was continued for 392 d but no notable rifampicin release was detected. The remaining rifampicin in the composites was measured after the drug release test had been terminated. The measurement was done in a similar way to the initial rifampicin content measurements. The brownish red color of the pellet-shaped samples indicated that some rifampicin remained in the samples even though drug release had ceased. There has been evidence about rifampicin being incorporated within the crystalline parts of a polycaprolactone polymer and this might be the case here although the crystals may be formed of L-lactide units.Citation30 The remaining portion of the initial rifampicin that was measured after the termination of the test series was 10.7% for PLCL + R, 5.0% for PLCL + TCP50 + R and 3.4% for PLCL + TCP60 + R. When combined with the measured released rifampicin, the total rifampicin amount detected did not reach 100%. The fact that rifampicin oxidizes to rifampicin quinone which is further degraded to compounds with no UV-absorbance, explains why part of the rifampicin seems to have disappeared.Citation25 Unfortunately, this affects the accuracy of this method in analyzing the released rifampicin. However, the method can be used to estimate the rifampicin release and compare the composites with each other. In in vivo conditions, the situation may be different. Le Guellec et al.Citation27 found that rifampicin stability was better in rifampicin containing plasma samples taken from patients treated with rifampicin than in plasma samples where rifampicin was added in the laboratory. This improved stability suggests that rifampicin may be more stable in vivo than in vitro.
Inhibition zone testing
The effect of the rifampicin releasing composite containing 50 wt% of β-TCP against the common osteomyelitis causing bacteria Pseudomonas aeruginosa was tested using bioluminescence imaging. The results are illustrated with , where the bioluminescence imaging results (16 h incubation) of a 6-well plate cultured with Pseudomonas aeruginosa and rifampicin containing composite pellets are shown. The antibiotic containing composite pellets are on the lower row and control pellets without antibiotics are on the top row. The results show that the composite material releases rifampicin in levels high enough to eradicate Pseudomonas aeruginosa. The inhibition zone can be seen as dark blue area surrounding the antibiotic releasing pellets in . The blue area indicates dead bacteria whereas the other colors indicate still living bacteria. First signs of the forming inhibition zone were observed already after two hours of incubation. If compared with the bioluminescence results of ciprofloxacin releasing pelletsCitation1 the inhibition zone was smaller with rifampicin. The larger molecular weight of rifampicin may affect the diffusion of the antibiotic in agar and make it rather slow, which is seen as the slow growth of the inhibition zone. However, as seen in the drug release results, the initial burst was larger with rifampicin releasing pellets than with ciprofloxacin pellets and this is probably due to the better solubility of rifampicin.
Figure 2. Bioluminescence results of the rifampicin containing (8 wt%) composites of poly(L-lactide-co-ε-caprolactone) and 50 wt% of β-tricalcium phosphate on a bacterial culture of light emitting Pseudomonas aeruginosa. Pellets containing rifampicin are on the lower row and corresponding composites without rifampicin are on the top row and act as controls.
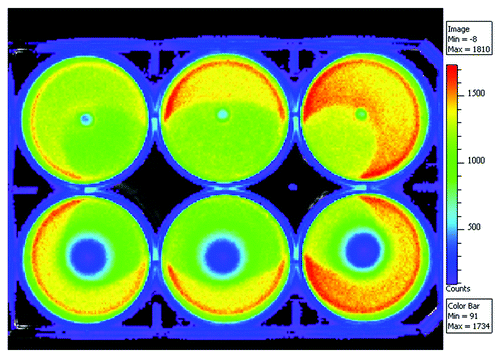
In vitro degradation of the composites
Molecular structure
The 1H NMR spectra were measured from eight samples (raw material PLCL, plain rifampicin, PLCL + R at 0, 26 and 52 weeks and PLCL + TCP50 + R at 0, 26 and 52 weeks in vitro). The 1H NMR signals of the polymer were assigned and the data were analyzed in the same way as in our accompanying study.Citation1 The results show a similar effect of change in the comonomer ratio of the copolymer as the hydrolysis proceeded that was seen with ciprofloxacin antibiotic containing composites.Citation1 The L-lactide to ε-caprolactone molar ratio was increased from 68/32 of the raw material and the samples at 0 weeks to 77/23 of the PLCL + TCP50 + C and 82/18 of the PLCL + C at 52 weeks. A similar effect has also been reported by In Jeong et al.Citation31
The microstructure of the copolymer was also studied similarly to what was presented in our accompanying study.Citation1 It was done according to HerbertCitation32 and Ferńndez.Citation33 The results of the average sequence length calculations showed the expected results based on the findings of the ciprofloxacin containing materials.Citation1 The PLCL copolymer had a block structure with more random parts in between. The randomness factor R had a value of 0.25 for the raw material and close to that for the samples prior to in vitro testing. The R factor decreased dramatically during the degradation of the polymer to 0.18 for both PLCL + TCP50 + R and PLCL + R at 52 weeks. This decrease indicates that the more random parts of the copolymer degrade first and the blocky structures that comprise long L-lactide blocks remain in the structure. At the beginning of the test series, the sequence lengths of L-lactide were 13 and increased to 23 and 31 for PLCL + TCP50 + R and PLCL + R at 52 weeks respectively. This supports the general interpretation that random parts of the copolymer degrade first, and thus the average sequence length is increased. The average sequence length of ε-caprolactone was not significantly changed during hydrolysis for any of the tested samples and had values of 5.5–7 for all the analyzed samples.
Signals of rifampicin were visible in the plain rifampicin sample and for the composite samples only in the 0 week (after processing and sterilization) samples. Due to the rather low sensitivity of NMR, the low contents of rifampicin in the samples from the 26- and 52-week time points were not seen in the NMR spectra. The 1H spectrum of plain rifampicin was compared with the spectra of the 0 week samples. 1H NMR analysis reported by Cellai et al. was also used when the signals were assigned to the protons of the rifampicin molecule.Citation34
Some of the rifampicin signals overlapped with the polymer signal in the composite samples and could not be analyzed. These were some of the protons in the ansa chain (protons 16, 18, 20, 21, 22, 2 and 31), protons 32 of the naphtalene ring, and protons 59 of the methyl group attached to the piperazinyl ring. The signal assignment is presented as and the spectra can be found as supplementary data.
The amide proton and the OH-groups of the naphthalene ring were clearly visible at δ 11.98 ppm, 13.11 ppm and 13.41 ppm, respectively. Their chemical shifts were stable and the integrals were in good stoichiometric ratio. The protons of the methylene group located above the plane of the naphthalene ring (protons 45) showed a signal below 0 at δ -0.31 ppm in plain unprocessed rifampicin and processed PLCL + R and PLCL + TCP50 + R samples. The fact that this signal is identical in all the samples proves that the ansa chain has retained its original configuration. If not, the signal of this group would have appeared elsewhere in the spectrum. The ansa bridge protons 13, 14, 15, 17, 19, 23 and 25 were clearly visible, stable when compared between the samples, and were in good stoichimetric agreement with the rifampicin structure. The signals of the protons 37, 39 and 41 of the methyl substituents of the ansa chain and the signals of the protons 54 and 56 of the piperazine ring were in the shoulder of a large polymer signal, and thus their accurate integration was not possible. Additionally, signals of the equivalent protons 53 and 57 of piperazine ring appeared at δ 3.02 overlapping with signals of the protons 19 and 47 and thus could not be accurately integrated. However, their combined integral showed that there was no change between samples in either the positions of the signals or their integrals.
As a conclusion of the NMR analysis of rifampicin, it can be stated that no deviations of the original rifampicin structure were found after the analysis of the composite samples and plain rifampicin sample. Thus, rifampicin had maintained its original structure during the processing and sterilization stages.
Molecular weights, mass loss and water absorption
The decrease of the molecular weights (both Mw and Mn) was rapid (), and when compared with the composites with ciprofloxacin antibiotic reported in the accompanying study,Citation1 there were no significant differences in the degradation behavior. At the beginning of the hydrolysis test series, the molecular weight of the composites containing antibiotics degraded more rapidly than composites without antibiotics (Ahola et al., accepted to Journal of Biomaterial Applications), but the differences rapidly leveled out. Overall, the degradation obeyed first order kinetics with k values of 1.6 × 10−3 1/h for PLCL + R, 1.4 × 10−3 1/h for PLCL + TCP50 + R, and 1.4 × 10−3 1/h for PLCL + TCP60 + R. The values are very similar to those calculated for ciprofloxacin containing composites.Citation1 After 52 weeks of hydrolysis at 37°C and pH 7.4, the molecular weights of all the composites had decreased by 98% to 2% of the initial value of the raw material.
Figure 4. Weight average molar weight (Mw) (A), and mass loss and water absorption (B) of the studied composites as a function of time in vitro. The composites comprised of poly(L-lactide-co-ε-caprolactone) (PLCL) and β-tricalcium phosphate (TCP) and rifampicin (R) with initial TCP contents of 0 wt%, 50 wt% and 60 wt% and rifampicin content of 8 wt%. Error bars in part B are not visible due to the small values of standard deviations.
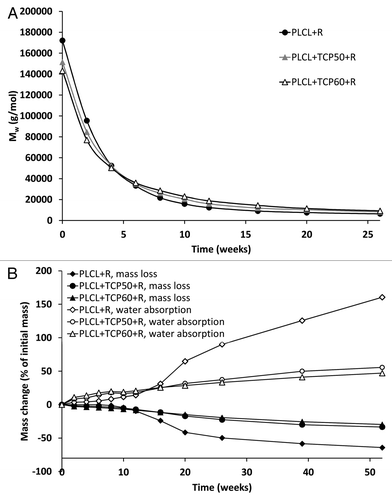
The SEC distribution plots showed emerging bimodality at 20 weeks for PLCL + R and clear bimodality at 39 weeks for PLCL + TCP50 + R and PLCL + TCP60 + R. The same kind of bimodality is present in composites with ciprofloxacin antibioticCitation1 beginning from the 20th week of the test series. Bimodality in the SEC distribution suggest that the blocky structure of the copolymer, shown by the 1H NMR analysis, causes the random parts of the copolymer to degrade first and this might cause an increase in a certain part of the SEC distribution curve as the blocky parts comprising mainly L-lactide monomers remain in the polymer structure.
The mass loss of the tested materials was small and steady during the first ten weeks. () During the first six weeks, the mass loss was caused by the rifampicin release from the polymer matrix. After this time point, the mass loss of the polymer started. At the time point of 10 weeks, the mass loss of PLCL + R accelerated and had already reached 50% at 26 weeks. The mass losses of PLCL + TCP50 + R and PLCL + TCP60 + R were steady and proceeded similarly for both of the composites. The same kind of behavior was seen in the water absorption of the tested materials. The PLCL + R showed accelerated water absorption after 10 weeks. When compared with the results of ciprofloxacin containing composites,Citation1 the acceleration of the water absorption and mass loss started earlier. Rifampicin is likely to be more hydrophilic due to its molecular structure, and thus increases the water absorption of the material.
β-TCP contents of the materials
The proportion of β-TCP in the composites was seen to increase as polymer degradation proceeded, as shown in . The increase in the proportion of β-TCP accelerated after 10 weeks in vitro which correlates well with the acceleration of the mass loss of the polymer matrix. β-TCP remained in the samples due to the very slow dissolution of β-TCP (Ahola et al. accepted to Journal of Biomaterials Applications).
Figure 5. β-tricalcium phosphate (TCP) contents of the studied composites as a function of time in vitro. The composites comprised of poly(L-lactide-co-ε-caprolactone) (PLCL), TCP, and rifampicin (R) with initial TCP contents of 50 wt% (TCP50), and 60 wt% (TCP60) and rifampicin content of 8 wt%. Results shown as averages with standard deviations (n = 5).
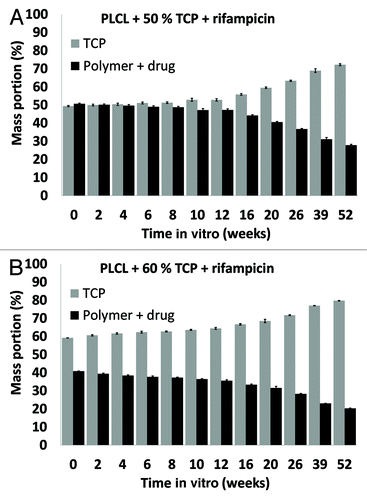
Thermal properties
The changes in the glass transition temperatures (Tg) of the materials during the in vitro test series are shown in . The Tg values presented in were taken from the second heating to ensure comparable values of the samples as their thermal histories were similar. Tgs of 23–25°C were measured after processing and sterilization of the composites. This is in agreement with the literature.Citation35 During the degradation test series, the Tgs of the rifampicin containing composites decreased until week 12. The same kind of decrease was observed with composites with ciprofloxacin but the decrease time period was longer, up to the 20th week.Citation1 During the first 12 weeks, the Mw of the polymer decreased dramatically, which is known to affect the Tg of a polymer.Citation36 Additionally, the solubility of the drug in the polymer may play a role in the glass transition of the polymers and may plasticize the polymer causing a decrease in Tg. An increase in the Tg after 12 weeks in vitro was due to the change in the comonomer ratio, seen in the 1H-NMR analysis of the copolymer. The proportion of ε-caprolactone comonomer in the polymer decreased and the L-lactide increased.
Figure 6. Glass transition temperatures (Tg) (A) and melting enthalpies (ΔHf) (B) of the copolymer in the studied composites as a function of time in vitro. The composites comprised of poly(L-lactide-co-ε-caprolactone) (PLCL) and β-tricalcium phosphate (TCP) and rifampicin (R) with initial TCP contents of 0 wt%, 50 wt% and 60 wt%. Results shown as averages with standard deviations (n = 2–5).
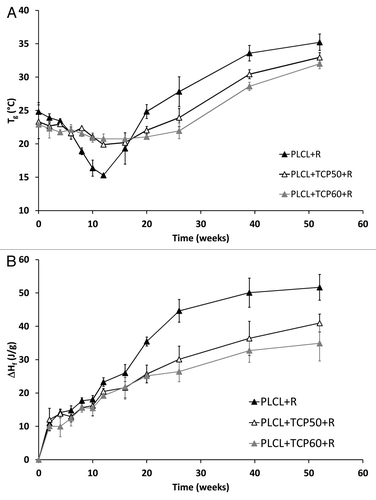
Melting temperatures and melting enthalpies were analyzed from the first heating of the samples because during the second heating, the melting peaks no longer appeared due to fast cooling in the DSC analysis. Most of the samples showed clear bimodality in the melting peaks the same way which was observed for ciprofloxacin containing composites.Citation1 Melting temperatures in general showed a clear increase up to the 20th week in the test series (data not shown). They increased from the initial value of 115°C to 120–126°C at 20 weeks. After that the melting temperatures started to decrease again and ended up at 106–109°C at 52 weeks.
Melting enthalpies, which describe the crystallinity of the polymer, were analyzed and corrected to correspond to the combined polymer and rifampicin part of the composites. Although absolute crystallinity values could not be calculated because the theoretical value of 100% crystalline poly(L-lactide-co-ε-caprolactone) 70/30 was not available, relative changes in the crystallinity were observed. There was a steady increase in the crystallinity of the composites throughout the test series (). This indicates an increase in crystallinity as the amorphous parts of the polymer degrade first. Furthermore, the fact that shorter polymer chains are more easily able to rearrange themselves into crystals has an effect on the increasing crystallinity.Citation37 The 1H NMR study showed that there were rather long L-lactide blocks in the copolymer and their proportion increased as the more amorphous random parts of the copolymer degraded. As the polymer chains were broken into shorter fragments, these blocks formed crystals when they were more able to rearrange themselves. Additionally, it was observed that the composite without β-TCP showed higher melting enthalpies than the composites with β-TCP, especially from the 20-week time point on. This observation suggests that the presence of β-TCP in the composites may have an inhibiting effect on the crystallization.
Microstructure
Some porosity was seen in the composites containing β-TCP throughout the degradation test series. The material without β-TCP did not show significant porosity. The differences between composites containing 50 wt% or 60 wt% of β-TCP were not notable. SEM micrographs showed pores of different sizes in the samples throughout the hydrolysis test series (). Pores up to the size of 100–200 μm were observed as well as smaller pores. Additionally, the surfaces of the pellet-shaped samples were porous. ().
Figure 7. SEM micrographs of the composites of poly(L-lactide-co-ε-caprolactone) (PLCL), 60 wt% of β-tricalcium phosphate (TCP) and rifampicin. (A–C) fractured surfaces after 0 weeks, 26 weeks and 52 weeks in vitro respectively. (D–F) outer surfaces after 0 weeks, 26 weeks and 52 weeks in vitro respectively.
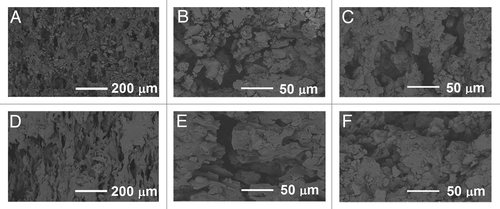
Rifampicin antibiotic, or ciprofloxacin antibiotic in the accompanying articleCitation1 were not observed to affect the porosity in any way.
Conclusion
The tested composites showed rifampicin release profile with four phases. The composite containing 50 wt% of β-TCP had the most promising steady release profile. The β-TCP was observed to accelerate the rifampicin release. The release from the composite without β-TCP showed a long lag phase that was probably due to the large size of the rifampicin molecule and low diffusivity of the drug in the polymer. As the polymer degradation proceeded and the permeability of the polymer increased, the rifampicin release also accelerated. The decrease of the molecular weights was rapid as was expected and the 1H NMR results showed a change in the comonomer ratio toward an increasing lactide comonomer content as degradation proceeded. Additionally, the polymer was observed to have a rather blocky structure, having long L-lactide blocks in the structure. The composite that contained 50 wt% of β-TCP showed activity in eradicating common osteomyelitis causing bacteria Pseudomonas aeruginosa which was seen as development of an inhibition zone in bacterial cultures utilizing bioluminescence.
Because rifampicin should not be used alone, a ciprofloxacin releasing composite material was developed in the accompanying studyCitation1 for use with the rifampicin-releasing composite material reported here. Both of the reported composite materials show potential to be developed into biodegradable, antibiotic releasing and osteoconductive bone-grafting materials that can be used together in the treatment of osteomyelitis and other bone related infections. In vitro tests are always needed to select the optimal composition of the materials for further studies and according to the results reported here, the choice is the composite with 50 wt% of β-TCP. However, preclinical followed by clinical studies are needed to establish the effect of these materials when they are implanted in living tissue both alone and together.
Materials and Methods
The processing and characterization methods, except UV-analysis, follow the same principles as in our article concerning ciprofloxacin releasing materials.Citation1
Materials
Medical grade poly(L-lactide-co-ε-caprolactone) (PLCL) with the comonomer ratio of 70/30 and Mw of 246,000 g/mol was purchased from Purac Biomaterials. β-tricalcium phosphate (β-TCP) (granule size < 38 μm) was purchased from Plasma Biotal Ltd. Rifampicin antibiotic (molecular structure shown in ) was purchased from Orion Pharma. Sörensen buffer solution was prepared according to the standard ISO 15814Citation38 and the chemicals used for the buffer solution (Na2HPO4 and KH2PO4) were purchased from J.T. Baker. The chemicals used for the bioluminescence bacterial cultures were: Gentamicin sulfate (Sigma-Aldrich), Isopropyl-β-D-thiogalaktopyranoside (IPTG) (Fermentas), Trypton (Lab M Limited), Yeast extract (Lab M Limited), Sodium Chloride (Merck), Agar (Merck).
Processing and sterilization
Dried polymer, β-TCP powder, and rifampicin (72 h in vacuum at room temperature) were processed into rod-shaped billets with a diameter of approximately 2.5 mm with a co-rotating custom-built intermeshing twin-screw extruder (L/D ratio = 22.5) in a nitrogen atmosphere. PLCL copolymer, β-TCP and rifampicin antibiotic were delivered to the process with separate gravimetric screw feeders. The mixing of the components took place in the extruder. A haul-off unit was used to guide the extrudate from the die and the diameter of the billets was fine-tuned adjusting the speed of the haul-off unit. Three different composites were processed. Each composite had 8 wt% rifampicin antibiotic in feed and different β-TCP contents (0, 50 and 60 wt%). These composites are denoted PLCL + R, PLCL + TCP50 + R and PLCL + TCP60 + R, respectively. Pellet-shaped samples (length approximately 2.5 mm) were cut from the billets. Before degradation tests were performed, the samples were packed and sterilized using gamma irradiation (minimum dose 25 kGy).
Drug release study
Weighed test samples (each test sample consisted of 15 pellets, approximately 300 mg in total) were placed in brown glass bottles along with 20 ml Sörensen buffer solution. Five parallel test samples were tested for each composite material. The bottles were placed in an incubator shaker at 37°C. At predetermined time intervals, the buffer solution was withdrawn from each of the bottles and replaced with fresh solution. The amount of buffer solution and the periodical change to fresh buffer solution enabled sink conditions to be valid throughout the test series. The amount of released rifampicin was determined from the buffer solution using a Unicam UV 500 spectrometer (ThermoSpectronic) at isosbestic point at wavelength of 226 nm. A wavelength area from 190 to 650 nm was scanned in order to detect possible changes in the rifampicin molecular structure which cause deviation in the UV-spectrum. Rifampicin is known to oxidize to rifampicin quinone in mildly alkaline aqueous solutions and in the presence of atmospheric oxygen. This can be seen as a change in the UV-spectrum of a rifampicin solution as well as a change in the color of the solution. Rifampicin quinone has a partly similar UV-spectrum to rifampicin and it also possesses antibacterial properties.Citation25 At a wavelength of 226 nm, an isosbestic point was found that could be used in the rifampicin content measurements. At this point, we can calculate the initial rifampicin content of the solution despite the fact that it has partly oxidized to rifampicin quinone, because the absorbance of these compounds is the same at this wavelength. The fact that rifampicin quinone degrades further to other compounds without UV-absorbance, may affect the accuracy of this method. The accuracy is at its best if the measurements are made at short intervals, not letting the dissolution medium stay unchanged for long periods.
Inhibition zone testing using bioluminescence imaging
The effect of the antibiotic releasing composite materials against one of the common osteomyelitis causing bacteria Pseudomonas aeruginosa, which was used as a non-pathogenic model organism, was tested using bioluminescence imaging based on the ability of genetically engineered bacteria to emit light. The antibiotic containing composite material chosen for this study was the composite containing 50 wt% of β-TCP (PLCL + TCP50 + R) based on the most promising drug release results. Composite of PLCL and 50 wt% β-TCP without antibiotics was used as control.
An engineered bacteria strain of Pseudomonas aeruginosa PAO-LAC carrying plasmid pUCP24GWCitation39 was used as the biosensor. The bacteria were cultured on antibiotic plates overnight at 30°C and 300 rpm (1 mM IPTG, 10 µg/ml gentamycin), and suitable colonies were moved into liquid culturing in LB (5 g/l yeast extract, 10 g/l tryptone and 5 g/l NaCl). If the bacteria did not produce luminescence as wished in the morning, 1/50 dilution was made in a culture tube and it was incubated at 37°C and 300 rpm for three hours. The level of luminescence of the cultures was measured by using Plate ChameleonTM multilabel counter 1.001 (Hidex Ltd.), and at volume of 200 µl. Counts of 1.1–2.3 × 106 were found satisfying.
Layers of LB-agar (agar 15 g/l, 2 ml) were cast into 6-well plate, and the controls and antibiotic containing composite pellets were placed on top of them (each pellet into its own well). Bacteria were mixed with soft LB-agar (7.5 g/l) solution and cast on top of the first layers. The amount of bacterial culture was dependent on the luminescence level. It was 350–500 μl/well. After solidification, the plate was taken to the imaging station of Xenogen VivoVision IVIS® Lumina luminescence camera (Caliper LifeSciences). Pictures were taken every 20 min for 16 h with exposure time of 30 sec. The pictures were analyzed using Living Image® 3.1 program (Caliper LifeSciences).
In vitro degradation study
Degradation tests were conducted at 37°C in vitro following the standard ISO 15814.Citation38 First, weighed test samples (each test sample consisted of 15 pellets) were placed in brown glass bottles along with 20 ml Sörensen buffer solution. Five parallel test samples of each composite material were tested at each time point. The bottles were placed in an incubator shaker at 37°C. The pH of the buffer solution was measured periodically with a calibrated pH meter and the buffer solutions were changed every two weeks at the beginning of the test series and once a week as the degradation accelerated. Test samples were withdrawn at predetermined time points of 2, 4, 6, 8, 10, 12, 16, 20, 26, 30 and 52 weeks.
Methods of analysis
Residual monomer
The determination of residual L-lactide and ε-caprolactone monomer contents before and after processing was performed by Ramboll Analytics Oy. The ε-caprolactone and L-lactide contents were measured after chloroform extraction of the samples using gas chromatography (DC8000, CE Instruments) and after chloroform dilution using an FI-detector. The measuring resolution was 0.02%.
Initial drug content measurements
Samples of about 150 mg were weighed from each manufactured composition and dissolved in 50 ml of chloroform (J.T. Baker). The amount of rifampicin in the chloroform solution was determined using a Unicam UV 540 Spectrophotometer (ThermoSpectronic) at a maximum absorption wavelength of 349 nm. The rifampicin concentration in the solution was calculated using Beer-Lambert law and standard curves prepared with known concentrations of rifampicin.
Mass loss, water absorption and pH
After the test samples were withdrawn from the incubator shaker, they were rinsed twice with distilled water and the surfaces were carefully wiped with tissue paper. The test samples were weighed immediately after wiping. The weighed test samples were dried for at least three days at ambient conditions and for one week in vacuum. Finally, the test samples were reweighed to obtain the dry masses. The dried test samples were stored in a desiccator for further analysis.
The mass loss was calculated as the difference between the initial mass of the test sample and the mass of the dried test sample divided by the initial mass of the test sample. The water absorption was calculated as the difference between the mass of the wet test sample and the mass of the dried test sample divided by the mass of the dried test sample.
Molecular weights
The molecular weights (number average, Mn, and weight average, Mw, molecular weights) and polydispersity (PD) of the copolymer were determined at room temperature by size exclusion chromatography (SEC) (Waters Associates system equipped with a Waters 717plus autosampler, a Waters 510 HPLC solvent pump, four linear PL gel columns (104, 105, 103 and 100 Å) connected in series, and a Waters 2414 differential refractometer). Chloroform (Riedel-de Haën Ag, stabilized with 1% ethanol) was used as a solvent and eluent. The samples were filtered through a 0.5 μm Millex SR (Millipore) filter. The injected volume was 200 μl and the flow rate was 1.0 ml/min. Monodisperse polystyrene standards were used for primary calibration.
Thermal properties
Thermal analysis was performed using differential scanning calorimeter DSC Q1000 (TA Instruments). Nitrogen was used as the sweeping gas. To ensure the samples (4–6 mg) had a similar thermal history, they were heated twice and cooled rapidly in between. The heating rate was 20°C/min, the cooling rate was 50°C/min and the temperature range was -60°C to +200°C. Glass transition temperatures (Tg) were obtained from the second heating and melting temperatures and enthalpies (Tm and ΔHf respectively) were obtained from the first heating cycle. Two to five parallel samples were tested for each composite material and time point. The results were analyzed using Universal Analysis Software and averages and standard deviations were calculated.
Ceramic content
The β-TCP content of the test samples was measured using thermogravimetric analysis (TA Instruments). Approximately 20 mg of a sample was used and the samples were heated at a rate of 20°C/min up to 700°C. The results were analyzed using Universal Analysis Software. Five parallel samples of each composition at each time point were analyzed and the results were calculated as averages and standard deviations.
Molecular structure
The proton spectra of the samples were measured using Varian Mercury 300 MHz NMR Spectrometer (Varian Associates Inc.) at room temperature. Tetramethylsilane (TMS) was used as an internal standard, and chemical shifts were measured relative to TMS. The 1H NMR spectra were measured in standard 5 mm tubes in deuterochloroform. The data were acquired until the quality of the spectrum was sufficient and the number of scans was about 512. The proton NMR spectra of the samples were processed and analyzed using SpinWorks 3.1 and ACD/Spectrus software. Phase correction and baseline correction were applied to all spectra. Information on the molecular composition of rifampicin and the comonomer ratio of the copolymer was obtained.
Microstructure of the samples
The microstructure of the composites was observed using scanning electron microscopy (Philips XL-30 SEM equipped with a LaB6 filament, Philips) with an acceleration voltage of 12.0 kV. The micrographs were taken both on the surface of the samples and on fractured samples that were coated with gold (Edwards S150 Sputter Coater) prior to microstructure examination.
Acknowledgments
Research collaboration with Bioretec Ltd. and financial support from the Finnish Funding Agency for Technology and Innovation (TEKES) and National Graduate School of Musculoskeletal Disorders and Biomaterials (N.A. and M.V.) are gratefully appreciated. MSc Kalle Räsänen, Mrs Raija Reinikainen, Mrs Kaija Honkavaara, Ms Eija Ahonen and MSc Vuokko Heino are warmly thanked for technical assistance. Mr Peter Heath is thanked for checking the language of the manuscript.
Disclosure of Potential Conflicts of Interest
No potential conflicts of interest were disclosed.
Supplemental Materials
Supplemental materials may be found here: www.landesbioscience.com/journals/biomatter/article/22793
References
- Ahola N, Männistö N, Veiranto M, Karp M, Rich J, Efimov A, et al. An in vitro study of composites of poly(L-lactide-co-epsilon-caprolactone), β-tricalcium phosphate, and ciprofloxacin intended for local treatment of osteomyelitis. Biomatter 2012; In press
- Ginebra MP, Traykova T, Planell JA. Calcium phosphate cements as bone drug delivery systems: a review. J Control Release 2006; 113:102 - 10; http://dx.doi.org/10.1016/j.jconrel.2006.04.007; PMID: 16740332
- Chihara S, Segreti J. Osteomyelitis. Dis Mon 2010; 56:6 - 31; http://dx.doi.org/10.1016/j.disamonth.2009.07.001
- Parsons B, Strauss E. Surgical management of chronic osteomyelitis. Am J Surg 2004; 188:Suppl 57 - 66; http://dx.doi.org/10.1016/S0002-9610(03)00292-7; PMID: 15223504
- Zilberman M, Elsner JJ. Antibiotic-eluting medical devices for various applications. J Control Release 2008; 130:202 - 15; http://dx.doi.org/10.1016/j.jconrel.2008.05.020; PMID: 18687500
- Arruebo M, Vilaboa N, Santamaria J. Drug delivery from internally implanted biomedical devices used in traumatology and in orthopedic surgery. Expert Opin Drug Deliv 2010; 7:589 - 603; http://dx.doi.org/10.1517/17425241003671544; PMID: 20230306
- Perlroth J, Kuo M, Tan J, Bayer AS, Miller LG. Adjunctive use of rifampin for the treatment of Staphylococcus aureus infections: a systematic review of the literature. Arch Intern Med 2008; 168:805 - 19; http://dx.doi.org/10.1001/archinte.168.8.805; PMID: 18443255
- Zimmerli W, Widmer AF, Blatter M, Frei R, Ochsner PE, Foreign-Body Infection (FBI) Study Group. Role of rifampin for treatment of orthopedic implant-related staphylococcal infections: a randomized controlled trial. JAMA 1998; 279:1537 - 41; http://dx.doi.org/10.1001/jama.279.19.1537; PMID: 9605897
- Khanlari B, Elzi L, Estermann L, Weisser M, Brett W, Grapow M, et al. A rifampicin-containing antibiotic treatment improves outcome of staphylococcal deep sternal wound infections. J Antimicrob Chemother 2010; 65:1799 - 806; http://dx.doi.org/10.1093/jac/dkq182; PMID: 20542908
- Bliziotis IA, Ntziora F, Lawrence KR, Falagas ME. Rifampin as adjuvant treatment of Gram-positive bacterial infections: a systematic review of comparative clinical trials. Eur J Clin Microbiol Infect Dis 2007; 26:849 - 56; http://dx.doi.org/10.1007/s10096-007-0378-1; PMID: 17712583
- Mäkinen TJ, Veiranto M, Lankinen P, Moritz N, Jalava J, Törmälä P, et al. In vitro and in vivo release of ciprofloxacin from osteoconductive bone defect filler. J Antimicrob Chemother 2005; 56:1063 - 8; http://dx.doi.org/10.1093/jac/dki366; PMID: 16234335
- Alvarez H, Castro C, Moujir L, Perera A, Delgado A, Soriano I, et al. Efficacy of ciprofloxacin implants in treating experimental osteomyelitis. J Biomed Mater Res B Appl Biomater 2008; 85:93 - 104; http://dx.doi.org/10.1002/jbm.b.30921; PMID: 17696153
- Mäkinen TJ, Veiranto M, Knuuti J, Jalava J, Törmälä P, Aro HT. Efficacy of bioabsorbable antibiotic containing bone screw in the prevention of biomaterial-related infection due to Staphylococcus aureus. Bone 2005; 36:292 - 9; http://dx.doi.org/10.1016/j.bone.2004.11.009; PMID: 15780955
- Miyai T, Ito A, Tamazawa G, Matsuno T, Sogo Y, Nakamura C, et al. Antibiotic-loaded poly-ε-caprolactone and porous β-tricalcium phosphate composite for treating osteomyelitis. Biomaterials 2008; 29:350 - 8; http://dx.doi.org/10.1016/j.biomaterials.2007.09.040; PMID: 17977596
- Tiainen J, Knuutila K, Veiranto M, Suokas E, Törmälä P, Kaarela O, et al. Pull-out strength of multifunctional bioabsorbable ciprofloxacin-releasing polylactide-polyglycolide 80/20 tacks: an experimental study allograft cranial bone. J Craniofac Surg 2009; 20:58 - 61; http://dx.doi.org/10.1097/SCS.0b013e318190df48; PMID: 19164990
- Garvin KL, Miyano JA, Robinson D, Giger D, Novak J, Radio S. Polylactide/polyglycolide antibiotic implants in the treatment of osteomyelitis. A canine model. J Bone Joint Surg Am 1994; 76:1500 - 6; PMID: 7929497
- Waknis V, Jonnalagadda S. Novel poly-DL-lactide-polycaprolactone copolymer based flexible drug delivery system for sustained release of ciprofloxacin. Drug Deliv 2011; 18:236 - 45; http://dx.doi.org/10.3109/10717544.2010.528070; PMID: 21189060
- Castro C, Évora C, Baro M, Soriano I, Sánchez E. Two-month ciprofloxacin implants for multibacterial bone infections. Eur J Pharm Biopharm 2005; 60:401 - 6; http://dx.doi.org/10.1016/j.ejpb.2005.02.005; PMID: 15996581
- Castro C, Sánchez E, Delgado A, Soriano I, Núñez P, Baro M, et al. Ciprofloxacin implants for bone infection. In vitro-in vivo characterization. J Control Release 2003; 93:341 - 54; http://dx.doi.org/10.1016/j.jconrel.2003.09.004; PMID: 14644584
- Koort JK, Mäkinen TJ, Suokas E, Veiranto M, Jalava J, Knuuti J, et al. Efficacy of ciprofloxacin-releasing bioabsorbable osteoconductive bone defect filler for treatment of experimental osteomyelitis due to Staphylococcus aureus. Antimicrob Agents Chemother 2005; 49:1502 - 8; http://dx.doi.org/10.1128/AAC.49.4.1502-1508.2005; PMID: 15793132
- Koort JK, Suokas E, Veiranto M, Mäkinen TJ, Jalava J, Törmälä P, et al. In vitro and in vivo testing of bioabsorbable antibiotic containing bone filler for osteomyelitis treatment. J Biomed Mater Res A 2006; 78:532 - 40; http://dx.doi.org/10.1002/jbm.a.30766; PMID: 16736479
- Koort JK, Makinen TJ, Suokas E, Veiranto M, Jalava J, Tormala P, et al. Sustained release of ciprofloxacin from an osteoconductive poly(DL)-lactide implant. Acta Orthop 2008; 79:295 - 301; http://dx.doi.org/10.1080/17453670710015111; PMID: 18484258
- Daculsi G, Goyenvalle E, Cognet R, Aguado E, Suokas EO. Osteoconductive properties of poly(96L/4D-lactide)/beta-tricalcium phosphate in long term animal model. Biomaterials 2011; 32:3166 - 77; http://dx.doi.org/10.1016/j.biomaterials.2011.01.033; PMID: 21315446
- Paakinaho K, Ellä V, Syrjälä S, Kellomäki M. Melt spinning of poly(l/d)lactide 96/4: Effects of molecular weight and melt processing on hydrolytic degradation. Polym Degrad Stabil 2009; 94:438 - 42; http://dx.doi.org/10.1016/j.polymdegradstab.2008.11.010
- Bain DF, Munday DL, Cox PJ. Evaluation of biodegradable rifampicin-bearing microsphere formulations using a stability-indicating high-performance liquid chromatographic assay. Eur J Pharm Sci 1998; 7:57 - 65; http://dx.doi.org/10.1016/S0928-0987(98)00005-0; PMID: 9845778
- Jindal KC, Chaudhary RS, Singla AK, Gangwal SS, Khanna S. Effects of buffers and pH on rifampicin stability. Pharm Ind 1995; 57:420 - 2
- Le Guellec C, Gaudet ML, Lamanetre S, Breteau M. Stability of rifampin in plasma: consequences for therapeutic monitoring and pharmacokinetic studies. Ther Drug Monit 1997; 19:669 - 74; http://dx.doi.org/10.1097/00007691-199712000-00011; PMID: 9421109
- Baker R. Controlled Release of Biologically Active Agents. New York: John Wiley & Sons, 1987.
- Ritger PL, Peppas NA. A simple equation for desciption of solute release I. fickian and non-fickian release from non-swellable devices in the form of slabs, spheres, cylinders or discs. J Control Release 1987; 5:23 - 36; http://dx.doi.org/10.1016/0168-3659(87)90034-4
- Jones DS, McCoy CP, Andrews GP. Physicochemical and drug diffusion analysis of rifampicin containing polyethylene glycol-poly(ε-caprolactone) networks designed for medical device applications. Chem Eng J 2011; 172:1088 - 95; http://dx.doi.org/10.1016/j.cej.2011.05.024
- Jeong SI, Kim BS, Lee YM, Ihn KJ, Kim SH, Kim YH. Morphology of elastic poly(L-lactide-co-ε-caprolactone) copolymers and in vitro and in vivo degradation behavior of their scaffolds. Biomacromolecules 2004; 5:1303 - 9; http://dx.doi.org/10.1021/bm049921i; PMID: 15244444
- Herbert IR. Statistical analysis of copolymer sequence distribution. In: Ibbet RN, ed. NMR Spectroscopy of Polymers. London: Blackie Academic & Professional, 1993:50-79.
- Ferńndez J, Etxeberria A, Sarasua JR. Synthesis, structure and properties of poly(L-lactide-co-ε-caprolactone) statistical copolymers. J Mech Behav Biomed Mater 2012; 9:100 - 12; http://dx.doi.org/10.1016/j.jmbbm.2012.01.003; PMID: 22498288
- Cellai L, Cerrini S, Segre A, Brufani M, Fedeli W, Vaciago A. Comparative study of the conformations of rifamycins in solution and in the solid state by proton nuclear magnetic resonance and X-rays. J Org Chem 1982; 47:2652 - 61; http://dx.doi.org/10.1021/jo00134a028
- Ragaert K, Dekeyser A, Cardon L, Degrieck J. Quantification of thermal material degradation during the processing of biomedical thermoplastics. J Appl Polym Sci 2011; 120:2872 - 80; http://dx.doi.org/10.1002/app.33323
- Li S, Garreau H, Vert M. Structure-property relationships in the case of the degradation of massive poly(α-hydroxy acids) in aqueous media - part 3 influence of the morphology of poly(l-lactic acid). J Mater Sci Mater Med 1990; 1:198 - 206; http://dx.doi.org/10.1007/BF00701077
- Pitt CG, Chasalow FI, Hibionada YM, Klimas DM, Schindler A. Aliphatic Polyesters - 1. the Degradation of Poly(epsilon-caprolactone) in vivo. J Appl Polym Sci 1981; 26:3779 - 87; http://dx.doi.org/10.1002/app.1981.070261124
- ISO 15814. Implants for surgery – copolymers and blends based in polylactide – in vitro degradation testing.
- Moir DT, Ming Di, Opperman T, Schweizer HP, Bowlin TL. A high-throughput, homogeneous, bioluminescent assay for Pseudomonas aeruginosa gyrase inhibitors and other DNA-damaging agents. J Biomol Screen 2007; 12:855 - 64; http://dx.doi.org/10.1177/1087057107304729; PMID: 17644773