Abstract
In this article, I discuss the hallmarks of hypoxia in vitro and in vivo and review work showing that many types of stem cell proliferate more robustly in lowered oxygen. I then discuss recent studies showing that alterations in the levels and the types of cell and substrate adhesion molecules are a notable response to reduced O2 levels in both cultured primary neural stem cells and brain tissues in response to hypoxia in vivo. The ability of O2 levels to regulate adhesion molecule expression is linked to the Wnt signaling pathway, which can control and be controlled by adhesion events. The ability of O2 levels to influence cell adhesion also has far-reaching implications for development, ischemic trauma and neural regeneration, as well as for cancer and other diseases. Finally I discuss the possibility that the fluctuations in O2 levels known to have occurred over evolutionary time could, by influencing adhesion systems, have contributed to early symbiotic events in unicellular organisms and to the emergence of multicellularity. It is not my intention to be exhaustive in these domains, which are far from my own field of study. Rather this article is meant to provoke and stimulate thinking about molecular evolution involving O2 sensing and signaling during eras of geologic and atmospheric change that might inform modern studies on development and disease.
Elevation in Levels of the Hypoxia Inducible Factor, HIF1α, is a Hallmark of Reduced Oxygen Availability in Cell Culture and in Tissues
HIF1α is a constitutively produced protein that is normally hydroxylated by a family of prolyl hydroxylase enzymes, a modification that allows HIF to bind the von Hippel-Lindau tumor suppressor protein, targeting HIF for proteasomal degradation.Citation1-Citation4 When O2 levels are lowered, hydroxylation is reduced and degradation is prevented. The stabilized HIFα can then translocate into the nucleus and directly transactivate a number of genes to respond to hypoxia, among which are those involved in glycolysis, erythropoiesis, and angiogenesis.Citation4-Citation7 HIFs not only have direct transcriptional targets but also influence signaling systems to alter gene expression.Citation1 It is notable that HIF1α levels have been shown to be activated over a wide range of physiological O2 tensions, although the greatest increases were at levels consistent with hypoxia/ischemia in vivo.Citation8 These findings indicate that levels of HIF are functionally involved in O2 homeostasis in cells and organisms to enable the switch from aerobic to anaerobic metabolism when O2 levels are limiting. It is of interest that mice lacking HIF1α or its homolog HIF2α and their binding partner HIF1β all exhibit embryonic lethality, displaying multiple morphogenetic defects, including failure of neural tube closure.Citation9 The importance of O2 concentration on the formation of the neural folds and neural crest development was recognized in early studies of rat neural maturation in cultured embryos.Citation10
Hypoxia vs. In Situ Normoxia
The Merriam-Webster Dictionary defines hypoxia as “a deficiency of oxygen reaching the tissues of the body.” In the scientific literature, however, the word is often used to mean a level of O2 lower than that present in air, particularly in studies in vitro. Air does not reflect the in vivo environment of the majority of cell types and hence the term “in situ normoxia” has been suggested.Citation11 This novel term is meant to give a better indication what normoxia or hypoxia would be for a given cell type based on its exposure to O2 in vivo and allow cell culture to mimic more accurately the physiological state, as is beginning to be understood for neural cells.Citation12,Citation13 It also points to the importance of measurements of the levels of O2 in particular tissues in vivo. In warm-blooded organisms in vivo, such levels are critically dependent on multiple factors including vascularization and angiogenesis, levels of red blood cells and hemoglobin, cardiac capacity, as well as external oxygenation. These considerations are also critical for the interpretation of studies of “hypoxia” in cell lines that were derived in hyperoxic conditions, but later exposed to lower O2 levels.
In this regard, it is critical to emphasize two concepts that provide an outline for the sections to follow: (1) few tissues experience atmospheric levels of O2 in vivo and (2) metazoan life originated in an atmosphere considerably lower in levels of O2 than the modern atmosphere.
Cell Culture Conditions and O2 Levels
For over a decade it has been recognized that proliferation is enhanced and differentiation is altered in a number of stem cell types when cells are cultured in levels of O2 (1–8% O2) lower than those in air, previously the standard for in vitro studies (reviewed in refs. Citation12 and Citation13). Cells from the nervous system cultured under such low O2 conditions include neural progenitor cells from various brain regions,Citation14-Citation28 virally-immortalized human neural progenitor cells,Citation29 and neural crest stem cells.Citation24 Other sensitive cells are iPS cells,Citation30 embryonic stem cellsCitation22,Citation27,Citation31 and stem cells of different tissues.Citation32 These observations are consistent with what is known about O2 levels in the stem cell niches of the brain and other tissuesCitation12,Citation13,Citation16,Citation32-Citation37 and are likely to reflect more closely the native behavior of cells under physiological conditions.
A recent well-received book describes the origins of the now ubiquitous human HeLa cell line.Citation38 The author reviews the early history of cell culture, noting that the recovery of stable human cell lines was rare, an observation accounting for the extensive use of the surviving line HeLa. Indeed it is possible that many early cell cultures failed because they were grown in air, a hyperoxic environment compared with their in vivo environment. This consideration highlights the importance of taking in vivo physiological O2 tension into account when designing in vitro culture conditions.
Changes in the Expression of Multiple Types of Adhesion Molecules Accompany the Growth of Neural Stem Cells in Reduced O2 Atmospheres In Vitro
Cell adhesion between and among cells is a critical component of morphoregulation during development and in disease.Citation39,Citation40 Molecules of the immunoglobulin and cadherin families of cell adhesion molecules (CAMs) link cells together and collectively play major roles in the formation of tissues and organs. In addition, molecules in the extracellular matrix (ECM) surrounding cells, along with integrins and other ECM receptors on the cell surface, are critical for cell migration.
Several recent studies have linked the altered expression of cell and extracellular matrix adhesion molecules and matrix modifying enzymes to growth of neural stem cells in culture in a reduced O2 environment.Citation23,Citation41 Moreover, studies from my laboratory found that the levels of mRNAs for several CAMs, ECM proteins, and ECM modifying enzymes differed in rat embryonic neural stem cells cultured for 72 h in 1% vs. 20% O2.Citation23 Another study evaluated 5704 genes in rat neural stem cells and found that, of all the genes whose expression differed between cells cultured at 3% or 20% O2 for 24 h, a significant fraction were involved in cell adhesion or migration.Citation41
Adhesion molecule expression in vitro was also altered in several other cell types exposed to reduced O2 levels, including mesenchymal stem cells,Citation42-Citation44 hematopoietic stem cellsCitation45 and a melanoma cell line.Citation44
Connection between Oxygen Levels, HIF1α, Wnt Signaling and Adhesion Molecule Expression Suggests One Mechanism to Control Neural Stem Cell Proliferation
A significant finding in two recent papers is the connection between HIF1α stabilization and the activation of the Wnt pathway by lowered O2.Citation22,Citation23 As reported in many systems, activation of HIF-1α occurred in rat embryonic neural stem cells cultured in lowered O2.Citation22,Citation23 As described above, stabilization of the levels of the HIF1α protein is a hallmark of hypoxia,Citation2,Citation12,Citation13,Citation46,Citation47 but HIF1α levels are also known to vary significantly under different physiological levels of O2.Citation8
Our studyCitation23 also showed that a critical downstream target of Wnt was an increased expression of matrix metalloproteinase 9 (MMP9), an ECM modifying enzyme. MMP9 expression had previously been shown to be upregulated by Wnt activation in human lymphocytes.Citation48 More importantly, we showed that the increase in the level and activity of MMP9 was essential for the increased proliferation and migration of embryonic rat neural stem cells at reduced levels of O2.Citation23 Hence modifications to the ECM can control neural stem cell proliferation in vitro and may do so in vivo in the stem cell niche. Evaluating the proliferation and migration of neural stem cells in the MMP9 knockout mouse will be revealing and an assessment of the critical enzyme targets of MMP9 should be facilitated using this model.
Wnt is a critical regulator of cell adhesion in multiple cell types via its ability to alter β-catenin activity, the so-called canonical Wnt signaling pathway.Citation49-Citation51 Canonical Wnt signaling primarily influences cadherin-mediated adhesion, subcellular localization, and effects on cell junctions.Citation52-Citation56 The non-canonical, planar cell polarity Wnt pathwayCitation57 involves cell rearrangements and migration during development, particularly those in the nervous system.Citation58 These pathways involve extensive changes in cell adhesion and in the cytoskeleton.Citation57
Another mechanism by which O2 levels could affect adhesion systems is via the engagement of microRNAs, many of which target various adhesion molecule mRNAs and are known to change dramatically under hypoxic conditions.Citation59-Citation65 Thus O2 influences Wnt activity via HIF1α activation, and Wnt in turn can influence multiple adhesive systems directly or indirectly. Such adhesive interactions are clearly a substrate for the evolution of multicellular organisms over geological time.Citation57,Citation66,Citation67
Hypoxia in Early Embryonic Development
Studies of mammalian placental development were among the first to recognize the importance of O2 tension for proper development, both of the early placenta and later of the first trimester human embryo, prior to the complete establishment of maternal-fetal circulation.Citation68-Citation70 The hypoxic environment in utero has been postulated to play a key role in trophoblast proliferation and invasion, in both in vivo and in vitro studies.Citation68,Citation70 In this system, low O2 promotes the expression of several extracellular matrix adhesion molecules, such as those of the β1 integrin family as well as matrix modifying enzymes such as uPA and MMP-2, which play essential roles in trophoblast invasion (reviewed in ref. Citation68) This process is an essential initial mechanism for formation of the placenta, and aberrations in these early events can have serious consequences for placental function resulting in adverse pregnancy outcomes.Citation69,Citation70
Influence of Hypoxia-Ischemia on Adhesion Molecule Expression in the Fetal and Adult Brain
Hypoxia in utero is a key factor contributing to impaired neural development. In a mouse model of fetal hypoxia, neuronal migration was increased in the CA1 and dentate gyrus regions of the hippocampus along with biphasic changes in molecules that influence migration, including reelin, disabled and APP.Citation71 Using the hypoxia indicator pimonidazole, it was revealed that hypoxic areas colocalized with HIF1α expression and with the adhesion molecule PE-CAM, a marker of endothelial cells, indicating that hypoxia promotes the proliferation and migration of these cells to stimulate angiogenesis during brain development.Citation72
Studies of cerebral hypoxia in adult animals induced either by middle cerebral artery occlusion (stroke model) or by hypobaric O2 exposure (chronic hypoxia) demonstrated changes in multiple adhesion systems. For example, in microvessel-rich regions isolated from stroke-affected human brains by laser-capture microdissection, proangiogenic adhesion molecules were upregulated, including NR-CAM, MMP-2, TIMP-1 and β-catenin.Citation73 In a mouse stroke model, V-CAM, laminin, dystroglycan and α6 integrin were upregulated and the number of proliferative endothelial cells were increased suggesting that these molecules contribute to angiogenesis in response to cerebral ischemia.Citation74 In chronic cerebral ischemia, fibronectin was increased as was the expression of integrin α5β1 on endothelial cells.Citation75 Subsequently, changes in astrocytes occurred, including increases in α6β4 integrin and dystroclycan, presumably contributing to the cell interactions necessary for the formation of astrocytic end feet to support the restoration of a mature brain endothelium.Citation75 It is known that cerebral edema occurs after a hypoxic incident due to a compromised blood-brain barrier and the resultant increase in cerebrovascular permeability. In an in vitro model of the blood-brain barrier subjected to hypoxia, levels of expression of the E-cadherin adhesion molecule were decreased; this molecule is known to be important for blood-brain barrier function.Citation76
Adhesion Molecule Expression in Neural Stem Cells Favors Their Incorporation into Regenerating Tissues after Various Lesions to the CNS
Early studies revealed that the polysialylated form of N-CAM (PSA-N-CAM) was prominently expressed in neural stem cells migrating in the rostral migratory stream from the SVZ to the olfactory bulb.Citation77 Subsequent studies showed that loss of PSA or N-CAM decreased the normal migration of these cellsCitation78,Citation79 and allowed their increased migration into demyelinating lesionsCitation80,Citation81 or facilitated myelination when transplanted into lesioned spinal cord.Citation82 A recent study indicates that increased expression of reelin helps to facilitate migration of cells from the rostral migratory stream into demyelinating lesions in the corpus callosum.Citation83
Other studies have indicated that overexpression of the L1 neural cell adhesion molecule in mouse embryonic stem (ES) cells supported the regrowth of corticospinal axons and promoted functional recovery after spinal cord compression injury.Citation84,Citation85 The same L1-ES cells promoted recovery from excitotoxic lesions to the mouse striatum.Citation86 Expression of the extracellular matrix proteins thrombospondin 1 and 2 were correlated with a positive recovery of neuronal function after transient ischemia.Citation87
An interesting study relevant to the use of stem cells to promote recovery after stroke used cells selected for adhesion molecule expression.Citation88 Mouse neural stem cells were selected for expression of the integrin CD49d, which is a receptor for V-CAM in endothelial cells. These selected cells showed enhanced homing toward a hypoxic lesion and enhanced behavioral recovery in a mouse stroke model. The authors postulate that these effects are due to better transmigration through the vascular endothelium.
All of the foregoing perturbations to stem cells and their environments should be of great value for studies aimed at promoting regeneration by mimicking the mechanical forces that bias stem cell fate.Citation89 Alterations of adhesion molecule expression in the endothelial environment, in addition to influencing tumor vascularization and O2 delivery, can serve to generate signals for neighboring neural stem cells to proliferate and differentiate.Citation90,Citation91
Hypoxia and the Adhesive Glioma Cell Microenvironment
Glioma cells bear many striking resemblances to neural stem cells.Citation92-Citation95 These tumors are thought to arise when neural stem cells at specific stages of development either undergo mutation or respond to environmental signals and become immortalized. Like all solid tumors, gliomas show large areas of hypoxia and it has been suggested that hypoxia favors the proliferation and stabilization of cancer stem cells, which can maintain tumor propagationCitation13,Citation85,Citation93,Citation94,Citation96,Citation97 In glioma cells, the effects of “hypoxia,” or low O2 in situ, are similar to that of a low O2 niche in normal neural stem cells.Citation12,Citation13
HIFs are commonly expressed in gliomas and HIF1 blockade inhibited migration and invasion of malignant glioma.Citation92 At least in part of the decreased migration was due to the downregulation of MMPs 2 and 9. MMP-13 is expressed specifically in glioma stem cells and its inhibition also results in decreased glioma stem cell migration and invasion.Citation98 It has also been postulated that hypoxia increases the strength of cell-cell adhesion in glioma cells in culture.Citation99
Glioma stem cells have been shown to express the L1-CAM, which is implicated in increased invasiveness and metastasis.Citation98,Citation100 Expression of this adhesion molecule is correlated with poor outcome in patients.Citation100 In vitro, decreasing the levels of L1 inhibited cell growth and neurosphere formation and decreased apoptosis.Citation98 The Wnt signaling pathway, many downstream targets of which are adhesion molecules,Citation53-Citation55 has recently been shown to influence glioma tumorigenesis.Citation101
It is well established that the turnover of cell and matrix adhesions is essential to allow for epithelial mesenchymal transformation and support tumor migration and invasion into surrounding tissues.Citation85,Citation93,Citation97,Citation102 Targeting of these molecules has provided several avenues for developmental therapeutics,Citation93,Citation95,Citation97,Citation98 although the similarities between glioma cells and neural stem cells present difficulties in identifying targeted therapeutics that do not affect endogenous normal cells.Citation93,Citation95,Citation97,Citation98
HIFs and Hypoxia at the Organismal Level
Genetic variations in HIFs have been seen in human and animal populations dwelling at high altitudes,Citation103-Citation108 in Drosophila that have been adapted to low O2 levelsCitation109,Citation110 and in blind mole rats that live in hypoxic underground burrows.Citation111,Citation112 Thus modern organisms have adapted and are capable of adapting to alterations in the O2 environment via HIF1 changes and alterations in genes involved in aerobic metabolism. It is not surprising that HIF mutations and HIF dysregulation are associated with multiple diseasese.Citation171 As outlined in the final sections, it is tempting to speculate that cell adhesion events influenced by increasing O2 levels in the early earth atmosphere provided the critical control of cell and/or substrate adhesion that favored the emergence of multicellularity during evolutionary time.
Organismal Evolution Occurred at Vastly Lower O2 Levels than Those in the Present Atmosphere
Biologists think about molecular, cellular and organismal evolution, but we seldom consider them in light of geologic and atmospheric change. Integration of this key element in the evolution of life on earth could encourage new ways of thinking about molecular and organismal evolution. Many of us were not trained in earth and atmospheric sciences or even cognizant that such issues might play a key role in the development of life on earth, notwithstanding perhaps metabolic biochemists, who necessarily think about aerobic and anaerobic cellular energy production (e.g., refs. Citation5, Citation113 and Citation114). Indeed this point serves as a reminder that mitochondria, the key players in aerobic metabolism, are central to many aspects of development and evolution (see below). The evolution of metabolic processesCitation114,Citation115 played a critical role and continues to do so, especially in high energy-requiring tissues such as the nervous, cardiac, and muscular systems.
When unicellular organisms first appeared on earth, more than three billion years ago,Citation116 the Earth's atmosphere and oceans were anoxic (see ).Citation117 Around 2.5–3 billion years ago, photosynthesizing cyanobacteria evolved and began producing O2, which initially was absorbed by minerals but, exhausting this sink, eventually accumulated in the atmosphere, resulting in the Great Oxygenation Event.Citation117 This event is believed to have caused the extinction of a large number of organisms that were poisoned by toxic O2 levels.Citation116 Eukaryotes and archaebacteria appeared about two billion years ago during the Neoproterozoic period as O2 levels were rising, albeit still considerably lower than modern levels.Citation117,Citation118 Multicellular life probably arose 1–1.5 billion years ago and bilaterian animals were prevalent 500 million years ago just before the Cambrian explosion.Citation116,Citation119
These topics are complex and have been given short shrift by my exclusive focus on O2 levels. For example, a recent study indicated that as late as the Devonian era, O2 levels were lower than the present and impacted the emergence of larger vertebrates with high metabolic rates, e.g., predatory fish.Citation119 Ocean oxygenation appears to have acted (and appears to be doing so still) as an “evolutionary barrier” to the increasing size of predatory fish.Citation119,Citation120 In a similar vein, a recent paper postulates that variations in O2 levels acted to select among arthropods during the Cambrian ages, with the most successful species being those that were tolerant of, and had the ability to respond adaptively to, changes in O2 levels in seawater.Citation121 Consideration of O2 levels as drivers of evolution is still being intensely debated in studies of both modern evolution and marine ecology, increasingly so as ongoing data emerge from measurements of O2 levels both in geological samplesCitation117-Citation119,Citation122 and in regions of present-day oceans that have become hypoxic due to the influences of climate change.Citation123
Phylogeny of Some Oxygen Binding and Sensing Molecules
It is of interest that homologs of the globins, molecules that bind O2 via heme coordination, are found in modern prokaryotes.Citation124-Citation128 Perhaps such O2-carrying proteins evolved to capture the very small amounts of molecular O2 generated in early cellular life to be used for critical oxidation reactions such as tyrosine synthesis, hydroxylation of amino acids, and, in eukaryotes, sterol biosynthesisCitation129 and the hydroxylation of HIF1α.Citation2 Yet a scan of the current GenBank database indicates that the hypoxia responsive protein, HIF1α, is found only in eukaryotes. Homologs of the von Hippel-Lindau protein, which controls HIF degradation,Citation3,Citation4,Citation118 are found in Volvox.Citation130 Volvox is a spherical, sponge-like member of the green algae with a small number of specialized cell types, including germ cells.Citation67,Citation131 Of all extant organisms, Volvox is thought to be the best model system for understanding the emergence of the earliest multicellular organisms as they evolved from unicellular ancestors.Citation67,Citation131
Adhesion Molecules, Oxygen Levels, Prokaryotic Symbiosis and Multicellularity
The last universal common ancestor for all extant life was a prokaryote that had ribosomes and a plethora of redox proteins, but no organelles such as chloroplasts or mitochondria.Citation132-Citation136 The appearance of modern cellular organelles, including mitochondria and chloroplasts, and possibly cilia and flagella, almost certainly came about as a result of the symbiotic fusion of individual free-living unicellular organisms early in cellular evolution.Citation137-Citation139 Although famously championed by Lynn Margulis,Citation137 these ideas were suggested earlier by several authors.Citation115 Creation of such symbionts would likely be facilitated by adhesive mechanisms between cells. Support for an adhesive mechanism for symbiosis has been strengthened by the description of “predatory prokaryotes.”Citation140 These bacteria adhere to the surfaces of other bacteria allowing their entry into the host cell. Clearly molecular mechanisms for adhesivity, possibly driven by the induction of gene expression by increasing O2 during evolutionary time, might have facilitated such interactions.
Notably many transcription factors, in addition to HIF1α, are known to be sensitive to cellular redox state (which is influenced by, but not restricted to, alterations in O2 availability).Citation141-Citation144 Targets of these factors would increase the number of molecular changes that might increase in responsiveness to altered O2 concentrations in addition to the changes in adhesion molecules emphasized here.
The appearance of multicellularity also necessitated a mechanism for cell-to-cell and cell-to-substrate adhesion, which arose by diverse pathways in various lineages.Citation66,Citation67 As noted above, the volvocine algae are the extant organisms thought to most closely resemble the earliest multicellular organisms in evolution.Citation67 Volvox has a cell adhesion molecule homologous to fasciclin I in DrosophilaCitation145 as well as a sulfated glycoprotein that appears at a distinct phase of embryogenesis when it might facilitate cell interactions,Citation146,Citation147 such as those that form cytoplasmic bridges in the Volvox embryo.Citation148 A large number of extracellular matrix molecules in Volvox has been described biochemicallyCitation149,Citation150 and genomically.Citation130 Several distinct types of ECM zones have been observed ultrastructurally.Citation149 Moreover, ECM assembly has been shown to be inhibited by sulfhydryl reagents,Citation151 indicating sensitivity to the local redox environment. Thus molecules for cell and extracellular matrix adhesion exist and certainly play a critical role in this primitive multicellular organism.Citation57,Citation66,Citation67
Other organisms have been used in modern studies to mimic processes that supported the emergence of multicellularity. One is D. discoideum, a single-cell amoeboid organism that forms aggregates and subsequent fruiting bodies upon starvation. The process of aggregation is guided by the differential distribution of several cell and extracellular adhesion molecules.Citation152-Citation155 The genome of D. discoideum contains homologs to several families of adhesive proteins.Citation156 Indeed, the formation of epithelial structures during morphogenesis requires homologs of both α- and β-catenin, suggesting that these junctional and signaling proteins predated the appearance of metazoans.Citation157 In the case of multicellular colony formation in the single cell choanoflagellate, S. rosetta, colonies formed through cell division and not by the aggregation of individual cells.Citation158 Nevertheless, in both model organisms and the evolution of multicellularity, post-division adhesive mechanisms must have been operative to hold cells together, whether by direct cell adhesion or encasement within a common extracellular matrix, similar to what occurs in Volvox. A recent study that selected for aggregates in S. cerevisiae over several generations demonstrated that multicellularity could occur rapidly and provided another example of post-division colony formation.Citation159 Any of these model systems could be amenable to test whether exposure to O2 levels comparable to those that occurred during the emergence of multicellular organisms influences the expression of any of the known adhesion molecules in the respective systems. Such experiments would also be a good test of the extent of generality in the evolution of cell adhesion mechanisms.Citation66,Citation153
While it is not novel to suggest that various kinds of adhesion events were necessary for the emergence of multicellularity,Citation57,Citation66,Citation67 the recent studies described here raise the intriguing possibility that the increasing (and at times decreasing)Citation117,Citation160 O2 levels over evolutionary time might have exerted a strong selective influence on the evolution and production of adhesion molecule precursors.
Summary and Additional Considerations
Low atmospheric O2 concentrations, at levels that are now commonly referred to as “hypoxia,” were the norm during the evolution of multicellular organisms. It is possible that alterations in O2 levels drove the emergence and expression of molecules that supported adhesion thus facilitating prokaryotic symbiosis and multicellularity. HIF1 and its hydroxylating (PHD-family) enzymes probably did not evolve to deal with “hypoxia” but more likely acted as O2 sensors (as has been suggested for PHDCitation2,Citation161) in order to regulate the response of suites of genes to the local and atmospheric O2 environment over evolutionary time.Citation47,Citation162,Citation163 Consideration of the evolutionary origins of O2-responsive molecular mechanisms might greatly broaden our understanding of how such molecules function in development, especially in stem cell niches. For example, particular levels of O2 could select for suites of adhesion molecules that would select among and guide the processes of cell proliferation, migration, and differentiation. For example, altered adhesive mechanisms brought about by differing O2 levels may occur when stem cells leave the proliferative niche and migrate and differentiate in adjacent tissues. Such an idea is consistent with the observation that neural stem cells proliferate (and possibly switch between activity and quiescence) in the lowest O2 levels (reviewed in refs. Citation12 and Citation13) and that increased O2 levels support stem cell differentiation into particular lineages.Citation13
In addition to understanding diseases and development of the central nervous system,Citation13,Citation164 these issues are also useful in considering the consequences of hypoxia in tissues and the progression of diseases in which cellular niches low in O2 are thought to influence cell behavior, particularly cancerCitation165-Citation168 and other diseases of proliferative misregulation, such as inflammation, fibrosis and sclerosis.Citation169
Figure 1. Key events in biological evolution superimposed on the range of oxygen levels in the earth's atmosphere. The figure was modified from reference Citation117 with the author's permission. More recent data indicate a transient rise in levels (of 0.04–0.1 pO2) between 2.0 and 2.4 billion years before the present.Citation160 The biological evolutionary times are compiled from several references and citations within.Citation116,Citation117,Citation170 The geological times have wide variances and change as new data and models accumulate.
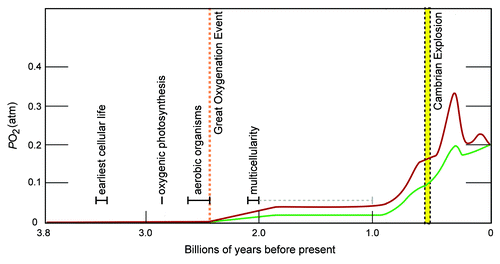
Acknowledgments
I thank Drs Gerald Edelman, David Edelman, Vincent Mauro, Joseph Gally and Bruce Cunningham for helpful discussions and for their critical reading of the manuscript. I also thank Dr Sigeng Chen for introducing me to the topic of changes in oxygen levels during geologic evolution. Thanks to Dr David Edelman for figure preparation. Work in the author's laboratory is supported by the Scripps Research Institute Department of Neurobiology, the Mathers Foundation and Promosome, L.L.P. There are no conflicts of interest.
References
- Lendahl U, Lee KL, Yang H, Poellinger L. Generating specificity and diversity in the transcriptional response to hypoxia. Nat Rev Genet 2009; 10:821 - 32; http://dx.doi.org/10.1038/nrg2665; PMID: 19884889
- Kaelin WG Jr., Ratcliffe PJ. Oxygen sensing by metazoans: the central role of the HIF hydroxylase pathway. Mol Cell 2008; 30:393 - 402; http://dx.doi.org/10.1016/j.molcel.2008.04.009; PMID: 18498744
- Kaelin WG Jr. The von Hippel-Lindau tumour suppressor protein: O2 sensing and cancer. Nat Rev Cancer 2008; 8:865 - 73; http://dx.doi.org/10.1038/nrc2502; PMID: 18923434
- Majmundar AJ, Wong WJ, Simon MC. Hypoxia-inducible factors and the response to hypoxic stress. Mol Cell 2010; 40:294 - 309; http://dx.doi.org/10.1016/j.molcel.2010.09.022; PMID: 20965423
- Webster KA. Evolution of the coordinate regulation of glycolytic enzyme genes by hypoxia. J Exp Biol 2003; 206:2911 - 22; http://dx.doi.org/10.1242/jeb.00516; PMID: 12878660
- Papandreou I, Cairns RA, Fontana L, Lim AL, Denko NC. HIF-1 mediates adaptation to hypoxia by actively downregulating mitochondrial oxygen consumption. Cell Metab 2006; 3:187 - 97; http://dx.doi.org/10.1016/j.cmet.2006.01.012; PMID: 16517406
- Mazumdar J, Dondeti V, Simon MC. Hypoxia-inducible factors in stem cells and cancer. J Cell Mol Med 2009; 13:4319 - 28; http://dx.doi.org/10.1111/j.1582-4934.2009.00963.x; PMID: 19900215
- Jiang BH, Semenza GL, Bauer C, Marti HH. Hypoxia-inducible factor 1 levels vary exponentially over a physiologically relevant range of O2 tension. Am J Physiol 1996; 271:C1172 - 80; PMID: 8897823
- Spellman PT, Sherlock G, Zhang MQ, Iyer VR, Anders K, Eisen MB, et al. Comprehensive identification of cell cycle-regulated genes of the yeast Saccharomyces cerevisiae by microarray hybridization. Mol Biol Cell 1998; 9:3273 - 97; PMID: 9843569
- Morriss GM, New DA. Effect of oxygen concentration on morphogenesis of cranial neural folds and neural crest in cultured rat embryos. J Embryol Exp Morphol 1979; 54:17 - 35; PMID: 528863
- Ivanovic Z. Hypoxia or in situ normoxia: The stem cell paradigm. J Cell Physiol 2009; 219:271 - 5; http://dx.doi.org/10.1002/jcp.21690; PMID: 19160417
- Simon MC, Keith B. The role of oxygen availability in embryonic development and stem cell function. Nat Rev Mol Cell Biol 2008; 9:285 - 96; http://dx.doi.org/10.1038/nrm2354; PMID: 18285802
- Panchision DM. The role of oxygen in regulating neural stem cells in development and disease. J Cell Physiol 2009; 220:562 - 8; http://dx.doi.org/10.1002/jcp.21812; PMID: 19441077
- Studer L, Csete M, Lee SH, Kabbani N, Walikonis J, Wold B, et al. Enhanced proliferation, survival, and dopaminergic differentiation of CNS precursors in lowered oxygen. J Neurosci 2000; 20:7377 - 83; PMID: 11007896
- Tsatmali M, Walcott EC, Crossin KL. Newborn neurons acquire high levels of reactive oxygen species and increased mitochondrial proteins upon differentiation from progenitors. Brain Res 2005; 1040:137 - 50; http://dx.doi.org/10.1016/j.brainres.2005.01.087; PMID: 15804435
- Chen HL, Pistollato F, Hoeppner DJ, Ni HT, McKay RD, Panchision DM. Oxygen tension regulates survival and fate of mouse central nervous system precursors at multiple levels. Stem Cells 2007; 25:2291 - 301; http://dx.doi.org/10.1634/stemcells.2006-0609; PMID: 17556599
- Milosevic J, Adler I, Manaenko A, Schwarz SC, Walkinshaw G, Arend M, et al. Non-hypoxic stabilization of hypoxia-inducible factor alpha (HIF-alpha): relevance in neural progenitor/stem cells. Neurotox Res 2009; 15:367 - 80; http://dx.doi.org/10.1007/s12640-009-9043-z; PMID: 19384570
- Zhou L, Miller CA. Mitogen-activated protein kinase signaling, oxygen sensors and hypoxic induction of neurogenesis. Neurodegener Dis 2006; 3:50 - 5; http://dx.doi.org/10.1159/000092093; PMID: 16909037
- Pistollato F, Chen HL, Schwartz PH, Basso G, Panchision DM. Oxygen tension controls the expansion of human CNS precursors and the generation of astrocytes and oligodendrocytes. Mol Cell Neurosci 2007; 35:424 - 35; http://dx.doi.org/10.1016/j.mcn.2007.04.003; PMID: 17498968
- Horie N, So K, Moriya T, Kitagawa N, Tsutsumi K, Nagata I, et al. Effects of oxygen concentration on the proliferation and differentiation of mouse neural stem cells in vitro. Cell Mol Neurobiol 2008; 28:833 - 45; http://dx.doi.org/10.1007/s10571-007-9237-y; PMID: 18236013
- Zhao T, Zhang CP, Liu ZH, Wu LY, Huang X, Wu HT, et al. Hypoxia-driven proliferation of embryonic neural stem/progenitor cells--role of hypoxia-inducible transcription factor-1alpha. FEBS J 2008; 275:1824 - 34; http://dx.doi.org/10.1111/j.1742-4658.2008.06340.x; PMID: 18341590
- Mazumdar J, O’Brien WT, Johnson RS, LaManna JC, Chavez JC, Klein PS, et al. O2 regulates stem cells through Wnt/β-catenin signalling. Nat Cell Biol 2010; 12:1007 - 13; http://dx.doi.org/10.1038/ncb2102; PMID: 20852629
- Ingraham CA, Park GC, Makarenkova HP, Crossin KL. Matrix metalloproteinase (MMP)-9 induced by Wnt signaling increases the proliferation and migration of embryonic neural stem cells at low O2 levels. J Biol Chem 2011; 286:17649 - 57; http://dx.doi.org/10.1074/jbc.M111.229427; PMID: 21460212
- Morrison SJ, Csete M, Groves AK, Melega W, Wold B, Anderson DJ. Culture in reduced levels of oxygen promotes clonogenic sympathoadrenal differentiation by isolated neural crest stem cells. J Neurosci 2000; 20:7370 - 6; PMID: 11007895
- Zhang CP, Zhu LL, Zhao T, Zhao H, Huang X, Ma X, et al. Characteristics of neural stem cells expanded in lowered oxygen and the potential role of hypoxia-inducible factor-1Alpha. Neurosignals 2006-2007; 15:259 - 65; http://dx.doi.org/10.1159/000103385; PMID: 17551265
- Krabbe C, Courtois E, Jensen P, Jørgensen JR, Zimmer J, Marti´nez-Serrano A, et al. Enhanced dopaminergic differentiation of human neural stem cells by synergistic effect of Bcl-xL and reduced oxygen tension. J Neurochem 2009; 110:1908 - 20; http://dx.doi.org/10.1111/j.1471-4159.2009.06281.x; PMID: 19627448
- Millman JR, Tan JH, Colton CK. The effects of low oxygen on self-renewal and differentiation of embryonic stem cells. Curr Opin Organ Transplant 2009; 14:694 - 700; http://dx.doi.org/10.1097/MOT.0b013e3283329d53; PMID: 19779343
- Harms KM, Li L, Cunningham LA. Murine neural stem/progenitor cells protect neurons against ischemia by HIF-1alpha-regulated VEGF signaling. PLoS One 2010; 5:e9767; http://dx.doi.org/10.1371/journal.pone.0009767; PMID: 20339541
- Santilli G, Lamorte G, Carlessi L, Ferrari D, Rota Nodari L, Binda E, et al. Mild hypoxia enhances proliferation and multipotency of human neural stem cells. PLoS One 2010; 5:e8575; http://dx.doi.org/10.1371/journal.pone.0008575; PMID: 20052410
- Yoshida Y, Takahashi K, Okita K, Ichisaka T, Yamanaka S. Hypoxia enhances the generation of induced pluripotent stem cells. Cell Stem Cell 2009; 5:237 - 41; http://dx.doi.org/10.1016/j.stem.2009.08.001; PMID: 19716359
- Clarke L, van der Kooy D. Low oxygen enhances primitive and definitive neural stem cell colony formation by inhibiting distinct cell death pathways. Stem Cells 2009; 27:1879 - 86; http://dx.doi.org/10.1002/stem.96; PMID: 19544448
- Lin Q, Kim Y, Alarcon RM, Yun Z. Oxygen and Cell Fate Decisions. Gene Regul Syst Bio 2008; 2:43 - 51; PMID: 19606268
- Dirken MN, Heemstra H. Alveolar oxygen tension and lung circulation. Q J Exp Physiol 1948; 34:193 - 211; PMID: 18111539
- Horng CT, Liu CC, Wu DM, Wu YC, Chen JT, Chang CJ, et al. Visual fields during acute exposure to a simulated altitude of 7620 m. Aviat Space Environ Med 2008; 79:666 - 9; http://dx.doi.org/10.3357/ASEM.2160.2008; PMID: 18619125
- Erecińska M, Silver IA. Tissue oxygen tension and brain sensitivity to hypoxia. Respir Physiol 2001; 128:263 - 76; http://dx.doi.org/10.1016/S0034-5687(01)00306-1; PMID: 11718758
- Hoffman CE, Clark RT Jr., Brown EB Jr. Blood oxygen saturations and duration of consciousness in anoxia at high altitudes. Am J Physiol 1946; 145:685 - 92; PMID: 21021848
- Vieira HL, Alves PM, Vercelli A. Modulation of neuronal stem cell differentiation by hypoxia and reactive oxygen species. Prog Neurobiol 2011; 93:444 - 55; http://dx.doi.org/10.1016/j.pneurobio.2011.01.007; PMID: 21251953
- Skloot R. The Immortal Life of Henrietta Lacks. Crown, 2010.
- Crossin KL. Cell adhesion molecules in embryogenesis and disease. Ann N Y Acad Sci 1991; 615:172 - 86; http://dx.doi.org/10.1111/j.1749-6632.1991.tb37759.x; PMID: 2039143
- Edelman GM, Crossin KL. Cell adhesion molecules: implications for a molecular histology. Annu Rev Biochem 1991; 60:155 - 90; http://dx.doi.org/10.1146/annurev.bi.60.070191.001103; PMID: 1883195
- Zhu LL, Zhao T, huang X, Liu ZH, Wu LY, Wu KW, et al. Gene expression profiles and metabolic changes in embryonic neural progenitor cells under low oxygen. Cell Reprogram 2011; 13:113 - 20; http://dx.doi.org/10.1089/cell.2010.0043; PMID: 21473688
- Song SW, Chang W, Song BW, Song H, Lim S, Kim HJ, et al. Integrin-linked kinase is required in hypoxic mesenchymal stem cells for strengthening cell adhesion to ischemic myocardium. Stem Cells 2009; 27:1358 - 65; http://dx.doi.org/10.1002/stem.47; PMID: 19489098
- Grayson WL, Zhao F, Bunnell B, Ma T. Hypoxia enhances proliferation and tissue formation of human mesenchymal stem cells. Biochem Biophys Res Commun 2007; 358:948 - 53; http://dx.doi.org/10.1016/j.bbrc.2007.05.054; PMID: 17521616
- Cowden Dahl KD, Robertson SE, Weaver VM, Simon MC. Hypoxia-inducible factor regulates alphavbeta3 integrin cell surface expression. Mol Biol Cell 2005; 16:1901 - 12; http://dx.doi.org/10.1091/mbc.E04-12-1082; PMID: 15689487
- Lekli I, Gurusamy N, Ray D, Tosaki A, Das DK. Redox regulation of stem cell mobilization. Can J Physiol Pharmacol 2009; 87:989 - 95; http://dx.doi.org/10.1139/Y09-102; PMID: 20029535
- Mohyeldin A, Garzón-Muvdi T, Quiñones-Hinojosa A. Oxygen in stem cell biology: a critical component of the stem cell niche. Cell Stem Cell 2010; 7:150 - 61; http://dx.doi.org/10.1016/j.stem.2010.07.007; PMID: 20682444
- Harrison JF, Haddad GG. Effects of oxygen on growth and size: synthesis of molecular, organismal, and evolutionary studies with Drosophila melanogaster. Annu Rev Physiol 2011; 73:95 - 113; http://dx.doi.org/10.1146/annurev-physiol-012110-142155; PMID: 20936942
- Wu B, Crampton SP, Hughes CC. Wnt signaling induces matrix metalloproteinase expression and regulates T cell transmigration. Immunity 2007; 26:227 - 39; http://dx.doi.org/10.1016/j.immuni.2006.12.007; PMID: 17306568
- Barker N. The canonical Wnt/beta-catenin signalling pathway. Methods Mol Biol 2008; 468:5 - 15; http://dx.doi.org/10.1007/978-1-59745-249-6_1; PMID: 19099242
- Nelson WJ, Nusse R. Convergence of Wnt, beta-catenin, and cadherin pathways. Science 2004; 303:1483 - 7; http://dx.doi.org/10.1126/science.1094291; PMID: 15001769
- Jones FS, Jing J, Stonehouse AH, Stevens A, Edelman GM. Caffeine stimulates cytochrome oxidase expression and activity in the striatum in a sexually dimorphic manner. Mol Pharmacol 2008; 74:673 - 84; http://dx.doi.org/10.1124/mol.108.046888; PMID: 18583456
- Eisenmann DM. Wnt signaling. WormBook 2005; 1 - 17; PMID: 18050402
- Cadigan KM, Peifer M. Wnt signaling from development to disease: insights from model systems. Cold Spring Harb Perspect Biol 2009; 1:a002881; http://dx.doi.org/10.1101/cshperspect.a002881; PMID: 20066091
- Heuberger J, Birchmeier W. Interplay of cadherin-mediated cell adhesion and canonical Wnt signaling. Cold Spring Harb Perspect Biol 2010; 2:a002915; http://dx.doi.org/10.1101/cshperspect.a002915; PMID: 20182623
- Sylvie J, Ellen C, Kris V. The role of Wnt in cell signaling and cell adhesion during early vertebrate development. Front Biosci 2012; 17:2352 - 66; PMID: 21622181
- Wu KJ, Yang MH. Epithelial-mesenchymal transition and cancer stemness: the Twist1-Bmi1 connection. Biosci Rep 2011; 31:449 - 55; PMID: 21919891
- Saburi S, McNeill H. Organising cells into tissues: new roles for cell adhesion molecules in planar cell polarity. Curr Opin Cell Biol 2005; 17:482 - 8; http://dx.doi.org/10.1016/j.ceb.2005.08.011; PMID: 16099635
- Wada H, Okamoto H. Roles of noncanonical Wnt/PCP pathway genes in neuronal migration and neurulation in zebrafish. Zebrafish 2009; 6:3 - 8; http://dx.doi.org/10.1089/zeb.2008.0557; PMID: 19250033
- Devlin C, Greco S, Martelli F, Ivan M. miR-210: More than a silent player in hypoxia. IUBMB Life 2011; 63:94 - 100; PMID: 21360638
- Huang S, He X. microRNAs: tiny RNA molecules, huge driving forces to move the cell. Protein Cell 2010; 1:916 - 26; http://dx.doi.org/10.1007/s13238-010-0116-9; PMID: 21204018
- McCormick R, Buffa FM, Ragoussis J, Harris AL. The role of hypoxia regulated microRNAs in cancer. Curr Top Microbiol Immunol 2010; 345:47 - 70; http://dx.doi.org/10.1007/82_2010_76; PMID: 20549470
- Crosby ME, Devlin CM, Glazer PM, Calin GA, Ivan M. Emerging roles of microRNAs in the molecular responses to hypoxia. Curr Pharm Des 2009; 15:3861 - 6; http://dx.doi.org/10.2174/138161209789649367; PMID: 19925433
- Dalmay T, Edwards DR. MicroRNAs and the hallmarks of cancer. Oncogene 2006; 25:6170 - 5; http://dx.doi.org/10.1038/sj.onc.1209911; PMID: 17028596
- Pocock R. Invited review: decoding the microRNA response to hypoxia. Pflugers Arch 2011; 461:307 - 15; http://dx.doi.org/10.1007/s00424-010-0910-5; PMID: 21207057
- Schliekelman MJ, Gibbons DL, Faca VM, Creighton CJ, Rizvi ZH, Zhang Q, et al. Targets of the tumor suppressor miR-200 in regulation of the epithelial-mesenchymal transition in cancer. Cancer Res 2011; 71:7670 - 82; http://dx.doi.org/10.1158/0008-5472.CAN-11-0964; PMID: 21987723
- Abedin M, King N. Diverse evolutionary paths to cell adhesion. Trends Cell Biol 2010; 20:734 - 42; http://dx.doi.org/10.1016/j.tcb.2010.08.002; PMID: 20817460
- Kirk DL. A twelve-step program for evolving multicellularity and a division of labor. Bioessays 2005; 27:299 - 310; http://dx.doi.org/10.1002/bies.20197; PMID: 15714559
- James JL, Stone PR, Chamley LW. The regulation of trophoblast differentiation by oxygen in the first trimester of pregnancy. Hum Reprod Update 2006; 12:137 - 44; http://dx.doi.org/10.1093/humupd/dmi043; PMID: 16234296
- Pringle KG, Kind KL, Sferruzzi-Perri AN, Thompson JG, Roberts CT. Beyond oxygen: complex regulation and activity of hypoxia inducible factors in pregnancy. Hum Reprod Update 2010; 16:415 - 31; http://dx.doi.org/10.1093/humupd/dmp046; PMID: 19926662
- Tuuli MG, Longtine MS, Nelson DM. Review: Oxygen and trophoblast biology--a source of controversy. Placenta 2011; 32:Suppl 2 S109 - 18; http://dx.doi.org/10.1016/j.placenta.2010.12.013; PMID: 21216006
- Golan MH, Mane R, Molczadzki G, Zuckerman M, Kaplan-Louson V, Huleihel M, et al. Impaired migration signaling in the hippocampus following prenatal hypoxia. Neuropharmacology 2009; 57:511 - 22; http://dx.doi.org/10.1016/j.neuropharm.2009.07.028; PMID: 19635490
- Lee YM, Jeong CH, Koo SY, Son MJ, Song HS, Bae SK, et al. Determination of hypoxic region by hypoxia marker in developing mouse embryos in vivo: a possible signal for vessel development. Dev Dyn 2001; 220:175 - 86; http://dx.doi.org/10.1002/1097-0177(20010201)220:2<175::AID-DVDY1101>3.0.CO;2-F; PMID: 11169851
- Slevin M, Krupinski J, Rovira N, Turu M, Luque A, Baldellou M, et al. Identification of pro-angiogenic markers in blood vessels from stroked-affected brain tissue using laser-capture microdissection. BMC Genomics 2009; 10:113; http://dx.doi.org/10.1186/1471-2164-10-113; PMID: 19292924
- Li L, Liu F, Welser-Alves JV, McCullough LD, Milner R. Upregulation of fibronectin and the alpha5beta1 and alphavbeta3 integrins on blood vessels within the cerebral ischemic penumbra. Exp Neurol 2012; 233:283 - 91; http://dx.doi.org/10.1016/j.expneurol.2011.10.017; PMID: 22056225
- Li L, Welser JV, Dore-Duffy P, del Zoppo GJ, Lamanna JC, Milner R. In the hypoxic central nervous system, endothelial cell proliferation is followed by astrocyte activation, proliferation, and increased expression of the alpha 6 beta 4 integrin and dystroglycan. Glia 2010; 58:1157 - 67; http://dx.doi.org/10.1002/glia.20995; PMID: 20544851
- Abbruscato TJ, Davis TP. Protein expression of brain endothelial cell E-cadherin after hypoxia/aglycemia: influence of astrocyte contact. Brain Res 1999; 842:277 - 86; http://dx.doi.org/10.1016/S0006-8993(99)01778-3; PMID: 10526124
- Doetsch F, Caille´ I, Lim DA, Garci´a-Verdugo JM, Alvarez-Buylla A. Subventricular zone astrocytes are neural stem cells in the adult mammalian brain. Cell 1999; 97:703 - 16; http://dx.doi.org/10.1016/S0092-8674(00)80783-7; PMID: 10380923
- Cremer H, Lange R, Christoph A, Plomann M, Vopper G, Roes J, et al. Inactivation of the N-CAM gene in mice results in size reduction of the olfactory bulb and deficits in spatial learning. Nature 1994; 367:455 - 9; http://dx.doi.org/10.1038/367455a0; PMID: 8107803
- Ono K, Tomasiewicz H, Magnuson T, Rutishauser U. N-CAM mutation inhibits tangential neuronal migration and is phenocopied by enzymatic removal of polysialic acid. Neuron 1994; 13:595 - 609; http://dx.doi.org/10.1016/0896-6273(94)90028-0; PMID: 7917293
- Nait-Oumesmar B, Decker L, Lachapelle F, Avellana-Adalid V, Bachelin C, Van Evercooren AB. Progenitor cells of the adult mouse subventricular zone proliferate, migrate and differentiate into oligodendrocytes after demyelination. Eur J Neurosci 1999; 11:4357 - 66; http://dx.doi.org/10.1046/j.1460-9568.1999.00873.x; PMID: 10594662
- Decker L, Durbec P, Rougon G, Evercooren AB. Loss of polysialic residues accelerates CNS neural precursor differentiation in pathological conditions. Mol Cell Neurosci 2002; 19:225 - 38; http://dx.doi.org/10.1006/mcne.2001.1072; PMID: 11860275
- Keirstead HS, Ben-Hur T, Rogister B, O’Leary MT, Dubois-Dalcq M, Blakemore WF. Polysialylated neural cell adhesion molecule-positive CNS precursors generate both oligodendrocytes and Schwann cells to remyelinate the CNS after transplantation. J Neurosci 1999; 19:7529 - 36; PMID: 10460259
- Courtès S, Vernerey J, Pujadas L, Magalon K, Cremer H, Soriano E, et al. Reelin controls progenitor cell migration in the healthy and pathological adult mouse brain. PLoS One 2011; 6:e20430; http://dx.doi.org/10.1371/journal.pone.0020430; PMID: 21647369
- Chen J, Bernreuther C, Dihne´ M, Schachner M. Cell adhesion molecule l1-transfected embryonic stem cells with enhanced survival support regrowth of corticospinal tract axons in mice after spinal cord injury. J Neurotrauma 2005; 22:896 - 906; http://dx.doi.org/10.1089/neu.2005.22.896; PMID: 16083356
- Cui YF, Xu JC, Hargus G, Jakovcevski I, Schachner M, Bernreuther C. Embryonic stem cell-derived L1 overexpressing neural aggregates enhance recovery after spinal cord injury in mice. PLoS One 2011; 6:e17126; http://dx.doi.org/10.1371/journal.pone.0017126; PMID: 21445247
- Bernreuther C, Dihne´ M, Johann V, Schiefer J, Cui Y, Hargus G, et al. Neural cell adhesion molecule L1-transfected embryonic stem cells promote functional recovery after excitotoxic lesion of the mouse striatum. J Neurosci 2006; 26:11532 - 9; http://dx.doi.org/10.1523/JNEUROSCI.2688-06.2006; PMID: 17093074
- Liauw J, Hoang S, Choi M, Eroglu C, Choi M, Sun GH, et al. Thrombospondins 1 and 2 are necessary for synaptic plasticity and functional recovery after stroke. J Cereb Blood Flow Metab 2008; 28:1722 - 32; http://dx.doi.org/10.1038/jcbfm.2008.65; PMID: 18594557
- Guzman R, De Los Angeles A, Cheshier S, Choi R, Hoang S, Liauw J, et al. Intracarotid injection of fluorescence activated cell-sorted CD49d-positive neural stem cells improves targeted cell delivery and behavior after stroke in a mouse stroke model. Stroke 2008; 39:1300 - 6; http://dx.doi.org/10.1161/STROKEAHA.107.500470; PMID: 18309158
- Li D, Zhou J, Chowdhury F, Cheng J, Wang N, Wang F. Role of mechanical factors in fate decisions of stem cells. Regen Med 2011; 6:229 - 40; http://dx.doi.org/10.2217/rme.11.2; PMID: 21391856
- Doetsch F. A niche for adult neural stem cells. Curr Opin Genet Dev 2003; 13:543 - 50; http://dx.doi.org/10.1016/j.gde.2003.08.012; PMID: 14550422
- Shen Q, Goderie SK, Jin L, Karanth N, Sun Y, Abramova N, et al. Endothelial cells stimulate self-renewal and expand neurogenesis of neural stem cells. Science 2004; 304:1338 - 40; http://dx.doi.org/10.1126/science.1095505; PMID: 15060285
- Barami K. Relationship of neural stem cells with their vascular niche: implications in the malignant progression of gliomas. J Clin Neurosci 2008; 15:1193 - 7; http://dx.doi.org/10.1016/j.jocn.2008.01.002; PMID: 18617407
- Huang Z, Cheng L, Guryanova OA, Wu Q, Bao S. Cancer stem cells in glioblastoma--molecular signaling and therapeutic targeting. Protein Cell 2010; 1:638 - 55; http://dx.doi.org/10.1007/s13238-010-0078-y; PMID: 21203936
- Bar EE. Glioblastoma, cancer stem cells and hypoxia. Brain Pathol 2011; 21:119 - 29; http://dx.doi.org/10.1111/j.1750-3639.2010.00460.x; PMID: 21054626
- Westphal M, Lamszus K. The neurobiology of gliomas: from cell biology to the development of therapeutic approaches. Nat Rev Neurosci 2011; 12:495 - 508; http://dx.doi.org/10.1038/nrn3060; PMID: 21811295
- Wouters BG, Koritzinsky M. Hypoxia signalling through mTOR and the unfolded protein response in cancer. Nat Rev Cancer 2008; 8:851 - 64; http://dx.doi.org/10.1038/nrc2501; PMID: 18846101
- Bernhard EJ. Interventions that induce modifications in the tumor microenvironment. Cancer Radiother 2011; 15:376 - 82; http://dx.doi.org/10.1016/j.canrad.2011.01.007; PMID: 21571567
- Binello E, Germano IM. Targeting glioma stem cells: a novel framework for brain tumors. Cancer Sci 2011; 102:1958 - 66; http://dx.doi.org/10.1111/j.1349-7006.2011.02064.x; PMID: 21848914
- Khain E, Katakowski M, Hopkins S, Szalad A, Zheng X, Jiang F, et al. Collective behavior of brain tumor cells: the role of hypoxia. Phys Rev E Stat Nonlin Soft Matter Phys 2011; 83:031920; http://dx.doi.org/10.1103/PhysRevE.83.031920; PMID: 21517536
- Siesser PF, Maness PF. L1 cell adhesion molecules as regulators of tumor cell invasiveness. Cell Adh Migr 2009; 3:275 - 7; http://dx.doi.org/10.4161/cam.3.3.8689; PMID: 19483471
- Zhang N, Wei P, Gong A, Chiu WT, Lee HT, Colman H, et al. FoxM1 promotes β-catenin nuclear localization and controls Wnt target-gene expression and glioma tumorigenesis. Cancer Cell 2011; 20:427 - 42; http://dx.doi.org/10.1016/j.ccr.2011.08.016; PMID: 22014570
- Friedl P, Alexander S. Cancer invasion and the microenvironment: plasticity and reciprocity. Cell 2011; 147:992 - 1009; http://dx.doi.org/10.1016/j.cell.2011.11.016; PMID: 22118458
- Li HG, Ren YM, Guo SC, Cheng L, Wang DP, Yang J, et al. The protein level of hypoxia-inducible factor-1alpha is increased in the plateau pika (Ochotona curzoniae) inhabiting high altitudes. J Exp Zool A Ecol Genet Physiol 2009; 311:134 - 41; http://dx.doi.org/10.1002/jez.510; PMID: 19048601
- Yi X, Liang Y, Huerta-Sanchez E, Jin X, Cuo ZX, Pool JE, et al. Sequencing of 50 human exomes reveals adaptation to high altitude. Science 2010; 329:75 - 8; http://dx.doi.org/10.1126/science.1190371; PMID: 20595611
- Peng Y, Yang Z, Zhang H, Cui C, Qi X, Luo X, et al. Genetic variations in Tibetan populations and high-altitude adaptation at the Himalayas. Mol Biol Evol 2011; 28:1075 - 81; http://dx.doi.org/10.1093/molbev/msq290; PMID: 21030426
- Storz JF, Scott GR, Cheviron ZA. Phenotypic plasticity and genetic adaptation to high-altitude hypoxia in vertebrates. J Exp Biol 2010; 213:4125 - 36; http://dx.doi.org/10.1242/jeb.048181; PMID: 21112992
- Bigham A, Bauchet M, Pinto D, Mao X, Akey JM, Mei R, et al. Identifying signatures of natural selection in Tibetan and Andean populations using dense genome scan data. PLoS Genet 2010; 6:e1001116; http://dx.doi.org/10.1371/journal.pgen.1001116; PMID: 20838600
- Simonson TS, Yang Y, Huff CD, Yun H, Qin G, Witherspoon DJ, et al. Genetic evidence for high-altitude adaptation in Tibet. Science 2010; 329:72 - 5; http://dx.doi.org/10.1126/science.1189406; PMID: 20466884
- Zhou D, Xue J, Chen J, Morcillo P, Lambert JD, White KP, et al. Experimental selection for Drosophila survival in extremely low O(2) environment. PLoS One 2007; 2:e490; http://dx.doi.org/10.1371/journal.pone.0000490; PMID: 17534440
- Zhou D, Visk DW, Haddad GG. Drosophila, a golden bug, for the dissection of the genetic basis of tolerance and susceptibility to hypoxia. Pediatr Res 2009; 66:239 - 47; http://dx.doi.org/10.1203/PDR.0b013e3181b27275; PMID: 19542900
- Shams I, Avivi A, Nevo E. Hypoxic stress tolerance of the blind subterranean mole rat: expression of erythropoietin and hypoxia-inducible factor 1 alpha. Proc Natl Acad Sci U S A 2004; 101:9698 - 703; http://dx.doi.org/10.1073/pnas.0403540101; PMID: 15210955
- Shams I, Nevo E, Avivi A. Ontogenetic expression of erythropoietin and hypoxia-inducible factor-1 alpha genes in subterranean blind mole rats. FASEB J 2005; 19:307 - 9; PMID: 15574488
- Semenza GL. Hypoxia-inducible factor 1: regulator of mitochondrial metabolism and mediator of ischemic preconditioning. Biochim Biophys Acta 2011; 1813:1263 - 8; http://dx.doi.org/10.1016/j.bbamcr.2010.08.006; PMID: 20732359
- Raymond J, Segrè D. The effect of oxygen on biochemical networks and the evolution of complex life. Science 2006; 311:1764 - 7; http://dx.doi.org/10.1126/science.1118439; PMID: 16556842
- Broda E. The Evolution of the Bioenergetic Processes. Great Britain: Pergamon Press, 1978.
- Cavalier-Smith T. Cell evolution and Earth history: stasis and revolution. Philos Trans R Soc Lond B Biol Sci 2006; 361:969 - 1006; http://dx.doi.org/10.1098/rstb.2006.1842; PMID: 16754610
- Holland HD. The oxygenation of the atmosphere and oceans. Philos Trans R Soc Lond B Biol Sci 2006; 361:903 - 15; http://dx.doi.org/10.1098/rstb.2006.1838; PMID: 16754606
- Johnston DT, Wolfe-Simon F, Pearson A, Knoll AH. Anoxygenic photosynthesis modulated Proterozoic oxygen and sustained Earth’s middle age. Proc Natl Acad Sci U S A 2009; 106:16925 - 9; http://dx.doi.org/10.1073/pnas.0909248106; PMID: 19805080
- Dahl TW, Hammarlund EU, Anbar AD, Bond DP, Gill BC, Gordon GW, et al. Devonian rise in atmospheric oxygen correlated to the radiations of terrestrial plants and large predatory fish. Proc Natl Acad Sci U S A 2010; 107:17911 - 5; http://dx.doi.org/10.1073/pnas.1011287107; PMID: 20884852
- Dahl TW, Hammarlund EU. Do large predatory fish track ocean oxygenation?. Commun Integr Biol 2011; 4:92 - 4; http://dx.doi.org/10.4161/cib.4.1.14119; PMID: 21509191
- Williams M, Vannier J, Corbari L, Massabuau JC. Oxygen as a driver of early arthropod micro-benthos evolution. PLoS One 2011; 6:e28183; http://dx.doi.org/10.1371/journal.pone.0028183; PMID: 22164241
- Saltzman MR, Young SA, Kump LR, Gill BC, Lyons TW, Runnegar B. Pulse of atmospheric oxygen during the late Cambrian. Proc Natl Acad Sci U S A 2011; 108:3876 - 81; http://dx.doi.org/10.1073/pnas.1011836108; PMID: 21368152
- Deutsch C, Brix H, Ito T, Frenzel H, Thompson L. Climate-forced variability of ocean hypoxia. Science 2011; 333:336 - 9; http://dx.doi.org/10.1126/science.1202422; PMID: 21659566
- Hoffmann FG, Opazo JC, Storz JF. Differential loss and retention of cytoglobin, myoglobin, and globin-E during the radiation of vertebrates. Genome Biol Evol 2011; 3:588 - 600; http://dx.doi.org/10.1093/gbe/evr055; PMID: 21697098
- Nothnagel HJ, Winer BY, Vuletich DA, Pond MP, Lecomte JT. Structural properties of 2/2 hemoglobins: the group III protein from Helicobacter hepaticus. IUBMB Life 2011; 63:197 - 205; http://dx.doi.org/10.1002/iub.430; PMID: 21445851
- Ascenzi P, Bolognesi M, Milani M, Guertin M, Visca P. Mycobacterial truncated hemoglobins: from genes to functions. Gene 2007; 398:42 - 51; http://dx.doi.org/10.1016/j.gene.2007.02.043; PMID: 17532149
- Freitas TA, Saito JA, Hou S, Alam M. Globin-coupled sensors, protoglobins, and the last universal common ancestor. J Inorg Biochem 2005; 99:23 - 33; http://dx.doi.org/10.1016/j.jinorgbio.2004.10.024; PMID: 15598488
- Vuletich DA, Lecomte JT. A phylogenetic and structural analysis of truncated hemoglobins. J Mol Evol 2006; 62:196 - 210; http://dx.doi.org/10.1007/s00239-005-0077-4; PMID: 16474979
- Summons RE, Bradley AS, Jahnke LL, Waldbauer JR. Steroids, triterpenoids and molecular oxygen. Philos Trans R Soc Lond B Biol Sci 2006; 361:951 - 68; http://dx.doi.org/10.1098/rstb.2006.1837; PMID: 16754609
- Prochnik SE, Umen J, Nedelcu AM, Hallmann A, Miller SM, Nishii I, et al. Genomic analysis of organismal complexity in the multicellular green alga Volvox carteri. Science 2010; 329:223 - 6; http://dx.doi.org/10.1126/science.1188800; PMID: 20616280
- Herron MD, Hackett JD, Aylward FO, Michod RE. Triassic origin and early radiation of multicellular volvocine algae. Proc Natl Acad Sci U S A 2009; 106:3254 - 8; http://dx.doi.org/10.1073/pnas.0811205106; PMID: 19223580
- Forterre P, Philippe H. The last universal common ancestor (LUCA), simple or complex?. Biol Bull 1999; 196:373 - 5, discussion 375-7; http://dx.doi.org/10.2307/1542973; PMID: 11536914
- Doolittle WF. Phylogenetic classification and the universal tree. Science 1999; 284:2124 - 9; http://dx.doi.org/10.1126/science.284.5423.2124; PMID: 10381871
- Cavalier-Smith T. The neomuran origin of archaebacteria, the negibacterial root of the universal tree and bacterial megaclassification. Int J Syst Evol Microbiol 2002; 52:7 - 76; PMID: 11837318
- Harris JK, Kelley ST, Spiegelman GB, Pace NR. The genetic core of the universal ancestor. Genome Res 2003; 13:407 - 12; http://dx.doi.org/10.1101/gr.652803; PMID: 12618371
- Baymann F, Lebrun E, Brugna M, Schoepp-Cothenet B, Giudici-Orticoni MT, Nitschke W. The redox protein construction kit: pre-last universal common ancestor evolution of energy-conserving enzymes. Philos Trans R Soc Lond B Biol Sci 2003; 358:267 - 74; http://dx.doi.org/10.1098/rstb.2002.1184; PMID: 12594934
- Margulis L. Symbiosis and evolution. Sci Am 1971; 225:48 - 57; http://dx.doi.org/10.1038/scientificamerican0871-48; PMID: 5089455
- Margulis L. Symbiotic theory of the origin of eukaryotic organelles; criteria for proof. Symp Soc Exp Biol 1975; 29:21 - 38; PMID: 822529
- Sagan D, Margulis L. Bacterial bedfellows: a microscopic menage a trois may be responsible for a major step in evolution. Nat Hist 1987; 96:26 - 33; PMID: 11542100
- Guerrero R, Pedros-Alio C, Esteve I, Mas J, Chase D, Margulis L. Predatory prokaryotes: predation and primary consumption evolved in bacteria. Proc Natl Acad Sci U S A 1986; 83:2138 - 42; http://dx.doi.org/10.1073/pnas.83.7.2138; PMID: 11542073
- Bauer CE, Elsen S, Bird TH. Mechanisms for redox control of gene expression. Annu Rev Microbiol 1999; 53:495 - 523; http://dx.doi.org/10.1146/annurev.micro.53.1.495; PMID: 10547699
- Poyton RO. Models for oxygen sensing in yeast: implications for oxygen-regulated gene expression in higher eucaryotes. Respir Physiol 1999; 115:119 - 33; http://dx.doi.org/10.1016/S0034-5687(99)00028-6; PMID: 10385027
- Liu H, Colavitti R, Rovira II, Finkel T. Redox-dependent transcriptional regulation. Circ Res 2005; 97:967 - 74; http://dx.doi.org/10.1161/01.RES.0000188210.72062.10; PMID: 16284189
- Shih SC, Claffey KP. Hypoxia-mediated regulation of gene expression in mammalian cells. Int J Exp Pathol 1998; 79:347 - 57; http://dx.doi.org/10.1046/j.1365-2613.1998.00088.x; PMID: 10319016
- Huber O, Sumper M. Algal-CAMs: isoforms of a cell adhesion molecule in embryos of the alga Volvox with homology to Drosophila fasciclin I. EMBO J 1994; 13:4212 - 22; PMID: 7925267
- Wenzl S, Sumper M. Sulfation of a cell surface glycoprotein correlates with the developmental program during embryogenesis of Volvox carteri. Proc Natl Acad Sci U S A 1981; 78:3716 - 20; http://dx.doi.org/10.1073/pnas.78.6.3716; PMID: 6943578
- Hallmann A, Kirk DL. The developmentally regulated ECM glycoprotein ISG plays an essential role in organizing the ECM and orienting the cells of Volvox. J Cell Sci 2000; 113:4605 - 17; PMID: 11082052
- Green KJ, Viamontes GI, Kirk DL. Mechanism of formation, ultrastructure, and function of the cytoplasmic bridge system during morphogenesis in Volvox. J Cell Biol 1981; 91:756 - 69; http://dx.doi.org/10.1083/jcb.91.3.756; PMID: 7328120
- Sumper M, Hallmann A. Biochemistry of the extracellular matrix of Volvox. Int Rev Cytol 1998; 180:51 - 85; http://dx.doi.org/10.1016/S0074-7696(08)61770-2; PMID: 9496634
- Ender F, Godl K, Wenzl S, Sumper M. Evidence for autocatalytic cross-linking of hydroxyproline-rich glycoproteins during extracellular matrix assembly in Volvox. Plant Cell 2002; 14:1147 - 60; http://dx.doi.org/10.1105/tpc.000711; PMID: 12034903
- Sumper M, Nink J, Wenzl S. Self-assembly and cross-linking of Volvox extracellular matrix glycoproteins are specifically inhibited by Ellman’s reagent. Eur J Biochem 2000; 267:2334 - 9; http://dx.doi.org/10.1046/j.1432-1327.2000.01241.x; PMID: 10759858
- Siu CH, Harris TJ, Wang J, Wong E. Regulation of cell-cell adhesion during Dictyostelium development. Semin Cell Dev Biol 2004; 15:633 - 41; http://dx.doi.org/10.1016/j.semcdb.2004.09.004; PMID: 15561583
- Bowers-Morrow VM, Ali SO, Williams KL. Comparison of molecular mechanisms mediating cell contact phenomena in model developmental systems: an exploration of universality. Biol Rev Camb Philos Soc 2004; 79:611 - 42; http://dx.doi.org/10.1017/S1464793103006389; PMID: 15366765
- Siu CH, Sriskanthadevan S, Wang J, Hou L, Chen G, Xu X, et al. Regulation of spatiotemporal expression of cell-cell adhesion molecules during development of Dictyostelium discoideum. Dev Growth Differ 2011; 53:518 - 27; http://dx.doi.org/10.1111/j.1440-169X.2011.01267.x; PMID: 21585356
- Sriskanthadevan S, Zhu Y, Manoharan K, Yang C, Siu CH. The cell adhesion molecule DdCAD-1 regulates morphogenesis through differential spatiotemporal expression in Dictyostelium discoideum. Development 2011; 138:2487 - 97; http://dx.doi.org/10.1242/dev.060129; PMID: 21561987
- Kuspa A, Loomis WF. The Genome of Dictyostelium discoideum. Methods Mol Biol 2006; 346:15 - 30; http://dx.doi.org/10.1385/1-59745-144-4:15; PMID: 16957282
- Dickinson DJ, Nelson WJ, Weis WI. A polarized epithelium organized by beta- and alpha-catenin predates cadherin and metazoan origins. Science 2011; 331:1336 - 9; http://dx.doi.org/10.1126/science.1199633; PMID: 21393547
- Fairclough SR, Dayel MJ, King N. Multicellular development in a choanoflagellate. Curr Biol 2010; 20:R875 - 6; http://dx.doi.org/10.1016/j.cub.2010.09.014; PMID: 20971426
- Ratcliff WC, Denison RF, Borrello M, Travisano M. Experimental evolution of multicellularity. Proc Natl Acad Sci USA 2012; 1009:1595 - 600; http://dx.doi.org/10.1073/pnas.1115323109; PMID: 22307617
- Bekker A, Holland HD. Oxygen overshoot and recovery during the early Paleoproterozoic. Earth Planet Sci Lett 2012; 317-318:295 - 304; http://dx.doi.org/10.1016/j.epsl.2011.12.012
- Roberts AM, Ohh M. Beyond the hypoxia-inducible factor-centric tumour suppressor model of von Hippel-Lindau. Curr Opin Oncol 2008; 20:83 - 9; http://dx.doi.org/10.1097/CCO.0b013e3282f310de; PMID: 18043261
- Loenarz C, Coleman ML, Boleininger A, Schierwater B, Holland PW, Ratcliffe PJ, et al. The hypoxia-inducible transcription factor pathway regulates oxygen sensing in the simplest animal, Trichoplax adhaerens. EMBO Rep 2011; 12:63 - 70; http://dx.doi.org/10.1038/embor.2010.170; PMID: 21109780
- Rytkönen KT, Storz JF. Evolutionary origins of oxygen sensing in animals. EMBO Rep 2011; 12:3 - 4; http://dx.doi.org/10.1038/embor.2010.192; PMID: 21109778
- Zhang K, Zhu L, Fan M. Oxygen, a Key Factor Regulating Cell Behavior during Neurogenesis and Cerebral Diseases. Front Mol Neurosci 2011; 4:5; http://dx.doi.org/10.3389/fnmol.2011.00005; PMID: 21503147
- Allen M, Louise Jones J. Jekyll and Hyde: the role of the microenvironment on the progression of cancer. J Pathol 2011; 223:162 - 76; http://dx.doi.org/10.1002/path.2803; PMID: 21125673
- Welford SM, Giaccia AJ. Hypoxia and senescence: the impact of oxygenation on tumor suppression. Mol Cancer Res 2011; 9:538 - 44; http://dx.doi.org/10.1158/1541-7786.MCR-11-0065; PMID: 21385881
- Semenza GL. Defining the role of hypoxia-inducible factor 1 in cancer biology and therapeutics. Oncogene 2010; 29:625 - 34; http://dx.doi.org/10.1038/onc.2009.441; PMID: 19946328
- Lu X, Kang Y. Hypoxia and hypoxia-inducible factors: master regulators of metastasis. Clin Cancer Res 2010; 16:5928 - 35; http://dx.doi.org/10.1158/1078-0432.CCR-10-1360; PMID: 20962028
- Cassavaugh J, Lounsbury KM. Hypoxia-mediated biological control. J Cell Biochem 2011; 112:735 - 44; http://dx.doi.org/10.1002/jcb.22956; PMID: 21328446
- El Albani A, Bengtson S, Canfield DE, Bekker A, Macchiarelli R, Mazurier A, et al. Large colonial organisms with coordinated growth in oxygenated environments 2.1 Gyr ago. Nature 2010; 466:100 - 4; http://dx.doi.org/10.1038/nature09166; PMID: 20596019
- Semenza GL. Hypoxia inducible factors in physiology and medicine. Cell 2012; 148:399 - 408; http://dx.doi.org/10.1016/j.cell.2012.01.021; PMID: 22304911