Abstract
Telomere length homeostasis is achieved by a balance of telomere shortening caused by DNA replication and nucleolytic attack and telomere lengthening by telomerase. The importance of telomere length maintenance to human health is best illustrated by dyskeratosis congenita (DC) a disease of telomere shortening caused by mutations in telomerase subunits. DC patients suffer stem cell depletion and die of bone marrow stem cell failure. Recently a new class of particularly severe DC patients was found to harbor mutations in the shelterin subunit TIN2. The DC-TIN2 mutations were clustered in small domain of unknown function. In a recently published study we showed that the DC mutation cluster in TIN2 harbored a binding site for heterochromatin protein 1 (HP1) and further, that HP1 binding to TIN2 was required for sister telomere cohesion in S phase and for telomere length maintenance by telomerase. We briefly review and discuss the implications of our findings in this Extra View, and present some new data that may shed light on how sister telomere cohesion could influence telomere elongation by telomerase.
Introduction
Human telomeres are comprised of double-stranded TTAGGG repeats that run out as a single stranded overhang to the 3′ end of the chromosome and a six-subunit complex called shelterin.Citation1 Due to the end replication problem and nucleolytic processing, telomeres shorten with each round of cell division. This shortening functions as a molecular clock inducing cells to cease division and senesce.Citation2,Citation3 Shortening can be counteracted by telomerase, a specialized reverse transcriptase that adds telomere repeats to chromosome ends.Citation4,Citation5 In humans, telomerase is expressed in the germ line and during embryogenesis but is repressed for the most part in the human soma.Citation6 Hence, the stock of telomeres that an individual possesses at birth must suffice over their lifetime. Defects in telomere maintenance can have severe consequences for human health, as evidenced by the genetic disease dyskeratosis congenita (DC).Citation7 DC is caused by mutations in telomerase subunits, resulting in very short telomeres in highly proliferating tissues.Citation8,Citation9 DC patients suffer stem cell depletion and die of bone marrow stem cell failure. Understanding how telomerase functions in the germ line and during embryogenesis will be crucial to understanding the mechanism of disease in DC and the role of telomerase in human health.
Telomere Length Maintenance in Human Tumor Cells: Multiple Mechanisms
Although repressed in the human soma,Citation6 telomerase is upregulated in most tumorsCitation10–Citation13 and is required for telomere length maintenance and tumor cell growth.Citation14,Citation15 Telomere length regulation by telomerase has been primarily studied in human tumor cells, where it has been shown that telomerase activity is regulated by proteins that act in cis at individual chromosome ends to control recruitment or access to telomerase.Citation16 Telomerase-positive human tumor cells maintain their telomeres at a constant length setting via a negative feedback loop involving shelterin. Shelterin contains two double-strand telomere repeat binding proteins, TRF1Citation17 and TRF2.Citation18,Citation19 Both are bound by the central subunit TIN2Citation20 that further binds TPP1,Citation21–Citation23 the linker to POT1, the single-strand overhang binding protein.Citation24,Citation25 The sixth subunit, RAP1, is bound to TRF2.Citation26 Telomere length regulation has been studied by generating stable tumor cell lines overexpressing or depleting individual shelterin subunits and analyzing telomere length changes over multiple generations. In this way, TRF1, the first mammalian telomere protein to be identified, was shown to be a negative regulator of telomere length by telomerase.Citation27,Citation28 Overexpression of TRF1 in HTC75 telomerase-positive tumor cells resulted in progressive telomere shortening, whereas removal of TRF1 from telomeres by a dominant-negative allele led to progressive telomere elongation.Citation28
Shortly after TRF1 was identified, two-hybrid screens yielded two TRF1 binding partners, tankyrase1Citation29 and TIN2Citation20 (). TIN2 turned out to be a bona fide component of the shelterin complex, while tankyrase 1 was a shelterin accessory factor that transiently associated with TRF1 and telomeres. However, both regulated TRF1 stability and telomere length. Overexpression of a nuclear allele of tankyrase 1 (FN-tankyrase 1) led to loss of TRF1 from telomeresCitation30 (due to PARsylation) and TRF1 degradation by the proteasome.Citation31 Stable HTC75 cell lines expressing FN-tankyrase 1 showed progressive telomere elongationCitation30 dependent on telomerase.Citation32 The rate of telomere elongation induced by tankyrase 1 was ∼48 bp per population doubling (PD).Citation30 A similar rate of elongation (∼35 bp per PD) had been observed with the TRF1 dominant-negative allele.Citation28 Overexpression of TIN2 had limited effect on telomere length, but TIN2 depletion (that led to loss of TRF1 from telomeresCitation33 and TRF1 degradation by the proteasomeCitation34) resulted in progressive telomere elongation at a similar rate (∼50 bp per PD)Citation33 to tankyrase 1 overexpression and TRF1 inhibition. Hence, these data fit with the model of TRF1 as a negative regulator of telomere length, whose removal leads to telomere elongation by telomerase.
However, there was an N-terminally truncated allele of TIN2 (TIN2–13) whose behavior was not easily reconciled with the model above. This allele contained the TRF1 binding site but lacked the (yet-to-be-identified) TPP1 and TRF2 binding sites (see ). Overexpression of TIN2–13 led to telomere lengthening dependent on telomerase,Citation20 but the mechanism was unknown. To gain insight into the mechanism, we generated a similar allele (termed TIN2-C) that, as expected, led to telomere lengthening.Citation35 However, when we compared TIN2-C side-by-side with FN-tankyrase 1, we noticed that the telomere lengthening with TIN2-C was more dramatic (∼156 bp per PD) than telomere lengthening by tankyrase 1 (∼44 bp per PD) (). Moreover, unlike tankyrase 1 (or the TRF1 dominantnegative allele,Citation28 or TIN2 depletionCitation33), TIN2-C did not lead to loss of TRF1 from telomeres. In fact, immunoblot () and immunofluorescence () analysis revealed a slight increase in TRF1 levels and TRF1 at telomeres in TIN2-C-overexpressing cells. We reasoned that TIN2-C might bind a novel factor that stimulated telomere elongation in a unique way. A two-hybrid screen identified heterochromatin protein 1γ as a TIN2-C binding partner.Citation35
A role for HP1γ in telomere elongation.
HP1 proteins are highly conserved non-histone chromosomal proteins that contain a chromodomain (CD) that binds to trimethylated lysine 9 of histone H3 (H3K9Me3) in heterochromatinCitation36,Citation37 and a chromo shadow domain (CSD) that binds with high affinity to proteins containing the consensus pentapeptide PXVXL.Citation38,Citation39 We found that TIN2 contained a canonical HP1 binding site (PTVML), and mutation of this site to RTDML (TIN2.RD) abrogated binding to HP1. While overexpression of TIN2 did not affect telomere length maintenance, overexpression of TIN2.RD led to telomere shortening. Moreover, the RD mutation in the context of the TIN2-C allele (TIN2-C.RD) abrogated telomere lengthening, indicating that HP1 binding to TIN2 was required for telomere length maintenance.Citation35 To determine if the telomere shortening observed with the RD mutations was due to interference with telomerase rather than enhancement of telomere shortening, we generated stable cell lines overexpressing TIN2.RD and TIN2-C.RD in normal human fibroblasts (IMR90) that lack telomerase (). We grew the lines for multiple population doublings and analyzed telomere length. As shown in , TIN2. RD and TIN2-C.RD did not induce telomere shortening compared with the vector control, indicating that the telomere shortening in HTC75 tumor cells (described above) was due to interference with telomerase.
Together, these data indicated that the HP1 binding site in TIN2 was required for telomere length maintenance by telomerase, but the mechanism was not apparent. HP1 is typically associated with repressive effects in heterochromatin. Hence, one might imagine that HP1 binding could block access to telomerase, not promote it, as appeared to be the case. A clue came in 2008 when TIN2 was found mutated in dyskeratosis congenita.Citation40,Citation41 The TIN2 mutations were clustered in an area of unknown function C-terminal to the TRF1 binding domain. Remarkably, the HP1 PTVML binding site was embedded in the TIN2-DC mutation cluster. We found that the TIN2-DC mutations that were associated with severe telomere shortening and abrogated binding of TIN2 to HP1,Citation35 suggesting that HP1 binding was important for telomere elongation in humans, but how did it work?
TIN2, HP1 and Sister Telomere Cohesion
Our studies indicated that TIN2 could influence telomere length by a unique mechanism, distinct from the TRF1-controlled negative feedback loop. Hence we looked to other aspects of TIN2 function for clues. We showed previously that TIN2 was required to establish/maintain cohesion between sister telomeres in S phase.Citation42 Cohesion between sister chromatids is mediated by cohesin, a ring structure containing Smc1, Smc3 and Scc1 and peripheral protein Scc3,Citation43,Citation44 that exists as two related homologs (SA1 and SA2).Citation45,Citation46 Cohesin complexes are found with either SA2 (abundant) or SA1 (minor).Citation47 We identified a unique complex comprised of SA1-cohesin bound to the shelterin subunits TIN2 and TRF1 Citation34 and showed that cells depleted of TIN2 (or SA1) were unable to establish telomere cohesion in S phase.Citation42 We thus asked if HP1γ was required for telomere cohesion. We found that depletion of HP1γ led to loss of cohesion at telomeres, and that overexpression of TIN2 with a mutated HP1 binding site (TIN2.RD) led to loss of cohesion at telomeres. Moreover, we showed that HP1γ localized to telomeres in early S phase during replication, coincident with the timing of cohesion establishment.Citation35 We speculate that HP1 acts as a crosslinker in telomeric heterochromatin by binding to TIN2 via its chromo shadow domain and to H3K9Me3 via its chromodomain to hold sisters together (see ).
TIN2, HP1 and Telomere Elongation by Telomerase
But the question still remains, how does sister telomere cohesion impact telomere lengthening by telomerase? Again, we looked to the TIN2-C allele. We wondered why this allele (that consisted essentially of a TRF1 and a HP1 binding site) was so effective at inducing telomere elongation. The allele lacked the TPP1 and TRF2 binding sites (see ), but this could not account for telomere elongation, since TIN2-C.RD also lacked these binding sites but did not induce telomere elongation.Citation35 Hence, we looked to cohesion. We measured telomere cohesion and found that telomeres were super cohered in TIN2-C but not in TIN2-C.RD cells. We speculate that overexpression of TIN2-C leads to increased binding sites for HP1 at telomeres, leading to increased cohesion. Together, our data show a strong correlation between telomere cohesion status and telomere elongation by telomerase; increased cohesion increases telomere elongation by telomerase (TIN2-C), and decreased cohesion decreases telomere elongation by telomerase (TIN2.RD).
Our findings above prompted us to reexamine the telomere elongation phenotype of the TIN2-C allele. We noticed a faint smear below the elongated telomeres that became more apparent with a longer exposure (, left parts). Under the denaturing conditions used for telomere length analysis, the probe (in this case 32P-CCCTAA) hybridizes all along the length of the telomere; hence, the longer the telomere, the stronger the signal. When we quantified the signal for the long telomeres vs. the smear below and adjusted for telomere length (roughly 24 kb vs. 4 kb), the longer telomeres constituted only a fraction (23%) of the total. We observed this phenomenon in three independent derivations of TIN2-C cells, and we did not observe it with tankyrase 1 overexpression (). To confirm that, indeed, only a fraction of telomeres were elongated, we hybridized the 32P-CCCTAA probe prior to denaturation. Under these native conditions, where only the G-strand overhang is available for hybridization, we again observed that only a fraction of the telomeres (15%) were elongated; the bulk stayed the same or even shortened slightly (, right part). Keeping in mind that in tumor cells, catalytically active telomerase is present a low levelsCitation48 and is limiting for telomere length homeostasis,Citation49 we speculated that super cohered telomeres in TIN2-C cells may promote processivity of telomerase but at the expense of the other telomeres; i.e., once telomerase binds to the super cohered sisters, it stays on and is not available to elongate other telomeres. Alternatively, or in addition, loss of cohesion may be required to release telomerase from chromosome ends.
How might the super cohered sister chromatids influence telomere lengthening by telomerase? Studies have suggested that telomerase functions as a dimerCitation48,Citation50,Citation51 with two molecules of hTERT and two molecules of hTR (). A dimer with two active sites could provide a mechanism for coordinated extension of the 3′ ends of newly replicated sister telomeres ( and D). Cohered sisters may be a preferred substrate for a dimeric telomerase enzyme, rendering it more efficient and/or processive. Techniques have recently been developed that allow investigation of the mode of action (processive or distributive) of telomerase during a single cell cycle.Citation52–Citation54 These studies suggest that in human cancer cells, telomerase extends most telomeres by about 60 nt, and that each end is extended processively by one molecule of telomerase. Using this type of approach, we should be able to determine how telomere cohesion influences telomere elongation by telomerase. We predict that increased cohesion (TIN2-C) will increase telomerase processivity, whereas loss of cohesion (TIN2. RD) will reduce it.
Telomere Cohesion, Telomere Elongation and Dyskeratosis Congenita
Our studies showed that TIN2 harbored a binding site for HP1 and that mutations in this site led to loss in sister telomere cohesion in S phase and interfered with telomere length maintenance by telomerase.Citation35 The HP1 binding site was embedded in the TIN2-DC mutation cluster. We further showed that human patient cells harboring TIN2 mutations were defective in sister telomere cohesion.Citation35 Patients with TIN2 mutations have severe manifestations of DC and extremely short telomeres, shorter than some of the other DC subtypes.Citation40,Citation41,Citation55,Citation56 Indeed, while inheritance of (heterozygous) telomerase subunit mutations results in progressive telomere shortening over successive generations (anticipation),Citation57,Citation58 TIN2 mutations are most often de novo and result in dramatic telomere shortening in the first generation at a young age. This distinction suggests that in the case of the TIN2-DC mutations, severe telomere dysfunction may occur during embryogenesis. We speculate that non-cohered telomeres are not efficiently elongated by telomerase during embryogenesis, thereby contributing to the extreme telomere shortening observed in TIN2-associated DC.
Recent studies have use induced pluripotent stem (iPS) cells to probe the disease mechanism of DC. During reprogramming, TERT is activated and telomerase activity is reconstituted.Citation59,Citation60 However, this may not be sufficient to rescue all classes of DC patient cells. For example, iPS cell reprogramming of DC patient cells harboring a mutation in TCAB1, a telomerase-associated protein that facilitates trafficking of telomerase to Cajal bodies,Citation61–Citation63 showed that despite wild-type telomerase activity, the ability of telomerase to elongate telomeres was abrogated, because telomerase was mislocalized.Citation64 Along these lines, it will be interesting to determine if iPS cell reprogramming of DC patient cells harboring mutations in TIN2 will rescue telomere shortening.
Figures and Tables
Figure 1 TIN2-C and FN-tankyrase 1 induce telomere lengthening by different mechanisms. (A) Schematic diagrams of TRF1 and its binding partners, tankyrase 1 (TNKS1) and TIN2. (B) Graphical representation of telomere length changes in stable HTC75 cell lines overexpressing TIN2-C, FNtankyrase 1 (TNKS1), or vector. (C) Immunoblot analysis of the stable lines probed with antibodies against TNKS1, TIN2, TRF1 or TRF2. (D) Immunofluorescence analysis of transiently transfected HeLaI.2.11 cells costained with antibodies against Flag (green) and TRF1 (red) and DAPI (blue).
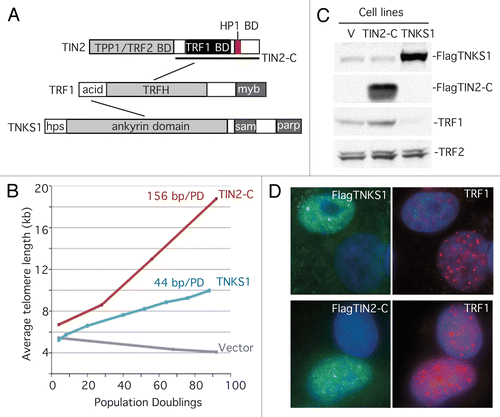
Figure 2 Telomere shortening by the TIN2.RD mutation depends on telomerase. (A) Immunoblot analysis of IMR90 stable cell lines expressing TIN2. RD, TIN2-C.RD and vector, probed with antibodies against TIN2 or α-tubulin. (B) Analysis of telomere restriction fragments isolated from the stable IMR90 cell lines at the indicated PD, fractionated on agarose gel, denatured and probed with a 32P-labeled CCCTAA probe.
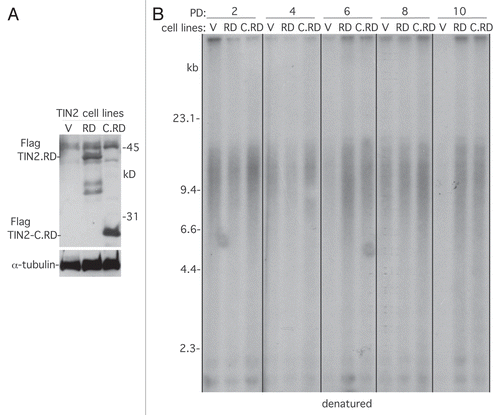
Figure 3 All telomeres are not elongated in TIN2-C cells. (A and B) Analysis of telomere restriction fragments isolated from the stable HTC75 cell lines expressing TIN2-C (A) or FN-tankyrase 1 (TNKS1) (B) at the indicated PD. Samples were fractionated on agarose gel and probed with a 32P-labeled CCCTAA probe under native and denatured conditions.
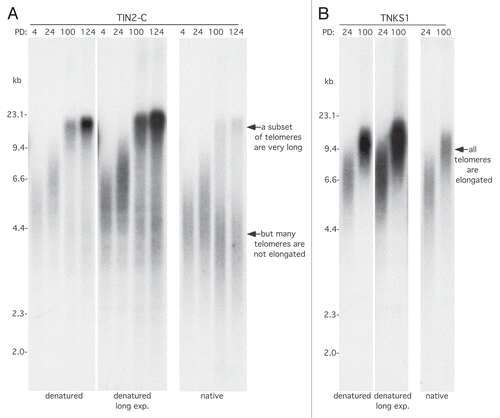
Figure 4 Schematic diagram showing how sister telomere cohesion could influence telomere elongation by telomerase. (A) HP1 promotes cohesion between sister telomeres by binding to TIN2 via its chromo shadow domain (CSD) and to H3K9Me3 via its chromodomain (CD). (B) Telomerase as a dimer with two molecules of TERT and two of the RNA (TR). (C) In TIN2.RD mutant cells, HP1 cannot bind to TIN2.RD, and cohesion is not established. Dimeric telomerase cannot efficiently elongate single sisters, and telomeres shorten. (D) In TIN2-C cells, HP1 has increased binding sites to TIN2, leading to super cohesion. Dimeric telomerase efficiently elongates cohered sisters, and telomeres are super elongated.
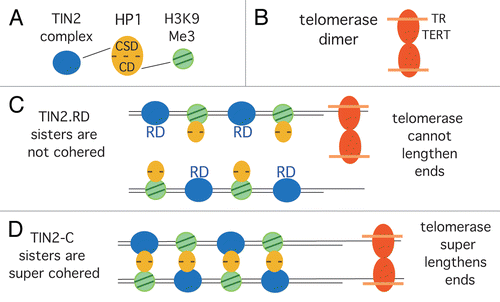
Acknowledgments
We are grateful to Tom Meier for comments on the manuscript. This work was supported by NIH Grant R01 CA116352 to S.S. S.C. was the 2010 Helen and Martin Kimmel Senior Fellow in Stem Cell Biology.
References
- de Lange T. Shelterin: the protein complex that shapes and safeguards human telomeres. Genes Dev 2005; 19:2100 - 2110; PMID: 16166375; http://dx.doi.org/10.1101/gad.1346005
- Harley CB, Futcher AB, Greider CW. Telomeres shorten during ageing of human fibroblasts. Nature 1990; 345:458 - 460; PMID: 2342578; http://dx.doi.org/10.1038/345458a0
- Hastie ND, Dempster M, Dunlop MG, Thompson AM, Green DK, Allshire RC. Telomere reduction in human colorectal carcinoma and with ageing. Nature 1990; 346:866 - 868; PMID: 2392154; http://dx.doi.org/10.1038/346866a0
- Greider CW, Blackburn EH. Identification of a specific telomere terminal transferase activity in Tetrahymena extracts. Cell 1985; 43:405 - 413; PMID: 3907856; http://dx.doi.org/10.1016/0092-8674(85)90170-9
- Greider CW, Blackburn EH. The telomere terminal transferase of Tetrahymena is a ribonucleoprotein enzyme with two kinds of primer specificity. Cell 1987; 51:887 - 898; PMID: 3319189; http://dx.doi.org/10.1016/0092-8674(87)90576-9
- Wright WE, Piatyszek MA, Rainey WR, Byrd W, Shay JW. Telomerase activity in human germline and embryonic tissues and cells. Dev Genet 1996; 18:173 - 179; PMID: 8934879; http://dx.doi.org/10.1002/(SICI)1520-6408(1996)18:2<173::AIDDVG10>3.0.CO;2-3
- Bessler M, Wilson DB, Mason PJ. Dyskeratosis congenita. FEBS Lett 2010; 584:3831 - 3838; PMID: 20493861; http://dx.doi.org/10.1016/j.febslet.2010.05.019
- Alter BP, Baerlocher GM, Savage SA, Chanock SJ, Weksler BB, Willner JP, et al. Very short telomere length by flow fluorescence in situ hybridization identifies patients with dyskeratosis congenita. Blood 2007; 110:1439 - 1447; PMID: 17468339; http://dx.doi.org/10.1182/blood-2007-02-075598
- Gadalla SM, Cawthon R, Giri N, Alter BP, Savage SA. Telomere length in blood, buccal cells, and fibroblasts from patients with inherited bone marrow failure syndromes. Aging (Albany NY) 2010; 2:867 - 874; PMID: 21113082
- Kim NW, Piatyszek MA, Prowse KR, Harley CB, West MD, Ho PL, et al. Specific association of human telomerase activity with immortal cells and cancer [see comments]. Science 1994; 266:2011 - 2015; PMID: 7605428; http://dx.doi.org/10.1126/science.7605428
- Meyerson M, Counter CM, Eaton EN, Ellisen LW, Steiner P, Caddle SD, et al. hEST2, the putative human telomerase catalytic subunit gene, is upregulated in tumor cells and during immortalization. Cell 1997; 90:785 - 795; PMID: 9288757; http://dx.doi.org/10.1016/S0092-8674(00)80538-3
- Nakamura TM, Morin GB, Chapman KB, Weinrich SL, Andrews WH, Lingner J, et al. Telomerase catalytic subunit homologs from fission yeast and human. Science 1997; 277:955 - 959; PMID: 9252327; http://dx.doi.org/10.1126/science.277.5328.955
- Shay JW, Bacchetti S. A survey of telomerase activity in human cancer. Eur J Cancer 1997; 33:787 - 791; PMID: 9282118; http://dx.doi.org/10.1016/S0959-8049(97)00062-2
- Hahn WC, Stewart SA, Brooks MW, York SG, Eaton E, Kurachi A, et al. Inhibition of telomerase limits the growth of human cancer cells [see comments]. Nat Med 1999; 5:1164 - 1170; PMID: 10502820; http://dx.doi.org/10.1038/13495
- Zhang X, Mar V, Zhou W, Harrington L, Robinson MO. Telomere shortening and apoptosis in telomerase-inhibited human tumor cells. Genes Dev 1999; 13:2388 - 2399; PMID: 10500096; http://dx.doi.org/10.1101/gad.13.18.2388
- Smogorzewska A, de Lange T. Regulation of Telomerase by Telomeric Proteins. Annu Rev Biochem 2004; 73:177 - 208; PMID: 15189140
- Chong L, van Steensel B, Broccoli D, Erdjument-Bromage H, Hanish J, Tempst P, et al. A human telomeric protein. Science 1995; 270:1663 - 1667; PMID: 7502076; http://dx.doi.org/10.1126/science.270.5242.1663
- Bilaud T, Brun C, Ancelin K, Koering CE, Laroche T, Gilson E. Telomeric localization of TRF2, a novel human telobox protein. Nat Genet 1997; 17:236 - 239; PMID: 9326951; http://dx.doi.org/10.1038/ng1097-236
- Broccoli D, Smogorzewska A, Chong L, de Lange T. Human telomeres contain two distinct Myb-related proteins, TRF1 and TRF2. Nat Genet 1997; 17:231 - 235; PMID: 9326950; http://dx.doi.org/10.1038/ng1097-231
- Kim SH, Kaminker P, Campisi J. TIN2, a new regulator of telomere length in human cells [see comments]. Nat Genet 1999; 23:405 - 412; PMID: 10581025; http://dx.doi.org/10.1038/13854
- Houghtaling BR, Cuttonaro L, Chang W, Smith S. A Dynamic Molecular Link between the Telomere Length Regulator TRF1 and the Chromosome End Protector TRF2. Curr Biol 2004; 14:1621 - 1631; PMID: 15380063; http://dx.doi.org/10.1016/j.cub.2004.08.052
- Liu D, Safari A, O'Connor MS, Chan DW, Laegeler A, Qin J, et al. PTOP interacts with POT1 and regulates its localization to telomeres. Nat Cell Biol 2004; 6:673 - 680; PMID: 15181449; http://dx.doi.org/10.1038/ncb1142
- Ye JZ, Hockemeyer D, Krutchinsky AN, Loayza D, Hooper SM, Chait BT, et al. POT1-interacting protein PIP1: a telomere length regulator that recruits POT1 to the TIN2/TRF1 complex. Genes Dev 2004; 18:1649 - 1654; PMID: 15231715; http://dx.doi.org/10.1101/gad.1215404
- Baumann P, Cech TR. Pot1, the putative telomere end-binding protein in fission yeast and humans. Science 2001; 292:1171 - 1175; PMID: 11349150; http://dx.doi.org/10.1126/science.1060036
- Loayza D, de Lange T. POT1 as a terminal transducer of TRF1 telomere length control. Nature 2003; 423:1013 - 1018; PMID: 12768206; http://dx.doi.org/10.1038/nature01688
- Li B, Oestreich S, de Lange T. Identification of human Rap1: implications for telomere evolution. Cell 2000; 101:471 - 483; PMID: 10850490; http://dx.doi.org/10.1016/S0092-8674(00)80858-2
- Ancelin K, Brunori M, Bauwens S, Koering CE, Brun C, Ricoul M, et al. Targeting assay to study the cis functions of human telomeric proteins: evidence for inhibition of telomerase by TRF1 and for activation of telomere degradation by TRF2. Mol Cell Biol 2002; 22:3474 - 3487; PMID: 11971978; http://dx.doi.org/10.1128/MCB.22.10.3474-87.2002
- van Steensel B, de Lange T. Control of telomere length by the human telomeric protein TRF1. Nature 1997; 385:740 - 743; PMID: 9034193; http://dx.doi.org/10.1038/385740a0
- Smith S, Giriat I, Schmitt A, de Lange T. Tankyrase, a poly(ADP-ribose) polymerase at human telomeres [see comments]. Science 1998; 282:1484 - 1487; PMID: 9822378; http://dx.doi.org/10.1126/science.282.5393.1484
- Smith S, de Lange T. Tankyrase promotes telomere elongation in human cells. Curr Biol 2000; 10:1299 - 1302; PMID: 11069113; http://dx.doi.org/10.1016/S0960-9822(00)00752-1
- Chang W, Dynek JN, Smith S. TRF1 is degraded by ubiquitin-mediated proteolysis after release from telomeres. Genes Dev 2003; 17:1328 - 1333; PMID: 12782650; http://dx.doi.org/10.1101/gad.1077103
- Cook BD, Dynek JN, Chang W, Shostak G, Smith S. Role for the related poly(ADP-Ribose) polymerases tankyrase 1 and 2 at human telomeres. Mol Cell Biol 2002; 22:332 - 342; PMID: 11739745; http://dx.doi.org/10.1128/MCB.22.1.332-42.2002
- Ye JZ, de Lange T. TIN2 is a tankyrase 1 PARP modulator in the TRF1 telomere length control complex. Nat Genet 2004; 36:618 - 623; PMID: 15133513; http://dx.doi.org/10.1038/ng1360
- Canudas S, Houghtaling BR, Kim JY, Dynek JN, Chang WG, Smith S. Protein requirements for sister telomere association in human cells. EMBO J 2007; 26:4867 - 4878; PMID: 17962804; http://dx.doi.org/10.1038/sj.emboj.7601903
- Canudas S, Houghtaling BR, Bhanot M, Sasa G, Savage SA, Bertuch AA, et al. A role for heterochromatin protein 1{gamma} at human telomeres. Genes Dev 2011; 25:1807 - 1819; PMID: 21865325; http://dx.doi.org/10.1101/gad.17325211
- Bannister AJ, Zegerman P, Partridge JF, Miska EA, Thomas JO, Allshire RC, et al. Selective recognition of methylated lysine 9 on histone H3 by the HP1 chromo domain. Nature 2001; 410:120 - 124; PMID: 11242054; http://dx.doi.org/10.1038/35065138
- Lachner M, O'Carroll D, Rea S, Mechtler K, Jenuwein T. Methylation of histone H3 lysine 9 creates a binding site for HP1 proteins. Nature 2001; 410:116 - 120; PMID: 11242053; http://dx.doi.org/10.1038/35065132
- Brasher SV, Smith BO, Fogh RH, Nietlispach D, Thiru A, Nielsen PR, et al. The structure of mouse HP1 suggests a unique mode of single peptide recognition by the shadow chromo domain dimer. EMBO J 2000; 19:1587 - 1597; PMID: 10747027; http://dx.doi.org/10.1093/emboj/19.7.1587
- Smothers JF, Henikoff S. The HP1 chromo shadow domain binds a consensus peptide pentamer. Curr Biol 2000; 10:27 - 30; PMID: 10660299; http://dx.doi.org/10.1016/S0960-9822(99)00260-2
- Savage SA, Giri N, Baerlocher GM, Orr N, Lansdorp PM, Alter BP. TINF2, a component of the shelterin telomere protection complex, is mutated in dyskeratosis congenita. Am J Hum Genet 2008; 82:501 - 509; PMID: 18252230; http://dx.doi.org/10.1016/j.ajhg.2007.10.004
- Walne AJ, Vulliamy T, Beswick R, Kirwan M, Dokal I. TINF2 mutations result in very short telomeres: analysis of a large cohort of patients with dyskeratosis congenita and related bone marrow failure syndromes. Blood 2008; 112:3594 - 3600; PMID: 18669893; http://dx.doi.org/10.1182/blood-2008-05-153445
- Canudas S, Smith S. Differential regulation of telomere and centromere cohesion by the Scc3 homologues SA1 and SA2, respectively, in human cells. J Cell Biol 2009; 187:165 - 173; PMID: 19822671; http://dx.doi.org/10.1083/jcb.200903096
- Anderson DE, Losada A, Erickson HP, Hirano T. Condensin and cohesin display different arm conformations with characteristic hinge angles. J Cell Biol 2002; 156:419 - 424; PMID: 11815634; http://dx.doi.org/10.1083/jcb.200111002
- Haering CH, Lowe J, Hochwagen A, Nasmyth K. Molecular architecture of SMC proteins and the yeast cohesin complex. Mol Cell 2002; 9:773 - 788; PMID: 11983169; http://dx.doi.org/10.1016/S1097-2765(02)00515-4
- Losada A, Yokochi T, Kobayashi R, Hirano T. Identification and characterization of SA/Scc3p subunits in the Xenopus and human cohesin complexes. J Cell Biol 2000; 150:405 - 416; PMID: 10931856; http://dx.doi.org/10.1083/jcb.150.3.405
- Sumara I, Vorlaufer E, Stukenberg PT, Kelm O, Redemann N, Nigg EA, et al. The dissociation of cohesin from chromosomes in prophase is regulated by Polo-like kinase. Mol Cell 2002; 9:515 - 525; PMID: 11931760; http://dx.doi.org/10.1016/S1097-2765(02)00473-2
- Holzmann J, Fuchs J, Pichler P, Peters JM, Mechtler K. Lesson from the stoichiometry determination of the cohesin complex: a short protease mediated elution increases the recovery from crosslinked antibody-conjugated beads. J Proteome Res 2011; 10:780 - 789; PMID: 21043528; http://dx.doi.org/10.1021/pr100927x
- Cohen SB, Graham ME, Lovrecz GO, Bache N, Robinson PJ, Reddel RR. Protein composition of catalytically active human telomerase from immortal cells. Science 2007; 315:1850 - 1853; PMID: 17395830; http://dx.doi.org/10.1126/science.1138596
- Cristofari G, Lingner J. Telomere length homeostasis requires that telomerase levels are limiting. EMBO J 2006; 25:565 - 574; PMID: 16424902; http://dx.doi.org/10.1038/sj.emboj.7600952
- Prescott J, Blackburn EH. Functionally interacting telomerase RNAs in the yeast telomerase complex. Genes Dev 1997; 11:2790 - 2800; PMID: 9353249; http://dx.doi.org/10.1101/gad.11.21.2790
- Wenz C, Enenkel B, Amacker M, Kelleher C, Damm K, Lingner J. Human telomerase contains two cooperating telomerase RNA molecules. EMBO J 2001; 20:3526 - 3534; PMID: 11432839; http://dx.doi.org/10.1093/emboj/20.13.3526
- Giraud-Panis MJ, Teixeira MT, Geli V, Gilson E. CST meets shelterin to keep telomeres in check. Mol Cell 2010; 39:665 - 676; PMID: 20832719; http://dx.doi.org/10.1016/j.molcel.2010.08.024
- Zhao Y, Abreu E, Kim J, Stadler G, Eskiocak U, Terns MP, et al. Processive and Distributive Extension of Human Telomeres by Telomerase under Homeostatic and Nonequilibrium Conditions. Mol Cell 2011; 42:297 - 307; PMID: 21549308; http://dx.doi.org/10.1016/j.molcel.2011.03.020
- Zhao Y, Sfeir AJ, Zou Y, Buseman CM, Chow TT, Shay JW, et al. Telomere extension occurs at most chromosome ends and is uncoupled from fill-in in human cancer cells. Cell 2009; 138:463 - 475; PMID: 19665970; http://dx.doi.org/10.1016/j.cell.2009.05.026
- Sasa G, Ribes-Zamora A, Nelson N, Bertuch A. Three novel truncating TINF2 mutations causing severe dyskeratosis congenita in early childhood. Clin Genet 2011; In press
- Vulliamy T, Beswick R, Kirwan M, Hossain U, Walne A, Dokal I. Telomere length measurement can distinguish pathogenic from non-pathogenic variants in the shelterin component, TIN2. Clin Genet 2011; In press
- Vulliamy T, Marrone A, Szydlo R, Walne A, Mason PJ, Dokal I. Disease anticipation is associated with progressive telomere shortening in families with dyskeratosis congenita due to mutations in TERC. Nat Genet 2004; 36:447 - 449; PMID: 15098033; http://dx.doi.org/10.1038/ng1346
- Armanios M, Chen JL, Chang YP, Brodsky RA, Hawkins A, Griffin CA, et al. Haploinsufficiency of telomerase reverse transcriptase leads to anticipation in autosomal dominant dyskeratosis congenita. Proc Natl Acad Sci USA 2005; 102:15960 - 15964; PMID: 16247010; http://dx.doi.org/10.1073/pnas.0508124102
- Agarwal S, Loh YH, McLoughlin EM, Huang J, Park IH, Miller JD, et al. Telomere elongation in induced pluripotent stem cells from dyskeratosis congenita patients. Nature 2010; 464:292 - 296; PMID: 20164838; http://dx.doi.org/10.1038/nature08792
- Takahashi K, Tanabe K, Ohnuki M, Narita M, Ichisaka T, Tomoda K, et al. Induction of pluripotent stem cells from adult human fibroblasts by defined factors. Cell 2007; 131:861 - 872; PMID: 18035408; http://dx.doi.org/10.1016/j.cell.2007.11.019
- Venteicher AS, Abreu EB, Meng Z, McCann KE, Terns RM, Veenstra TD, et al. A human telomerase holoenzyme protein required for Cajal body localization and telomere synthesis. Science 2009; 323:644 - 648; PMID: 19179534; http://dx.doi.org/10.1126/science.1165357
- Venteicher AS, Artandi SE. TCAB1: driving telomerase to Cajal bodies. Cell Cycle 2009; 8:1329 - 1331; PMID: 19342896; http://dx.doi.org/10.4161/cc.8.9.8288
- Zhong F, Savage SA, Shkreli M, Giri N, Jessop L, Myers T, et al. Disruption of telomerase trafficking by TCAB1 mutation causes dyskeratosis congenita. Genes Dev 2011; 25:11 - 16; PMID: 21205863; http://dx.doi.org/10.1101/gad.2006411
- Batista LF, Pech MF, Zhong FL, Nguyen HN, Xie KT, Zaug AJ, et al. Telomere shortening and loss of self-renewal in dyskeratosis congenita induced pluripotent stem cells. Nature 2011; 474:399 - 402; PMID: 21602826; http://dx.doi.org/10.1038/nature10084