Abstract
Analysis of microarrays performed in p53-, TAp63α- and ΔNp63α-inducible SaOs-2 cell lines allowed the identification of NCF2 mRNA upregulation in response to p53 induction. NCF2 gene encodes for p67phox, the cytosolic subunit of the NADPH oxidase enzyme complex. The recruitment of p67phox to the cell membrane causes the activation of the NADPH oxidase complex followed by the generation of NADP+ and superoxide from molecular oxygen. The presence of three putative p53 binding sites on the NCF2 promoter was predicted, and the subsequent luciferase and chromatin immunoprecipitation assays showed the activation of NCF2 promoter by p53 and its direct binding in vivo to at least one of the sites, thus confirming the hypothesis. NCF2 upregulation was also confirmed by real-time PCR in several cell lines after p53 activation. NCF2 knockdown by siRNA results in a significant reduction of ROS production and stimulates cell death, suggesting a protective function of Nox2-generated ROS in cells against apoptosis. These results provide insight into the redox-sensitive signaling mechanism that mediates cell survival involving p53 and its novel target NCF2/p67phox.
Introduction
The family of nicotinamide adenine dinucleotide phosphate (NADPH) oxidase was originally detected in neutrophils as a multicomponent enzyme that catalyzes monoelectronic reduction of external oxygen using NADPH or NADH as an internal electron donor, thus producing superoxide anions and NADP+ or NAD+.Citation1 Currently, the family is formed by seven members, Nox1–5 and Duox1/2, which are now recognized to have specific cellular and subcellular localizations, thus serving a variety of biological functions, including signal transduction, host defense, development, angiogenesis, blood pressure regulation and biosynthetic processes.Citation2-Citation6 The most extensively studied member is Nox2; this enzyme is composed of two membrane-bound subunits (p22phox and gp91phox), three cytosolic subunits (p67phox, p47phox and p40phox) and a small G-protein Rac (Rac1 and Rac2). Nox2 becomes activated during phagocytosis of invading microbes as a result of membrane translocation of a ternary regulatory complex, formed by p47phox, p67phox and p40phox subunits, as well as of the small GTPase Rac.Citation7 The p47phox organizer protein contains two SH3 domains, which cooperatively interact with the proline-rich-domain of p22phox, thus promoting membrane translocation and oxidase activation.Citation8 In normal conditions, the two SH3 domains are masked by intramolecular interaction with an auto-inhibitory region. Upon stimulation, serine residues are unmasked by phosphorylation. The p67phox, encoded by the NCF2 gene, is the “activator” of the Nox2 complex. p67phox contains four conserved domains: a C-terminal SH3 domain, which mediates membrane translocation through binding to the proline-rich region present in the p47phox tail; four tetratricopeptide repeat motifs at the N-terminal domain interacting with the Rac; an activation domain able to act on gp91phox and a domain interacting with p40phox (PB1).Citation9,Citation10 The PB1 domain is dispensable for oxidase activation, but is necessary for oxidase assembly, as it enhances p67phox recruitment to the phagosomal membrane.
Nox2 signaling has been extensively studied, given its physiological relevance in immune defense.Citation6 Along with its well-established role in immune function, Nox2 has also been shown to be activated in endothelial cells by several stimuli, including vascular endothelial growth factor and thrombin, thus implicating Nox2 in new blood vessel formation.Citation11-Citation13 Recently, Nox2 and other Nox isoforms have been shown to be involved in tumor cell proliferation.Citation14-Citation18 The upregulation of Nox is critical to support the elevated glycolysis by providing additional NAD+, and it has been consistently observed in cancer cells and in primary pancreatic cancer tissues with compromised mitochondria.Citation9,Citation19 In addition, in acute leukemic cell lines, Nox2- and/or Nox4-derived ROS are crucially involved in the modulation of glucose transport (mediated by Glut1), which is frequently upregulated in cancer cells, suggesting that both Glut1 and Nox homologs may be considered new potential targets in the treatment of leukemia.Citation16 In parallel with these studies, other results support the finding that ROS-generating Nox family enzymes are involved in neoplastic formation. For instance, Nox1 downregulation suppresses oncogenic Ras transformation,Citation20 and Nox4/Nox5 appear to control cell survival of malignant melanoma, pancreatic carcinoma and prostate cancer cells.Citation21-Citation25 These data suggest that the modulation of survival signaling by ROS is also critical for some types of cancer development, although the genotoxic effect has been mainly emphasized for the role of ROS in tumor formations.Citation26-Citation30 In pancreatic cancer, instead, Nox4-generated ROS have a protective function against apoptosis through the inhibition of AKT-ASK1 phosphorylation signaling.Citation22,Citation23,Citation28
ROS are even generated downstream of p53 and p53 family members, p63 and p73, most likely by the transcriptional modulation of genes that regulate the cellular redox state and that directly contribute to p53/p63/p73-mediated cell death.Citation31-Citation36 To maintain the cellular redox state, ROS levels need to be tightly controlled, a task which is performed by two interconnected systems: thioredoxin and glutathione system.Citation37-Citation41 Interestingly, p53 and its family members positively regulate expression of genes whose products are directly involved in both systems; therefore, p53 clearly acts in an anti-apoptotic manner.Citation42-Citation46 The list of p53 target genes includes the glutathione peroxidase (GPX), manganese superoxide dismutase (MnSOD, SOD2), aldehyde dehydrogenase 4 (ALDH4), p53-induced glycolysis and apoptosis regulator (TIGAR), as well as PA26 and Hi95, which encode two proteins of the sestrin family, namely sestrin1 (SESN1) and sestrin 2 (SESN2), respectively.Citation47-Citation49
In the present study, we identified NCF2, the gene encoding for p67phox, the cytosolic subunit of the NADPH oxidase enzyme complex, as direct p53 target gene. We demonstrated that NCF2 downregulation by siRNA implicates a significant reduction in cellular ROS production that stimulates apoptosis, suggesting a protective function of Nox2-generated ROS in cells against apoptosis. Here, we provide insight into the redox-sensitive signaling mechanism that mediates cell survival involving p53 and its novel target NCF2/p67phox.
Results
NCF2 expression and p53 family members
To characterize how downstream functions of the p53 family members are mediated, we performed a microarray analysis to identify genes that are differentially regulated by the TAp63α and ΔNp63α isoforms of p63 compared with p53.Citation50 We focused our attention on the NCF2 gene encoding for p67phox protein. Following upregulation of p53 expression by doxicycline addiction in a Tet-On-inducible SaOs2 cell line, the gene NCF2 was found upregulated (14-fold over control), while no upregulation was observed upon TAp63α and ΔNp63α induction (). As positive control, the data related to p21 are also shown (). In order to verify the data obtained by the array, we transiently transfected H1299 cell line with expression vectors bearing the p53, TAp63α and ΔNp63αHA-tagged cDNAs. Twenty-four hours post-transfection, NCF2 levels were greatly upregulated, as evaluated by real-time PCR, in p53-expressing cells as compared with TAp63α- and ΔNp63α-expressing cells(). p21 expression levels are also shown as positive controls (). These data indicate that p53 possibly regulates NCF2 expression in two different cellular systems.
Figure 1. mRNA expression of NCF2/p67phox in SaOs-2 and in H1299 by p53 family members. (A) Microarray in SaOs-2 inducible clones for each transcription factor. Expression of NCF2/p67phox was measured 24 h after induction with doxycycline. p21 is used as a positive control. (B) Real-time PCR analysis of NCF2/p67phox expression following transfection of p53, ΔN or Tap63 in non-small cell lung carcinoma H1299 cells. p21 is used as a positive control.
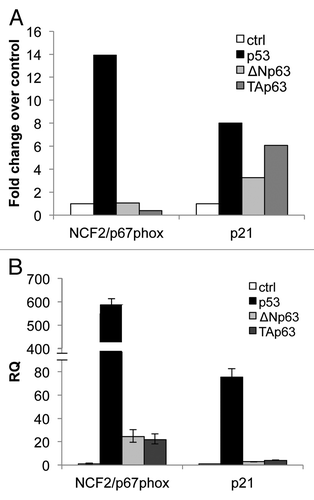
NCF2 is a direct transcriptional target of p53
Based on the observations described above, we investigated the possibility that p53 directly transactivates the NCF2 gene. By using bioinformatics tools (MatInspector Professional softwareCitation51), we searched the promoter region of human NCF2 for putative p53-responsive elements (REs). We identified three p53 consensus motifs (RE1, RE2 and RE3), which contained the core sequence CWWG (). A luciferase reporter vector containing the promoter region,Citation52 with the three REs (RE1, RE2 and RE3) to control the expression of a luciferase cDNA, was used in co-transfection with expression vectors for different p53/p63/p73 isoforms (). Twenty-four hours after co-transfection of HEK293 cells, luciferase assays were performed. p53 overexpression significantly increased luciferase activity (10.2-fold activation over control), TAp73α also produced an enhancement of NCF2 promoter activity although to a minor extent (4.0-fold activation over control); the other members of p53 family did not exert a positive control on NCF2 promoter (). As control, using the same cell extracts of the luciferase assay, we performed a western blot to show that the absence of luciferase upregulation is not due to evident differences in the expression level of the different transcription factors (). This strongly argues in favor of the conclusion that the NCF2 gene is a direct p53 transcriptional target. Direct evidence has been found by chromatin immunoprecipitation (ChIP) experiments followed by PCR amplification of REs genomic fragments. clearly shows the ability of p53 protein to bind directly only the p53 RE2 of the NCF2 promoter sequence.
Figure 2. p53 transcriptionally activates NCF2/p67phox. (A) Map of the human NCF2/p67phox promoter. The promoter possesses three putative p53-responsive elements as indicated by the boxes. (B) p53 induces NCF2/p67phox promoter activity. HEK293 cells were transfected with pGL3-p67phox and the transactivators cloned in pcDNA vectors to evaluate the promoter activity by luciferase assay. Results are shown as the mean of three independent experiments. (C) Following transfection, HEK293 cells were lysed, and a western blot was performed using an anti-HA antibody to verify the transcription factors expression. The figure shows a representative experiment. (D) p53 binds to NCF2/p67phox promoter on RE2. Chromatin immunoprecipitation was performed in p53-inducible SaOs-2 cells using an anti-p53 antibody (IP p53) or a non-specific serum IgG. Non-immunoprecipitated chromatin was loaded as a positive control (input).The figure shows one representative experiment of three.
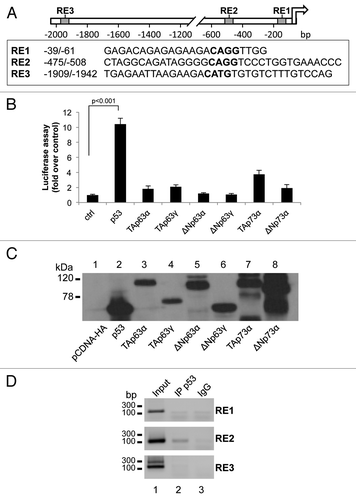
To further demonstrate a direct link between p53 activation and NCF2/p67phox expression, we treated HCT116 p53+/+ or HCT116 p53−/− cell lines with doxorubicin (1 μM, for 24 h). We observed an increase in NCF2 expression (13-fold over control), evaluated by real-time PCR, only in HCT116 p53+/+ cells (). As a positive control, we evaluated using the same experimental conditions p21 expression ().
Figure 3. Endogenous-activated p53 induces NCF2/p67phox expression. (A) Colorectal carcinoma HCT116 cells, available in both p53 negative or positive clones, were treated with doxorubicin 1 μM to induce activation of p53. Cells were collected 24 h after treatment and real-time PCR was performed. (B) p21 is used as a positive control. Results are shown as the mean of three independent experiments.
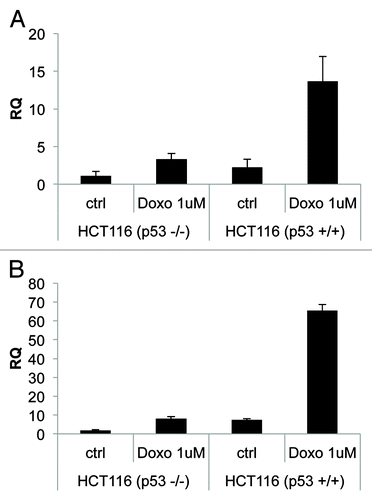
NCF2/p67phox-mediated ROS production in HCT116 and in HaCat cell lines
Data previously published point out that the activity of the Nox enzyme families is critical for survival in cancer cell lines.Citation22,Citation23 To investigate the role of NCF2/p67phox in cell signaling and survival, we took advantage of the loss-of-function analysis using siRNA. By transfection of specific NCF2 siRNA in HCT116 cells, we strongly suppressed its expression as evaluated at mRNA and at protein levels (). Intracellular superoxide production was evaluated by using flow cytometry in cells loaded with oxidation-sensitive 2’,7’-dichlorodihydrofluorescein diacetate (DCFH-DA). In normal growing conditions, HCT116 spontaneously generated a certain level of ROS; upon siNCF2, ROS production was significantly reduced (50% less), as compared with scramble control transfected cells (). This decrease indicates that NCF2/p67phox, and therefore Nox2, at least in part, is responsible for the intracellular ROS generation occurring in proliferating cells, and that other mechanisms also contributed to ROS synthesis. To demonstrate that this result is not cell line-specific, we performed the same experiment in HaCat cell line. HaCat cells were transiently transfected to obtain NCF2 silencing. As indicated in , we totally abrogated NCF2 expression as evaluated at mRNA and at protein levels. In this experimental condition, we evaluated ROS levels by DCFH-DA cell loading followed by flow cytometry. We found that ROS production was significantly reduced (80% less) as compared with scramble control transfected cells (), indicating that NCF2/p67phox is responsible for the majority of intracellular ROS generation in HaCat cells as well, suggesting that our observation is not cell line-specific.
Figure 4. Inhibition of NCF2/p67phox in HCT116 cells decreases ROS and induces apoptosis. (A) HCT116 cells were transfected with either a control siRNA (si-scr) or specific NCF2/p67phox-targeting siRNA (si-NCF2/p67phox) and collected after 48 h. NCF2/p67phox protein and transcript levels were examined by western blot and real-time PCR, respectively. The western blot shown is one representative experiment. Real-time PCR shows the mean of three independent experiments. Actin is shown as a loading control. (B) ROS levels were assayed using a DCFDA staining and FACS analysis. Left panel shows one representative experiment of four independent ones. Right panel shows the mean of four independent experiments as a percentage respect to the control (presented as 100%). (C) Apoptosis levels were assayed by propidium iodide staining and FACS analysis. Percentage of sub-G1 events (M1) is shown. Left panel shows one representative experiment of three. Right panel shows the mean of three independent experiments.
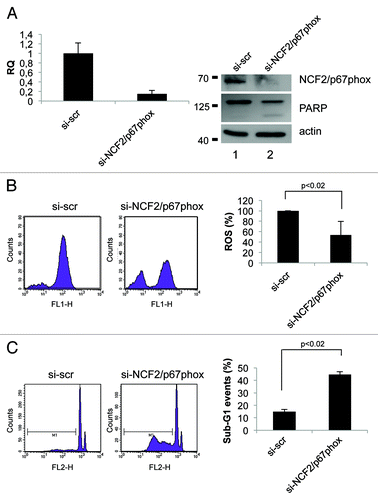
Figure 5. Inhibition of NCF2/p67phox in HaCat cells decreases ROS and induces apoptosis. (A) HaCat cells were transfected with either a control siRNA (si-scr) or specific NCF2/p67phox-targeting siRNA (si-NCF2/p67phox) and collected after 48 h. NCF2/p67phox protein and transcript levels were examined by western blot and real-time PCR, respectively. Western blot for both whole and cleaved PARP was performed to assay apoptosis. The western blot is one representative experiment. Real-time PCR shows the mean of three independent experiments. Actin is shown as a loading control. (B) ROS levels were assayed using a DCFDA staining and FACS analysis. The mean of three independent experiments as a percentage respect to the control (presented as 100%) is shown. (C) Apoptosis levels were assayed by PI staining and FACS analysis. Percentage of sub-G1 events (M1) is shown for one of three experiments. The mean of three independent experiments is shown on the right panel.
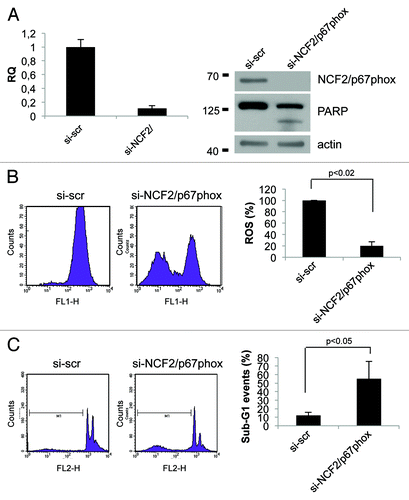
Suppression of ROS generation by siNCF2 induces apoptosis in HCT-116 and in HaCat cell lines
We next examined whether suppression of NCF2/p67phox by siNCF2 affects cell viability in HCT116 and in HaCat cell lines. Apoptosis in NCF2-silenced cells was evaluated as sub-G1 events by propidium iodide (PI) staining, followed by flow cytometry analysis and PARP enzyme cleavage. The data indicate that NCF2 knockdown in HCT116 induces an increasing cell number undergoing to apoptosis (from 15–44%), whereas scrambled control had no effect (), suggesting that depletion of ROS, obtained by impairing Nox2 activity, is sufficient for apoptosis induction. Similar results were obtained in HaCat cells (), suggesting that depletion of Nox2-generated ROS induces apoptosis (sub-G1 events increase from 12–55% and PARP enzyme is cleaved, ). Taken together, the data suggested that Nox2, and its subunit NCF2/p67phox, exerts an anti-apoptotic activity in HCT116 and in HaCat cells, and that suppression of this activity, lowering the intracellular ROS, leads to cell death.
Discussion
ROS are not only generated as a cellular response to exogenous stress stimuli, but also as products of normal aerobic metabolismCitation53-Citation59 or as second messengers in various signal transduction pathways.Citation27,Citation60-Citation64 ROS production may have deleterious effects on cells, and this event has been traditionally related to cancer development, aging and neurodegeneration.Citation65-Citation69 However, it is now accepted that ROS may have an important role in regulating signal transduction pathways, gene expression and differentiation, although the molecular mechanisms upstream and downstream ROS generation are not fully understood.Citation70-Citation75 The main non-mitochondrial sources of ROS are the NADPH oxidases, which are membrane-associated multi-protein complexes, of which NFC2/p67phox is an essential and crucial component, which produce superoxide. In this study, we identified NCF2 gene as a novel p53 target.Citation76-Citation79 Expression of NCF2/p67phox, by activating the Nox2 complex,Citation4 generates ROS that have protective functions against apoptosis in HCT116 and HaCat cell lines, confirming previous results obtained for Nox4 in pancreatic cancer cells.Citation22,Citation23 The pro-apoptotic activity of p53 is well documented and important for its multiple tumor suppressor functions. However, over the past years, it became clear that there is a second, less known, aspect of p53 function, implicating p53 also as an active mediator of pro-survival pathwaysCitation45,Citation80-Citation84 as demonstrated by a long list of pro-survival p53 targets.Citation47,Citation83-Citation86 The data presented here suggest that the novel p53 target gene, NCF2/p67phox, should be part of the above mentioned list. Furthermore, our results provide a molecular connection between the redox-sensitive signaling mechanism that mediates cell survival and p53 activity with its targets NFC2/p67phox.
Materials and Methods
Cell culture and transfection
HCT116, TAp63, ΔNp63 inducible SaOs-2 cells were cultured as described in Gressner et al., 2005.
RNA extraction, quantitative real-time RT-PCR and array
RNA was extracted from cells by using the RNAeasy Mini Kit (Qiagen). A total of 1,000 ng of RNA was used for reverse transcription using the GoScript Kit (Promega), and 2 ul of the reaction were used for real-time PCR. Normalization was performed amplifying human β-actin housekeeping mRNA using the following primers: forward 5′-GTTGCTATCCAGGCTGTGCTA-3′ and reverse 5′-AATGTCACGCACGATTTCCCGC-3′ (237 bp product). For detection of human p21 isoform expression, the primers used were: forward 5′-TGAGCGATGGAACTTCGAC-3′ and reverse 5′-ACAAGACAGTGACAGGTCC-3′ (214 bp product); while for human NCF2 expression, the primers were: forward 5′-ATCAGCCTCTGGAATGAAGGGG-3′ and reverse 5′-GCAGCCAATGTTGAAGCAAATCC-3′ (117 bp product). Real-time PCR was performed using an Applied Biosystems 7500 real-time PCR system. All the details related to the p53/TAp63/ΔNp63 dox-inducible SaOs-2 array have already been described previously.Citation50
Luc assay and constructs
HEK293 cells were cultured in 12-well dishes and transfected at 50–80% confluency. Transfections were performed with Effectene (Qiagen) according to the manufacturer’s recommendations. We used a 1:3 ratio between the reporter plasmid (containing the firefly luciferase gene under the control of the NCF2 promoter) and the given expression vectors encoding for all p53 family members. When needed, empty vector was added to keep the total amount of DNA (400 ng) used in each transfection constant. In all cases, 10 ng of Renilla Luciferase Vector (pRL-CMV; Promega) was co-transfected as a control of transfection efficiency. Twenty-four hours after transfection, luciferase activities in cellular extracts were measured by using a Dual Luciferase Reporter Assay System (Promega); light emission was measured over 10 sec using a Lumat LB9507 luminometer. pGL3-p67phox promoter construct was kindly provided by Professor Mark T. Quinn of Montana State University.
Western blotting
Cells were resuspended in RIPA buffer (1% Nonidet P-40, 0.5% sodium deoxycolate, 0.1% SDS) plus protease inhibitor cocktail (Sigma-Aldrich). After homogenization through QIA shredder columns (Qiagen) and protein determination, 50 μg of total proteins were loaded onto 10%SDS-PAGE and transferred to PVDF membranes (GE Healthcare). The blots were blocked with TBST (Tris-buffered saline and Tween 20)/10% nonfat dry milk (Biorad) and then incubated with the specific primary antibody: anti-NCF2/p67phox (610913, BD Biosciences), anti-HA-7 monoclonal mouse antibody (Sigma) and anti-PARP (SA-250, Enzo Life Sciences). Actin (A5441 mouse monoclonal antibody, Sigma-Aldrich) was used as a loading control. After washings and incubation with the appropriate horseradish peroxidase-conjugated secondary antibodies (Biorad), signal detection was performed with the Western Lightning Plus ECL (Perkin Elmer).
Chromatin immunoprecipitation
ChIP was performed using MAGnify Chromatin Immunoprecipitation System (Life Technologies) according to manufacturer’s protocol. Cells (1.5 × 106) were incubated with anti-p53 antibody DO-1 (sc-126, Santa Cruz Biotechnology). DNA samples were analyzed with 33 cycles of PCR to amplify NCF2 promoter sequences (94°C for 25 sec, 59°C for 25 sec, 72°C for 25 sec). We used three primer pairs amplifying the RE1, RE2 and RE3 p53 responsive element in the NCF2 promoter (RE1: forward 5′-CTGCCAGGAGACAGAGAGAAG -3′ and reverse 5′-GCCCAGAAAGTGAACACCTTG -3′; RE2: forward 5′-GGTGATAATGACAGGAGGCAGC-3′ and reverse 5′-TGGCTTGAAGGTGGGGTTTC-3′; RE3: forward 5′-TGCTTGTAGGATTGAAGGTGTGAG-3′ and reverse 5′-GAAAGATGAGTAGGAGTTTGCCAGG-3′).
A valuation of apoptosis and ROS by flow cytometry
HaCat and HCT-116 cells were collected 48 h after transfection, washed twice in PBS, stained with 10 μM DCFDA (dissolved in DMSO to a concentration of 1 mM then further diluted in PBS) (Life Technologies) for 30 min at 37°C. Twenty thousand events were collected by FacsCalibur flow cytometer (BD Biosciences) and analyzed with CellQuest software. For subsequent apoptosis analysis, cells were fixed with Methanol:Acetone 4:1, stored at 4°C overnight, then pelleted, treated with RNase and propidium iodide and analyzed by flow cytometry as described.
Bioinformatics
NCF2/p67phox promoter sequence was analyzed in search of p53-like responsive elements using GenomatixMatInspector software (www.genomatix.de).
Abbreviations: | ||
Nox | = | NADPH oxidase |
ROS | = | reactive oxygen species |
NADPH | = | nicotinamide adenine dinucleotide phosphate |
DCFH-DA | = | 2’,7’-dichlorodihydrofluorescein diacetate |
PI | = | propidium iodide |
ChIP | = | chromatin immunoprecipitation |
Acknowledgments
NCF2 promoter was kindly provided by Dr. Mark Quinn (Montana State University). We thank Dr. Marco Ranalli for technical support. This work has been supported by “Alleanza Contro il Cancro” (ACC12), Italian Human Proteome Net RBRN07BMCT, MIUR/PRIN 2008MRLSNZ_004, Telethon Grant GGPO9133, AIRC 5xmille #9979, AIRC IG1195, to G.M. Research described in this article was also supported in part by Min. Salute (Ricercaoncologica 26/07) and IDI-IRCCS (RF07 c.57, RF08 c.15, RF07 c.57) to G.M. and E.C.
Disclosure of Potential Conflicts of Interest
No potential conflicts of interest were disclosed.
References
- Craige SE, Keaney JF Jr.. Polyploidy and dysregulated ROS signaling: the school of hard Nox?. Cell Cycle 2009; 8:797; http://dx.doi.org/10.4161/cc.8.6.8336; PMID: 19279401
- Brown DI, Griendling KK. Nox proteins in signal transduction. Free Radic Biol Med 2009; 47:1239 - 53; http://dx.doi.org/10.1016/j.freeradbiomed.2009.07.023; PMID: 19628035
- Geiszt M, Leto TL. The Nox family of NAD(P)H oxidases: host defense and beyond. J Biol Chem 2004; 279:51715 - 8; http://dx.doi.org/10.1074/jbc.R400024200; PMID: 15364933
- Nauseef WM. Biological roles for the NOX family NADPH oxidases. J Biol Chem 2008; 283:16961 - 5; http://dx.doi.org/10.1074/jbc.R700045200; PMID: 18420576
- Geering B, Simon HU. A novel signaling pathway in TNFα-induced neutrophil apoptosis. Cell Cycle 2011; a 10:2821 - 2; http://dx.doi.org/10.4161/cc.10.17.16747; PMID: 21857151
- Geering B, Simon HU. Peculiarities of cell death mechanisms in neutrophils. Cell Death Differ 2011; b 18:1457 - 69; http://dx.doi.org/10.1038/cdd.2011.75; PMID: 21637292
- Clark RA, Volpp BD, Leidal KG, Nauseef WM. Translocation of cytosolic components of neutrophil NADPH oxidase. Trans Assoc Am Physicians 1989; 102:224 - 30; PMID: 2638527
- Sumimoto H, Hata K, Mizuki K, Ito T, Kage Y, Sakaki Y, et al. Assembly and activation of the phagocyte NADPH oxidase. Specific interaction of the N-terminal Src homology 3 domain of p47phox with p22phox is required for activation of the NADPH oxidase. J Biol Chem 1996; 271:22152 - 8; http://dx.doi.org/10.1074/jbc.271.36.22152; PMID: 8703027
- Lu W, Hu Y, Chen G, Chen Z, Zhang H, Wang F, et al. Novel role of NOX in supporting aerobic glycolysis in cancer cells with mitochondrial dysfunction and as a potential target for cancer therapy. PLoS Biol 2012; 10:e1001326; http://dx.doi.org/10.1371/journal.pbio.1001326; PMID: 22589701
- Del Principe D, Avigliano L, Savini I, Catani MV. Trans-plasma membrane electron transport in mammals: functional significance in health and disease. Antioxid Redox Signal 2011; 14:2289 - 318; http://dx.doi.org/10.1089/ars.2010.3247; PMID: 20812784
- Ushio-Fukai M. VEGF signaling through NADPH oxidase-derived ROS. Antioxid Redox Signal 2007; 9:731 - 9; http://dx.doi.org/10.1089/ars.2007.1556; PMID: 17511588
- Ushio-Fukai M, Zafari AM, Fukui T, Ishizaka N, Griendling KK. p22phox is a critical component of the superoxide-generating NADH/NADPH oxidase system and regulates angiotensin II-induced hypertrophy in vascular smooth muscle cells. J Biol Chem 1996; 271:23317 - 21; http://dx.doi.org/10.1074/jbc.271.38.23317; PMID: 8798532
- McCrann DJ, Yang D, Chen H, Carroll S, Ravid K. Upregulation of Nox4 in the aging vasculature and its association with smooth muscle cell polyploidy. Cell Cycle 2009; 8:902 - 8; http://dx.doi.org/10.4161/cc.8.6.7900; PMID: 19221493
- Brown DI, Griendling KK. Nox proteins in signal transduction. Free Radic Biol Med 2009; 47:1239 - 53; http://dx.doi.org/10.1016/j.freeradbiomed.2009.07.023; PMID: 19628035
- Kamata T. Roles of Nox1 and other Nox isoforms in cancer development. Cancer Sci 2009; 100:1382 - 8; http://dx.doi.org/10.1111/j.1349-7006.2009.01207.x; PMID: 19493276
- Prata C, Maraldi T, Fiorentini D, Zambonin L, Hakim G, Landi L. Nox-generated ROS modulate glucose uptake in a leukaemic cell line. Free Radic Res 2008; 42:405 - 14; http://dx.doi.org/10.1080/10715760802047344; PMID: 18473264
- Ben Mkaddem S, Pedruzzi E, Werts C, Coant N, Bens M, Cluzeaud F, et al. Heat shock protein gp96 and NAD(P)H oxidase 4 play key roles in Toll-like receptor 4-activated apoptosis during renal ischemia/reperfusion injury. Cell Death Differ 2010; 17:1474 - 85; http://dx.doi.org/10.1038/cdd.2010.26; PMID: 20224597
- Kang MA, So EY, Simons AL, Spitz DR, Ouchi T. DNA damage induces reactive oxygen species generation through the H2AX-Nox1/Rac1 pathway. Cell Death Dis 2012; 3:e249; http://dx.doi.org/10.1038/cddis.2011.134; PMID: 22237206
- Chen ZX, Pervaiz S. Involvement of cytochrome c oxidase subunits Va and Vb in the regulation of cancer cell metabolism by Bcl-2. Cell Death Differ 2010; 17:408 - 20; http://dx.doi.org/10.1038/cdd.2009.132; PMID: 19834492
- Mitsushita J, Lambeth JD, Kamata T. The superoxide-generating oxidase Nox1 is functionally required for Ras oncogene transformation. Cancer Res 2004; 64:3580 - 5; http://dx.doi.org/10.1158/0008-5472.CAN-03-3909; PMID: 15150115
- Brar SS, Kennedy TP, Sturrock AB, Huecksteadt TP, Quinn MT, Whorton AR, et al. An NAD(P)H oxidase regulates growth and transcription in melanoma cells. Am J Physiol Cell Physiol 2002; 282:C1212 - 24; PMID: 11997235
- Vaquero EC, Edderkaoui M, Pandol SJ, Gukovsky I, Gukovskaya AS. Reactive oxygen species produced by NAD(P)H oxidase inhibit apoptosis in pancreatic cancer cells. J Biol Chem 2004; 279:34643 - 54; http://dx.doi.org/10.1074/jbc.M400078200; PMID: 15155719
- Mochizuki T, Furuta S, Mitsushita J, Shang WH, Ito M, Yokoo Y, et al. Inhibition of NADPH oxidase 4 activates apoptosis via the AKT/apoptosis signal-regulating kinase 1 pathway in pancreatic cancer PANC-1 cells. Oncogene 2006; 25:3699 - 707; http://dx.doi.org/10.1038/sj.onc.1209406; PMID: 16532036
- Brar SS, Corbin Z, Kennedy TP, Hemendinger R, Thornton L, Bommarius B, et al. NOX5 NAD(P)H oxidase regulates growth and apoptosis in DU 145 prostate cancer cells. Am J Physiol Cell Physiol 2003; 285:C353 - 69; PMID: 12686516
- Weyemi U, Lagente-Chevallier O, Boufraqech M, Prenois F, Courtin F, Caillou B, et al. ROS-generating NADPH oxidase NOX4 is a critical mediator in oncogenic H-Ras-induced DNA damage and subsequent senescence. Oncogene 2012; 31:1117 - 29; http://dx.doi.org/10.1038/onc.2011.327; PMID: 21841825
- Tobaben S, Grohm J, Seiler A, Conrad M, Plesnila N, Culmsee C. Bid-mediated mitochondrial damage is a key mechanism in glutamate-induced oxidative stress and AIF-dependent cell death in immortalized HT-22 hippocampal neurons. Cell Death Differ 2011; 18:282 - 92; http://dx.doi.org/10.1038/cdd.2010.92; PMID: 20689558
- Lemarie A, Huc L, Pazarentzos E, Mahul-Mellier AL, Grimm S. Specific disintegration of complex II succinate:ubiquinone oxidoreductase links pH changes to oxidative stress for apoptosis induction. Cell Death Differ 2011; 18:338 - 49; http://dx.doi.org/10.1038/cdd.2010.93; PMID: 20706275
- Choi K, Ryu SW, Song S, Choi H, Kang SW, Choi C. Caspase-dependent generation of reactive oxygen species in human astrocytoma cells contributes to resistance to TRAIL-mediated apoptosis. Cell Death Differ 2010; 17:833 - 45; http://dx.doi.org/10.1038/cdd.2009.154; PMID: 19876066
- Kim JJ, Lee SB, Park JK, Yoo YD. TNF-alpha-induced ROS production triggering apoptosis is directly linked to Romo1 and Bcl-X(L). Cell Death Differ 2010; 17:1420 - 34; http://dx.doi.org/10.1038/cdd.2010.19; PMID: 20203691
- Magenta A, Cencioni C, Fasanaro P, Zaccagnini G, Greco S, Sarra-Ferraris G, et al. miR-200c is upregulated by oxidative stress and induces endothelial cell apoptosis and senescence via ZEB1 inhibition. Cell Death Differ 2011; 18:1628 - 39; http://dx.doi.org/10.1038/cdd.2011.42; PMID: 21527937
- Torii S, Goto Y, Ishizawa T, Hoshi H, Goryo K, Yasumoto K, et al. Pro-apoptotic activity of inhibitory PAS domain protein (IPAS), a negative regulator of HIF-1, through binding to pro-survival Bcl-2 family proteins. Cell Death Differ 2011; 18:1711 - 25; http://dx.doi.org/10.1038/cdd.2011.47; PMID: 21546903
- Lee JJ, Kim BC, Park MJ, Lee YS, Kim YN, Lee BL, et al. PTEN status switches cell fate between premature senescence and apoptosis in glioma exposed to ionizing radiation. Cell Death Differ 2011; 18:666 - 77; http://dx.doi.org/10.1038/cdd.2010.139; PMID: 21072054
- Edgar CE, Lindquist LD, McKean DL, Strasser A, Bram RJ. CAML regulates Bim-dependent thymocyte death. Cell Death Differ 2010; 17:1566 - 76; http://dx.doi.org/10.1038/cdd.2010.30; PMID: 20300112
- Ye F, Gao SJ. A novel role of hydrogen peroxide in Kaposi sarcoma-associated herpesvirus reactivation. Cell Cycle 2011; 10:3237 - 8; http://dx.doi.org/10.4161/cc.10.19.17299; PMID: 21941083
- Fan Y, Lee TV, Xu D, Chen Z, Lamblin AF, Steller H, et al. Dual roles of Drosophila p53 in cell death and cell differentiation. Cell Death Differ 2010; 17:912 - 21; http://dx.doi.org/10.1038/cdd.2009.182; PMID: 19960025
- Barlev NA, Sayan BS, Candi E, Okorokov AL. The microRNA and p53 families join forces against cancer. Cell Death Differ 2010; 17:373 - 5; http://dx.doi.org/10.1038/cdd.2009.73; PMID: 20062068
- Ueda S, Masutani H, Nakamura H, Tanaka T, Ueno M, Yodoi J. Redox control of cell death. Antioxid Redox Signal 2002; 4:405 - 14; http://dx.doi.org/10.1089/15230860260196209; PMID: 12215208
- Marino ML, Fais S, Djavaheri-Mergny M, Villa A, Meschini S, Lozupone F, et al. Proton pump inhibition induces autophagy as a survival mechanism following oxidative stress in human melanoma cells. Cell Death Dis 2010; 1:e87; http://dx.doi.org/10.1038/cddis.2010.67; PMID: 21368860
- Gough DR, Cotter TG. Hydrogen peroxide: a Jekyll and Hyde signalling molecule. Cell Death Dis 2011; 2:e213; http://dx.doi.org/10.1038/cddis.2011.96; PMID: 21975295
- Papaconstantinou J, Hsieh CC. Activation of senescence and aging characteristics by mitochondrially generated ROS: how are they linked?. Cell Cycle 2010; 9:3831 - 3; http://dx.doi.org/10.4161/cc.9.19.13324; PMID: 20890113
- Lin CL, Tseng HC, Chen RF, Chen WP, Su MJ, Fang KM, et al. Intracellular zinc release-activated ERK-dependent GSK-3β-p53 and Noxa-Mcl-1 signaling are both involved in cardiac ischemic-reperfusion injury. Cell Death Differ 2011; 18:1651 - 63; http://dx.doi.org/10.1038/cdd.2011.80; PMID: 21660051
- Kang MY, Kim HB, Piao C, Lee KH, Hyun JW, Chang IY, et al. The critical role of catalase in prooxidant and antioxidant function of p53. Cell Death Differ 2012; In press http://dx.doi.org/10.1038/cdd.2012.102; PMID: 22918438
- Wang X, Zeng L, Wang J, Chau JF, Lai KP, Jia D, et al. A positive role for c-Abl in Atm and Atr activation in DNA damage response. Cell Death Differ 2011; 18:5 - 15; http://dx.doi.org/10.1038/cdd.2010.106; PMID: 20798688
- Collavin L, Lunardi A, Del Sal G. p53-family proteins and their regulators: hubs and spokes in tumor suppression. Cell Death Differ 2010; 17:901 - 11; http://dx.doi.org/10.1038/cdd.2010.35; PMID: 20379196
- Tanaka T, Halicka HD, Huang X, Traganos F, Darzynkiewicz Z. Constitutive histone H2AX phosphorylation and ATM activation, the reporters of DNA damage by endogenous oxidants. Cell Cycle 2006; 5:1940 - 5; http://dx.doi.org/10.4161/cc.5.17.3191; PMID: 16940754
- Marchenko ND, Hanel W, Li D, Becker K, Reich N, Moll UM. Stress-mediated nuclear stabilization of p53 is regulated by ubiquitination and importin-alpha3 binding. Cell Death Differ 2010; 17:255 - 67; http://dx.doi.org/10.1038/cdd.2009.173; PMID: 19927155
- Jänicke RU, Sohn D, Schulze-Osthoff K. The dark side of a tumor suppressor: anti-apoptotic p53. Cell Death Differ 2008; 15:959 - 76; http://dx.doi.org/10.1038/cdd.2008.33; PMID: 18356920
- Sourbier C, Valera-Romero V, Giubellino A, Yang Y, Sudarshan S, Neckers L, et al. Increasing reactive oxygen species as a therapeutic approach to treat hereditary leiomyomatosis and renal cell carcinoma. Cell Cycle 2010; 9:4183 - 9; http://dx.doi.org/10.4161/cc.9.20.13458; PMID: 20953139
- Alexander A, Walker CL. Differential localization of ATM is correlated with activation of distinct downstream signaling pathways. Cell Cycle 2010; 9:3685 - 6; http://dx.doi.org/10.4161/cc.9.18.13253; PMID: 20890104
- Gressner O, Schilling T, Lorenz K, Schulze Schleithoff E, Koch A, Schulze-Bergkamen H, et al. TAp63alpha induces apoptosis by activating signaling via death receptors and mitochondria. EMBO J 2005; 24:2458 - 71; http://dx.doi.org/10.1038/sj.emboj.7600708; PMID: 15944736
- Quandt K, Frech K, Karas H, Wingender E, Werner T. MatInd and MatInspector: new fast and versatile tools for detection of consensus matches in nucleotide sequence data. Nucleic Acids Res 1995; 23:4878 - 84; http://dx.doi.org/10.1093/nar/23.23.4878; PMID: 8532532
- Gauss KA, Bunger PL, Quinn MT. AP-1 is essential for p67(phox) promoter activity. J Leukoc Biol 2002; 71:163 - 72; PMID: 11781392
- Pinton P, Giorgi C, Pandolfi PP. The role of PML in the control of apoptotic cell fate: a new key player at ER-mitochondria sites. Cell Death Differ 2011; 18:1450 - 6; http://dx.doi.org/10.1038/cdd.2011.31; PMID: 21475307
- Thomenius M, Freel CD, Horn S, Krieser R, Abdelwahid E, Cannon R, et al. Mitochondrial fusion is regulated by Reaper to modulate Drosophila programmed cell death. Cell Death Differ 2011; 18:1640 - 50; http://dx.doi.org/10.1038/cdd.2011.26; PMID: 21475305
- Jeyaraju DV, McBride HM, Hill RB, Pellegrini L. Structural and mechanistic basis of Parl activity and regulation. Cell Death Differ 2011; 18:1531 - 9; http://dx.doi.org/10.1038/cdd.2011.22; PMID: 21415861
- Liu X, Hajnóczky G. Altered fusion dynamics underlie unique morphological changes in mitochondria during hypoxia-reoxygenation stress. Cell Death Differ 2011; 18:1561 - 72; http://dx.doi.org/10.1038/cdd.2011.13; PMID: 21372848
- Hagen N, Hans M, Hartmann D, Swandulla D, van Echten-Deckert G. Sphingosine-1-phosphate links glycosphingolipid metabolism to neurodegeneration via a calpain-mediated mechanism. Cell Death Differ 2011; 18:1356 - 65; http://dx.doi.org/10.1038/cdd.2011.7; PMID: 21331079
- Stefanatos R, Sanz A. Mitochondrial complex I: a central regulator of the aging process. Cell Cycle 2011; 10:1528 - 32; http://dx.doi.org/10.4161/cc.10.10.15496; PMID: 21471732
- Martinez-Outschoorn UE, Balliet RM, Rivadeneira DB, Chiavarina B, Pavlides S, Wang C, et al. Oxidative stress in cancer associated fibroblasts drives tumor-stroma co-evolution: A new paradigm for understanding tumor metabolism, the field effect and genomic instability in cancer cells. Cell Cycle 2010; 9:3256 - 76; http://dx.doi.org/10.4161/cc.9.16.12553; PMID: 20814239
- Sablina AA, Budanov AV, Ilyinskaya GV, Agapova LS, Kravchenko JE, Chumakov PM. The antioxidant function of the p53 tumor suppressor. Nat Med 2005; 11:1306 - 13; http://dx.doi.org/10.1038/nm1320; PMID: 16286925
- Lu MY, Liao FL. Interferon-stimulated gene ISG12b2 is localized to the inner mitochondrial membrane and mediates virus-induced cell death. Cell Death Differ 2011; 18:925 - 36; http://dx.doi.org/10.1038/cdd.2010.160; PMID: 21151029
- John K, Alla V, Meier C, Pützer BM. GRAMD4 mimics p53 and mediates the apoptotic function of p73 at mitochondria. Cell Death Differ 2011; 18:874 - 86; http://dx.doi.org/10.1038/cdd.2010.153; PMID: 21127500
- Bouman L, Schlierf A, Lutz AK, Shan J, Deinlein A, Kast J, et al. Parkin is transcriptionally regulated by ATF4: evidence for an interconnection between mitochondrial stress and ER stress. Cell Death Differ 2011; 18:769 - 82; http://dx.doi.org/10.1038/cdd.2010.142; PMID: 21113145
- Tang Y, Chen Y, Jiang H, Nie D. Short-chain fatty acids induced autophagy serves as an adaptive strategy for retarding mitochondria-mediated apoptotic cell death. Cell Death Differ 2011; 18:602 - 18; http://dx.doi.org/10.1038/cdd.2010.117; PMID: 20930850
- Genestra M. Oxyl radicals, redox-sensitive signalling cascades and antioxidants. Cell Signal 2007; 19:1807 - 19; http://dx.doi.org/10.1016/j.cellsig.2007.04.009; PMID: 17570640
- Nakamura T, Lipton SA. Redox modulation by S-nitrosylation contributes to protein misfolding, mitochondrial dynamics, and neuronal synaptic damage in neurodegenerative diseases. Cell Death Differ 2011; 18:1478 - 86; http://dx.doi.org/10.1038/cdd.2011.65; PMID: 21597461
- Maccarrone M, Brüne B. Redox regulation and the metabolic syndrome. Cell Death Differ 2011; 18:1234 - 6; http://dx.doi.org/10.1038/cdd.2011.58; PMID: 21566661
- Lee JJ, Kim BC, Park MJ, Lee YS, Kim YN, Lee BL, et al. PTEN status switches cell fate between premature senescence and apoptosis in glioma exposed to ionizing radiation. Cell Death Differ 2011; 18:666 - 77; http://dx.doi.org/10.1038/cdd.2010.139; PMID: 21072054
- Edgar CE, Lindquist LD, McKean DL, Strasser A, Bram RJ. CAML regulates Bim-dependent thymocyte death. Cell Death Differ 2010; 17:1566 - 76; http://dx.doi.org/10.1038/cdd.2010.30; PMID: 20300112
- Goldstone SD, Milligan AD, Hunt NH. Oxidative signalling and gene expression during lymphocyte activation. Biochim Biophys Acta 1996; 1314:175 - 82; http://dx.doi.org/10.1016/S0167-4889(96)00082-1; PMID: 8972731
- Tatla S, Woodhead V, Foreman JC, Chain BM. The role of reactive oxygen species in triggering proliferation and IL-2 secretion in T cells. Free Radic Biol Med 1999; 26:14 - 24; http://dx.doi.org/10.1016/S0891-5849(98)00133-6; PMID: 9890636
- Sardina JL, López-Ruano G, Sánchez-Abarca LI, Pérez-Simón JA, Gaztelumendi A, Trigueros C, et al. p22phox-dependent NADPH oxidase activity is required for megakaryocytic differentiation. Cell Death Differ 2010; 17:1842 - 54; http://dx.doi.org/10.1038/cdd.2010.67; PMID: 20523355
- Buggisch M, Ateghang B, Ruhe C, Strobel C, Lange S, Wartenberg M, et al. Stimulation of ES-cell-derived cardiomyogenesis and neonatal cardiac cell proliferation by reactive oxygen species and NADPH oxidase. J Cell Sci 2007; 120:885 - 94; http://dx.doi.org/10.1242/jcs.03386; PMID: 17298980
- Tsatmali M, Walcott EC, Makarenkova H, Crossin KL. Reactive oxygen species modulate the differentiation of neurons in clonal cortical cultures. Mol Cell Neurosci 2006; 33:345 - 57; http://dx.doi.org/10.1016/j.mcn.2006.08.005; PMID: 17000118
- Rikka S, Quinsay MN, Thomas RL, Kubli DA, Zhang X, Murphy AN, et al. Bnip3 impairs mitochondrial bioenergetics and stimulates mitochondrial turnover. Cell Death Differ 2011; 18:721 - 31; http://dx.doi.org/10.1038/cdd.2010.146; PMID: 21278801
- Ago T, Matsushima S, Kuroda J, Zablocki D, Kitazono T, Sadoshima J. The NADPH oxidase Nox4 and aging in the heart. Aging (Albany NY) 2010; 2:1012 - 6; PMID: 21212466
- Hwang AB, Lee SJ. Regulation of life span by mitochondrial respiration: the HIF-1 and ROS connection. Aging (Albany NY) 2011; 3:304 - 10; PMID: 21389351
- Ozden O, Park SH, Kim HS, Jiang H, Coleman MC, Spitz DR, et al. Acetylation of MnSOD directs enzymatic activity responding to cellular nutrient status or oxidative stress. Aging (Albany NY) 2011; 3:102 - 7; PMID: 21386137
- Vigneron A, Vousden KH. p53, ROS and senescence in the control of aging. Aging (Albany NY) 2010; 2:471 - 4; PMID: 20729567
- Vousden KH. Outcomes of p53 activation--spoilt for choice. J Cell Sci 2006; 119:5015 - 20; http://dx.doi.org/10.1242/jcs.03293; PMID: 17158908
- Lee S, Kim JY, Kim YJ, Seok KO, Kim JH, Chang YJ, et al. Nucleolar protein GLTSCR2 stabilizes p53 in response to ribosomal stresses. Cell Death Differ 2012; 19:1613 - 22; http://dx.doi.org/10.1038/cdd.2012.40; PMID: 22522597
- Kim DY, Kim W, Lee KH, Kim SH, Lee HR, Kim HJ, et al. hnRNP Q regulates translation of p53 in normal and stress conditions. Cell Death Differ 2012; In press http://dx.doi.org/10.1038/cdd.2012.109; PMID: 22935615
- Wang Y, Cui J, Sun X, Zhang Y. Tunneling-nanotube development in astrocytes depends on p53 activation. Cell Death Differ 2011; 18:732 - 42; http://dx.doi.org/10.1038/cdd.2010.147; PMID: 21113142
- Morizot A, Mérino D, Lalaoui N, Jacquemin G, Granci V, Iessi E, et al. Chemotherapy overcomes TRAIL-R4-mediated TRAIL resistance at the DISC level. Cell Death Differ 2011; 18:700 - 11; http://dx.doi.org/10.1038/cdd.2010.144; PMID: 21072058
- Schug ZT, Gonzalvez F, Houtkooper RH, Vaz FM, Gottlieb E. BID is cleaved by caspase-8 within a native complex on the mitochondrial membrane. Cell Death Differ 2011; 18:538 - 48; http://dx.doi.org/10.1038/cdd.2010.135; PMID: 21072056
- Lalier L, Cartron PF, Olivier C, Logé C, Bougras G, Robert JM, et al. Prostaglandins antagonistically control Bax activation during apoptosis. Cell Death Differ 2011; 18:528 - 37; http://dx.doi.org/10.1038/cdd.2010.128; PMID: 20966963