Abstract
SIRT1 is an evolutionarily conserved protein deacetylase that modulates stress response, cellular metabolism and aging in model organisms. While SIRT1 exerts beneficial effects in protecting against age-related diseases, the role of SIRT1 in cancer has been controversial. SIRT1 promotes cell survival by deacetylating, and thereby negatively regulating the activity of important tumor suppressors such as p53. In this regard, SIRT1 has been considered to be a potential oncogene, and SIRT1 inhibitors have been studied for possible anticancer therapeutic effects. In contrast, it has been shown that SIRT1 deficiency leads to increased genomic instability and tumorigenesis, and that overexpression of SIRT1 attenuates cancer formation in mice, suggesting it may also act as a tumor suppressor. Based on this evidence, SIRT1-activating molecules could act as candidate chemotherapeutic drugs. In order to gain insight into the role of SIRT1 in cancer, we performed a comprehensive resequencing analysis of the SIRT1 gene in 41 tumor cell lines and found an unusually excessive homozygosity, which was confirmed to be allelic loss by microsatellite analysis. Furthermore, we found two novel SIRT1 mutations (D739Y and R65_A72del) in addition to the known, rare non-synonymous variation resulting in I731V. In vitro assays using purified SIRT1 protein showed that these mutations do not alter SIRT1 deacetylase activity or telomerase activity, which was shown to be regulated by SIRT1. We conclude that allelic loss or mutations in the SIRT1 gene occur prevalently during tumorigenesis, supporting the assertion that SIRT1 may serve as a tumor suppressor.
Introduction
SIRT1 is a member of the sirtuin protein family, which is implicated in the modulation of aging and lifespan in invertebrates. Overexpression of Sir2, a founding member of the sirtuin family, extends lifespan in yeast, worms and flies under certain conditions.Citation1-Citation3 SIRT1 is the closest mammalian homolog of yeast Sir2. SIRT1 encodes a NAD+-dependent protein deacetylase that plays a critical role in diverse cellular functions, including cell survival, cell cycle, DNA repair, inflammation, senescence and metabolic regulation.Citation4,Citation5
Despite the wealth of data from animal disease models on the beneficial effects of SIRT1 in preventing aging-related diseases,Citation6,Citation7 the role of SIRT1 in tumorigenesis has been controversial.Citation8,Citation9 Some reports support the notion that SIRT1 is implicated in tumor promotion, as increased SIRT1 expression has been observed in a variety of cancers, including prostate, skin, colon and acute myeloid leukemia.Citation10-Citation14 Furthermore, SIRT1 deacetylates and inactivates p53, rendering cells resistant to apoptosis,Citation15 and pharmacological inhibition of SIRT1 leads to activation of p53 and arrest of tumor cell growth in vitro and in vivo.Citation16 Similarly, SIRT1 deacetylates FOXOs, inhibiting its proapoptotic function under stress conditions.Citation17 Yet, paradoxically, there is no reported evidence of increased cancer in numerous mouse models in which SIRT1 is overexpressed, arguing against an oncogenic role for SIRT1.Citation18
On the other hand, there is strong evidence that SIRT1 plays a tumor-suppressive role in mouse models of cancer. In p53 heterozygous mice, SIRT1 deficiency leads to increased incidence of sarcomas and lymphomas, whereas increased SIRT1 expression results in delay of their development caused by p53 deficiency.Citation19,Citation20 Overexpression of SIRT1 in APCmin/+ mice results in suppression of intestinal tumorigenesis via attenuation of β-catenin activity.Citation21 Moreover, incidence of age-related spontaneous cancers, such as carcinomas and sarcomas, decreases in Sirt1 transgenic mice.Citation18 In humans, some cancer tissues, such as breast cancer samples, show reduced SIRT1 expression levels as compared with their normal counterparts, suggesting a tissue-specific role for SIRT1 as a tumor suppressor.Citation19
Identification of SIRT1 gene mutations in human cancers can potentially provide valuable information as to the role of SIRT1 in cancer and provide an additional therapeutic target for treatment. However, no comprehensive sequencing for SIRT1 mutations in human cancer has been reported. Thus far, six somatic mutations in SIRT1 have been reported in cancers (www.sanger.ac.uk/genetics/CGP/cosmic). Here we analyzed 41 human cancer cell lines to search for possible alterations in the SIRT1 gene.
Results
We resequenced the SIRT1 gene of 40 human breast cancer cell lines and a cervical cancer cell line to detect all possible genetic variations in exons, exon-intron junctions and 1 kb proximal promoter. Remarkably, the sequencing results indicated that 66% of the cancer cell lines (27 out of the 41 cell lines) showed a homozygosity in the SIRT1 locus (; Table S1), which was significantly higher than the average homozygosity of the SIRT1 locus (29%) detected in normal populations from the HapMap database, including a total of 1,417 individuals (p = 2 × 10−5, binomial test). Since this excessive homozygosity suggests an allelic loss of the SIRT1 locus in these cancer cell lines, we further analyzed the allelic loss by microsatellite analysis using three microsatellite markers, which are located at 122 kb, 47 kb and 30 kb upstream of the SIRT1 gene (Table S1). This is in line with the previous results from a genome-wide survey showing an excessive heterozygous loss of chromosome 10q (15 out of 24 breast cancer cell lines), where the SIRT1 gene is located.Citation22
Table 1.SIRT1 mutation analysis of 40 human breast cancer cell lines and HeLa cell line
In this study, we identified a total of 31 sequence variants, among which six were previously unknown, novel variants that were not reported in the various SNP databases including the 1,000 genomes database ( and ). Four variants were in a coding region, among which two missense mutations, 2244A > G (I731V) and 2268G > T (D739Y), were discovered in the breast cancer cell lines SUM185PE and ZR75–30, respectively ( and ). The 24-bp deletion mutation of nucleotides between positions 245–268, resulting in an in-frame deletion of eight amino acids (R65_A72del) in the SIRT1 protein (), was identified in the cervical cancer cell line HeLa. We validated the presence of these coding variants by confirmatory sequencing analysis (). None of the mutations were accompanied by loss of the other SIRT1 allele (). The D739Y mutation was predicted to have an impact on SIRT1 protein function by in silico analyses using both Polyphen (www.genetics.bwh.harvard.edu/pph) and SIFT (www.blocks.fhcrc.org/sift), while the I731V mutation was predicted to be deleterious by SIFT only.
Table 2.SIRT1 sequence variants among 41 cancer cell lines
Figure 1. Identification of three SIRT1 mutant cancer cell lines by PCR amplification and direct sequencing. (A) Twenty-four-bp deletion of nucleotides 245–268 was found in the HeLa cell line, corresponding to an in-frame deletion of eight amino acids (R65_A72del). (B) One missense mutation 2244A > G, resulting in one amino acid change I731V, was discovered in breast cancer cell line, SUM185PE. (C) One missense mutation 2268G > T, resulting in one amino acid change D739Y, was discovered in breast cancer cell line, ZR75–30. Top row of each data represents electropherograms showing the wild-type sequence. Bottom row of each data represents electropherograms showing the mutations.
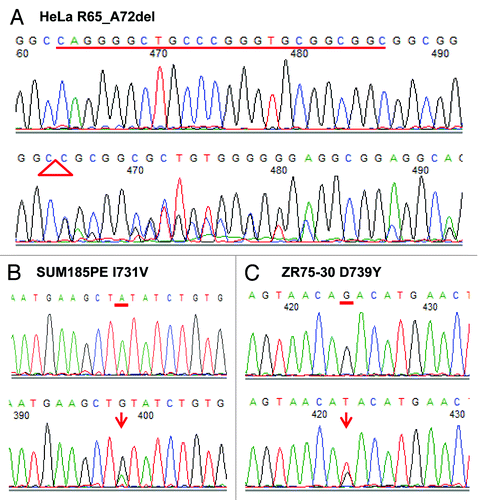
To investigate the functional consequences of the SIRT1 mutations, we performed the following in vitro assays. First, we examined whether the SIRT1 mutations identified had any impact on the deacetylase activity. We produced wild-type and mutant recombinant SIRT1 proteins in E. coli, and employed two complementary in vitro deacetylation assays to compare the activity of these proteins. We found that all three mutant proteins displayed wild-type enzyme activity (). Second, since it has been reported that SIRT1 expression attenuates telomere erosion with age in adult tissues, an effect which is largely dependent on telomerase activity,Citation23 we determined whether the three mutant SIRT1 proteins affect telomerase activity using a TRAP assay. Our results showed that the three mutant SIRT1 proteins did not influence the telomerase activity (). Lastly, since SIRT1 was reported to positively regulate the expression of Rictor in liver cells,Citation24 a component of the mTORC2 complex, we performed a western blot in the HEK293 cell line to determine whether the SIRT1 mutations caused changes in the expression levels of Rictor. When SIRT1 was overexpressed, Rictor was only marginally increased (approximately 1.2-fold), and, similarly, none of the three mutants caused detectable changes in the expression levels of Rictor ().
Figure 2. In vitro deacetylase activity assay of wild-type and mutant SIRT1 proteins. (A) Coomassie-stained gel showing expression and purity of wild-type and mutant SIRT1 proteins. (B) Activity of wild-type and mutant SIRT1 proteins determined using the BIOMOL assay (n = 2; mean + s.d. shown). (C) Activity of wild-type and mutant SIRT1 proteins determined using the PNC1-OPT assay with a natural amino acid substrate (n = 3; mean + s.d. shown).
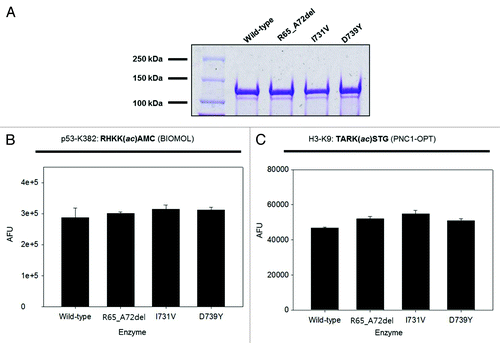
Figure 3. Telomerase activity in 293T cells overexpressing SIRT1 mutants. Cells were transfected with plasmids coding for indicated mutants or empty vector, and TRAP assays were conducted after 48 h of transfections. The graph shows mean ± s.d. from triplicate experiments.
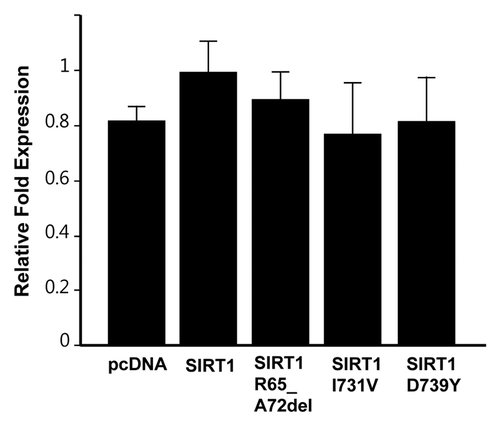
Figure 4. Western blot analysis of Rictor levels. (A) HEK293 cells were transfected with either pcDNA3.1 vector, Flag-tagged wild-type SIRT1 or Flag-tagged mutant SIRT1 constructs. Forty-eight hours post-transfection, the cells were collected and the proteins were analyzed by western blot with antibodies against Rictor, Flag and α-tubulin. (B) Bar graph showing the quantification of Rictor compared with α-tubulin. Values are means ± SE for three different sets of western blots.
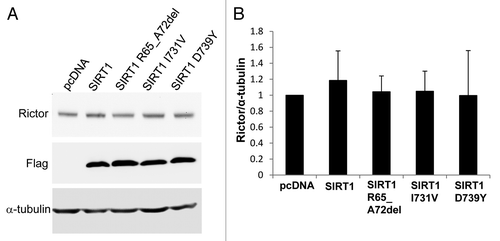
Discussion
Although there has been a growing body of evidence suggesting that SIRT1 plays a protective role in multiple age-related diseases,Citation6 the functional role of SIRT1 in cancer remains controversial.Citation25,Citation26 Depending on the cellular and tissue context, SIRT1 has been linked to increased or decreased cancer risks.Citation10,Citation12,Citation13,Citation19,Citation27,Citation28 These results suggest that SIRT1 may function either as an oncogene or tumor suppressor. Identification of SIRT1 gene mutations in human cancers can provide valuable information as to the controversial role of SIRT1 in tumorigenesis. However, no comprehensive search for SIRT1 mutations in human cancer has been reported thus far. In this study, we found an excessive allelic loss of the SIRT1 gene as well as mutations predicted to be deleterious among 40 breast cancer cell lines and a cervical cancer cell line by sequencing the complete coding and proximal promoter regions of SIRT1. None of the mutations were accompanied by loss of the other SIRT1 allele. Our results support the notion that SIRT1 might act as a non-traditional tumor suppressor, as suggested by recent studies demonstrating that in the context of a p53 heterozygote-null mouse, haploinsufficiency of SIRT1 results in increased tumor formation,Citation19 while overexpressing SIRT1 reduces tumors.Citation20 Furthermore, a reduced expression of SIRT1 has been observed in many types of cancers as compared with their normal control counterparts, particularly in the breast, suggesting that SIRT1 may act as a tumor suppressor in this tissue.
The two breast cancer cell lines we characterized to harbor SIRT1 missense mutations, SUM185PE (I731V) and ZR75–30 (D739Y), and the cervical cancer cell line HeLa, which we found to carry a SIRT1 in-frame deletion mutation of eight amino acids (R65_A72del), did not show allelic loss of SIRT1. However, when we treated these cell lines with a demethylating agent, 5-Aza-Cytidine (5 μM), the SIRT1 mRNA levels increased by 3.5~5-fold, as measured by qPCR (data not shown), suggesting that the intact wild-type allele may be epigenetically silenced in these cell lines. Recently, SIRT1 was shown to be positively regulated by BRCA1 via direct binding to the SIRT1 promoter region.Citation27 Also, SIRT1 mRNA levels have been shown to be significantly lower in breast cancer samples with a BRCA1 mutation compared with breast cancer samples without BRCA1 mutation.Citation27 Consistent with these findings, among the breast cancer cell lines with the allelic loss of SIRT1, HCC1937, MDA-MB-436 and SUM149PT were found to harbor BRCA1 mutations.Citation29 In addition, the two breast cell lines that harbor SIRT1 missense mutations, SUM185PE and ZR75-30, have significantly lower BRCA1 transcript levels.Citation29 These results suggest a possible mechanism for lower SIRT1 expression in these cell lines.
The deleted region (R65_A72del) of SIRT1 gene in HeLa cells is located in a canonical N-myristoylation region (66–71), potentially implicating this deletion in impaired membrane targeting, signal transduction or protein-protein interactions. The two missense SIRT1 mutations detected in the breast cancer cell lines SUM185PE (I731V) and ZR75–30 (D739Y) were predicted to be deleterious. In addition, these missense mutations are positioned in the vicinity of lysine 734, a residue which is sumoylated and known to modulate SIRT1 deacetylase activity.Citation30 To study the functional consequences of the three SIRT1 mutations, we performed functional analysis using in vitro assays. First of all, since SIRT1 is known to modulate cancer risk by deacetylating targets such as p53,Citation15,Citation16 NFκB,Citation31 c-Myc,Citation32 β-catenin,Citation21 FOXOsCitation17 and Ku70,Citation33 we tested if these mutations cause changes in the deacetylase activity of SIRT1. Utilizing two sensitive SIRT1 activity assays, we detected no differences between the wild-type and mutant proteins (). Based on the role of SIRT1 in TERT expression and activity,Citation23,Citation32,Citation34 we examined whether the three mutants had any effects on telomerase activity. We could not detect any significant change in telomerase activity caused by these mutations as measured by TRAP assay (). In addition, we also tested the impact of SIRT1 mutations on Rictor expression. According to a recent study, Sirt1 was shown to positively regulate Rictor expression both in vivo and in vitro.Citation24 However, no significant change in Rictor expression was detected by qPCR and immunoblot analysis (data not shown and ). We concluded that there was no impact on SIRT1 function for these mutations on the parameters tested.
In summary, we conducted a comprehensive sequence analysis of the SIRT1 gene in 40 breast cancer cell lines and a HeLa cell line. In our study, we report an unusually excessive homozygosity as well as novel, potentially functional mutations. Our results support the notion that SIRT1 may act as a non-classical tumor suppressor under certain conditions in humans. Identification of gene mutations in cancer cell lines will provide valuable research tools to elucidate the functional roles of genes implicated in cancer.
Materials and Methods
Cell lines
The 40 human breast cancer cell lines used in this study were previously reportedCitation29 and are listed in . A human cervical cancer cell line, HeLa, was a kind gift from Dr. M. Levy (Albert Einstein College of Medicine). The HEK293 cell line was obtained from the American Type Culture Collection.
Mutation analysis
The complete coding sequences, exon-intron boundaries and 1 kb upstream of the 5′UTR region of SIRT1 (GenBank AF083106) were amplified from genomic DNA in seven gene fragments. Fragment 1 contained a 5′UTR and was amplified using primers 5′-CACGCAGGTAATTGATGCAG-3′ and 5′-GGGCCAGACCACAACACTAC-3′. Phusion high-fidelity DNA polymerase (Thermo Scientific) was used for the PCR reaction at 98°C for 2 min, followed by 40 cycles at 98°C for 20 s, 65°C for 30 s and 72°C for 1 min. Fragment 2 contained the 5′UTR and exon 1 and was amplified using primers 5′-CGAATTTGGCTGCACTACAC-3′ and 5′-CAACCTCAGGCCAGTAGGAG-3′. GC-rich polymerase (Roche Applied Science) was used for PCR reaction at 96°C for 2 min, followed by 40 cycles at 96°C for 20 s, 63°C for 30 s and 72°C for 1 min. Fragment 3 contained exons 2–3 and was amplified using primers 5′-CCAATGAACAGTGCAAGCTG-3′ and 5′-GCTGCTGCACTCAGTTTTTG-3′. Phusion high-fidelity DNA polymerase (Thermo Scientific) was used for the PCR reaction at 96°C for 2 min, followed by 40 cycles at 96°C for 20 s, 63°C for 30 s and 72°C for 1.5 min. Fragment 4 contained exon 4 and was amplified using primers 5′-TTCTGAAATAAGGGTAGGGTTG-3′ and 5′-CCTCTAGGAATAAAGGAATTCTAGCC-3′. Phusion high-fidelity DNA polymerase (Thermo Scientific) was used for the PCR reaction at 96°C for 2 min, followed by 40 cycles at 96°C for 20 s, 60°C for 30 s and 72°C for 1 min. Fragment 5 contained exons 5–7 and was amplified twice using initial primers 5′-AGCAACCGTCCTTTTGTAGG-3′ and 5′-TGAGCCAAGATATGCGACTG-3′. Phusion high-fidelity DNA polymerase (Thermo Scientific) was used for the PCR reaction at 96°C for 2 min, followed by 40 cycles at 96°C for 20 s, 63°C for 30 s and 72°C for 3 min. Then, a second nested amplification was performed using primers 5′-TAGGTGTGTGTCGCATCCAT-3′ and 5′- GCAACAAGAGGGAAACTCCA-3′. Phusion high-fidelity DNA polymerase (Thermo Scientific) was used with the same PCR conditions as the initial PCR amplification. Fragment 6 contained exon 8 and was amplified using primers 5′-TCAAGCCCTTGTTGGATTTT-3′ and 5′-GCACTATTAAACACTTCCTTCTCCA-3′. Phusion high-fidelity DNA polymerase (Thermo Scientific) was used for the PCR reaction at 96°C for 2 min, followed by 40 cycles at 96°C for 20 s, 63°C for 30 s and 72°C for 30 s. The last fragment contained exon 9 and 3′UTRs and was amplified using 5′-CTAATGTAGGCTGGGCTGGA-3′ and 5′-ACACTGCTGGCCTCTTCAGT-3′. Expand high-fidelity plus taq polymerase (Roche Applied Science) was used for the PCR reaction at 96°C for 2 min, followed by 40 cycles at 96°C for 20 s, 67°C for 30 s and 72°C for 3 min. Amplified products were than analyzed for sequence alterations using Big Dye Terminator v3.1 kit (Applied Biosystems) using an ABI 3730xl DNA Analyzer. All unique sequence alterations were confirmed by sequencing of an independently amplified template.
Microsatellite analysis
Three microsatellites near the SIRT1 gene (NM_012238.4, chr10:69644427–69678147) were chosen for determining allelic loss of the SIRT1 gene. The positions of the di-nucleotide repeat microsatellites were chr10:69518082–69518114 (16AC repeat), chr10:69595476–69595506 (15AC repeat) and chr10:69614278–69614320 (21AC repeat). Primers for amplifying each region were custom tailed and were 5′-fluorescent labeled by NED or PET (Applied Biosystems). The 16AC repeat was amplified using primers 5′-PETTTCTCAGCCTCCTTAACCACA-3′ and 5′-ABDtailGGAAAACCAAGTCTTTTTCCTTG-3′. Phusion high-fidelity DNA polymerase (Thermo Scientific) was used for the PCR reaction at 98°C for 2 min, followed by 35 cycles at 98°C for 20 s, 64°C for 30 s and 72°C for 30 s. The 15 AC repeat was amplified using primers 5′-NEDTTGCAGTGACCTGAGATTGC-3′ and 5′-ABDtailTTCCTTGTCTCAACATTCCTTTT-3′. Phusion high-fidelity DNA polymerase (Thermo Scientific) was used for the PCR reaction at 98°C for 2 min, followed by 35 cycles at 98°C for 20 s, 66°C for 30 s and 72°C for 30 s. The 21AC repeat was amplified using primers 5′-NEDAGCCTGGGTAGCAGAATGAA-3′ and 5′-ABDtailTGTCTATAGGGGCCAGAACG-3′. Phusion high-fidelity DNA polymerase (Thermo Scientific) was used for the PCR reaction at 98°C for 2 min, followed by 30 cycles at 98°C for 20 s, 60°C for 30 s and 72°C for 30 s. Amplified regions were separated by electrophoresis in standard denaturing sequencing gels, and the reactions were visualized by autoradiography.
Estimation of homozygosity in SIRT1 gene in general population
Hapmap data for public release #28 (August 2010), encompassing phases I+II+III (NCBI build 36, dbSNP b126) merged, were used to estimate the homozygosity in the SIRT1 gene. Eighty-seven individuals from ASW (African ancestry in Southwest USA) with 37 SNPs were analyzed, and 23 individuals had homozygosity throughout the SIRT1 gene. One hundred and seventy-four individuals from CEU (Utah residents with Northern and Western European ancestry from the CEPH collection) with 89 SNPs were analyzed, and 83 individuals had homozygosity throughout the SIRT1 gene. One hundred and thirty-nine individuals from CHB (Han Chinese in Beijing, China) with 88 SNPs were analyzed, and 35 individuals had homozygosity throughout the SIRT1 gene. One hundred and nine individuals from CHD (Chinese in Metropolitan Denver, Colorado) with 32 SNPs were analyzed, and 30 individuals had homozygosity throughout the SIRT1 gene. One hundred and one individuals from GIH (Gujarati Indians in Houston, Texas) with 35 SNPs were analyzed, and 32 individuals had homozygosity throughout the SIRT1 gene. One hundred and sixteen individuals from JPT (Japanese in Tokyo, Japan) with 88 SNPs were analyzed, and 26 individuals had homozygosity throughout the SIRT1 gene. One hundred and ten individuals from LWK (Luhya in Webuye, Kenya) with 36 SNPs were analyzed, and 24 individuals had homozygosity throughout the SIRT1 gene. Eighty-six individuals from MEX (Mexican ancestry in Los Angeles, California) with 31 SNPs were analyzed, and 24 individuals had homozygosity throughout the SIRT1 gene. One hundred and eighty-four individuals from MKK (Maasai in Kinyawa, Kenya) with 38 SNPs were analyzed, and 40 individuals had homozygosity throughout the SIRT1 gene. One hundred and two individuals from TSI (Tuscan in Italy) with 34 SNPs were analyzed, and 50 individuals had homozygosity throughout the SIRT1 gene. Two hundred and nine individuals from YRI (Yoruban in Ibadan, Nigeria) with 85 SNPs were analyzed, and 47 individuals had homozygosity throughout the SIRT1 gene. Statistical analysis for comparing the homozygosity rate of the SIRT1 gene between Hapmap data and our data was performed using a binomial test.
SIRT1 domain analysis
SIRT1 domain analysis was performed using MyHits Motif Scanner (www.myhits.isb-sib.ch/cgi-bin/motif_scan). The full-length human SIRT1 protein sequence (isoform a; NP_036370.2 GI:7657575) was used as the query. All available databases were used in the search.
Recombinant protein purification
Full-length human SIRT1 cDNA was cloned into a proprietary pet21MDX7 expression vector (a kind gift from Sirtris Pharmaceuticals). The expression construct was transformed into BL21 (DE3) bacteria. Bacteria were cultured at 37°C until an O.D. of 0.6 was reached, at which time IPTG was added to a final concentration of 0.5 mM. Cultures were then transferred to a 16°C incubator and grown overnight for an additional 16 h prior to harvest. His-tagged SIRT1 and yPNC1 protein purification were performed as previously described (Hubbard et al,. 2012, submitted).
BIOMOL assay
The Fleur de Lys BIOMOL assay was performed in accordance with the manufacturers’ instructions. The final B-NAD+ concentration in each reaction was 100 µM, and the final FdL-p53 substrate concentration used was 50 µM. Five µg of recombinant His-hSIRT1 was added to each reaction, and developer was added following a 1 h incubation at 37°C. All reactions were normalized to control reactions in the absence of B-NAD (Ffinal = F+NAD - F-NAD).
PNC1-OPT assay
The PNC1-OPT assay was performed as previously described.Citation35 A native H3K9-based peptide was used as the substrate [TARK(ac)STG], and the final B-NAD and substrate concentrations were 100 µM and 50 µM, respectively. Five µg of recombinant His-hSIRT1 was added to each reaction, and 1 µg of yPNC1 was added to each reaction. OPT developer solution was added following a 1 h incubation at 37°C. All reactions were normalized to control reactions in the absence of B-NAD (Ffinal = F+NAD - F-NAD).
Telomerase repeat amplification protocol (TRAP) assay
TRAP assays were conducted as previously described with minor modification.Citation36 Briefly, cells were extracted using TRAP lysis buffer 10 mM TRIS-HCl, pH 7.5, 0.5% 3-[(3-cholamidopropyl)-dimethyl-ammonio]-1-propane sulfonate (CHAPS), 1 mM MgCl2, 1 mM EGTA, 5 mM β-mercaptoethanol, 10% Glycerol, proteinase inhibitor cocktail (Calbiochem), RNase inhibitor (Invitrogen) in diethyl pyrocarbonate (DEPC)-treated water and aliquots of 500 ng total protein were subjected to telomerase-mediated extension of TS nucleotide (10 pmole) in TRAP reaction buffer (20 mM TRIS-HCl, pH 8.3, 63 mM KCl, 1.5 mM MgCl2, 1 mM EGTA, 0.05% Tween-20, 0.1 mM dNTP). The telomerase-mediated extension was measured by quantitative PCR (Q-PCR) analysis using SYBR Green Master Mix (Bio-Rad) and following oligonucleotides: 5′-AATCCGTCGAGCAGAGTT-3′ (TS) and 5′-GCGCGGCTTACCCTTACCCTTACCCTAACC-3′ (ACX) for extended telomere repeats, 5′-ATCGCTTCTCGGCCTTTT-3′ (NT) and 5′-AATCCGTCGAGCAGAGTTAAAAGGCCGAGAAGCGAT-3′ (TSNT) for internal telomerase assay standard (ITAS).
Transfection and western blot
Transfections of pcDNA3.1 vector, Flag-tagged wild-type SIRT1 and Flag-tagged mutant SIRT1 constructs were performed with X-tremeGENE HP DNA transfection reagent (Roche Applied Science) according to the manufacturer’s protocol. In brief, HEK293 cells were seeded in a 6-well plate at 4 × 105/ml concentration, and transfections were performed after 24 h of growth with a 3:1 ratio of Reagent to plasmid DNA. After 48 h of transfection, cells were collected and western blot was performed with the following antibodies; Rictor (#2114, Cell Signaling), anti-FLAG M2-Peroxidase (HRP) conjugated (A8592, Sigma) and anti-α-tubulin (T9026, Sigma).
Additional material
Download Zip (105.2 KB)Acknowledgments
We would like to thank the Genomics Shared Facility at the Albert Einstein College of Medicine for their assistance with the Microsatellite analysis. We also thank Dr. Mieke Schutte for providing the DNAs for all the breast cancer cell lines and Dr. Matthew Levy for providing the HeLa cell line. All the amplified products were sequenced from Macrogen USA. This work was funded by NIH grant AG024391, AG027734 and AG17242. Y.S. is the recipient of a Glenn Award for Research in Biological Mechanisms of Aging. B.P.H. was supported by an NSERC PGS-D Fellowship.
Disclosure of Potential Conflicts of Interest
No potential conflicts of interest were disclosed.
References
- Kaeberlein M, McVey M, Guarente L. The SIR2/3/4 complex and SIR2 alone promote longevity in Saccharomyces cerevisiae by two different mechanisms. Genes Dev 1999; 13:2570 - 80; http://dx.doi.org/10.1101/gad.13.19.2570; PMID: 10521401
- Tissenbaum HA, Guarente L. Increased dosage of a sir-2 gene extends lifespan in Caenorhabditis elegans. Nature 2001; 410:227 - 30; http://dx.doi.org/10.1038/35065638; PMID: 11242085
- Rogina B, Helfand SL. Sir2 mediates longevity in the fly through a pathway related to calorie restriction. Proc Natl Acad Sci USA 2004; 101:15998 - 6003; http://dx.doi.org/10.1073/pnas.0404184101; PMID: 15520384
- Haigis MC, Guarente LP. Mammalian sirtuins--emerging roles in physiology, aging, and calorie restriction. Genes Dev 2006; 20:2913 - 21; http://dx.doi.org/10.1101/gad.1467506; PMID: 17079682
- Fusco S, Maulucci G, Pani G. Sirt1: Def-eating senescence?. Cell Cycle 2012; 11:4135 - 46; http://dx.doi.org/10.4161/cc.22074; PMID: 22983125
- Guarente L, Franklin H. Franklin H. Epstein Lecture: Sirtuins, aging, and medicine. N Engl J Med 2011; 364:2235 - 44; http://dx.doi.org/10.1056/NEJMra1100831; PMID: 21651395
- Lavu S, Boss O, Elliott PJ, Lambert PD. Sirtuins--novel therapeutic targets to treat age-associated diseases. Nat Rev Drug Discov 2008; 7:841 - 53; http://dx.doi.org/10.1038/nrd2665; PMID: 18827827
- Deng CX. SIRT1, is it a tumor promoter or tumor suppressor?. Int J Biol Sci 2009; 5:147 - 52; http://dx.doi.org/10.7150/ijbs.5.147; PMID: 19173036
- Calvanese V, Fraga MF. SirT1 brings stemness closer to cancer and aging. Aging (Albany NY) 2011; 3:162 - 7; PMID: 21307403
- Huffman DM, Grizzle WE, Bamman MM, Kim JS, Eltoum IA, Elgavish A, et al. SIRT1 is significantly elevated in mouse and human prostate cancer. Cancer Res 2007; 67:6612 - 8; http://dx.doi.org/10.1158/0008-5472.CAN-07-0085; PMID: 17638871
- Stünkel W, Peh BK, Tan YC, Nayagam VM, Wang X, Salto-Tellez M, et al. Function of the SIRT1 protein deacetylase in cancer. Biotechnol J 2007; 2:1360 - 8; http://dx.doi.org/10.1002/biot.200700087; PMID: 17806102
- Hida Y, Kubo Y, Murao K, Arase S. Strong expression of a longevity-related protein, SIRT1, in Bowen’s disease. Arch Dermatol Res 2007; 299:103 - 6; http://dx.doi.org/10.1007/s00403-006-0725-6; PMID: 17180656
- Bradbury CA, Khanim FL, Hayden R, Bunce CM, White DA, Drayson MT, et al. Histone deacetylases in acute myeloid leukaemia show a distinctive pattern of expression that changes selectively in response to deacetylase inhibitors. Leukemia 2005; 19:1751 - 9; http://dx.doi.org/10.1038/sj.leu.2403910; PMID: 16121216
- Kriegl L, Vieth M, Kirchner T, Menssen A. Up-regulation of c-MYC and SIRT1 expression correlates with malignant transformation in the serrated route to colorectal cancer. Oncotarget 2012; 3:1182 - 93; PMID: 23045412
- Chen WY, Wang DH, Yen RC, Luo J, Gu W, Baylin SB. Tumor suppressor HIC1 directly regulates SIRT1 to modulate p53-dependent DNA-damage responses. Cell 2005; 123:437 - 48; http://dx.doi.org/10.1016/j.cell.2005.08.011; PMID: 16269335
- Lain S, Hollick JJ, Campbell J, Staples OD, Higgins M, Aoubala M, et al. Discovery, in vivo activity, and mechanism of action of a small-molecule p53 activator. Cancer Cell 2008; 13:454 - 63; http://dx.doi.org/10.1016/j.ccr.2008.03.004; PMID: 18455128
- Brunet A, Sweeney LB, Sturgill JF, Chua KF, Greer PL, Lin Y, et al. Stress-dependent regulation of FOXO transcription factors by the SIRT1 deacetylase. Science 2004; 303:2011 - 5; http://dx.doi.org/10.1126/science.1094637; PMID: 14976264
- Herranz D, Serrano M. SIRT1: recent lessons from mouse models. Nat Rev Cancer 2010; 10:819 - 23; http://dx.doi.org/10.1038/nrc2962; PMID: 21102633
- Wang RH, Sengupta K, Li C, Kim HS, Cao L, Xiao C, et al. Impaired DNA damage response, genome instability, and tumorigenesis in SIRT1 mutant mice. Cancer Cell 2008; 14:312 - 23; http://dx.doi.org/10.1016/j.ccr.2008.09.001; PMID: 18835033
- Oberdoerffer P, Michan S, McVay M, Mostoslavsky R, Vann J, Park SK, et al. SIRT1 redistribution on chromatin promotes genomic stability but alters gene expression during aging. Cell 2008; 135:907 - 18; http://dx.doi.org/10.1016/j.cell.2008.10.025; PMID: 19041753
- Firestein R, Blander G, Michan S, Oberdoerffer P, Ogino S, Campbell J, et al. The SIRT1 deacetylase suppresses intestinal tumorigenesis and colon cancer growth. PLoS One 2008; 3:e2020; http://dx.doi.org/10.1371/journal.pone.0002020; PMID: 18414679
- Harkes IC, Elstrodt F, Dinjens WN, Molier M, Klijn JG, Berns EM, et al. Allelotype of 28 human breast cancer cell lines and xenografts. Br J Cancer 2003; 89:2289 - 92; http://dx.doi.org/10.1038/sj.bjc.6601448; PMID: 14676808
- Palacios JA, Herranz D, De Bonis ML, Velasco S, Serrano M, Blasco MA. SIRT1 contributes to telomere maintenance and augments global homologous recombination. J Cell Biol 2010; 191:1299 - 313; http://dx.doi.org/10.1083/jcb.201005160; PMID: 21187328
- Wang RH, Kim HS, Xiao C, Xu X, Gavrilova O, Deng CX. Hepatic Sirt1 deficiency in mice impairs mTorc2/Akt signaling and results in hyperglycemia, oxidative damage, and insulin resistance. J Clin Invest 2011; 121:4477 - 90; http://dx.doi.org/10.1172/JCI46243; PMID: 21965330
- Fang Y, Nicholl MB. Sirtuin 1 in malignant transformation: friend or foe?. Cancer Lett 2011; 306:10 - 4; http://dx.doi.org/10.1016/j.canlet.2011.02.019; PMID: 21414717
- Li K, Luo J. The role of SIRT1 in tumorigenesis. N Am J Med Sci (Boston) 2011; 4:104 - 6; http://dx.doi.org/10.7156/v4i2p104; PMID: 22180829
- Wang RH, Zheng Y, Kim HS, Xu X, Cao L, Luhasen T, et al. Interplay among BRCA1, SIRT1, and Survivin during BRCA1-associated tumorigenesis. Mol Cell 2008; 32:11 - 20; http://dx.doi.org/10.1016/j.molcel.2008.09.011; PMID: 18851829
- Kabra N, Li Z, Chen L, Li B, Zhang X, Wang C, et al. SirT1 is an inhibitor of proliferation and tumor formation in colon cancer. J Biol Chem 2009; 284:18210 - 7; http://dx.doi.org/10.1074/jbc.M109.000034; PMID: 19433578
- Elstrodt F, Hollestelle A, Nagel JH, Gorin M, Wasielewski M, van den Ouweland A, et al. BRCA1 mutation analysis of 41 human breast cancer cell lines reveals three new deleterious mutants. Cancer Res 2006; 66:41 - 5; http://dx.doi.org/10.1158/0008-5472.CAN-05-2853; PMID: 16397213
- Yang Y, Fu W, Chen J, Olashaw N, Zhang X, Nicosia SV, et al. SIRT1 sumoylation regulates its deacetylase activity and cellular response to genotoxic stress. Nat Cell Biol 2007; 9:1253 - 62; http://dx.doi.org/10.1038/ncb1645; PMID: 17934453
- Yeung F, Hoberg JE, Ramsey CS, Keller MD, Jones DR, Frye RA, et al. Modulation of NF-kappaB-dependent transcription and cell survival by the SIRT1 deacetylase. EMBO J 2004; 23:2369 - 80; http://dx.doi.org/10.1038/sj.emboj.7600244; PMID: 15152190
- Yuan J, Minter-Dykhouse K, Lou Z. A c-Myc-SIRT1 feedback loop regulates cell growth and transformation. J Cell Biol 2009; 185:203 - 11; http://dx.doi.org/10.1083/jcb.200809167; PMID: 19364925
- Sawada M, Sun W, Hayes P, Leskov K, Boothman DA, Matsuyama S. Ku70 suppresses the apoptotic translocation of Bax to mitochondria. Nat Cell Biol 2003; 5:320 - 9; http://dx.doi.org/10.1038/ncb950; PMID: 12652308
- Narala SR, Allsopp RC, Wells TB, Zhang G, Prasad P, Coussens MJ, et al. SIRT1 acts as a nutrient-sensitive growth suppressor and its loss is associated with increased AMPK and telomerase activity. Mol Biol Cell 2008; 19:1210 - 9; http://dx.doi.org/10.1091/mbc.E07-09-0965; PMID: 18184747
- Yang Y, Hubbard BP, Sinclair DA, Tong Q. Characterization of murine SIRT3 transcript variants and corresponding protein products. J Cell Biochem 2010; 111:1051 - 8; http://dx.doi.org/10.1002/jcb.22795; PMID: 20677216
- Herbert BS, Hochreiter AE, Wright WE, Shay JW. Nonradioactive detection of telomerase activity using the telomeric repeat amplification protocol. Nat Protoc 2006; 1:1583 - 90; http://dx.doi.org/10.1038/nprot.2006.239; PMID: 17406450
- den Dunnen JT, Antonarakis SE. Mutation nomenclature extensions and suggestions to describe complex mutations: a discussion. Hum Mutat 2000; 15:7 - 12; http://dx.doi.org/10.1002/(SICI)1098-1004(200001)15:1<7::AID-HUMU4>3.0.CO;2-N; PMID: 10612815