Abstract
Growth hormone (GH) overexpression throughout life in transgenic mice is associated with the development of liver tumors at old ages. The preneoplastic pathology observed in the liver of young adult GH-overexpressing mice is similar to that present in humans at high risk of hepatic cancer. To elucidate the molecular pathogenesis underlying the pro-oncogenic liver pathology induced by prolonged exposure to elevated GH levels, the activation and expression of several components of signal transduction pathways that have been implicated in hepatocellular carcinogenesis were evaluated in the liver of young adult GH-transgenic mice. In addition, males and females were analyzed in parallel in order to evaluate sexual dimorphism. Transgenic mice from both sexes exhibited hepatocyte hypertrophy with enlarged nuclear size and exacerbated hepatocellular proliferation, which were higher in males. Dysregulation of several oncogenic pathways was observed in the liver of GH-overexpressing transgenic mice. Many signaling mediators and effectors were upregulated in transgenic mice compared with normal controls, including Akt2, NFκB, GSK3β, β-catenin, cyclin D1, cyclin E, c-myc, c-jun and c-fos. The molecular alterations described did not exhibit sexual dimorphism in transgenic mice except for higher gene expression and nuclear localization of cyclin D1 in males. We conclude that prolonged exposure to GH induces in the liver alterations in signaling pathways involved in cell growth, proliferation and survival that resemble those found in many human tumors.
Introduction
Growth hormone (GH) is a major regulator of body growth and metabolism. It acts either directly on target cells by binding to its membrane receptor, or indirectly by inducing the synthesis of the insulin-like growth factor 1 (IGF1), which mediates many of GH actions. At the cellular level, growth hormone modulates several processes, including proliferation, differentiation, survival and motility. The main intracellular signaling pathways activated by GH include the signal transducers and activators of transcription (STATs), the MAPK kinase (MEK)/extracellularly regulated kinase (ERK) and the phosphatidylinositol 3 kinase (PI3K)/Akt pathways.Citation1-Citation3 GH also modulates the expression and activity of the epidermal growth factor receptor (EGFR), which initiates these signaling pathways as well.Citation4-Citation6
Evidence from both humans and animal models has supported a link between GH/IGF1 status and cancer risk.Citation7,Citation8 Transgenic mice overexpressing GH exhibit increased adult body size, organomegaly and elevated circulating IGF1 levels along with other endocrine and metabolic alterations.Citation9-Citation12 Particularly, these animals exhibit hepatomegaly due to hypertrophy and hyperplasia, with hepatocytes presenting large cellular and nuclear size. GH-transgenic mice display high levels of hepatocellular replication throughout lifespan, and old animals frequently develop liver tumors.Citation11-Citation18
The development of hepatocellular carcinoma (HCC) is associated with and preceded by morphological changes in the tissue known as preneoplastic lesions, in which increasingly aberrant populations of hepatocytes evolve.Citation19,Citation20 The preneoplastic pathology observed in the liver of GH overexpressing mice is similar to that present in humans at high risk of developing hepatic cancer.Citation17 GH overexpression synergistically promoted carcinogen-induced liver tumorogenesis in GH-transgenic mice by stimulating tumor cell proliferation.Citation21
The cytological alterations on hepatocytes in GH-transgenic mice are believed to be a consequence of the direct effect of GH on the liver rather than mediated by IGF1, as transgenic mice overexpressing IGF1 do not exhibit the same liver pathology.Citation11,Citation15 Moreover, GH treatment to hepatocytes in culture produced a marked heterogeneity in cell size, enlargement of nuclei, nuclear protusions and increase in the number of large nucleoli, resembling the morphological alterations observed in vivo in the liver of GH-transgenic mice, consistent with a direct effect of GH on liver cell morphology.Citation22 Elevated circulating GH levels were reported to have mitogenic and anti-apoptotic effects independent of IGF1 through activation of signaling pathways involved in cell proliferation and survival.Citation8
The molecular mechanisms implicated in hepatocellular carcinogenesis are complex, and the precise factors involved depend on the underlying disease. There is evidence of aberrant activation of several signaling cascades, including EGFR, Ras/Erk, PI3K/Akt/mammalian target of rapamycin (mTOR), HGF/c-met and Wnt/β-catenin, while the relevance of other signaling pathway as JAK/STAT and Hedgehog is currently under study.Citation23-Citation25
The general objective of this study was to elucidate the molecular pathogenesis and signal transduction pathways underlying the pro-oncogenic liver pathology induced by prolonged exposure to elevated GH levels. Our group has previously reported that female young adult transgenic mice overexpressing GH exhibit hepatic constitutive activation and/or upregulation of several signaling mediators involved in cell proliferation and survival, including STAT3, Akt, mTOR, Erk1/2, EGFR and Src.Citation6
Therefore, in the present work we aimed to determine if downstream components and effectors of these signaling pathways and other signaling cascades known to participate in the hepatocarcinogenesis process are dysregulated in the liver of young GH-transgenic mice that present preneoplastic pathology. Considering that the incidence of HCC is known to be higher in males both in humans and in mice,Citation26,Citation27 but in GH-transgenic mice no sex difference was reported,Citation21 it was important to determine whether the molecular alterations described exhibited sexual dimorphism. For that purpose, the activation and expression of several signal transduction pathways that have been implicated in hepatocellular carcinogenesis were evaluated in the liver of young adult male and female GH-transgenic mice and their normal controls to assess its possible association with the liver pathology observed in these animals.
Results
Liver weight, histological characteristics and hepatocellular proliferation
In accordance with previous publications using GH-overexpressing transgenic mouse models,Citation12,Citation13,Citation18 9-week-old PEPCK-bGH-transgenic mice used in the present work exhibited hepatomegaly, manifested by a higher liver weight than normal mice, even when corrected by the body weight. No sex differences were detected in the relative liver weight, either in normal or transgenic mice (). No macroscopic liver lesions were observed in any group at this age. The histological analysis of liver sections confirmed the expected preneoplastic morphological alterations in young adult GH-transgenic mice. In both sexes, areas of dysplasia were observed, with hepatocytes presenting large cellular and nuclear size and nuclear polymorphism, which was more pronounced in the centrolobular areas (). To evaluate hepatocyte hypertrophy, the relative cell size was estimated by counting hepatocytes per field in liver sections. Transgenic mice displayed a significant decrease in the number of hepatocytes per microscope field as compared with normal controls, indicative of hepatocyte enlargement; males presented significantly lower number of hepatocytes per field than females in both genotypes (). Transgenic mice also displayed significantly higher mean nuclear size than their normal controls () with a shift to larger nuclear areas and a broader area range in the size distribution profile (). In both genotypes, the nuclear area was greater in males than in females ().
Table 1. Body and liver weight in young adult mice overexpressing GH and normal controls
Figure 1. Histological analysis of livers from GH-overexpressing transgenic mice and normal controls. Representative photomicrographs of liver sections from normal female (NF), normal male (NM), GH-transgenic female (TF) and GH-transgenic male (TM) mice are shown. (A) H&E staining, original magnification 100×; (B) H&E staining, original magnification 400×; (C) fluorescence microscopy after Hoechst dye staining, original magnification 400×. The analysis of hepatocytes number per visual field (original magnification 400×) is displayed in (D). The analysis of the hepatocyte nuclear area is displayed in (E) mean hepatocyte nuclear area; (F and G) hepatocyte nuclear area size distribution for normal and GH-transgenice mice, respectively. Data are the mean ± SEM of nine sets of different individuals per group. Different letters (D and E) denote significant differences. Asterisks (F and G) indicate significant differences between males and females for a same nuclear area range at p < 0.05.
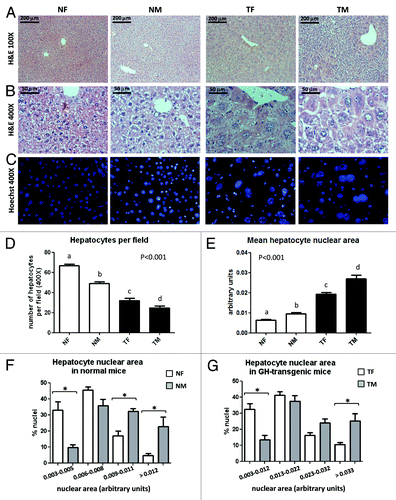
To evaluate hepatocellular proliferation, immunohistochemistry and immunoblotting analysis of the S-phase-related proliferating cell nuclear antigen (PCNA) was performed. Transgenic mice displayed a marked increase in the percentage of hepatocytes positive for nuclear labeling with PCNA compared with their normal controls, with males presenting significantly more PCNA-positive cells than females in the transgenic group (). Similar results were observed by immunoblotting analysis, although the differences were less pronounced (). Although normal males exhibited a consistent trend to lower PCNA levels than normal females, this was not statistically significant.
Figure 2. PCNA protein content and immunostaining. To evaluate hepatocellular proliferation, the expression of the proliferating cell nuclear antigen (PCNA) was assessed in the liver from young adult normal female (NF), normal male (NM), GH-transgenic female (TF) and GH-transgenic male (TM) mice. (A) Immunohistochemical staining of liver sections with anti-PCNA antibody: representative microphotographs are shown; the % of positive hepatocyte nuclei was determined in 10 high-power fields per mouse, nine animals per group were analyzed. Negative controls were performed in parallel on additional slides and incubated with PBS instead of primary antibody. (B) Liver extracts were analyzed by immunobloting with anti-PCNA antibody. Quantification was performed by scanning densitometry and expressed as % of the mean values in normal female mice. Data are the mean ± SEM of nine sets of different individuals per group (n). Different letters denote significant difference at p < 0.05. A representative result of immunoblots is shown. (C) Loading controls were performed by immunoblotting with anti-β-tubulin, anti-β-actin and anti-STAT5 antibodies and by staining the PVDF membrane with Coomasie blue. Each lane contains 40 μg of solubilized liver proteins from an individual animal.
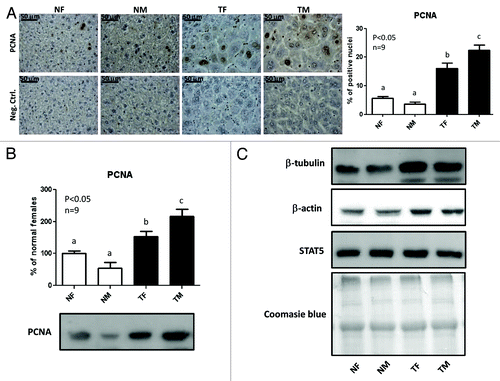
In a first attempt to check uniformity of loading in the immunoblotting assays, membranes were probed with anti-β-actin and anti-β-tubulin antibodies, but GH-transgenic mice displayed higher content of these proteins than normal mice. Changes in β-actin and β-tubulin were described in preneoplastic and tumoral cells, which accompany the structural reorganization of the cytoskeleton.Citation28,Citation29 We therefore decided to control loading homogeneity by immunoblotting with anti-STAT5, as no differences in the amount of this protein were found between adult normal and transgenic animals (), in accordance with previous reports from our.Citation6,Citation30,Citation31 Finally, after immunoblotting, PVDF membranes were stained with Coomasie Blue to assess homogeneity of protein load.
Expression and activation of STAT3, STAT5, Akt2, mTOR and EGFR
We have previously described that female adult GH-transgenic mice (4–6-mo-old) exhibited higher hepatic basal phosphorylation of STAT3, but not of STAT5, and protein overexpression of Akt, mTOR and EGFR, among other signaling mediators.Citation6 In the present work, the phosphorylation on activating residues and the expression of these signaling proteins were analyzed in 9-week-old female and male mice in parallel, to address whether the previously reported variations in the expression or activation of these signaling mediators exhibit sexual dimorphism.
Representative results of immunoblots are shown in . For each protein, the phosphorylation and protein content was determined by immunoblotting, and the phosphorylation/protein content ratio was calculated. When significant differences in the protein abundance were detected, the mRNA content was determined by qRT-PCR. The graphs shown for STAT5 (), STAT3 (), Akt (), mTOR () and EGFR () were obtained by analyzing nine different individuals per group.
Figure 3. Expression and phosphorylation of STAT5, STAT3, Akt, mTOR and EGFR in the liver of GH-overexpressing transgenic mice and normal controls. Liver extracts from young adult normal female (NF), normal male (NM), GH-transgenic female (TF) and GH-transgenic male (TM) mice were analyzed by immunoblotting to determine the phosphorylation and protein content, or by reverse-transcriptase quantitative PCR (qRT-PCR) to assess mRNA levels. (A) Representative results of immunoblots are shown. (B) STAT5 phosphorylation at Tyr694/696 (pSTAT5), protein content and phosphorylation/protein content ratio. STAT5 was also used as a control for equal loading. (C) STAT3 phosphorylation at Tyr705 (pSTAT3), protein content and phosphorylation/protein content ratio. (D) Akt phosphorylation at Ser473 (pAkt), Akt2 protein content, phosphorylation/protein content ratio and mRNA levels. (E) mTOR phosphorylation at Ser2448 (pmTOR), protein content, phosphorylation/protein content ratio and mRNA levels. (F) EGFR phosphorylation at Tyr845 (pEGFR), protein content, phosphorylation/protein content ratio and mRNA levels. Results are expressed as % of the mean values in normal female mice. Data are the mean ± SEM of nine sets of different individuals per group (n). Different letters denote significant difference at p < 0.05. NS, not significant.
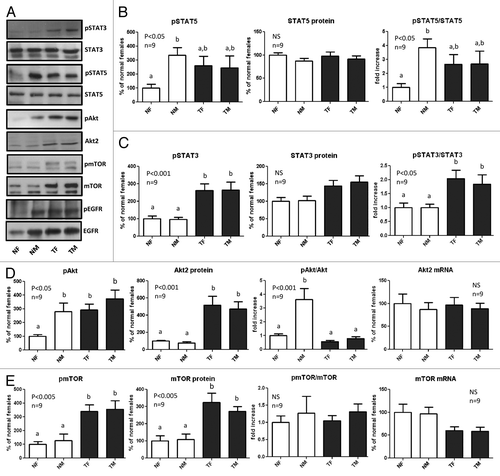
No sex differences were observed for transgenic mice in the proteins analyzed; however, sexual dimorphism was observed in normal mice for STAT5, Akt and EGFR, with males presenting higher phosphorylation levels. In the case of STAT5 and Akt, the higher phosphorylation levels observed in normal males were not related to elevated protein expression compared with normal females, indicating an increase in the relative activation of these proteins (). However, the elevated level of phosphorylated EGFR in normal males was associated with a parallel increase in the protein and mRNA content of this receptor, indicating a higher expression of EGFR in normal males compared with females ().
Transgenic mice from both sexes displayed basal phosphorylation levels of STAT5, which were in between those of normal males and females (), although these differences did not reach statistical significance. The high level of phosphorylated Akt and EGFR observed in transgenic mice was the consequence of elevated protein levels of these signaling mediators, which was not associated with higher gene expression, as the mRNA abundance was not increased in transgenic animals, in accordance with previous results reported for adult female mice.Citation6
Female and male transgenic mice displayed significantly higher phosphorylation levels of STAT3 at its activating residue, Tyr705, compared with normal mice, with slightly elevated protein levels that were not significantly different from normal controls (). Transgenic mice also exhibited higher phosphorylation of mTOR at Ser2448, which was most probably the result of elevated abundance of this protein. When statistical analysis was performed by ANOVA, no significant variation in mTOR mRNA content was found (), but when mRNA levels were compared by Student’s t test for genotype within sex, a significant reduction was detected for the transgenic groups, in line with previous reports for adult female mice.Citation6
Expression of GSK3β and β-catenin
The glycogen synthase kinase 3 (GSK3) is a Ser/Thr kinase implicated in glycogen metabolism, cell differentiation, proliferation, survival and transformation through multiple signaling pathways; it exists in two isoforms, α and β, but the β-isoform has a more established role in cell survival and viability.Citation32,Citation33 GSK3β is a component of both PI3K/Akt and Wnt/β-catenin signaling pathways, two major systems that are frequently dysregulated in liver cancer.Citation23,Citation32 Akt and other Ser/Thr kinases phosphorylate the GSK3β isoform at Ser9, inhibiting its activity and consequently stabilizing cyclin D1 and β-catenin protein levels.Citation32-Citation35 The accumulation of β-catenin in the cytoplasm promotes its translocation to the nucleus, where it activates the transcription of several oncogenes, including cyclin D1, c-Myc and c-Jun.Citation32,Citation34,Citation35
In normal mice, a higher phosphorylation of GSK3β at Ser9 was observed in males compared with females, with no differences in the protein or mRNA content (), suggesting that the relatively higher phosphorylation degree of GSK3β in normal males may be a consequence of higher activity of Akt, as reflected by the elevated phosphorylated Akt levels observed in this group. In contrast, no sex differences were detected in transgenic mice. For both sexes, GH-transgenic animals displayed elevated GSK3β protein content, accompanied by a further increase in the phosphorylation levels, even when corrected by protein content, with no variation in mRNA abundance (). In addition, the protein content of β-catenin was increased in GH-transgenic mice, with no significant differences in its mRNA abundance (), suggesting that the protein upregulation was not related to enhanced expression of β-catenin gene, but probably to higher stability of the protein. Immunohistochemistry analysis revealed a higher β-catenin staining in transgenic than in normal mice, which was mainly localized at the hepatocytes plasma membrane and cytoplasm. In normal mice, β-catenin staining was barely detected and located at the plasma membrane ().
Figure 4. Expression of GSK3β and β-catenin in the liver of GH-overexpressing transgenic mice and normal controls. Liver extracts from young adult normal female (NF), normal male (NM), GH-transgenic female (TF) and GH-transgenic male (TM) mice were analyzed by immunoblotting to determine the phosphorylation and protein content, or by reverse-transcriptase quantitative PCR (qRT-PCR) to assess mRNA levels. (A) Phosphorylation of GSK3β at Ser9 (pGSK3β). (B) GSK3β protein content. (C) Phosphorylated GSK3β levels corrected by GSK3β protein content. (D) GSK3β mRNA levels. (E) β-catenin protein content. (F) β-catenin mRNA levels. Results are expressed as % of the mean values in normal female mice. Data are the mean ± SEM of nine sets of different individuals per group (n). Different letters denote significant difference at p < 0.05. NS: not significant. (G) Representative results of immunoblots are shown. Reprobing with anti-STAT5 antibody demonstrated similar protein loading in all lanes. (H) Immunohistochemical staining of liver sections with anti-β-catenin: representative microphotographs are shown. Negative controls were performed in parallel on additional slides and incubated with PBS instead of primary antibody.
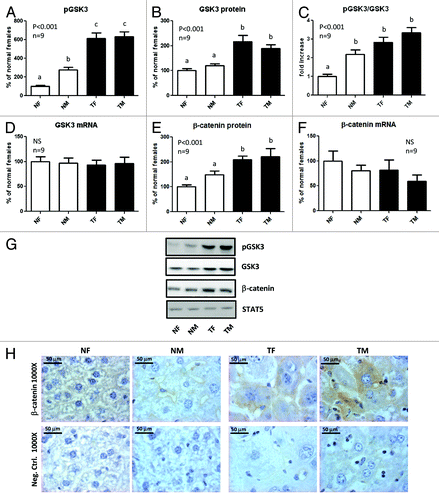
Phosphorylation and expression of NFκB and CREB
The transcription factor nuclear factor kappa B (NFκB) family regulates the expression of a large number of genes that play critical roles in cell proliferation, survival, tumorigenesis, inflammation and immune response. In the liver, NFκB plays an essential role in the regulation of inflammatory pathways, hepatic homeostasis and wound-healing processes.Citation36 The phosphorylation and expression of p65 (RelA), a member of the NFκB family, was determined in the liver of GH-transgenic mice and normal controls. Transgenic mice exhibited higher protein and mRNA abundance of p65 NFκB (RelA) with a parallel increase in its phosphorylation at Ser536 (), which enhances its transcriptional activity.Citation37
Figure 5. Expression and phosphorylation of NFκB p65 (RelA) and CREB in the liver of GH-overexpressing transgenic mice and normal controls. Liver extracts from young adult normal female (NF), normal male (NM), GH-transgenic female (TF) and GH-transgenic male (TM) mice were analyzed by immunoblotting to determine the phosphorylation and protein content, or by reverse-transcriptase quantitative PCR (qRT-PCR) to assess mRNA levels. (A) NFκB p65 (Rel A) phosphorylation at Ser536 (pNFκB), protein content, phosphorylation/protein content ratio and mRNA levels. (B) CREB phosphorylation at Ser133 (pCREB), protein content, phosphorylation/protein content ratio and mRNA levels. (C) Representative results of immunoblots are shown. Reprobing with anti-STAT5 antibody demonstrated similar protein loading in all lanes. Results are expressed as % of the mean values in normal female mice. Data are the mean ± SEM nine sets of different individuals per group (n). Different letters denote significant difference at p < 0.05. NS, not significant.
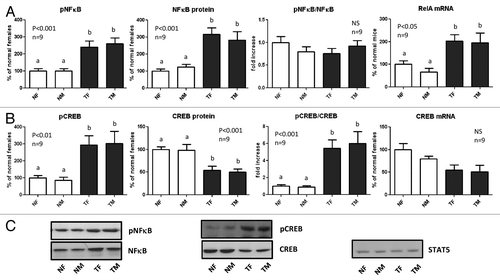
The transcription factor c-AMP-response element binding protein (CREB) is phosphorylated at Ser133 by various kinases, including Akt, which increase its transcriptional activation and induce the expression of survival genes.Citation38 Transgenic mice displayed significantly lower protein abundance of CREB, but its phosphorylation at Ser133 was markedly increased compared with normal controls (). CREB mRNA exhibited lower levels in GH-transgenic mice than in normal controls, but this was not statistically significant. No sex differences were detected in the protein content, mRNA levels or in the phosphorylation of NFκB and CREB, either in normal or transgenic mice.
Expression of proto-oncogenes involved in cell cycle progression
The expression of c-myc, c-jun and c-fos, immediate early genes involved in the regulation of the priming phase of the cell cycle, is induced through the signaling pathways that are exacerbated in the liver of GH-transgenic mice. These oncogenes were reported to participate in the progress of hepatocarcinogenesis.Citation23,Citation39,Citation40 Therefore, the expression of these proteins was determined in the liver of GH-transgenic mice and normal controls. The protein and mRNA content of c-myc, c-jun and c-fos was analyzed by western blotting and quantitative RT-PCR, respectively. Representative results of immunoblots are shown in . Higher mRNA and protein levels were observed for the three proto-oncogenes in GH-transgenic mice compared with normal controls, with no sex differences ().
Figure 6. Expression of proto-oncogenes in the liver of GH-overexpressing transgenic mice and normal controls. Liver extracts from young adult normal female (NF), normal male (NM), GH-transgenic female (TF) and GH-transgenic male (TM) mice were analyzed by immunoblotting to determine protein content and by reverse-transcriptase quantitative PCR (qRT-PCR) to assess mRNA levels. Results are expressed as % of the mean values in normal female mice. (A) Representative results of immunoblots are shown. STAT5 antibody was used to control equal protein loading in all lanes. (B) c-Fos protein and mRNA levels. (C) c-Jun protein and mRNA levels. (D) c-Myc protein and mRNA levels. (E) Cyclin E protein and mRNA levels. (F) Cyclin D1 protein and mRNA levels. (G) Immunohistochemical staining of liver sections with anti-cyclin D1 antibody: representative microphotographs are shown; the % of positive hepatocyte nuclei was determined in 10 high-power fields per mouse. Black arrows show representative positive nuclei. Negative controls were performed in parallel on additional slides and incubated with PBS instead of primary antibody. Data are the mean ± SEM of nine sets of different individuals per group (n). Different letters denote significant difference at p < 0.05. NS, not significant.
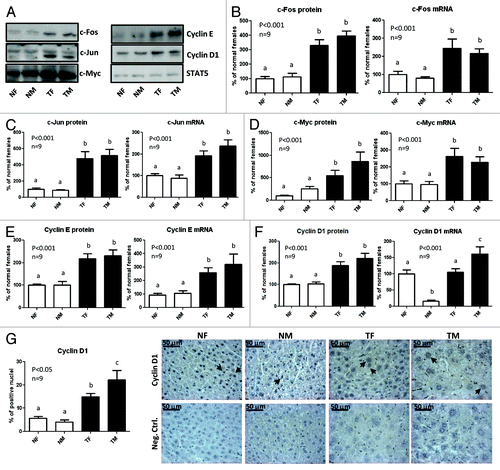
The cyclins D1 and E facilitate the transition from G1 to S phase of the cell cycle, and their dysregulation has been associated with cancer.Citation35,Citation41 Transgenic mice exhibited higher cyclin E protein levels than normal controls, associated with elevated mRNA levels, which did not show sexual dimorphism (). The protein content of cyclin D1 was also higher in GH-transgenic mice compared with normal controls, with no significant sex differences (). In contrast, sexual dimorphism was observed in the mRNA content of cyclin D1, which was markedly low in normal male mice, and high in transgenic male mice; hence, transgenic mice displayed higher cyclin D1 mRNA levels than normal controls only in males (). As results obtained for cyclin D1 by western blotting differed from those obtained by quantitative RT-PCR, nuclear localization of cyclin D1 was assessed by immunohistochemistry (). In both sexes, transgenic mice exhibited a higher nuclear immunostaining than normal controls, in agreement with results obtained by western blotting. The immunohistochemistry analysis also showed an increase in the number of cyclin D1-positive nuclei in transgenic males compared with females. Likewise, in normal males the proportion of positive nuclei was slightly lower than in females, although this difference was not statistically significant.
Molecular alterations in the liver of transgenic mice overexpressing growth hormone-releasing hormone (GHRH)
In order to corroborate that the molecular changes observed in the liver of GH-transgenic mice were a consequence of the elevated GH circulating levels and not the result of the hepatic local expression of the transgene, as occurs in PEPCK-bGH transgenic mice used, another line of mice with chronic GH excess was studied, Mt-GHRH transgenic mice, which exhibit enhanced production of the endogenous pituitary GH.
The phosphorylation and/or protein expression of signaling molecules that were found to be altered in the PEPCK-bGH transgenic line were determined in young female GHRH-transgenic mice.
We have previously reported that GHRH-transgenic mice exhibit upregulation of Akt, mTOR and EGFR, and high phosphorylation levels of STAT3.Citation6 In the present work, we show that GHRH- transgenic mice also exhibit similar alterations as those observed for PEPCK-bGH transgenic mice in other signaling molecules known to participate in the hepatocarcinogenesis process. Compared with normal controls, GHRH-transgenic mice displayed lower CREB protein level, elevated protein abundance of GSK3β and NFκB with a parallel increase in their phosphorylation levels, and higher protein content of β-catenin, cyclin D1, cyclin E, c-Myc, c-Jun and c-Fos ().
Figure 7. Molecular alterations in the liver of transgenic mice overexpressing growth hormone-releasing hormone (GHRH). Immunoblotting analysis of liver extracts from female 3–5-mo-old GHRH-transgenic mice (T) and their normal controls (N). (A) Results are expressed as % of the mean values in normal female mice. Quantification was performed by optical densitometry. Data are the mean ± SEM of five subsets of different individuals. Different letters denote significant difference at p < 0.05. (B) Representative immunoblots are shown.
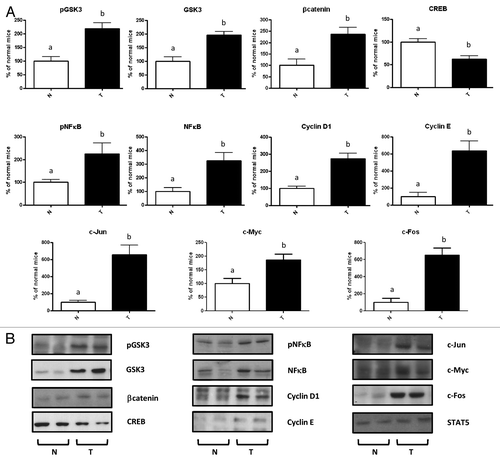
Discussion
To elucidate the molecular mechanisms involved in the preneoplastic morphological alterations in the liver of young adult transgenic mice overexpressing GH, signaling pathways known to participate in the hepatocarcinogenesis process were assessed. In a previous article we reported that female transgenic mice overexpressing GH exhibit hepatic constitutive activation and/or upregulation of several signaling mediators, including STAT3, Akt, mTOR, Erk1/2, EGFR and Src.Citation6 In the present work, we evaluated the expression and activation of downstream signaling mediators and effectors of these and other signaling pathways, including NFκB, CREB, GSK3β, β-catenin, c-Myc, c-Jun, c-Fos, cyclin D1 and cyclin E. In addition, we assessed whether sexual dimorphism was observed, as in normal mice, tumor incidence is known to be higher in males, but no sex differences were reported in GH-transgenic mice.Citation16,Citation21
The liver is a sexually dimorphic organ, with gender differences detected in the mitochondrial function, metabolic enzymes, lipid composition of the cellular membranes, immune system, gene expression and cancer incidence.Citation26,Citation27,Citation42,Citation43 The hepatic expression of many of the sex-specific genes is regulated by the GH-induced STAT5 pathway. Growth hormone exerts sex-dependent effects on the liver in many species, including rats, mice and humans through its sexually dimorphic temporal pattern of pituitary secretion, which differently activates intracellular signaling pathways and induces sex-specific gene transcription.Citation42-Citation44 In rodents it is well-established that GH is secreted by the pituitary in a pulsatile manner, which is highly episodic in males, whereas females present more frequent pulses, attaining a near-continuous pattern in the rat.Citation43,Citation45,Citation46 The activity of the transcription factor STAT5 in liver is determined by GH secretory patterns, with males presenting intermittent pulses of high STAT5 activation followed by periods of undetectable levels, whereas in females, STAT5 activation is lower and persistent.Citation43-Citation45,Citation47
In the current work, we show that normal male mice exhibited higher hepatocyte nuclear and cellular size, but cell proliferation was not increased. Sexual dimorphism was also observed in some of the molecular signaling mediators under study in the normal group, with males exhibiting upregulation of EGFR mRNA and protein levels and higher content of phosphorylated STAT5, Akt and GSK3β. The higher expression of EGFR in males is in accordance with previous reports on the modulation of EGFR expression in the liver by GH secretory profile showing that the pulsatile pattern typical of male rodents was more effective in inducing EGFR mRNA levels and EGF binding than the more continuous feminine pattern.Citation4,Citation5 Results of increased mean STAT5 phosphorylation levels in male mice compared with female controls are in line with a recent report from our group in Swiss-Webster mice of the same age as the animals used for the present work, and are most probably a direct consequence of sex-specific GH secretion patterns.Citation48 As GSK3β is a substrate of Akt, the elevated phosphorylation of GSK3β at Ser9 found in normal males is probably a result of the higher activation of Akt, reflected by enhanced phosphorylation of Akt at Ser473 in normal males compared with females. However, a similar pattern was not detected for other downstream targets of Akt, such as mTOR, suggestive of differential regulation of signaling pathways downstream Akt, in line with previous observations.Citation49 The exacerbated activation of Akt found in normal males compared with females may be related to the higher hepatocellular size in males. Signaling through the PI3K/Akt pathway is known to play an important role in hepatocyte cell growth, and it was suggested that Akt contributes to liver regeneration mainly by inducing cell size growth rather than cell proliferation.Citation50,Citation51 Whether the reported sex differences in normal mice liver also contribute to the higher incidence of HCC in males remains to be elucidated.
In line with previous publications,Citation12,Citation13,Citation16,Citation18 young adult GH-overexpressing transgenic mice from both sexes exhibit hepatomegaly, hepatocyte hypertrophy with enlarged nuclear size and exacerbated hepatocellular proliferation compared with normal controls. Interestingly, sexual dimorphism was also observed in the hepatocytes in the transgenic group. The results of enlarged hepatocyte cell and nuclear size and proliferation in transgenic males compared with females are unexpected, as it had been previously reported that no significant differences between male and female transgenic mice could be found in terms of tumor incidence and progression.Citation16 Based on those observations, authors decided to pool both sexes of GH-transgenic mice in subsequent studies,Citation17,Citation21 and therefore subtle differences between male and female GH-transgenic mice may have been undetected. The expression and activation of several of the signaling mediators evaluated was exacerbated in transgenic mice compared with normal controls, but no sex differences were detected within the transgenic group, except for higher mRNA levels and nuclear localization for cyclin D1 in males.
In contrast to our previous findings in 3–6-mo-old females, where basal phosphorylation levels of STAT5 were similar in normal and transgenic animals,Citation6,Citation30 in the current work, 9-week-old transgenic mice displayed a clear tendency to higher basal phosphorylation levels of STAT5 when values are compared with those of normal females. This difference did not reach statistical significance when analyzed by ANOVA but was significant when Student’s t test was performed for female mice only. Even when pSTAT5 levels may be higher in transgenic mice than in normal controls for females, this is not the case for males, which exhibit slightly higher STAT5 phosphorylation levels than transgenic mice. These results seem to contradict a recent report, in which liver STAT5 was proposed to be a major contributor to the pathology observed in GH-transgenic mice, as its loss reversed most of the alterations induced by persistently high GH levels, including increased hepatocyte size and turnover.Citation52 It is possible that tyrosine phosphorylation levels of this protein in whole tissue homogenates are not a direct reflection of the effective activity of STAT5 in GH-transgenic mice, as other factors are involved in its transcriptional activation.
In accordance with our previous report,Citation6 in females GH-transgenic mice displayed higher protein abundance of EGFR than normal controls. However, in males, the protein content of EGFR was not increased in transgenic mice, as normal males exhibited high EGFR levels similar to those observed in the transgenic groups. Our results suggest that the mechanism underlying the increase in EGFR protein levels compared with normal females is different for normal males and for transgenic mice; as in the first case, the concomitant increase in mRNA levels is indicative of higher gene expression, while in the case of transgenic mice, no variations in mRNA levels were detected, indicative of a higher stability of EGFR protein. Autocrine signals as EGFR ligands are known to exert a protective role in the liver; however, EGFR may also activate antiapoptotic signals.Citation23,Citation53 In fact, EGF acts as a liver mitogen, and EGFR is involved in the pathogenesis and progression of different carcinoma types, including HCC.Citation54
The Ser/Thr kinase Akt is a crucial regulator of cellular processes, including proliferation, differentiation, apoptosis and metabolism, and disruption of normal Akt signaling was reported in several human cancers, including HCC.Citation23,Citation34,Citation38 Akt family comprises three isoforms, known as Akt1, Akt2 and Akt3, all of which contain two regulatory sites, Thr308 and Ser473.Citation38 In the present work, the protein content of the isoform Akt2 was determined, and it was found to be elevated in the liver of GH-transgenic mice, with no differences in its mRNA levels. A parallel increment in the phophorylation of the activating residue Ser473 was also found. This is of interest since Akt2 expression was reported to be increased in HCC and to correlate with the progression of the disease,Citation55 and therefore its dysregulation may constitute a pivotal step in the prooncogenic process in GH-transgenic mice liver. In fact, many downstream mediators or signaling pathways that interplay with Akt, as mTOR and GSK3, were altered in transgenic mice overexpressing GH.
The glycogen synthase kinase 3β is known to participate in many cellular processes, including differentiation, proliferation and transformation.Citation32,Citation33,Citation35 GSK3β is involved in the β-catenin signaling pathway, which is believed to play a role in the neoplastic progression of hepatic cells.Citation56,Citation57 A high percentage of human hepatocellular carcinomas show high levels of β-catenin, usually located at the cytoplasm or nucleus rather than at the plasma membrane.Citation57,Citation58 β-catenin cytoplasmic content is normally kept low by phosphorylation mediated by GSK3, which is part of an inactivating complex that targets β-catenin to proteasome degradation. When GSK3 is inhibited, β-catenin accumulates in the cytoplasm, translocates to the nucleus and acts as a coactivator of transcription factors involved in cell proliferation, survival and angiogenesis.Citation33,Citation35 In the present work, we describe that GH-transgenic mice displayed increased protein content of β-catenin, without an increase in its mRNA levels, concomitant with elevated levels of GSK3β phosphorylated at an inactivating residue. Elevated levels of inactive GSK3β were reported in hepatocellular carcinoma harboring β-catenin accumulation.Citation57-Citation59 These results suggest that the upregulation of β-catenin is due to increased protein stability rather than higher gene expression. In accordance with the exacerbation of β-catenin signaling, the expression of the β-catenin oncogenic target genes cyclin D1, c-myc and c-jun was increased in transgenic mice liver. However, the expression of these genes is modulated by additional signaling pathways.
Cyclin D1 is frequently overexpressed in many human cancers, including HCC.Citation35,Citation60,Citation58 In addition to its role in cell cycle progression, cyclin D1 promotes cell growth in hepatocytes.Citation61,Citation62 Akt modulates cyclin D1 levels by multiple and complex mechanisms. In addition to its action on β-catenin, GSK3β directly phosphorylates cyclin D1, stimulating its proteolysis.Citation34,Citation35 Therefore, Akt mediated phosphorylation of GSK3β at Ser9, which inactivates it, would result in the accumulation of β-catenin and cyclin D1. Moreover, mTOR is known to promote the translation of cyclin D1 mRNA via direct phosphorylation of substrates that control mRNA translation and by an additional mechanism that would involve the transcription factor CREB.Citation34 In addition, cyclin D1 transcription is induced by NFκB.Citation63 Therefore, the elevated cyclin D1 levels in GH-transgenic mice liver are probably the result of the multiple alterations detected in different signaling pathways downstream Akt.
Altered expression of the protooncogenes c-myc, c-jun and c-fos is a common phenomenon in carcinogenesis. The transcription factor c-Myc is involved in the regulation of cellular proliferation, growth and apoptosis, and its deregulated expression has been associated with the development of tumors, including HCC.Citation64 The ability of c-Myc to induce hepatocyte proliferation or growth in mice is dependent on the age of the host. c-Myc overexpression in the liver induced marked cell proliferation and immediate onset of neoplasia in neonatal mice, while in adults it induced increased nuclear and cellular growth rather than cell proliferation, with the appearance of malignant transformation after a prolonged latency period.Citation65 The activator protein-1 (AP1) complex is a transcription factor formed principally by Jun homodimers or Jun-Fos heterodimers, which regulates the expression of multiple genes involved in cell proliferation, differentiation and apoptosis and is believed to play a major role in human oncogenesis.Citation66 c-Jun is recognized as a major regulator of hepatocyte proliferation and survival during liver development and regeneration.Citation67 c-Fos is frequently overexpressed in tumors, including HCC.Citation40,Citation68 In the present work, we report enhanced expression of c-myc, c-jun and c-fos, in the liver of young adult transgenic mice overexpressing GH, suggestive of a prominent role of these protooncogenes in the preneoplastic pathology observed in this model.
The nuclear factor NFκB is involved in cell proliferation and survival, immunity and inflammation, and its dysregulation has been implicated in cancer development. In fact, constitutive activation of NFκB was found in a wide range of cancers, and it has been implicated in the association between chronic inflammation and carcinogenesis.Citation63,Citation69,Citation70 In liver, NFκB controls the fate of death or survival of the hepatocyte, and its activation would be involved in the proliferation of oncogenic cells in hepatocarcinogenesis.Citation71 NFκB induces the expression of cell cycle-related genes, including cyclin D1 and c-myc, and it was proposed to mediate GH anti-apoptotic and proliferative effects in immune cells.Citation72 In the present work, we show that NFκB is increased in GH-transgenic mice liver, which could thus contribute to the liver pathology observed in these animals. No sex differences were detected for this protein, either in normal or transgenic animals. The elevated expression of NFκB in the liver of GH-transgenic mice is in line with a recent study performed in males showing increased RelA mRNA and p65 positive inflammatory cells in GH-overexpressing mice.Citation52 Therefore, the high NFκB content observed in the liver of GH-transgenic mice is most probably the result of the hepatic inflammation present in this mouse model. NFκB is known to induce the expression of the pro-inflammatory cytokine IL6, which activates the transcription factor STAT3. In fact, NFκB and STAT3 play important roles in liver inflammation and are critical contributors to liver cancer development and progression.Citation73 In GH-transgenic mice liver, elevated levels of activated STAT3 were observed in both sexes, consistent with the reported high hepatic expression of IL6 in GH-transgenic mice.Citation52 However, the high STAT3 phosphorylation may also be the consequence of elevated levels of other growth factors and cytokines or of their receptors in GH-transgenic mice. In fact EGF and GH receptors, which are upregulated in the liver of transgenic mice, are known to activate STAT3.
The transcription factor CREB was also suggested to play a role in hepatocellular carcinoma progression.Citation74 Despite the lower expression of CREB, its phosphorylation at the activating residue Ser133 was increased in transgenic mice, suggesting a higher relative activation of this transcription factor. Interestingly, underexpression of CREB-H, a liver-specific transcription factor, has been described in tumoral endocrine cell lines.Citation75
The aforementioned molecular alterations observed in the PEPCK-bGH transgenic mice were confirmed in the Mt-GHRH transgenic animals, therefore validating the results and indicating that the described changes are due to the persistently high circulating levels of GH and not to the local expression of the hormone in the liver.
To sum up, the hepatocarcinogenesis process that arises in the setting of chronic liver injury is complex and involves multiple mechanisms. Transgenic mice overexpressing GH serve as a model for hepatocellular carcinogenesis as a result of sustained proliferative growth stimulus in the liver.Citation14 In this work, we describe that several oncogenic pathways are dysregulated in the liver of young adult GH-overexpressing transgenic mice. We present evidence that prolonged exposure to GH induces in the liver alterations in signaling pathways involved in cell growth, proliferation and survival that resemble those found in many human tumors. The hepatocellular hypertrophy and proliferation found in transgenic mice were exacerbated in males; however, the alterations observed for the examined signaling mediators and oncogenes did not present sexual dimorphism in this group, except for higher cyclin D1 in males. These results suggest that additional molecular mechanisms may account for the higher nuclear and cell size and proliferation of the hepatocytes in transgenic male mice. Whether the observed alterations are a direct effect of prolonged GH action on the liver or secondary to other endocrine or metabolic alterations remains to be determined.
Materials and Methods
Animals
Young adult transgenic mice overexpressing GH were studied; non-transgenic siblings were used as controls. All the experiments were performed in PEPCK-bGH transgenic mice, and some of the results were also confirmed in Mt-hGHRH transgenic mice. PEPCK-bGH mice containing the bovine GH (bGH) gene fused to control sequences of the rat phosphoenolpyruvatecarboxykinase (PEPCK) gene were derived from animals kindly provided by Dr T.E. Wagner and Dr J.S. Yun (Ohio University).Citation9 The hemizygous transgenic mice were derived from a founder male and were produced by mating transgenic males with normal C57BL/6 × C3H F1 hybrid females purchased from the Jackson Laboratory. Matings produced approximately equal proportion of transgenic and normal progeny. Normal siblings of transgenic mice were used as controls. Female and male 9-week-old animals were used.
Transgenic Mt-hGHRH animals were derived from animals originally produced by Dr K. Mayo and kindly provided by Dr J. Hyde.Citation76 Adult transgenic mice and their normal siblings were produced by mating hemizygous male carriers of the Mt-hGHRH gene with normal C57BL/6J × C3H/J F1 females. Female adult animals (4–6-mo-old) were used.
The mice were housed 3–5 per cage in a room with controlled light (12 h light/day) and temperature (22 ± 2°C). The animals had free access to food (Lab Diet Equation 5001; PMI Inc.) and tap water. The appropriateness of the experimental procedure, the required number of animals used and the method of acquisition were in compliance with federal and local laws and with institutional regulations.
Reagents
Polyvinylidenedifluoride (PVDF) membranes, ECL-Plus reagent and Hyperfilm were purchased from GE Healthcare. Secondary antibodies conjugated with horseradish peroxidase and antibodies anti-cyclin D1, anti-PCNA and anti-STAT5 were purchased from Santa Cruz Biotechnology Laboratories. Antibody anti-phospho-STAT5 Tyr694/696 was from Upstate Laboratories. Antibodies anti-phospho-Akt Ser473, anti-Akt2, anti-phospho-mTOR Ser2448, anti-mTOR, anti-p44/42 MAP kinase (Erk1/2), anti-phospho-p44/42 MAP kinase Thr202/Tyr204 (pErk1/2), anti-phospho-STAT3 Tyr705, anti-phospho-EGFR Tyr845, anti-EGFR, anti-phospho-NFκB p65 Ser536, anti-NFκB p65, anti-phospho-CREB Ser133, anti-CREB, anti-phospho-GSK3β Ser9, anti-GSK3β, anti-c-Jun, anti-c-Myc, anti-c-Fos, were from Cell Signaling Technology Inc. Antibody anti-STAT3 was purchased from Transduction Laboratories. Antibodies anti-cyclin E and anti-β-tubulin were from Abcam Inc., and anti-β-actin from Sigma-Aldrich. Anti-β-catenin antibody used for immunoblotting was from Cell Signaling Technology, and anti-β-catenin antibody used for immunohistochemistry was from Santa Cruz Biotechnology Laboratories. All other chemicals were of reagent grade.
Histology and immunohistochemical staining
Freshly dissected livers were fixed in 10% formalin, dehydrated and embedded in paraffin to process sections at a thickness of 5 μm. For histomorphological evaluation, hematoxylin and eosin (H&E) staining was performed using standard procedures. Nuclear morphology was also assessed by staining the tissue slides for 30 min at room temperature with Hoechst 33258 (Sigma-Aldrich) 50 ng/ml in phosphate buffered saline (PBS). After washing twice with PBS, the slides were mounted for fluorescence microscopy.
For immunostaining, deparaffinized liver sections were rehydrated and subjected to antigenic recuperation with sodium citrate (pH 6.0) at 98°C for 30 min. Endogenous peroxidase activity was blocked incubating slides on PBS containing 3% hydrogen peroxide for 30 min. Non-specific protein binding was blocked using PBS containing 1% bovine serum albumin (BSA) for 1 h and then normal horse serum for 2 h. Incubation with primary antibodies anti-PCNA (1:1,000), anti-cyclin D1 (1:200) or anti-β-catenin (1:50) was performed overnight at 4°C. Negative controls were performed in parallel in slides in which the primary antibody was replaced by PBS. Subsequently, incubations with biotin-labeled secondary antibodies followed by incubation with streptavidin-horse radish peroxidase complex were each performed for 30 min at room temperature (R.T.U. vectastain kit, Vector Laboratories). The antigen-antibody binding was visualized with diaminobenzidine (DAB) (Peroxidase substrate kit, DAB sk-4100, Vector Laboratories), and sections were counterstained with hematoxylin.
H&E and immunostained sections were observed under light microscopy using a Leica DM2000 microscope; photomicrographs were obtained using a Leica DFC400 digital camera and Leica Application Suite software (Leica Microsystems). Measurements were performed using the image analyzer ImageJ 1.45s software. The cell and nuclear size were estimated by counting the number of hepatocytes per field and the nuclear area, respectively. Only cells that were unequivocally parenchymal in origin were analyzed. Hepatocyte proliferation was determined by immunohistochemical staining for proliferating cell nuclear antigen (PCNA). Ten fields per animal were analyzed, and all hepatocytes within the field were counted. Hepatocyte proliferation was expressed as the percentage of PCNA-positive (brown stained) nuclei.
Preparation of liver extracts
Mice were killed by cervical dislocation under isofluorane anesthesia; the livers were removed and homogenized at the ratio 0.1 g/1ml in buffer composed of 1% Triton, 100 mM Hepes, 100 mM sodium pyrophosphate, 100 mM sodium fluoride, 10 mM EDTA, 10 mM sodium vanadate, 2 mM phenylmethylsulfonyl fluoride (PMSF) and 0.035 trypsin inhibitory units/ml aprotinin (pH 7.4) at 4 C. Liver homogenates were centrifuged at 100,000 × g for 40 min at 4°C to remove insoluble material. Protein concentration of supernatants was determined by the BCA assay (BCA Protein Assay Reagent, Thermo Scientific Pierce). An aliquot of solubilized liver was diluted in Laemmli buffer, boiled for 5 min and stored at −20°C until electrophoresis.
Western blotting analysis
Samples were subjected to electrophoresis in SDS-polyacrylamide gels using Bio-Rad Mini Protean apparatus (Bio-Rad Laboratories). Equal amount of total protein was loaded in each lane. Electrotransference of proteins from gel to polyvinylidenedifluoride membranes was performed for 1 h at 100 mA per transferred membrane (constant current) using the V20-SDB semi-dry blotting apparatus (Scie-Plas) in 0.025 M Tris, 0.192 M glycine, 20% (v/v) methanol and 0.03% (w/v) SDS (pH 8.3). To reduce non-specific antibody binding, membranes were incubated for 1 h at room temperature in T-TBS buffer (0.01 M TRIS-HCl, 0.15 M NaCl and 0.02% w/v Tween 20, pH 7.6) containing 3% w/v BSA. The membranes were then incubated overnight at 4°C with the primary antibody. After washing with T-TBS, the membranes were incubated with a secondary antibody conjugated with horseradish peroxidase for 1 h at room temperature and washed in T-TBS. Immunoreactive proteins were revealed by enhanced chemiluminescence (ECL-Plus, Amersham Biosciences). Band intensities were quantified using Gel-Pro Analyzer 4.1 software (Media Cybernetics). After all detection steps membranes were stained with Coomasie Blue to control the total protein load and blotting efficiency.Citation77
Real-time reverse transcriptase PCR
Total hepatic RNA was extracted using the phenol chloroform method.Citation78 cDNA was obtained using iScriptcDNA synthesis kit (Bio-Rad), and the relative expression of the genes was analyzed by real time PCR as previously described.Citation79 shows the sequence of the primers used. Cyclophilin A was used as a housekeeping gene, and the relative expression levels were calculated according to the Equation 2A−B/2C−D [A = cycle threshold (Ct) number of the gene of interest in the first control sample, B = Ct number of the gene of interest in each sample, C = Ct number of the housekeeping gene in the first control sample, D = Ct number of the housekeeping gene in each sample], as described before.Citation79,Citation80 The relative expression of the first normal sample was expressed as 1, and the relative expression of all other samples was calculated using this equation for each gene. Results are expressed as percentage for the mean normal female mice group value.
Table 2. Sequences of the primers used in real-time reverse transcriptase PCR
Statistical analysis
Experiments were performed analyzing all groups of animals in parallel, n representing the number of different individuals used in each group. Results are presented as mean ± SEM of the number of samples indicated. Statistical analyses were performed by Two Way ANOVA followed by the Newman-Keuls multiple comparison test using the GraphPad Prism 4 statistical program by GraphPad Software, Inc. Student’s t-test was used when the values of two groups were analyzed. Data were considered significantly different if p < 0.05.
Abbreviations: | ||
GH | = | growth hormone |
bGH | = | bovine GH |
CREB | = | c-AMP- response element binding protein |
EGFR | = | epidermal growth factor receptor |
GSK3 | = | glycogen synthase kinase 3 |
GHRH | = | growth hormone releasing hormone |
hGHRH | = | human GHRH |
H&E | = | hematoxylin and eosin |
HCC | = | 3 carcinoma |
IGF1 | = | insulin-like growth factor 1 |
mTOR | = | mammalian target of rapamycin |
NFκB | = | nuclear factor kappa B |
PI3K | = | phosphatidylinositol 3 kinase |
PEPCK | = | phosphoenolpyruvatecarboxykinase |
PVDF | = | polyvinylidenedifluoride |
PCNA | = | proliferating cell nuclear antigen |
STATs | = | signal transducers and activators of transcription |
Acknowledgments
This work was supported by the National Council of Scientific and Technological Research CONICET (grant number PIP 427) to Dr D. Turyn, by the National Agency for Promotion of Science and Technology ANPCyT (grant number PICT 2008-0397) to Dr J.G. Miquet and by the National Institute on Aging (grant numbers AG19899, AG031736) to Dr A. Bartke. Drs J.G. Miquet, L. González, A.I. Sotelo and D. Turyn are Career Investigators from CONICET; B.Sc. C.S. Martinez and M.E. Díaz were supported by a fellowship from CONICET; G.P. Micucci was supported by a fellowship from the University of Buenos Aires. The Fulbright Commission and the CONICET awarded Dr J.G. Miquet a grant for a postdoctoral research stay at the Southern Illinois University.
Disclosure of Potential Conflicts of Interest
No potential conflicts of interest were disclosed.
References
- Brooks AJ, Waters MJ. The growth hormone receptor: mechanism of activation and clinical implications. Nat Rev Endocrinol 2010; 6:515 - 25; http://dx.doi.org/10.1038/nrendo.2010.123; PMID: 20664532
- Lichanska AM, Waters MJ. How growth hormone controls growth, obesity and sexual dimorphism. Trends Genet 2008; 24:41 - 7; http://dx.doi.org/10.1016/j.tig.2007.10.006; PMID: 18063438
- Xu J, Messina JL. Crosstalk between growth hormone and insulin signaling. Vitam Horm 2009; 80:125 - 53; http://dx.doi.org/10.1016/S0083-6729(08)00606-7; PMID: 19251037
- Jansson JO, Ekberg S, Hoath SB, Beamer WG, Frohman LA. Growth hormone enhances hepatic epidermal growth factor receptor concentration in mice. J Clin Invest 1988; 82:1871 - 6; http://dx.doi.org/10.1172/JCI113804; PMID: 3264288
- Ekberg S, Carlsson L, Carlsson B, Billig H, Jansson JO. Plasma growth hormone pattern regulates epidermal growth factor (EGF) receptor messenger ribonucleic acid levels and EGF binding in the rat liver. Endocrinology 1989; 125:2158 - 66; http://dx.doi.org/10.1210/endo-125-4-2158; PMID: 2791983
- Miquet JG, González L, Matos MN, Hansen CE, Louis A, Bartke A, et al. Transgenic mice overexpressing GH exhibit hepatic upregulation of GH-signaling mediators involved in cell proliferation. J Endocrinol 2008; 198:317 - 30; http://dx.doi.org/10.1677/JOE-08-0002; PMID: 18480380
- Jenkins PJ, Mukherjee A, Shalet SM. Does growth hormone cause cancer?. Clin Endocrinol (Oxf) 2006; 64:115 - 21; http://dx.doi.org/10.1111/j.1365-2265.2005.02404.x; PMID: 16430706
- Perry JK, Emerald BS, Mertani HC, Lobie PE. The oncogenic potential of growth hormone. Growth Horm IGF Res 2006; 16:277 - 89; http://dx.doi.org/10.1016/j.ghir.2006.09.006; PMID: 17101287
- McGrane MM, Yun JS, Moorman AFM, Lamers WH, Hendrick GK, Arafah BM, et al. Metabolic effects of developmental, tissue-, and cell-specific expression of a chimeric phosphoenolpyruvate carboxykinase (GTP)/bovine growth hormone gene in transgenic mice. J Biol Chem 1990; 265:22371 - 9; PMID: 1702419
- Kopchick JJ, Bellush LL, Coschigano KT. Transgenic models of growth hormone action. Annu Rev Nutr 1999; 19:437 - 61; http://dx.doi.org/10.1146/annurev.nutr.19.1.437; PMID: 10448532
- Bartke A. Can growth hormone (GH) accelerate aging? Evidence from GH-transgenic mice. Neuroendocrinology 2003; 78:210 - 6; http://dx.doi.org/10.1159/000073704; PMID: 14583653
- Wanke R, Hermanns W, Folger S, Wolf E, Brem G. Accelerated growth and visceral lesions in transgenic mice expressing foreign genes of the growth hormone family: an overview. Pediatr Nephrol 1991; 5:513 - 21; http://dx.doi.org/10.1007/BF01453693; PMID: 1911131
- Orian JM, Lee CS, Weiss LM, Brandon MR. The expression of a metallothionein-ovine growth hormone fusion gene in transgenic mice does not impair fertility but results in pathological lesions in the liver. Endocrinology 1989; 124:455 - 63; http://dx.doi.org/10.1210/endo-124-1-455; PMID: 2642419
- Orian JM, Tamakoshi K, Mackay IR, Brandon MR. New murine model for hepatocellular carcinoma: transgenic mice expressing metallothionein-ovine growth hormone fusion gene. J Natl Cancer Inst 1990; 82:393 - 8; http://dx.doi.org/10.1093/jnci/82.5.393; PMID: 2154583
- Quaife CJ, Mathews LS, Pinkert CA, Hammer RE, Brinster RL, Palmiter RD. Histopathology associated with elevated levels of growth hormone and insulin-like growth factor I in transgenic mice. Endocrinology 1989; 124:40 - 8; http://dx.doi.org/10.1210/endo-124-1-40; PMID: 2642417
- Snibson KJ, Bhathal PS, Hardy CL, Brandon MR, Adams TE. High, persistent hepatocellular proliferation and apoptosis precede hepatocarcinogenesis in growth hormone transgenic mice. Liver 1999; 19:242 - 52; http://dx.doi.org/10.1111/j.1478-3231.1999.tb00042.x; PMID: 10395045
- Snibson KJ. Hepatocellular kinetics and the expression of growth hormone (GH) in the livers and liver tumours of GH-transgenic mice. Tissue Cell 2002; 34:88 - 97; http://dx.doi.org/10.1016/S0040-8166(02)00012-5; PMID: 12165243
- Hoeflich A, Nedbal S, Blum WF, Erhard M, Lahm H, Brem G, et al. Growth inhibition in giant growth hormone transgenic mice by overexpression of insulin-like growth factor-binding protein-2. Endocrinology 2001; 142:1889 - 98; http://dx.doi.org/10.1210/en.142.5.1889; PMID: 11316754
- Coleman WB. Mechanisms of human hepatocarcinogenesis. Curr Mol Med 2003; 3:573 - 88; http://dx.doi.org/10.2174/1566524033479546; PMID: 14527088
- Teoh NC. Proliferative drive and liver carcinogenesis: too much of a good thing?. J Gastroenterol Hepatol 2009; 24:1817 - 25; http://dx.doi.org/10.1111/j.1440-1746.2009.06121.x; PMID: 20002941
- Snibson KJ, Bhathal PS, Adams TE. Overexpressed growth hormone (GH) synergistically promotes carcinogen-initiated liver tumour growth by promoting cellular proliferation in emerging hepatocellular neoplasms in female and male GH-transgenic mice. Liver 2001; 21:149 - 58; http://dx.doi.org/10.1034/j.1600-0676.2001.021002149.x; PMID: 11318985
- Vergani G, Mayerhofer A, Bartke A. Acute effects of human growth hormone on liver cells in vitro: a comparison with livers of mice transgenic for human growth hormone. Tissue Cell 1991; 23:607 - 12; http://dx.doi.org/10.1016/0040-8166(91)90017-N; PMID: 1776152
- Llovet JM, Bruix J. Molecular targeted therapies in hepatocellular carcinoma. Hepatology 2008; 48:1312 - 27; http://dx.doi.org/10.1002/hep.22506; PMID: 18821591
- Spangenberg HC, Thimme R, Blum HE. Targeted therapy for hepatocellular carcinoma. Nat Rev Gastroenterol Hepatol 2009; 6:423 - 32; http://dx.doi.org/10.1038/nrgastro.2009.86; PMID: 19488072
- Zender L, Villanueva A, Tovar V, Sia D, Chiang DY, Llovet JM. Cancer gene discovery in hepatocellular carcinoma. J Hepatol 2010; 52:921 - 9; http://dx.doi.org/10.1016/j.jhep.2009.12.034; PMID: 20385424
- Rogers AB, Theve EJ, Feng Y, Fry RC, Taghizadeh K, Clapp KM, et al. Hepatocellular carcinoma associated with liver-gender disruption in male mice. Cancer Res 2007; 67:11536 - 46; http://dx.doi.org/10.1158/0008-5472.CAN-07-1479; PMID: 18089782
- Ruggieri A, Barbati C, Malorni W. Cellular and molecular mechanisms involved in hepatocellular carcinoma gender disparity. Int J Cancer 2010; 127:499 - 504; http://dx.doi.org/10.1002/ijc.25298; PMID: 20201099
- Popow A, Nowak D, Malicka-Błaszkiewicz M. Actin cytoskeleton and beta-actin expression in correlation with higher invasiveness of selected hepatoma Morris 5123 cells. J Physiol Pharmacol 2006; 57:Suppl 7 111 - 23; PMID: 17228099
- Miller LM, Menthena A, Chatterjee C, Verdier-Pinard P, Novikoff PM, Horwitz SB, et al. Increased levels of a unique post-translationally modified betaIVb-tubulin isotype in liver cancer. Biochemistry 2008; 47:7572 - 82; http://dx.doi.org/10.1021/bi8005225; PMID: 18570381
- Miquet JG, Sotelo AI, Bartke A, Turyn D. Suppression of growth hormone (GH) Janus tyrosine kinase 2/signal transducer and activator of transcription 5 signaling pathway in transgenic mice overexpressing bovine GH. Endocrinology 2004; 145:2824 - 32; http://dx.doi.org/10.1210/en.2003-1498; PMID: 15016718
- Sotelo AI, Miquet JG, González L, Bartke A, Turyn D. Vitamin D3 cannot revert desensitization of growth hormone (GH)-induced STAT5-signaling in GH-overexpressing mice non-calcemic tissues. Growth Horm IGF Res 2008; 18:148 - 56; http://dx.doi.org/10.1016/j.ghir.2007.08.001; PMID: 17881271
- Jope RS, Yuskaitis CJ, Beurel E. Glycogen synthase kinase-3 (GSK3): inflammation, diseases, and therapeutics. Neurochem Res 2007; 32:577 - 95; http://dx.doi.org/10.1007/s11064-006-9128-5; PMID: 16944320
- Jacobs KM, Bhave SR, Ferraro DJ, Jaboin JJ, Hallahan DE, Thotala D. GSK-3β: A Bifunctional Role in Cell Death Pathways. Int J Cell Biol 2012; 2012:930710; http://dx.doi.org/10.1155/2012/930710; PMID: 22675363
- Nicholson KM, Anderson NG. The protein kinase B/Akt signalling pathway in human malignancy. Cell Signal 2002; 14:381 - 95; http://dx.doi.org/10.1016/S0898-6568(01)00271-6; PMID: 11882383
- Takahashi-Yanaga F, Sasaguri T. GSK-3beta regulates cyclin D1 expression: a new target for chemotherapy. Cell Signal 2008; 20:581 - 9; http://dx.doi.org/10.1016/j.cellsig.2007.10.018; PMID: 18023328
- Luedde T, Schwabe RFNF. NF-κB in the liver--linking injury, fibrosis and hepatocellular carcinoma. Nat Rev Gastroenterol Hepatol 2011; 8:108 - 18; http://dx.doi.org/10.1038/nrgastro.2010.213; PMID: 21293511
- Schmid JA, Birbach A. IkappaB kinase beta (IKKbeta/IKK2/IKBKB)--a key molecule in signaling to the transcription factor NF-kappaB. Cytokine Growth Factor Rev 2008; 19:157 - 65; http://dx.doi.org/10.1016/j.cytogfr.2008.01.006; PMID: 18308615
- Song G, Ouyang G, Bao S. The activation of Akt/PKB signaling pathway and cell survival. J Cell Mol Med 2005; 9:59 - 71; http://dx.doi.org/10.1111/j.1582-4934.2005.tb00337.x; PMID: 15784165
- Feitelson MA, Sun B, Satiroglu Tufan NL, Liu J, Pan J, Lian Z. Genetic mechanisms of hepatocarcinogenesis. Oncogene 2002; 21:2593 - 604; http://dx.doi.org/10.1038/sj.onc.1205434; PMID: 11971194
- Yuen MF, Wu PC, Lai VC, Lau JY, Lai CL. Expression of c-Myc, c-Fos, and c-jun in hepatocellular carcinoma. Cancer 2001; 91:106 - 12; http://dx.doi.org/10.1002/1097-0142(20010101)91:1<106::AID-CNCR14>3.0.CO;2-2; PMID: 11148566
- Stamatakos M, Palla V, Karaiskos I, Xiromeritis K, Alexiou I, Pateras I, et al. Cell cyclins: triggering elements of cancer or not?. World J Surg Oncol 2010; 8:111; http://dx.doi.org/10.1186/1477-7819-8-111; PMID: 21176227
- Baik M, Yu JH, Hennighausen L. Growth hormone-STAT5 regulation of growth, hepatocellular carcinoma, and liver metabolism. Ann N Y Acad Sci 2011; 1229:29 - 37; http://dx.doi.org/10.1111/j.1749-6632.2011.06100.x; PMID: 21793836
- Waxman DJ, O’Connor C. Growth hormone regulation of sex-dependent liver gene expression. Mol Endocrinol 2006; 20:2613 - 29; http://dx.doi.org/10.1210/me.2006-0007; PMID: 16543404
- Zhang Y, Laz EV, Waxman DJ. Dynamic, sex-differential STAT5 and BCL6 binding to sex-biased, growth hormone-regulated genes in adult mouse liver. Mol Cell Biol 2012; 32:880 - 96; http://dx.doi.org/10.1128/MCB.06312-11; PMID: 22158971
- Tannenbaum GS, Choi HK, Gurd W, Waxman DJ. Temporal relationship between the sexually dimorphic spontaneous GH secretory profiles and hepatic STAT5 activity. Endocrinology 2001; 142:4599 - 606; http://dx.doi.org/10.1210/en.142.11.4599; PMID: 11606424
- MacLeod JN, Pampori NA, Shapiro BH. Sex differences in the ultradian pattern of plasma growth hormone concentrations in mice. J Endocrinol 1991; 131:395 - 9; http://dx.doi.org/10.1677/joe.0.1310395; PMID: 1783886
- Sueyoshi T, Yokomori N, Korach KS, Negishi M. Developmental action of estrogen receptor-alpha feminizes the growth hormone-Stat5b pathway and expression of Cyp2a4 and Cyp2d9 genes in mouse liver. Mol Pharmacol 1999; 56:473 - 7; PMID: 10462534
- Martinez CS, Piazza VG, Ratner LD, Matos MN, González L, Rulli SB, et al. Growth hormone STAT5-mediated signaling and its modulation in mice liver during the growth period. Growth Horm IGF Res 2013; 23:19 - 28; http://dx.doi.org/10.1016/j.ghir.2012.11.002; PMID: 23245546
- Miquet JG, Muñoz MC, Giani JF, González L, Dominici FP, Bartke A, et al. Ames dwarf (Prop1(df)/Prop1(df)) mice display increased sensitivity of the major GH-signaling pathways in liver and skeletal muscle. Growth Horm IGF Res 2010; 20:118 - 26; http://dx.doi.org/10.1016/j.ghir.2009.11.003; PMID: 20022531
- Haga S, Ozaki M, Inoue H, Okamoto Y, Ogawa W, Takeda K, et al. The survival pathways phosphatidylinositol-3 kinase (PI3-K)/phosphoinositide-dependent protein kinase 1 (PDK1)/Akt modulate liver regeneration through hepatocyte size rather than proliferation. Hepatology 2009; 49:204 - 14; http://dx.doi.org/10.1002/hep.22583; PMID: 19065678
- Mullany LK, Nelsen CJ, Hanse EA, Goggin MM, Anttila CK, Peterson M, et al. Akt-mediated liver growth promotes induction of cyclin E through a novel translational mechanism and a p21-mediated cell cycle arrest. J Biol Chem 2007; 282:21244 - 52; http://dx.doi.org/10.1074/jbc.M702110200; PMID: 17517888
- Friedbichler K, Themanns M, Mueller KM, Schlederer M, Kornfeld JW, Terracciano LM, et al. Growth-hormone-induced signal transducer and activator of transcription 5 signaling causes gigantism, inflammation, and premature death but protects mice from aggressive liver cancer. Hepatology 2012; 55:941 - 52; http://dx.doi.org/10.1002/hep.24765; PMID: 22031092
- Fabregat I. Dysregulation of apoptosis in hepatocellular carcinoma cells. World J Gastroenterol 2009; 15:513 - 20; http://dx.doi.org/10.3748/wjg.15.513; PMID: 19195051
- Normanno N, De Luca A, Bianco C, Strizzi L, Mancino M, Maiello MR, et al. Epidermal growth factor receptor (EGFR) signaling in cancer. Gene 2006; 366:2 - 16; http://dx.doi.org/10.1016/j.gene.2005.10.018; PMID: 16377102
- Xu X, Sakon M, Nagano H, Hiraoka N, Yamamoto H, Hayashi N, et al. Akt2 expression correlates with prognosis of human hepatocellular carcinoma. Oncol Rep 2004; 11:25 - 32; PMID: 14654898
- Dahmani R, Just PA, Perret C. The Wnt/β-catenin pathway as a therapeutic target in human hepatocellular carcinoma. Clin Res Hepatol Gastroenterol 2011; 35:709 - 13; http://dx.doi.org/10.1016/j.clinre.2011.05.010; PMID: 21778132
- Monga SP. Role of Wnt/β-catenin signaling in liver metabolism and cancer. Int J Biochem Cell Biol 2011; 43:1021 - 9; http://dx.doi.org/10.1016/j.biocel.2009.09.001; PMID: 19747566
- Gotoh J, Obata M, Yoshie M, Kasai S, Ogawa K. Cyclin D1 over-expression correlates with beta-catenin activation, but not with H-ras mutations, and phosphorylation of Akt, GSK3 beta and ERK1/2 in mouse hepatic carcinogenesis. Carcinogenesis 2003; 24:435 - 42; http://dx.doi.org/10.1093/carcin/24.3.435; PMID: 12663502
- Ban KC, Singh H, Krishnan R, Seow HF. GSK-3beta phosphorylation and alteration of beta-catenin in hepatocellular carcinoma. Cancer Lett 2003; 199:201 - 8; http://dx.doi.org/10.1016/S0304-3835(03)00421-X; PMID: 12969793
- Joo M, Kang YK, Kim MR, Lee HK, Jang JJ. Cyclin D1 overexpression in hepatocellular carcinoma. Liver 2001; 21:89 - 95; http://dx.doi.org/10.1034/j.1600-0676.2001.021002089.x; PMID: 11318977
- Nelsen CJ, Rickheim DG, Timchenko NA, Stanley MW, Albrecht JH. Transient expression of cyclin D1 is sufficient to promote hepatocyte replication and liver growth in vivo. Cancer Res 2001; 61:8564 - 8; PMID: 11731443
- Nelsen CJ, Rickheim DG, Tucker MM, Hansen LK, Albrecht JH. Evidence that cyclin D1 mediates both growth and proliferation downstream of TOR in hepatocytes. J Biol Chem 2003; 278:3656 - 63; http://dx.doi.org/10.1074/jbc.M209374200; PMID: 12446670
- Ahn KS, Aggarwal BB. Transcription factor NF-kappaB: a sensor for smoke and stress signals. Ann N Y Acad Sci 2005; 1056:218 - 33; http://dx.doi.org/10.1196/annals.1352.026; PMID: 16387690
- Lin CP, Liu CR, Lee CN, Chan TS, Liu HE. Targeting c-Myc as a novel approach for hepatocellular carcinoma. World J Hepatol 2010; 2:16 - 20; PMID: 21160952
- Beer S, Zetterberg A, Ihrie RA, McTaggart RA, Yang Q, Bradon N, et al. Developmental context determines latency of MYC-induced tumorigenesis. PLoS Biol 2004; 2:e332; http://dx.doi.org/10.1371/journal.pbio.0020332; PMID: 15455033
- Hartl M, Bader AG, Bister K. Molecular targets of the oncogenic transcription factor jun. Curr Cancer Drug Targets 2003; 3:41 - 55; http://dx.doi.org/10.2174/1568009033333781; PMID: 12570660
- Behrens A, Sibilia M, David JP, Möhle-Steinlein U, Tronche F, Schütz G, et al. Impaired postnatal hepatocyte proliferation and liver regeneration in mice lacking c-jun in the liver. EMBO J 2002; 21:1782 - 90; http://dx.doi.org/10.1093/emboj/21.7.1782; PMID: 11927562
- Milde-Langosch K. The Fos family of transcription factors and their role in tumourigenesis. Eur J Cancer 2005; 41:2449 - 61; http://dx.doi.org/10.1016/j.ejca.2005.08.008; PMID: 16199154
- Okamoto T, Sanda T, Asamitsu K. NF-kappa B signaling and carcinogenesis. Curr Pharm Des 2007; 13:447 - 62; http://dx.doi.org/10.2174/138161207780162944; PMID: 17348842
- Perkins ND. The diverse and complex roles of NF-κB subunits in cancer. Nat Rev Cancer 2012; 12:121 - 32; PMID: 22257950
- Wullaert A, van Loo G, Heyninck K, Beyaert R. Hepatic tumor necrosis factor signaling and nuclear factor-kappaB: effects on liver homeostasis and beyond. Endocr Rev 2007; 28:365 - 86; http://dx.doi.org/10.1210/er.2006-0031; PMID: 17431229
- Jeay S, Sonenshein GE, Postel-Vinay MC, Kelly PA, Baixeras E. Growth hormone can act as a cytokine controlling survival and proliferation of immune cells: new insights into signaling pathways. Mol Cell Endocrinol 2002; 188:1 - 7; http://dx.doi.org/10.1016/S0303-7207(02)00014-X; PMID: 11911939
- He G, Karin MNF. NF-κB and STAT3 - key players in liver inflammation and cancer. Cell Res 2011; 21:159 - 68; http://dx.doi.org/10.1038/cr.2010.183; PMID: 21187858
- Abramovitch R, Tavor E, Jacob-Hirsch J, Zeira E, Amariglio N, Pappo O, et al. A pivotal role of cyclic AMP-responsive element binding protein in tumor progression. Cancer Res 2004; 64:1338 - 46; http://dx.doi.org/10.1158/0008-5472.CAN-03-2089; PMID: 14973073
- Chin KT, Zhou HJ, Wong CM, Lee JM, Chan CP, Qiang BQ, et al. The liver-enriched transcription factor CREB-H is a growth suppressor protein underexpressed in hepatocellular carcinoma. Nucleic Acids Res 2005; 33:1859 - 73; http://dx.doi.org/10.1093/nar/gki332; PMID: 15800215
- Mayo KE, Hammer RE, Swanson LW, Brinster RL, Rosenfeld MG, Evans RM. Dramatic pituitary hyperplasia in transgenic mice expressing a human growth hormone-releasing factor gene. Mol Endocrinol 1988; 2:606 - 12; http://dx.doi.org/10.1210/mend-2-7-606; PMID: 3137455
- Welinder C, Ekblad L. Coomassie staining as loading control in Western blot analysis. J Proteome Res 2011; 10:1416 - 9; http://dx.doi.org/10.1021/pr1011476; PMID: 21186791
- Chomczynski P, Sacchi N. Single-step method of RNA isolation by acid guanidinium thiocyanate-phenol-chloroform extraction. Anal Biochem 1987; 162:156 - 9; http://dx.doi.org/10.1016/0003-2697(87)90021-2; PMID: 2440339
- Masternak MM, Al-Regaiey KA, Del Rosario Lim MM, Bonkowski MS, Panici JA, Przybylski GK, et al. Caloric restriction results in decreased expression of peroxisome proliferators-activated receptors (PPARs) superfamily in muscle of normal and long-lived GHR-KO mice. J Gerontol A Biol Sci Med Sci 2005; 60:1238 - 45; http://dx.doi.org/10.1093/gerona/60.10.1238; PMID: 16282554
- Wang Z, Masternak MM, Al-Regaiey KA, Bartke A. Adipocytokines and the regulation of lipid metabolism in growth hormone transgenic and calorie-restricted mice. Endocrinology 2007; 148:2845 - 53; http://dx.doi.org/10.1210/en.2006-1313; PMID: 17347312