Abstract
The only canonical Holliday junction (HJ) resolvase identified in eukaryotes thus far is Yen1/GEN1. Nevertheless, Yen1/GEN1 appears to have a minor role in HJ resolution, and, instead, other structure-specific endonucleases (SSE) that recognize branched DNA play the leading roles, Mus81-Mms4/EME1 being the most important in budding yeast. Interestingly, cells tightly regulate the activity of each HJ resolvase during the yeast cell cycle. Thus, Mus81-Mms4 is activated in G2/M, while Yen1 gets activated shortly afterwards. Nevertheless, cytological studies have shown that Yen1 is sequestered out of the nucleus when cyclin-dependent kinase activity is high, i.e., all of the cell cycle but G1. We here show that the mitotic master phosphatase Cdc14 targets Yen1 to the nucleus in early anaphase through the FEAR network. We will further show that this FEAR-mediated Cdc14-driven event is sufficient to back-up Mus81-Mms4 in removing branched DNA structures, which are especially found in the long chromosome arms upon replication stress. Finally, we found that MEN-driven Cdc14 re-activation in late anaphase is essential to keep Yen1 in the nucleus until the next G1. Our results highlight the essential role that early-activated Cdc14, i.e., through the FEAR network, has in removing all kind of non-proteinaceous linkages that preclude faithful sister chromatid segregation in anaphase. In addition, our results support the general idea of Yen1 acting as a last resource endonuclease to deal with any remaining HJ that might compromise genetic stability during chromosome segregation.
Introduction
Joint molecules (JMs) comprise different branched DNA junctions that link chromatids and/or chromosomes. JMs are formed as intermediate products during the repair of DNA double-strand breaks (DSBs) through the homologous recombination pathway (HR).Citation1 HR-dependent JM are also formed during the bypass of stalled replication forks (SRF) and postreplicative gaps, which may arise due to DNA base damage, interstrand crosslink, etc.Citation2,Citation3 Several JMs can be distinguished according to the physical nature of the branched junction, being one of the most studied the so-called Holliday junction (HJ).Citation1,Citation4,Citation5 This basic 4-way structure has, in turn, several variants with special features related to their processing: the intact (i.e., uninterrupted) HJ (iHJ), the nicked HJ (nHJ), and the double HJ (dHJ). Central to HJs processing, eukaryotic cells possess one helicase–topoisomerase complex and 3 conserved structure-specific endonucleases (SSEs).Citation3,Citation6,Citation7 The helicase–topoisomerase complex (hereafter refer to as STR) comprises the budding yeast helicase Sgs1 (Bloom syndrome BLM in humans), the type I topoisomerase Top3 (TOPOIIIα), and the cofactor Rmi1 (RMI1-RMI2); whereas, the 3 SSEs are Slx1-Slx4 (SLX1-SLX4), Mus81-Mms4 (MUS81-EME1), and Yen1 (GEN1). In vitro, STR can eliminate model dHJ by branch migrating each iHJ toward each other in a process termed “dHJ dissolution”.Citation8 By contrast, SSEs cut HJs, albeit with different specificities. Thus, Yen1 and Slx1-Slx4 are mostly active against iHJs, and Mus81-Mms4 prefers nHJs.Citation9-Citation14 In vivo, STR appears as the cell first and best choice in mitosis, because dHJ dissolution always results in no sequence exchange between chromatids (i.e., non-crossovers).Citation8,Citation15,Citation16 As for the SSEs, cells may choose them when STR is presumed to fail (e.g., single iHJs and nHJs).Citation7,Citation17 Whereas many studies have shown that disruption of different SSEs and the STR synergistically enhance the presence of JMs in vivo,Citation18-Citation24 the question of why eukaryotes have 3 different SSE to process HJs remains open. This is more puzzling when just one SSE, the nHJ resolvase Mus81-Mms4, appears to be sufficient to accomplish all HJ resolution.Citation7 Even more intriguing is the fact that the actual equivalent to the bacterial HJ resolvase RuvC, i.e., Yen1, has only a minor role in eukaryotes. In this context, several recent reports have defined the cell cycle regulation of STR, Mus81-Mms4, and Yen1 activities, and found that, while STR is active all throughout, Mus81-Mms4 becomes active once cells are in G2/M, and Yen1 appears active shortly afterwards.Citation13,Citation24,Citation25 Mus81–Mms4 activity depends on both high cyclin-dependent kinase (CDK) activity and the activation of the Polo-like kinase Cdc5, which, in turns, takes place at the G2/M transition.Citation13,Citation24-Citation26 Less is known about Yen1 activity. Aside from being active when purified from cells transiting in the M phase,Citation13 Yen1 contains a nuclear localization signal (NLS) that is masked by phosphorylation through CDK.Citation27 Thus, Yen1 is nuclear when CDK activity is low, i.e., G1, and cytoplasmic when CDK activity is high, i.e., S/G2/M. This implies an intriguing paradox, since Yen1 appears active against HJ in vitro, when it is actually sequestered out of the nucleus in vivo.
CDK-mediated phosphorylation can be reverted in targeted proteins through 3 different, though not necessarily exclusive, manners. First, when all CDK activity drops at the very end of mitosis, de novo CDK-targeted proteins would remain unphosphorylated. This is particularly effective in those proteins with a rapid turnover. Second, the pool of different cyclins which substitute one another to maintain a high CDK activity from S to telophase can also change the substrate specificity of CDK.Citation28 Lastly, specific phosphatases can counteract CDK-dependent phosphorylation. When coupled to the lowering of CDK activity, these phosphatases accomplish that even stable proteins remain unphosphorylated. The master phosphatase that removes the phosphate from the CDK consensus site in S. cerevisiae is Cdc14.Citation29,Citation30 Sequestered through most of the cell cycle within the nucleolus, Cdc14 is released in 2 waves at the end of mitosis.Citation31,Citation32 The first wave takes place shortly after the anaphase onset and mediates dephosphorylation of selected CDK-targeted proteins. This wave is controlled by the so-called CDC fourteen early anaphase release network (FEAR). The FEAR coordinates precisely critical events that take place during anaphase, i.e., lengthening of the mitotic spindle, chromosome condensation, topological unlinkage of sister chromatids, control of the forces that pull apart sister chromatids, correct positioning of the spindle pole bodies (SPBs) in each daughter cell, etc.Citation33-Citation37 The second wave is triggered by the mitotic exit network (MEN), which is responsible for switching off CDK activity, thus promoting cytokinesis and the transition into a new G1.Citation38-Citation41
The nuclear location of Yen1 is a prerequisite for its HJ activity since budding yeast performed a close mitosis, i.e., the nuclear membrane is not dismantled in mitosis. Hence, in this work, we aimed to determine if Yen1 was ever targeted to the nucleus out of G1, particularly in anaphase, and, if so, for how long and whether Cdc14 was important for this targeting in vivo. We indeed found that Yen1 shuttles from the cytoplasm to the nucleus in early anaphase, and that this is performed through the activation of Cdc14. We further show that Cdc14 is epistatic to Yen1 in terms of JM resolution in the absence of Mus81–Mms4 activity.
Results and Discussion
We tried to address the issue of Yen1 subcellular localization by fluorescence microscopy through tagging the YEN1 gene with the gene which encodes for the yeast-enhanced green fluorescent protein (yeGFP, or simply GFP hereafter). In order to follow the dynamics of Yen1–GFP localization, we performed time-course experiments during one synchronous G1-to-telophase cell cycle. To block cells in telophase, before the CDK activity drops, we made use of a thermosensitive conditional allele for the critical MEN component Cdc15 (i.e., cdc15-2). To precisely follow up anaphase we further tagged the SPB component Spc42 with the RedStar fluorescent protein.
Yen1 seems to be present at low concentrations within the cell,Citation42 and, indeed, we were unable to see the Yen1–GFP fusion when controlled by its own promoter. Because of this caveat, we chose to put the Yen1–GFP gene under the strong GAL promoter. The overexpressed C-terminal Yen1–GFP chimera happened to be non-toxic in galactose and fully functional in a growth assay with MMS (). Thus, in glucose, a GAL-YEN1:GFP strain that also carries the mms4Δ deletion was as hypersensitive to MMS as the double mutant mms4Δ yen1Δ. By contrast, growth in galactose abolished this high hypersensitivity, and the strain was able to grow as good as the mms4Δ single mutant ().
Figure 1. Overexpressed Yen1-GFP suppresses MMS hypersensitivity of the mms4Δ yen1Δ strain. Overnight cultures of the strains FM1292 (cdc15-2) and FM1272 (cdc14-1) plus their derivatives carrying mms4Δ, mms4Δ yen1Δ, GAL:YEN1:GFP SPC42:RedStar, and mms4Δ GAL:YEN1:GFP SPC42:RedStar were serial diluted and plated onto 4 different plates; YP glucose, YP galactose, and either media further supplemented with MMS 0.004% (v/v). Pictures to determine ability to grow were taken after 5 d of incubation at 25 °C.
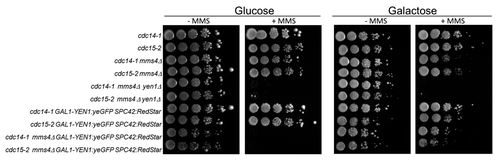
Next, we tried to visualize Yen1-GFP under the microscope. At least 1 h of continuous galactose induction was needed to see Yen1-GFP within the cell. As for the location pattern, galactose induction in the asynchronous culture yielded a strong nuclear signal in unbudded cells and a weaker and diffuse signal in budded cells (). In order to better follow up the dynamics of Yen1-GFP we synchronized cells in G1 before inducing YEN1-GFP, induced then the gene in galactose while keeping the cells in G1, and finally released all cells into a synchronous cell cycle. In order to avoid interference with de novo synthesis of Yen1-GFP out of G1, we switched the gene off at the time of the G1 release. Finally, the temperature was also shifted to 37 °C in order to inactivate Cdc15-2 and thus cause a final block in telophase, i.e., a G1-to-telophase synchronous cell cycle. Thus, we observed that Yen1-GFP was nuclear at the G1 arrest but rapidly disappeared from the nucleus upon S-phase entry (). Interestingly, there was a short window at about 100–120 min after the G1 release, where we observed Yen1-GFP coming back to the nucleus when it was stretched between the mother and the bud (). This new nuclear location peaked when the distance between the SPBs was between 3–6 μm, which roughly corresponds to early anaphase (). When cells reached late anaphase/telophase; SPB distances > 8 μm, this nuclear Yen1–GFP signal faded away greatly. As mentioned above, Yen1 is a HJ resolvase, and steady-state levels of HJ can be increased by making cells use HR more often. Thus, we also follow a synchronous cell cycle upon continuous low levels of MMS (0.004% v/v). This low MMS concentration interfered little with the cell cycle progression in the cdc15-2 and cdc15-2 GAL-YEN1:GFP strains (data not shown). Remarkably, the same relocalization pattern was observed upon MMS treatment, although the Yen1-GFP nuclear signal appeared more granulated and frequently formed distinct foci (). These foci happened to be either on the nuclear DNA, as they clearly colocalized with DAPI, or between the 2 segregated nuclear masses ().
Figure 2. Yen1 re-localizes back to the nucleus in early anaphase in a Cdc14-dependent manner. Overnight YP raffinose cultures of the strains FM1799 (cdc15-2 GAL:YEN1:GFP SPC42:RedStar) and FM1804 (cdc14-1 GAL:YEN1:GFP SPC42:RedStar) were first arrested in G1 with αF for 3 h in the growth media. Next, galactose 2% (w/v) was added and the culture was left blocked in G1 for another 2 h. They then were split into 2 fresh YP glucose media, one of them containing MMS 0.004% (v/v), and finally released into a synchronous cell cycle at 37 °C for 4 h. (A) Representative micrographs of z-stack maximum projections from the G1 blocks after 2 h of galactose addition (G1 arrest), and about 100–120 min after the G1 release at 37 °C with or without MMS. The label (m) points to an S/G2 cell with an unaligned ~2 μm spindle (i.e., distance between SPBs) and no nuclear Yen1-GFP signal; the label (a) points to examples of cells in early anaphase according to spindle orientation and length, all with a visible nuclear Yen1; and the label (t) points to a cell already blocked in telophase with a much fainter nuclear Yen1 signal. (B) Percentage of cells with nuclear Yen1-GFP relative to the distance between SPBs (mean ± SEM, n = 3). Only budded cells with 2 SPBs were counted. (C) A sample from the FM1799 G1-to-telophase time course in MMS was treated with DAPI to address if foci colocalized with nuclear DNA. The label (f) points to an example of a cell in anaphase with foci within the main nuclear DNA mass, whereas the label (f') points to a cell in anaphase with foci located between the 2 segregated nuclear masses. BF, bright field.
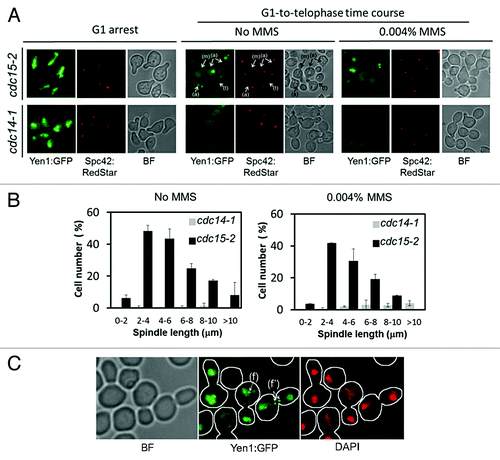
The nuclear relocalization of Yen1 in early anaphase prompted us to check whether Cdc14 might be targeting Yen1 to the nucleus through the FEAR network. As described above, Yen1 bears a CDK consensus site within its NLS region. Phosphorylation of such a site masks the NLS and keeps Yen1 out of the nucleus.Citation27 Besides, Yen1 gets broadly dephosphorylated around G2/M,Citation13 and Cdc14 is the master anti-CDK phosphatase that acts in that period of the cell cycle.Citation31 In order to test the Cdc14-driven relocalization hypothesis, we performed the same cell cycle-dependent Yen1-GFP localization in a cdc14-1 background at 37 °C. Upon Yen1-GFP overexpression in G1, its nuclear localization was equivalent to that of cdc15-2, and this location was lost in a similar way after entering S phase (). However, no nuclear relocalization was seen throughout, not even when MMS was present (). This indicates that Cdc14 plays a key role in targeting Yen1 to the nucleus in early anaphase. Our results also point out that Cdc14-driven Yen1 relocalization occurs always in every cell cycle (i.e., this relocalization does not necessarily depend on induced DNA damage), although DNA damage causes Yen1-GFP to shift from a dispersed nucleoplasmic signal into distinct foci.
A striking result from the location pattern in the cdc15-2 G1-to-telophase time course was the apparent transient nuclear location of Yen1-GFP. Thus, Yen1-GFP is nuclear in G1, cytoplasmic in S/G2, nuclear in early anaphase, and again cytoplasmic in late anaphase (). This is intriguing, since it points out toward 2 waves of nuclear relocalization for Yen1, one in early anaphase and another one in G1. Nevertheless, the result we obtained with the cdc15-2 can be logic taking into account that FEAR elicits a first wave of Cdc14 activity, which is then turned off, unless MEN is immediately triggered afterwards.Citation31,Citation32 Since Cdc15 is a MEN component, cdc15-2 cannot sustain a prolonged Cdc14 activity at the restrictive condition. In addition, since MEN-driven full Cdc14 activation is the one that achieves the CDK activity drop, it is thus expected that Yen1 would become phosphorylated again and, hence, expelled from the nucleus, in late anaphase in a cdc15-2 background. In order to confirm this hypothesis, we performed a release from the telophase arrest into a new G1 (i.e., telophase-to-G1 time course). In this case, we first synchronized the cell cycle in G2 and then induced the YEN1 gene to make sure that de novo Yen1-GFP was cytoplasmic and had never been in the nucleus before. Thus, induction of Yen1-GFP in nocodazole (Nz) yielded a clear cytoplasmic signal (). A G2-to-telophase time course yielded a transient nuclear relocalization in the cdc15-2 strain and no relocalization in cdc14-1 (). For instance, 30 min after Nz removal, 23% of anaphase cells had a nuclear signal in the cdc15-2, whereas this percentage dropped to 4% after an additional hour. In all cases, the whole Yen1-GFP protein levels remained constant (), confirming that the loss of nuclear Yen1-GFP signal was due to re-localization rather than degradation. Upon release from telophase by shifting the temperature from 37 °C to 25 °C, Yen1 went to the nucleus in both strains, although the signal in the cdc15-2 was much stronger than in the cdc14-1 (likely reflecting the need of Cdc14-1 to get fully reconstituted by prolonging the incubation at the permissive temperature) ().
Figure 3. Yen1 nuclear relocalization in anaphase is dynamic and governed by the CDK/Cdc14 balance. Overnight YP raffinose cultures of the strains FM1799 (cdc15-2 GAL:YEN1:GFP SPC42:RedStar) and FM1804 (cdc14-1 GAL:YEN1:GFP SPC42:RedStar) were first arrested in G2 with nocodazole (Nz) for 3 h. Next, galactose 2% (w/v) was added, and the culture was left blocked in G2 for another 90 min. Next, Nz was removed from the media at the same time that glucose was added and the culture shifted to 37 °C. Cells were incubated in this condition for 90 min (G2-to-telophase cell cycle) before shifting the temperature to 25 °C and adding αF (Telophase-to-G1 cell cycle). (A) Representative micrographs of z-stack maximum projections from the different cell cycle blocks and/or time points of the corresponding releases. (B) Percentage of cells with nuclear Yen1-GFP at the different cell cycle blocks and/or time points of the corresponding releases. (C) Western blot against Yen1-GFP during the G2-to-telophase and telophase-to-G1 cell cycles. (D) Overnight YP raffinose cultures of the strains FM1799 (cdc15-2 GAL:YEN1:GFP SPC42:RedStar) and FM1850 (cdc15-2 GAL-CDC14 GAL:YEN1:GFP SPC42:RedStar) were first arrested in G2 with nocodazole (Nz) for 3 h. Next, galactose 2% (w/v) was added while the cultures were left blocked in G2 for another 90 min. BF, bright field.
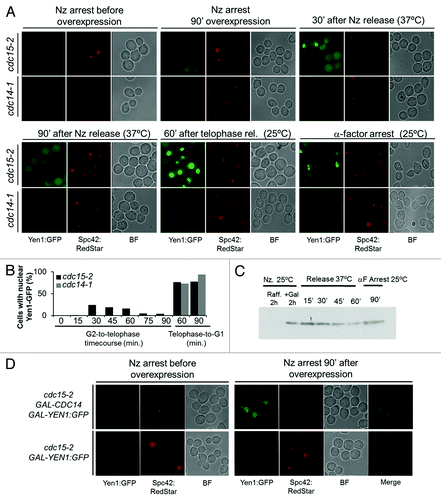
All the results we have just shown above strongly point out that the 2 waves of Cdc14 activation, taking place in early and late anaphase, respectively, drive and sustain the relocalization of Yen1 to the nucleus. In order to further confirm that activation of Cdc14 was sufficient to relocalize Yen1-GFP to the nucleus, we overexpressed CDC14 in a cell cycle stage other than anaphase; more precisely, in cells blocked in G2 with Nz. This condition has been shown to activate Cdc14 without the need of FEAR or MEN execution.Citation34,Citation43 Thus, we found that galactose-driven co-overexpression of GAL-CDC14 and GAL-YEN1:GFP causes Yen1-GFP to be nuclear in more than 80% of G2-blocked cells upon 90 min of induction ().
In order to see whether the regulation of Cdc14 over Yen1 was important to prevent and/or resolve HJs, we looked at the presence of JMs in the cdc14-1 background by pulse field gel electrophoresis (PFGE). Sister chromatids with DNA-mediated linkages (i.e., JMs or persistent SRFs) are known to not enter a PFGE.Citation21,Citation44 Thus, we observed that large chromosomes such as chromosome XII (cXII) and IV (cIV) were less visible in the cdc15-2 mms4Δ yen1Δ strain upon MMS treatment than in any other mutant combinations for cdc15-2 (). Like in the cdc15-2 reference strain, most chromosomes were visible in a cdc14-1 telophase block, even when DNA damage was induced with MMS (). Strikingly, cXII was barely visible in the cdc14-1 mms4Δ strain upon DNA damage, in a pattern very similar to cdc15-2 mms4Δ yen1Δ (). However, cdc14-1 yen1Δ was not different to just cdc14-1, nor was cdc14-1 mms4Δ yen1Δ to cdc14-1 mms4Δ. Lastly, to make sure that this PFGE behavior was not due to stalled replication in the cdc14-1 mms4Δ mutant, we followed bulk DNA replication in all mutants and found no differences (). In all cases, replication was completed by 90 min after the G1 release (i.e., 2.5 h before we took the samples for PFGE analysis). Therefore, all these findings fit well with Cdc14 having a role in JM resolution through Yen1. Besides, this Yen1 activity is complementary to that of Mus81-Mms4, which, in turn, is independent of Cdc14. Lastly, but not least, the Cdc14 pool activated by FEAR elicits enough endogenous Yen1 activity to resolve all remaining JMs.
Figure 4. Yen1 and Cdc14 are epistatic in resolving Joint Molecules in the absence of Mus81-Mms4. Overnight YP glucose cultures of the strains FM1292 (cdc15-2) and FM1272 (cdc14-1), knocked out single mutant derivatives of them for MMS4 and YEN1, and the double mutants mms4Δ yen1Δ were first arrested in G1 at 25 °C. They were then split into 2 media, one of them containing MMS 0.004% v/v, and finally released into a synchronous cell cycle at 37 °C for 4 h. (A) PFGE of all yeast chromosomes at the telophase blocks. Minus sign, no MMS; plus sign, MMS 0.004% v/v. (B) Quantitation of the electrophoretic bands for the large chromosomes relative to the middle-sized chromosome pair XVI/XIII (mean ± SEM, n = 3). (C) Flow cytometry analysis of the DNA content of the G1-to-telophase time-course for the cdc14-1 strains.
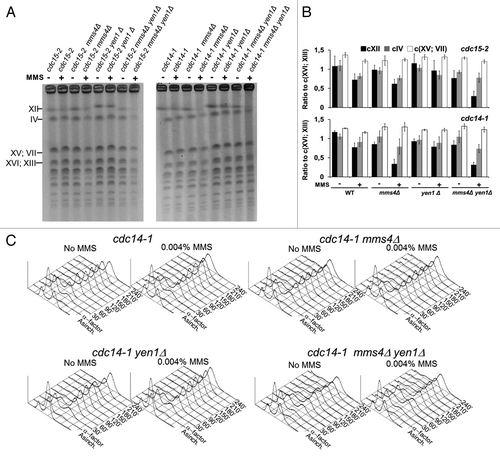
The data we present herein showed that Yen1 is targeted to the nucleus in early anaphase, and this event depends on Cdc14. Taking into account that Mus81-Mms4 has been reported to mainly act in G2/M,Citation13,Citation24-Citation26 Yen1 may therefore serve as a last-resource backup JM-resolving pathway that operates from early anaphase until cytokinesis. Another interesting possibility is that, by restricting Yen1 to anaphase, the spindle pulling forces may give directionality to the resolution of HJs toward a less toxic or genetic-compromising outcome. The observed regulation of Cdc14 over Yen1 extends the key role of the former in preventing all types of non-proteinaceous sister chromatid linkages (i.e., catenations and DNA-mediated) that might form a bridge between segregating chromosomes in anaphase.Citation36,Citation43,Citation45
Materials and Methods
Yeast strains, growth, and experimental conditions
All yeast strains used in this work come from the S288C background and are listed in . N- and C-terminal tagging with yeGFP or RedStar, gene deletions, marker swap, and allele/promoter replacements were engineered through PCR strategies.Citation46 All strains were grown overnight in air orbital incubators at 25 °C in YP raffinose media (yeast extract 1% w/v, peptone 2% w/v, and raffinose 2% w/v) supplemented with adenine 0.08 mg/ml unless stated otherwise. G1 blocks were performed by incubating cultures at 0.3 OD600 with 50 ng/ml of the α-factor mating pheromone (αF, all tested strains were bar1Δ) for 3 h. Yen1-GFP was then induced for 2 h by adding galactose 2% (w/v). The induction was monitored by fluorescence microscopy. The G1-to-telophase synchronous cell cycle was performed as follows: G1-blocked cells with induced Yen1-GFP were released from the block by first washing twice with YP glucose 2% w/v (stops Yen1-GFP production); resuspended in fresh YP supplemented with raffinose 2% w/v, glucose 2% w/v, adenine 0.08 mg/ml and pronase E 0.1 mg/ml; and incubated at 37 °C for 4 h. In the instances where DNA damage was exogenously induced, 0.004% (v/v) MMS was added at the time of the G1 release. MMS was kept in the media until the telophase arrest. Throughout the G1-to-telophase cell cycle, samples were taken at different time points for further analysis. G2-to-telophase cell cycle was carried out in a similar manner. G2 block was achieved by incubating the strains with 15 μg/ml of nocodazole for 3 h at 25 °C. GAL-YEN1:GFP galactose induction during the G2 block lasted 1.5 h, and then the promoter was shut down by adding glucose. The same induction procedure was performed for the co-induction of GAL-CDC14 and GAL-YEN1:GFP. G2-to-telophase synchronous cell cycle was achieved by washing out Nz and shifting the temperature to 37 °C. For the telophase-to-G1 cell cycle, cells were released from telophase by shifting the temperature from 37 °C back to 25 °C and adding 50 ng/ml of αF.
Table 1. Strains used in this work. Parental strain is also included
Error bars in graphs represent the standard error of the mean (SEM).
Fluorescence microscopy
Fluorescent proteins were analyzed by wide-field fluorescence microscopy as previously reported.Citation47 Synchrony was determined by cell morphology and segregation of the nucleus. In order to follow up segregation of the nucleus, an aliquot was frozen at −20 °C for 48 h before DNA was stained using DAPI at 0.05 µg/ml final concentration after short cell treatment with 0.01% v/v Triton X-100. This procedure was also used to determine if Yen1 foci colocalized with DNA. Yen1-GFP subcellular location and Spc42-RedStar were determined directly in cells freshly harvested. For the GFP we used 10 Z stacks, 0.6 μm each, obtained through an YFP filter cube (Leica) using the following parameters: 1 s exposure, gain 7/10, and intensity 5/5. For the RedStar we also used ten Z stacks, 0.6 μm each, obtained through a RFP filter cube (Leica) using the following parameters: 2 s exposure, gain 7/10, and intensity 4/5. Distances between Spc42-labeled SPBs was measured on 2D maximum projections.
Pulse field gel electrophoresis (PFGE), flow cytometry, and western blots
PFGE to see all yeast chromosomes was performed using a CHEF DR-III system (Bio-Rad) in a 0.8% agarose gel in 0.5 × TBE buffer and run at 12 °C for 20 h at 6 V/cm with an initial switching time of 80 s, a final of 150 s, and an angle of 120°. Yeast chromosomes were photographed after staining with ethidium bromide.
Flow cytometry analysis was performed as previously described in order to follow up bulk DNA replication.Citation47
Western blots against Yen1-GFP were performed using a Mini-PROTEAN II gel apparatus (Biorad) in an 8% SDS-PAGE gel. Five OD600 equivalents were harvested for each timepoint. Proteins were extracted with glass beads and SDS 2% for 2 min and then boiled in a water bath for 3 min. One hundred μl of 2× Laemmli buffer was added to each sample and boiled for an additional minute. Samples were briefly centrifuged, and 20 μl of the supernatant were loaded. After electrophoresis, proteins were transferred from the gel to an Immobilion-P PVDF membrane (Millipore). The membrane was blocked for 1 h in 5% (w/v) non-fat milk in TBST (100 mM Tris-Cl pH7.5, 0.9% NaCl, 0.1% Tween 20) at room temperature. After blocking, the membrane was incubated for 1 h with an anti-GFP antibody from rabbit at 1:8000 (Abcam) in 5% milk/TBST followed by an additional hour of incubation with a secondary antibody anti-rabbit-AP (1:50000) (Promega). The membrane was washed and finally visualized with CDPStar (GE Healthcare) on X-ray films (GE Healthcare, Amersham Hyperfilm ECL).
Abbreviations: | ||
HJ | = | Holliday junction |
iHJ | = | intact HJ |
nHJ | = | nicked HJ |
dHJ | = | double HJ |
SSE | = | structure-specific endonucleases |
JM | = | joint molecules |
HR | = | homologous recombination pathway |
SRF | = | stalled replication forks |
STR | = | Sgs1-Top3-Rmi1 complex |
CDK | = | cyclin-dependent kinase |
NLS | = | nuclear localization signal |
FEAR | = | CDC fourteen early anaphase release |
SPBs | = | spindle pole bodies |
MEN | = | mitotic exit network |
yeGFP | = | yeast enhanced green fluorescent protein |
MMS | = | methyl methanesulfonate |
Nz | = | nocodazole |
αF | = | alpha-factor mating pheromone |
PFGE | = | pulse field gel electrophoresis |
SEM | = | standard error of the mean |
BF | = | bright field |
Disclosure of Potential Conflicts of Interest
No potential conflicts of interest were disclosed.
Acknowledgments
We thank Stephen C West for sharing some unpublished data with us and Raimundo Freire for providing the anti-GFP antibody. We also thank other members of our labs for help and discussion. Authors contributed as follows: J.G.L. has performed most of the experimental work. J.G.L., A.C.B., L.A., and F.M. have planned the experiments. L.A. and F.M. have supervised this work. F.M. has written the paper. L.A. hosted J.G.L. in his lab for learning methods related to this work.
Funding
This work was supported by Instituto de Salud Carlos III (PS09/00106 and PS12/00280), Fundación Canaria de Investigación y Salud (08/42 to J.G.L.) and Spanish Ministry of Education (FPU fellowship AP2009-2511 to J.G.L.). All these programs were co-financed with the European Commission's ERDF structural funds
References
- Pâques F, Haber JE. Multiple pathways of recombination induced by double-strand breaks in Saccharomyces cerevisiae. Microbiol Mol Biol Rev 1999; 63:349 - 404; PMID: 10357855
- Heyer W-D, Ehmsen KT, Liu J. Regulation of homologous recombination in eukaryotes. Annu Rev Genet 2010; 44:113 - 39; http://dx.doi.org/10.1146/annurev-genet-051710-150955; PMID: 20690856
- Rass U. Resolving branched DNA intermediates with structure-specific nucleases during replication in eukaryotes. Chromosoma 2013; 122:499 - 515; http://dx.doi.org/10.1007/s00412-013-0431-z; PMID: 24008669
- Schwacha A, Kleckner N. Identification of double Holliday junctions as intermediates in meiotic recombination. Cell 1995; 83:783 - 91; http://dx.doi.org/10.1016/0092-8674(95)90191-4; PMID: 8521495
- Bzymek M, Thayer NH, Oh SD, Kleckner N, Hunter N. Double Holliday junctions are intermediates of DNA break repair. Nature 2010; 464:937 - 41; http://dx.doi.org/10.1038/nature08868; PMID: 20348905
- Schwartz EK, Heyer W-D. Processing of joint molecule intermediates by structure-selective endonucleases during homologous recombination in eukaryotes. Chromosoma 2011; 120:109 - 27; http://dx.doi.org/10.1007/s00412-010-0304-7; PMID: 21369956
- Hickson ID, Mankouri HW. Processing of homologous recombination repair intermediates by the Sgs1-Top3-Rmi1 and Mus81-Mms4 complexes. Cell Cycle 2011; 10:3078 - 85; http://dx.doi.org/10.4161/cc.10.18.16919; PMID: 21876385
- Wu L, Hickson ID. The Bloom’s syndrome helicase suppresses crossing over during homologous recombination. Nature 2003; 426:870 - 4; http://dx.doi.org/10.1038/nature02253; PMID: 14685245
- Kaliraman V, Mullen JR, Fricke WM, Bastin-Shanower SA, Brill SJ. Functional overlap between Sgs1-Top3 and the Mms4-Mus81 endonuclease. Genes Dev 2001; 15:2730 - 40; http://dx.doi.org/10.1101/gad.932201; PMID: 11641278
- Fricke WM, Brill SJ. Slx1-Slx4 is a second structure-specific endonuclease functionally redundant with Sgs1-Top3. Genes Dev 2003; 17:1768 - 78; http://dx.doi.org/10.1101/gad.1105203; PMID: 12832395
- Fricke WM, Bastin-Shanower SA, Brill SJ. Substrate specificity of the Saccharomyces cerevisiae Mus81-Mms4 endonuclease. DNA Repair (Amst) 2005; 4:243 - 51; http://dx.doi.org/10.1016/j.dnarep.2004.10.001; PMID: 15590332
- Ip SCY, Rass U, Blanco MG, Flynn HR, Skehel JM, West SC. Identification of Holliday junction resolvases from humans and yeast. Nature 2008; 456:357 - 61; http://dx.doi.org/10.1038/nature07470; PMID: 19020614
- Matos J, Blanco MG, Maslen S, Skehel JM, West SC. Regulatory control of the resolution of DNA recombination intermediates during meiosis and mitosis. Cell 2011; 147:158 - 72; http://dx.doi.org/10.1016/j.cell.2011.08.032; PMID: 21962513
- Schwartz EK, Wright WD, Ehmsen KT, Evans JE, Stahlberg H, Heyer W-D. Mus81-Mms4 functions as a single heterodimer to cleave nicked intermediates in recombinational DNA repair. Mol Cell Biol 2012; 32:3065 - 80; http://dx.doi.org/10.1128/MCB.00547-12; PMID: 22645308
- Ira G, Malkova A, Liberi G, Foiani M, Haber JE. Srs2 and Sgs1-Top3 suppress crossovers during double-strand break repair in yeast. Cell 2003; 115:401 - 11; http://dx.doi.org/10.1016/S0092-8674(03)00886-9; PMID: 14622595
- Oh SD, Lao JP, Hwang PY-H, Taylor AF, Smith GR, Hunter N. BLM ortholog, Sgs1, prevents aberrant crossing-over by suppressing formation of multichromatid joint molecules. Cell 2007; 130:259 - 72; http://dx.doi.org/10.1016/j.cell.2007.05.035; PMID: 17662941
- Cromie GA, Hyppa RW, Taylor AF, Zakharyevich K, Hunter N, Smith GR. Single Holliday junctions are intermediates of meiotic recombination. Cell 2006; 127:1167 - 78; http://dx.doi.org/10.1016/j.cell.2006.09.050; PMID: 17174892
- Kaliraman V, Brill SJ. Role of SGS1 and SLX4 in maintaining rDNA structure in Saccharomyces cerevisiae. Curr Genet 2002; 41:389 - 400; http://dx.doi.org/10.1007/s00294-002-0319-6; PMID: 12228808
- Gaillard P-HL, Noguchi E, Shanahan P, Russell P. The endogenous Mus81-Eme1 complex resolves Holliday junctions by a nick and counternick mechanism. Mol Cell 2003; 12:747 - 59; http://dx.doi.org/10.1016/S1097-2765(03)00342-3; PMID: 14527419
- Tay YD, Wu L. Overlapping roles for Yen1 and Mus81 in cellular Holliday junction processing. J Biol Chem 2010; 285:11427 - 32; http://dx.doi.org/10.1074/jbc.M110.108399; PMID: 20178992
- Ho CK, Mazón G, Lam AF, Symington LS. Mus81 and Yen1 promote reciprocal exchange during mitotic recombination to maintain genome integrity in budding yeast. Mol Cell 2010; 40:988 - 1000; http://dx.doi.org/10.1016/j.molcel.2010.11.016; PMID: 21172663
- Ashton TM, Mankouri HW, Heidenblut A, McHugh PJ, Hickson ID. Pathways for Holliday junction processing during homologous recombination in Saccharomyces cerevisiae. Mol Cell Biol 2011; 31:1921 - 33; http://dx.doi.org/10.1128/MCB.01130-10; PMID: 21343337
- Mazón G, Lam AF, Ho CK, Kupiec M, Symington LS. The Rad1-Rad10 nuclease promotes chromosome translocations between dispersed repeats. Nat Struct Mol Biol 2012; 19:964 - 71; http://dx.doi.org/10.1038/nsmb.2359; PMID: 22885325
- Szakal B, Branzei D. Premature Cdk1/Cdc5/Mus81 pathway activation induces aberrant replication and deleterious crossover. EMBO J 2013; 32:1155 - 67; http://dx.doi.org/10.1038/emboj.2013.67; PMID: 23531881
- Gallo-Fernández M, Saugar I, Ortiz-Bazán MÁ, Vázquez MV, Tercero JA. Cell cycle-dependent regulation of the nuclease activity of Mus81-Eme1/Mms4. Nucleic Acids Res 2012; 40:8325 - 35; http://dx.doi.org/10.1093/nar/gks599; PMID: 22730299
- Matos J, Blanco MG, West SC. Cell-Cycle Kinases Coordinate the Resolution of Recombination Intermediates with Chromosome Segregation. Cell Rep 2013;1–11.
- Kosugi S, Hasebe M, Tomita M, Yanagawa H. Systematic identification of cell cycle-dependent yeast nucleocytoplasmic shuttling proteins by prediction of composite motifs. Proc Natl Acad Sci U S A 2009; 106:10171 - 6; http://dx.doi.org/10.1073/pnas.0900604106; PMID: 19520826
- Loog M, Morgan DO. Cyclin specificity in the phosphorylation of cyclin-dependent kinase substrates. Nature 2005; 434:104 - 8; http://dx.doi.org/10.1038/nature03329; PMID: 15744308
- Visintin R, Craig K, Hwang ES, Prinz S, Tyers M, Amon A. The phosphatase Cdc14 triggers mitotic exit by reversal of Cdk-dependent phosphorylation. Mol Cell 1998; 2:709 - 18; http://dx.doi.org/10.1016/S1097-2765(00)80286-5; PMID: 9885559
- Jin F, Liu H, Liang F, Rizkallah R, Hurt MM, Wang Y. Temporal control of the dephosphorylation of Cdk substrates by mitotic exit pathways in budding yeast. Proc Natl Acad Sci U S A 2008; 105:16177 - 82; http://dx.doi.org/10.1073/pnas.0808719105; PMID: 18845678
- Stegmeier F, Amon A. Closing mitosis: the functions of the Cdc14 phosphatase and its regulation. Annu Rev Genet 2004; 38:203 - 32; http://dx.doi.org/10.1146/annurev.genet.38.072902.093051; PMID: 15568976
- Rock JM, Amon A. The FEAR network. Curr Biol 2009; 19:R1063 - 8; http://dx.doi.org/10.1016/j.cub.2009.10.002; PMID: 20064401
- Ross KE, Cohen-Fix O. A role for the FEAR pathway in nuclear positioning during anaphase. Dev Cell 2004; 6:729 - 35; http://dx.doi.org/10.1016/S1534-5807(04)00128-5; PMID: 15130497
- Sullivan M, Higuchi T, Katis VL, Uhlmann F. Cdc14 phosphatase induces rDNA condensation and resolves cohesin-independent cohesion during budding yeast anaphase. Cell 2004; 117:471 - 82; http://dx.doi.org/10.1016/S0092-8674(04)00415-5; PMID: 15137940
- Torres-Rosell J, Machín F, Jarmuz A, Aragón L. Nucleolar segregation lags behind the rest of the genome and requires Cdc14p activation by the FEAR network. Cell Cycle 2004; 3:496 - 502; http://dx.doi.org/10.4161/cc.3.4.802; PMID: 15004526
- Machín F, Torres-Rosell J, Jarmuz A, Aragón L. Spindle-independent condensation-mediated segregation of yeast ribosomal DNA in late anaphase. J Cell Biol 2005; 168:209 - 19; http://dx.doi.org/10.1083/jcb.200408087; PMID: 15657393
- Khmelinskii A, Lawrence C, Roostalu J, Schiebel E. Cdc14-regulated midzone assembly controls anaphase B. J Cell Biol 2007; 177:981 - 93; http://dx.doi.org/10.1083/jcb.200702145; PMID: 17562791
- Jaspersen SL, Charles JF, Tinker-Kulberg RL, Morgan DO. A late mitotic regulatory network controlling cyclin destruction in Saccharomyces cerevisiae. Mol Biol Cell 1998; 9:2803 - 17; http://dx.doi.org/10.1091/mbc.9.10.2803; PMID: 9763445
- Jaspersen SL, Morgan DO. Cdc14 activates cdc15 to promote mitotic exit in budding yeast. Curr Biol 2000; 10:615 - 8; http://dx.doi.org/10.1016/S0960-9822(00)00491-7; PMID: 10837230
- Sanchez-Diaz A, Nkosi PJ, Murray S, Labib K. The Mitotic Exit Network and Cdc14 phosphatase initiate cytokinesis by counteracting CDK phosphorylations and blocking polarised growth. EMBO J 2012; 31:3620 - 34; http://dx.doi.org/10.1038/emboj.2012.224; PMID: 22872148
- Shou W, Seol JH, Shevchenko A, Baskerville C, Moazed D, Chen ZW, Jang J, Shevchenko A, Charbonneau H, Deshaies RJ. Exit from mitosis is triggered by Tem1-dependent release of the protein phosphatase Cdc14 from nucleolar RENT complex. Cell 1999; 97:233 - 44; http://dx.doi.org/10.1016/S0092-8674(00)80733-3; PMID: 10219244
- Ghaemmaghami S, Huh W-K, Bower K, Howson RW, Belle A, Dephoure N, O’Shea EK, Weissman JS. Global analysis of protein expression in yeast. Nature 2003; 425:737 - 41; http://dx.doi.org/10.1038/nature02046; PMID: 14562106
- D’Amours D, Stegmeier F, Amon A. Cdc14 and condensin control the dissolution of cohesin-independent chromosome linkages at repeated DNA. Cell 2004; 117:455 - 69; http://dx.doi.org/10.1016/S0092-8674(04)00413-1; PMID: 15137939
- Hennessy KM, Lee A, Chen E, Botstein D. A group of interacting yeast DNA replication genes. Genes Dev 1991; 5:958 - 69; http://dx.doi.org/10.1101/gad.5.6.958; PMID: 2044962
- Dulev S, de Renty C, Mehta R, Minkov I, Schwob E, Strunnikov A. Essential global role of CDC14 in DNA synthesis revealed by chromosome underreplication unrecognized by checkpoints in cdc14 mutants. Proc Natl Acad Sci U S A 2009; 106:14466 - 71; http://dx.doi.org/10.1073/pnas.0900190106; PMID: 19666479
- Janke C, Magiera MM, Rathfelder N, Taxis C, Reber S, Maekawa H, Moreno-Borchart A, Doenges G, Schwob E, Schiebel E, et al. A versatile toolbox for PCR-based tagging of yeast genes: new fluorescent proteins, more markers and promoter substitution cassettes. Yeast 2004; 21:947 - 62; http://dx.doi.org/10.1002/yea.1142; PMID: 15334558
- Quevedo O, García-Luis J, Matos-Perdomo E, Aragón L, Machín F. Nondisjunction of a single chromosome leads to breakage and activation of DNA damage checkpoint in G2. PLoS Genet 2012; 8:e1002509; http://dx.doi.org/10.1371/journal.pgen.1002509; PMID: 22363215