Abstract
TRPC4 and TRPC5 channels are important regulators of electrical excitability in both gastrointestinal myocytes and neurons. Much is known regarding the assembly and function of these channels including TRPC1 as a homotetramer or a heteromultimer and the roles that their interacting proteins play in controlling these events. Further, they are one of the best-studied targets of G protein-coupled receptors and growth factors in general and Gαq protein coupled receptor or epidermal growth factor in particular. However, our understanding of the roles of Gαi/o proteins on TRPC4/5 channels is still rudimentary. We discuss potential roles for Gαi/o proteins in channel activation in addition to their known role in cellular signaling.
Introduction
The canonical or classic transient receptor potential ion channel (TRPC) subfamily is one of three TRP subfamilies known to mediate receptor-operated and store-operated Ca2+ entry.Citation1 TRPC channels are activated by G protein-coupled receptors, such as muscarinic receptors, adrenergic receptors, PAR receptors and serotonin receptors. The seven mammalian TRPC channels are divided into four subgroups, TRPC1, TRPC2, TRPC3/6/7 and TRPC4/5, based on sequence similarities. TRPC4 and TRPC5 are close homologs, sharing 64% identity. The heterotrimeric guanine nucleotide–binding proteins (G proteins) consist of four families, that is, Gαs, Gαi, Gαq/11 and Gα12/13 protein. Our focus here will be on the roles of heterotrimeric G proteins in the activation of TRPC4 and TRPC5 channels. Interested readers are directed to more comprehensive recent reviews on other aspects of TRPC channel function.Citation2-Citation4
TRPC4 and TRPC5 Channels
The TRPC4 and TRPC5 channels share many functional characteristics: both are potentiated by G protein-coupled receptors (GPCRs) that couple to Gαq/11 and/or Gαi/o and the two channels have similar current-voltage relationships.Citation5-Citation7 Homomeric TRPC4 or TRPC5 subunits underlie currents with a unique doubly rectifying current-voltage relationship and single-channel conductances of 42 and 66 pS, respectively. We also recorded the similar single channel activities from HEK cells stably expressing TRPC4β (). The unitary conductance and voltage dependence of the open probabilities are similar to those of single smooth muscle cells isolated from the gastrointestinal (GI) tract.Citation8
Figure 1. Single-channel currents recorded in the outside-out configuration from HEK293 cells that were stably expressing TRPC4β. (A) Representative single-channel current traces were recorded at voltages ranging from +80 to -140 mV. The holding potentials were 80, 30, -60 and -120 mV in A. GTPγS (200 μM) was intracellularly applied to activate the TRPC4 channel under the condition of 125 mM [Cs+]i and 125 mM [Cs+]o and nominally [Ca2+]o-free. (B) The corresponding I-V relationships from each potential are shown in the upper panel and the red line represents the fit to obtain a unitary conductance. The plot of NPo was estimated from each potential (bottom panel).
![Figure 1. Single-channel currents recorded in the outside-out configuration from HEK293 cells that were stably expressing TRPC4β. (A) Representative single-channel current traces were recorded at voltages ranging from +80 to -140 mV. The holding potentials were 80, 30, -60 and -120 mV in A. GTPγS (200 μM) was intracellularly applied to activate the TRPC4 channel under the condition of 125 mM [Cs+]i and 125 mM [Cs+]o and nominally [Ca2+]o-free. (B) The corresponding I-V relationships from each potential are shown in the upper panel and the red line represents the fit to obtain a unitary conductance. The plot of NPo was estimated from each potential (bottom panel).](/cms/asset/ce396622-597b-4463-baeb-e1a0f72e480f/kchl_a_10921198_f0001.gif)
In addition, TRPC4 and TRPC5 channels are unique among TRP channels in that they are potentiated by micromolar concentrations of the trivalent cations La3+ and Gd3+ independently of GPCR.Citation6,Citation9 In our experiments, TRPC5 channels are potentiated by micromolar concentrations of the trivalent cations La3+ and Gd3+ with bath solutions that mainly contain Na but inhibited with a 140 mM Cs solution.Citation10 However, TRPC4 was not potentiated by micromolar concentrations of the trivalent cations La3+ and Gd3+ even with a bath solution containing mainly Na+.Citation11 Other modulations include the potentiation of TRPC5 channels by extracellular thioredoxinCitation12 and nitric oxide,Citation13 heavy metalsCitation14,Citation15 and lipidsCitation16,Citation17; the inhibition of outward TRPC5 currents by intracellular Mg2+Citation18; and the desensitization of TRPC5 channels by PKC-mediated phosphorylationCitation19,Citation20 ( and ). In the studies showing the effect of redox on TRPC5, the authors used the C553 and C558 mutants.Citation12,Citation13 In our experiments, however, we could not record any currents from the C553A, C553S, C558A, C558S mutants of TRPC5.Citation10 Additionally, the NO-cGMP-PKG pathway may modulate TRPC4-mediated SOC.Citation21 In heterologous expression systems, homomeric TRPC5 channels can be rapidly delivered to the plasma membrane after stimulation of growth factor receptors via Rac, phosphatidylinositol 3 kinase and phosphatidylinositol 5 kinase.Citation22 As previously mentioned, we showed that homomeric TRPC5 channels can also be rapidly delivered to the plasma membrane via the CaM-MLCK pathway.Citation23 In addition, CaM itself was reported to accelerate TRPC5 agonist-activated currents via a CaM-binding site located at the C terminus of TRPC5 but not via the CaM/Ins(1,4,5)P3R binding (CIRB) domain.Citation24 Both TRPC4 and TRPC5 contain a C-terminal PDZ-binding motif (VTTRL). PDZ-domain scaffolding proteins, such as the Na+/H+ exchanger regulatory factor (NHERF) and signaling molecules, such as PLCβ1, have been reported to coimmunoprecipitate with TRPC4 and TRPC5.Citation25 The C-terminal PDZ (PSD-95, Dlg, ZO-1) motif of TRPC4 has been implicated in the control of channel surface expression and localization.Citation26 Deletion of the PDZ motif (T-R-L) of hTRPC4 dramatically reduced the plasma membrane levels of the channel, which led the authors to suggest that the interaction between hTRPC4 and NHERF is required for the retention and stabilization of hTRPC4 channels in the cell membrane.Citation20,Citation26 The activity of rat TRPC5 channels has also been shown to be influenced by interactions with NHERF.Citation27 In our experiments, deletion of the PDZ motif (T-R-L) in mouse TRPC5 dramatically reduced the plasma membrane levels of the channel.Citation20
Figure 2. Positive regulatory mechanisms of TRPC4 and TRPC5 channels. TRPC4 and TRPC5 channels are activated by many signals. Extracellular chemical signals, such as the GPCR agonist, lipids, metal ions, EGF and NO, activate TRPC4 and TRPC5 channels directly via Gi/o proteins, calcium, CaM, nitrosylation and PIP2 or indirectly via store depletion and exocytosis.
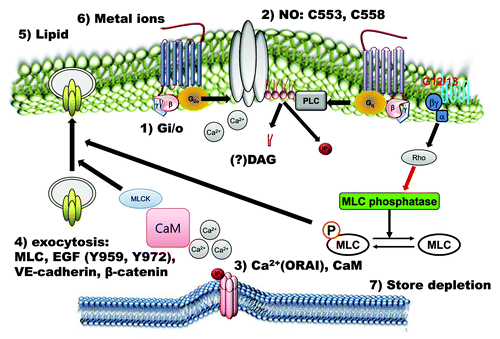
Figure 3. Negative regulatory mechanisms of TRPC4 and TRPC5 channels. Phosphorylation of TRPC4 and TRPC5 channels by PKA and PKC works as an inactivation pathway of TRPC4 and TRPC5 channels. On the other hand, PtdIns(4,5)P2 hydrolysis negatively regulates TRPC4 and TRPC5.
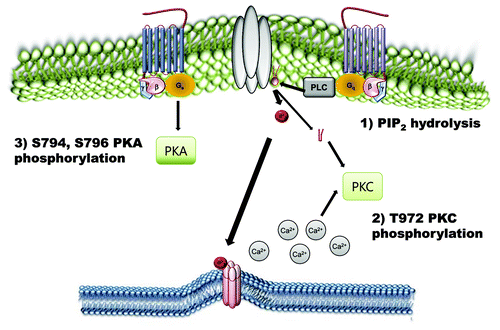
The most compelling experimental evidence for a role of a TRPC protein in store-operated Ca2+ entry came from studies on the TRPC4 null mouse, where TRPC4 was identified as an essential component of store-operated Ca2+ (SOC) channels in aortic endothelial cells.Citation28 Interestingly, evidence for a role of TRPC channels in receptor-operated Ca2+ (ROC) entry include the finding that isolated endothelial cells from the TRPC4 knockout mouse exhibited reduced Ca2+ entry in response to acetylcholine, ATP and thrombin application.Citation28,Citation29 Clearly, both store-operated and receptor-operated mechanisms can contribute to the activation of TRPC channels, particularly TRPC4. On the other hand, the most experimental evidence suggests that receptor-operated mechanisms contribute to the activation of TRPC5 channel. The matter about whether store-operated or/and receptor-operated mechanisms contribute to the activation of TRPC4 channels is especially important for the activation mechanism of epidermal growth factor (EGF) on TRPC4.
The addition of EGF causes an increase in the free cytoplasmic calcium concentration ([Ca2+]i), which completely depends on extracellular Ca2+ levels. More recently, however, several studies showed that EGF induced complex oscillatory changes in [Ca2+]i due to both a release of Ca2+ from the endoplasmic reticulum and a Ca2+ influx from the outer medium.Citation30 TRPC4 was involved in the activation of a store-operated current in corneal epithelial cells by EGF through an unknown mechanism.Citation31 Two tyrosine residues in the C terminus of human TRPC4 (hTRPC4), Tyr-959 and Tyr-972, were phosphorylated following EGF receptor (EGFR) stimulation of COS-7 cells.Citation32 This phosphorylation was mediated by Src family tyrosine kinases (STKs), with Fyn appearing to be the dominant kinase. In addition, EGF-receptor stimulation induced the exocytotic insertion of hTRPC4 into the plasma membrane in a manner dependent on the activity of STKs; this insertion was accompanied by a phosphorylation-dependent increase in the association of hTRPC4 with the Na+/H+ exchanger regulatory factor. In addition, the amino acids 730–758 of hTRPC4 were critical for the interaction with the spectrin cytoskeleton and a direct interaction between hTRPC4 and the spectrin cytoskeleton was involved in the regulation of hTRPC4 surface expression and activation.Citation33 We also expressed the TRPC4 deletion mutant without the spectrin binding domain (Δ730–758).Citation34 Intracellular GTPγS induced a small current, whereas the constitutively active form of Gαi2 induced a larger TRPC4 current, suggesting that Gαi2 can activate the TRPC4 channel independently of spectrin interaction. On the contrary, EGF led to a profound increase in TRPC4 ubiquitination via EGFR-MEKK1-AIP4 pathway and decreased the activity of TRPC4.Citation35 Thus, EGF has dual opposite effects: (1) enhancement of the degradation of TRPC4 and (2) exocytic surface expression and activation of TRPC4 via interaction with NHERF and spectrin. These opposing effects suggest that a delicate balance between the enhancement and inhibition effects on TRPC4 might be important for pathophysiological effect at in vivo situation.
The Gαi family was originally identified by their ability to inhibit adenylyl cyclase. The Gαi proteins, which include Gαi1, Gαi2 and Gαi3, among others, are highly similar, with more than 94% sequence identity between Gαi1 and Gαi3. Hormones and neurotransmitters use Gαi proteins to trigger physiological responses. The pertussis toxin (PTX)-sensitive Gαi/o proteins activate nonselective cation channels in single smooth muscle cells isolated from the gastrointestinal (GI) tract. We recently showed that Gαi/o proteins are important for the activation of TRPC4 and TRPC5.Citation34,Citation36 To determine which Gα isoform is involved in the activation of TRPC4 and TRPC5, we used constitutively active forms of the Gαi subunits (Gα QL mutants). Gαi2 and Gαi3 were the most potent activators for TRPC4 and TRPC5, respectively.Citation34 Constitutively active Gαi3 and Gαo mimicked the activation of TRPC4 by GTPγS. Constitutively active Gαi1 activated the TRPC4 channel, whereas application of GTPγS significantly inhibited the TRPC4 current. Gαi2 binds to C-terminal positively charged amino acids (K715 and R716) of TRPC4β via a negatively charged amino acid (D252) of the Gαi2 protein. Gαi3Q205L was the most effective activator of TRPC5, as all other Gα isoforms tested actually reduced the spontaneous current and GTPγS-induced currents, likely by competing with endogenous Gαi3. Interestingly, as in GIRK channels, Gαi/o proteins reduced the basal current of TRPC5 contrary to TRPC4 which were increased by all Gαi/o proteins. Although Gαi/o subunits are key activators of TRPC4 or TRPC5, Gβγ subunits may also be involved in the activation of the channels, as is the case with GIRK channels, or in the regulation of the channels by altering the availability of activated Gαi. To address these questions, we tested the effects of various Gβγ combinations on TRPC4 and TRPC5 activity. None of the Gβγ combinations tested activated the channels or reduced the activation by GTPγS.Citation34
Activation of the Gαq-PLC pathway has been shown to modestly activate TRPC4 and TRPC5 by an unknown mechanism. Hence, the inhibition of TRPC4/5 by the constitutively active Gαq was completely unexpected in our experiments.Citation34,Citation36 These results imply that intense overstimulation of a Gαq-activated pathway inhibits TRPC4 and TRPC5. We considered several potential mechanisms, including increased or decreased cytoplasmic Ca2+, interference of channel interaction with Gαi, reduced surface expression of TRPC4, modified cellular PtdIns(4,5)P2 and channel phosphorylation by PKC, known to induce desensitization of TRPC5 with phosphorylation of residue Thr-972 at the C terminus. Our experimental findings ruled out the effects of changes in PKC and altered interaction with Gαi in channel inhibition by Gαq as the major inhibitors of the current induced by activated Gαq. Moreover, they pointed to an indirect effect of Gαq on channel function. Our results indicated that PtdIns(4,5)P2 and perhaps increased cytoplasmic Ca2+ are required for TRPC4 and TRPC5 activation. In our previous study, we used TRPC4β isoform to test the effect of PtdIns(4,5)P2. The effects of PtdIns(4,5)P2 on TRPC4 and TRPC5 channels are controversial. One report showed that PtdIns(4,5)P2 inhibited TRPC4α but not TRPC4βCitation37 whereas others showed the stimulation of TRPC4 and TRPC5 channels by PtdIns(4,5)P2.Citation34,Citation38 In addition, Otsuguro et al.Citation37 showed that deletion of the PDZ binding domain (ΔTTRL) prevented PtdIns(4,5)P2 dependent TRPC4α inhibition. They also suggested a novel mechanistic model of TRPC4 gating, whereby TRPC4 interaction via its C-terminal PDZ-binding domain with the adaptor NHERF, ERM proteins and cortical actin is necessary for keeping the Δ84AA stretch close to the inner surface of the plasma membrane, thus stabilizing its binding with PtdIns(4,5)P2. Further studies are needed for solving the controversy about the roles of PtdIns(4,5)P2 on TRPC4. As in GIRK2 channel, PtdIns(4,5)P2 alone did not activate TRPC4 channels.Citation34,Citation37,Citation38
Isoproterenol elicited both positive and negative effects on TRPC5 depending on the constituents of the internal solution, GTPγS and Ins(1,4,5)P3, even when only endogenous levels of β2-adrenergic receptors were present in HEK293 cells. When the β2-adrenergic receptor was co-expressed with TRPC5 in HEK293 cells, it facilitated the effect of isoproterenol on TRPC5 channel.Citation11 β-adrenergic stimulation followed by the Gαs cascade induces a Ca2+ release mediated by Ins(1,4,5)P3R and this Ca2+ release potentiates TRPC5 channels. The cascade also promotes the plasma membrane translocation of TRPC5 channels. In addition, the furthest downstream effector molecule, PKA, phosphorylates and inhibits the TRPC5 channel. We reported previously that Gαs negatively regulated GTPγS-activated TRPC4 and TRPC5.Citation39 GTPγS-activated TRPC5 current was inhibited by treatment of isoproterenol (ISO), forskolin (FSK) and 8-Br-cAMP. Co-expression of constitutively active form of Gαs, GαsQ227L, also inhibited GTPγS-activated TRPC4 and TRPC5 current. Gαs-cAMP-PKA pathway inhibited TRPC5 current via phosphorylation of Ser794 and Ser796 site at C-terminus of TRPC5. GTPγS is one of the well-established TRPC4/5 activators.Citation34,Citation36 Under the GTPγS activation system, we would have dominantly observed negative regulation of TRPC5 by PKA. When we infused a solution containing both Ins(1,4,5)P3 and GTPγS, a decrease in TRPC5 current in response to ISO was observed, but the inhibition was considerably larger. When we alleviated the effect of the β-adrenergic stimulation-dependent Ca2+ response by using a pipette solution representing a high free Ca2+ state, ISO inhibited GTPγS-activated TRPC5 when intracellular Ca2+ was buffered to 5 μM. Similarly, Zhang et al.Citation40 suggested that cAMP is involved in TRPC4 function. The low-dose of ATP exerted part of its mitogenic effect in human pulmonary artery SMCs through cAMP response element-binding protein (CREB) mediated upregulation of TRPC4 channel surface expression and subsequent increase in Ca2+ influx. In their study treatment with ATP markedly increased TRPC4 expression through CREB phosphorylation, suggesting a possible role of TRPC4 in vascular remodeling during pathophysiological responses and its contribution to development of pulmonary hypertension.Citation40
Gα12/13-Rho-MLC phosphatase pathway might be involved in the activation process of TRPC5. In our previous study, carbachol-induced TRPC5 current was inhibited by CaM inhibitor and MLCK inhibitor whereas GTPγS-induced TRPC5 current was not.Citation23 Interestingly, application of both MLCK inhibitor and Rho kinase inhibitor to the bath blocked the TRPC5 current activated by GTPγS. On the other hand, constitutively active form of Gα12 and Gα13 did not show any effect on TRPC4.Citation36 Further studies about the interaction of small G proteins with TRPC4 and TRPC5 channels are needed.
TRPC5 is dramatically potentiated by intracellular Ca2+ in a manner that does not appear to involve calmodulin (CaM). Intracellular Ca2+ also potentiates TRPC4 channel activity to a lesser degree when the M1 receptor is expressed.Citation41 Gross et al.Citation42 also showed that the thapsigargin-induced release of Ca2+ from internal stores activated TRPC5 channels transiently and the subsequent Ca2+ entry produced a sustained TRPC5 activation, which in turn supported a long-lasting membrane depolarization. Furthermore, by co-expressing STIM1 plus ORAI1 or the α1C and β2 subunits of L-type Ca2+ channels, they found that Ca2+ entry through either calcium release-activated or voltage-dependent Ca2+ channels is sufficient for TRPC5 channel activation. The typical inward rectifying current-voltage (I-V) curve of CRAC (STIM1 plus ORAI1) and the bell-shaped I-V curve of voltage-dependent Ca2+ channels were recorded electrophysiologically before the appearance of the typical doubly rectifying I-V curve of TRPC5 channels. The Ca2+-entry activated TRPC5 channels with buffering of internal Ca2+ with EGTA but not with BAPTA. These data support the hypothesis that TRPC5 forms Ca2+-activated cation channels that are functionally coupled to Ca2+-selective ion channels through local Ca2+ increases beneath the plasma membrane.Citation42 In our experiments, intracellular Ca2+ does not potentiate TRPC4 channel activity without the co-expression of a muscarinic receptor, whereas it does potentiate TRPC5. In addition, even Gαi/o proteins need optimal intracellular calcium levels for the activation of TRPC4. When we used a pipette solution including 10 mM EGTA, the current decreased dramatically (). The activation was very slow compared with 0.5 mM EGTA, which we usually used for whole cell patch recording. These results were similar to the effect of Na+ on GIRK channels. However, Ca2+ itself was not enough for the activation of TRPC4 without Gαi/o proteins whereas internal Na itself can activate GIRK channels. GIRK2 channel is known to be activated by intracellular Na with an EC50 of 30–40 mM. Sodium activation of GIRK channels is thought to serve an important physiological function by producing negative feedback on excessive electrical activity. On the other hand, initial calcium facilitation of TRPC4 and TRPC5 channels is a kind of positive feedback and later typical PKC produces negative feedback on excessive TRPC4 and TRPC5 activity.
Figure 4. Current trace in the activation of TRPC4β by Gαi2. (A) Representative current traces recorded at -60 mV. The time course of activation of TRPC4β by Gαi2 was delayed in the presence of 10 mM EGTA in the patch pipette, in contrast to the current recorded with the low-calcium buffered internal solution (0.5 mM EGTA). (B) The current-voltage (I-V) relationship of TRPC4 showed a typical doubly rectifying shape. The I-V relationship shown in a and b was obtained at point a and b of (A).
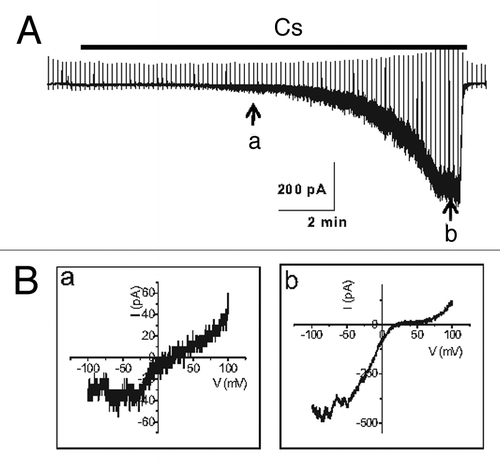
Gαi proteins are thought to play a role in EGF signaling based on its sensitivity to pertussis toxin (PTX).Citation43 EGF could activate TRPC5 channel electrophysiologically when recorded with patch clamp technique whereas EGF increased intracellular calcium or Mn2+ quenching in HEK cells expressing TRPC4 channel. Further studies why we could not record any current from TRPC4 channel by adding EGF are needed.
Nonselective Cation Channel Activated by Muscarinic Stimulation in the GI Tract
The muscarinic receptors play a key role in the parasympathetic nervous control of various peripheral tissues, including smooth muscles.Citation44-Citation46 The spontaneous activity of the gastrointestinal (GI) tract begins with the pacemaker potential from the interstitial cells of Cajal (ICCs).Citation46 The neurotransmitter acetylcholine, acting via muscarinic receptors on ICCs and smooth muscle cells, controls excitation-contraction coupling in GI smooth muscle cells.Citation45 Pharmacologic studies of gastrointestinal smooth muscle contraction in response to acetylcholine and other muscarinic agonists have suggested that M3 and M2 muscarinic receptors are involved in this process. While the M2 receptor is coupled functionally to pertussis toxin (PTX)-sensitive Gαi/o proteins, which when activated inhibit adenylyl cyclases and activate nonselective cation channels, the M3 receptor is coupled to PTX-insensitive Gαq/11 proteins that stimulate phospholipase Cβ (PLCβ).Citation45,Citation47 The latter leads to the breakdown of phosphoinositides and the production of inositol-1,4,5-trisphosphate (Ins(1,4,5)P3) and diacylglycerol (DAG). This signaling leads to an increase in intracellular [Ca2+] as a result of the release of Ca2+ from intracellular Ca2+ stores and the activation of Ca2+ entry. Intracellular Ca2+ release is triggered primarily by the binding of Ins(1,4,5)P3 to Ins(1,4,5)P3 receptors, whereas Ca2+ entry can potentially be mediated by several different mechanisms, such as store-operated or receptor-operated channels.
In various gastrointestinal smooth muscles, muscarinic receptor stimulation results in the generation of a nonselective cation current referred to as a muscarinic-activated cation current (mICat).Citation45,Citation47-Citation53 Intriguingly, the activation of mICat requires costimulation of both the M2 and M3 receptor subtypes.Citation48 Under physiologic ionic conditions, this current, primarily carried by Na+, causes depolarization of the cell, resulting in the activation of voltage-dependent Ca2+ channels.Citation44,Citation45 The net effect of these events is an increase in the cytosolic [Ca2+], which initiates contraction. Under physiologic conditions, the activation of voltage-dependent Ca2+ channels is critically dependent on the membrane potential change induced by mICat. Thus, the cationic channel activation, which occurs in response to muscarinic receptor stimulation and results in an influx of Na+ (and perhaps Ca2+53 as well), is suggested to be the primary and most important mechanism underlying the contractile response to cholinergic stimulation.
Members of the transient receptor potential canonical (TRPC) family have been receiving increasing attention as molecular candidates for the nonselective cationic channels that are activated by G-protein-coupled receptors in various tissues, including smooth muscles.Citation7,Citation38,Citation54,Citation55 TRPCs form Ca2+-permeable cation channels that are activated in response to the stimulation of plasma membrane GPCRs. TRPC channels have also been individually expressed in HEK293 cells and tested for their ability to generate currents similar to mICat of the gastric myocyte. Although a functional comparison demonstrated that the TRPC5 current exhibits an I–V relationship and relative ion selectivity similar to that of native mICat,Citation7,Citation56 the possible contribution of TRPC5 to intestinal smooth muscle contraction was not supported by expression data, which showed that the channel was not deleted in these tissues. In our experiments, we could detect TRPC5 in gastric smooth muscle cells with nested RT-PCR.Citation7 Initially, because we could not detect TRPC4 currents and because some results suggested that TRPC4 conducts SOC, we suggested that TRPC5 might be a molecular candidate for mICat.Citation7 However, similarities in the properties of heterologously expressed TRPC4 and native currents in intestinal smooth muscle suggested that this isoform may play a major role in the receptor-operated cation current mICat.Citation57 Subsequently, we could show that mICat was not recorded in TRPC4 knockout mice.Citation58 Importantly, the activation of TRPC4 requires PTX-sensitive G proteins and an increase in [Ca2+]i.Citation34,Citation36,Citation37 These properties are similar to those of mICat in native ileal smooth muscle cells.Citation47,Citation52 A recent study reported by Tsvilovskyy et al.Citation59 presented conclusive evidence that TRPC4 and TRPC6 function as two separate channels responsible for mICat. Their findings suggest that TRPC4 and TRPC6 couple muscarinic receptors to the depolarization of intestinal smooth muscle cells, voltage-activated Ca2+ influx and muscle contraction, consequently regulating small intestinal motility in vivo. Tsvilovskyy et al.Citation59 studied mICat activation and smooth muscle function in mice lacking TRPC4 alone, TRPC6 alone, or both TRPC4 and TRPC6. Their data showed that, in intestinal smooth muscle cells, TRPC4 forms a 55-pS cation channel whose currents contribute > 80% to mICat and TRPC6 currents contribute the remaining < 20%. There appeared to be no overlap of or compensation between the currents mediated by TRPC4 and TRPC6 in the single TRPC knockouts, whereas mICat was completely eliminated in the TRPC4/TRPC6 double knockouts. Furthermore, TRPC4-deficient ileal myocytes displayed greatly diminished carbachol-induced membrane depolarizations and atropine-sensitive contractions elicited by acetylcholine release from excitatory nerve terminals. Simultaneous deletion of TRPC6 aggravated these effects. Direct physiologic consequences were examined by assessing intestinal motility and clearance, both of which were reduced substantially in mice lacking TRPC4 and TRPC6. These findings demonstrated that TRPC4 and TRPC6 constitute the muscarinic receptor-activated channels in gastrointestinal smooth muscle cells and thereby, critically regulate smooth muscle contraction. However, they also showed that the Ca2+-dependent regulation of TRPC4, which includes facilitation at lower Ca2+ concentrations but inhibition at higher Ca2+ concentration, can explain the oscillations of the carbachol-induced membrane potential changes that were observed in the wild-type cells but not in the TRPC4-deficient myocytes. The TRPC4 channel can also account for the dependence of mICat on the M2 muscarinic receptor because TRPC4 activation involves PTX-sensitive Giα/o proteins, which is in contrast to TRPC6.Citation34,Citation36,Citation37 Therefore, TRPC4, the major contributor to mICat, appears to be the channel that requires costimulation of both the M2 and M3 signaling pathways. On the other hand, TRPC6, which has a smaller contribution to mICat (approximately 20%), is primarily activated via coupling to the M3 muscarinic receptor, which stimulates PLC-β and produces DAG.Citation59,Citation60 In the case of intestinal smooth muscle, the two channels seem to function independently but exert concurrent nonredundant effects on the tissue. Therefore, muscarinic receptors play a key role in the parasympathetic nervous control of the gastrointestinal tract. The neurotransmitter acetylcholine stimulates muscarinic receptors, M2 and M3, leading to the activation of cationic channel(s) and the generation of mICat, which depolarizes the cells, providing the critical trigger for the stimulation of voltage-dependent Ca2+ channels. The ensuing influx of Ca2+ is essential for contraction and intestinal motility. mICat is actually composed of currents from multiple channels: TRPC4 (> 80%) and TRPC6 (< 20%).
To understand the roles of muscarinic receptors in the parasympathetic nervous control of gastrointestinal tract, we should also consider the roles of ICCs on GI motilities.Citation61 The use of mutant animals with defective ICC networks (e.g., W/WV and Sl/Sld) has made it possible to learn more about the physiological role of ICCs. For example, postjunctional responses to excitatory and inhibitory neurotransmitters were reduced in the muscles of W/WV and Sl/Sld , which lack most of the intramuscular ICCs in the gastric fundus.Citation62,Citation63 The types of cells mediating cholinergic responses in GI muscles might be distinguished on the basis of the specific responses to neurotransmitter. Ca2+-activated Cl− currents were not observed in smooth muscle cells of the small intestine or stomach. Under current-clamp conditions, ICCs from the murine small intestine generated spontaneous transient depolarizations (STDs). Carbachol (100 nM–1 μM) depolarized resting membrane potential and increased the frequency of STDs.Citation64 Niflumic acid and 5-nitro-2-(3-phenylpropylamino)-benzoic acid (NPPB; both 100 μM) hyperpolarized cells and blocked STDs and responses to CCh. Recently, channels encoded by Tmem16a were suggested to be responsible for the enhanced inward current activated in the ICCs by muscarinic agonists and pacemaker channels.Citation65,Citation66 If different conductances are activated in smooth muscle cells and ICCs by muscarinic stimulation, then it might be possible to determine which type of cell generates the dominant responses to the ACh released from neurons. The electrical responses of intact muscles stimulated by either exogenous CCh or by cholinergic neurons were compared.Citation64 Their data suggested that ACh released from motor neurons primarily activates a Cl−conductance, which is expressed by ICCs. In the presence of niflumic acid, most of the response to ACh released from neurons was blocked. In contrast, the response of whole muscles exposed to exogenous CCh were not strongly affected by niflumic acid because most of this response may be due to the activation of non-selective cation conductances in smooth muscle cells. These data suggest that muscarinic regulation of GI muscles may depend upon the expression of Tmem16a in ICCs.Citation61 In conclusion, we should consider TMEM16A channels in the ICCs and the TRPC4 and TRPC6 channels as the muscarinic stimulators of the GI tract as a whole.
The Physiological Roles of TRPC4 in Endothelial Cells
Endothelial cells regulate endothelial functions, such as nitric oxide release and barrier stability, via intracellular Ca2+ signaling. Depending on the cell culture conditions, endothelial cells can adopt either a fibroblastoid, proliferative phenotype or, upon formation of cell-cell adhesions, the typical quiescent, epithelioid phenotype. The quiescent endothelial cell layer contains tight and adherens junctions; the latter provides the adhesive strength necessary for holding cells together physically and for the formation and maintenance of tight junctions.Citation67,Citation68 Cell-cell contact formation determines barrier function, inhibits endothelial proliferationCitation69 and has been recognized to govern the molecular organization of membrane-associated signaling complexes. Adhesion contacts between endothelial cells are mainly mediated by a vascular endothelium-specific member of the Ca2+-dependent adhesion molecules, VE-cadherin, which, like other classical cadherins, contains a cytoplasmic domain that is linked via catenin-type adaptor molecules to the cellular actin filament cytoskeleton.Citation70,Citation71 GPCR agonists (thrombin and histamine) and VEGF modulate the endothelial cell layer permeability by controlling tyrosine phosphorylation of the VE-cadherin/catenin complex.Citation72 The phenotypic change of vascular smooth muscle cell (SMC) from quiescent to synthetic is thought to be an integral part of the pathophysiological response of SMCs and is of paramount importance in the development of vascular disease. For instance, upon vascular injury the expression of TRPC channels is upregulated and is believed to take part in the definition of the proliferative migratory state of synthetic vascular SMCs.Citation73
The channels formed by the canonical transient receptor potential protein 4 (TRPC4) are expressed in endothelial cells and have repeatedly been suggested to be key determinants of endothelial Ca2+ signaling and of endothelial functions, such as nitric oxide release and barrier stability.Citation28,Citation29,Citation74,Citation75 In addition, TRPC5/TRPC4β channels have intact NO sensitivity because TRPC5 and hetero-multimeric TRPC5/TRPC1 and TRPC5/TRPC4 channels are likely to conduct native NO-activated Ca2+ influx in endothelial cells.Citation13 However, this concept was very recently questioned by the results of siRNA knockdown experiments in human macrovascular endothelial cells,Citation76 which argued against a prominent role for TRPC4 in endothelial Ca2+ signaling. Nonetheless, these authors confirmed a key role for TRPC4 as a determinant of endothelial proliferation. TRPC4 is a signaling molecule that is tightly associated with the actin cytoskeleton via a C-terminal PDZ interaction domain, which determines the membrane recruitment and the cellular targeting of functional channels.Citation26 Importantly, in addition to the debated role of TRPC4 as an endothelial Ca2+ channel (as ROC or SOC channels), it has repeatedly been demonstrated that TRPC4 governs the integrity of cell-cell junctions and barrier functions and thus, the endothelial phenotype.Citation29,Citation75
TRPC4 surface expression in human microvascular endothelial cells increased with the formation of cell-cell contacts.Citation77 Epidermal growth factor recruited TRPC4 into the plasma membrane of proliferating cells but initiated the retrieval of TRPC4 from the plasma membrane in quiescent, barrier-forming cells. Epidermal growth factor-induced Ca2+-entry was strongly promoted by the formation of cell-cell contacts. Both siRNA and dominant negative knockdown experiments revealed that TRPC4 mediates stimulated Ca2+-entry exclusively in proliferating clusters that form immature cell-cell contacts. TRPC4 co-precipitated with the junctional proteins β-catenin and VE-cadherin. Analysis of cellular localization of fluorescent fusion proteins provided further evidence for the recruitment of TRPC4 into junctional complexes. Analysis of TRPC4 function in the HEK293 expression system identified β-catenin as a signaling molecule that enables the cell-cell contact-dependent promotion of TRPC4 function. TRPC4 acted as a Ca2+-entry channel that is regulated by cell-cell contact formation and interaction with β-catenin. TRPC4 was suggested to conduct stimulated Ca2+ entry in a specific endothelial state during the transition from a proliferating to a quiescent phenotype. Thus, TRPC4 may emerge as a key player in endothelial phenotype-switching and the tuning of cellular growth factor signaling. We also observed that the confluence of the cell culture affected the expression level of TRPC4. Lower TRPC4 currents were recorded from cell cultures that were more confluent using the patch clamp method (unpublished data). Thus, the degree of cell confluence is very important when recording the TRPC4 current in a heterologous expression system. In addition, Zhang et al.Citation40 suggested CREB-mediated upregulation of TRPC4 channel surface expression and a possible role of TRPC4 in vascular remodeling during pathophysiological responses and its contribution to development of pulmonary hypertension. Considering interaction between EGFR and Gαi proteins, there is possibilities that EGFR-Gαi-TRPC4 pathway are important for stimulated Ca2+ entry in a specific endothelial state during the transition from a proliferating to a quiescent phenotype and vascular remodeling during pathophysiological responses.
As previously discussed, both the receptor-operated and the store-operated Ca2+ entry mechanisms can be activated in response to GPCR signaling. Studies from the past 10 y have suggested that TRPCs can contribute to both types of Ca2+-entry channels. Both TRPC1 and TRPC4 contribute subunits to the protein 4.1-bound channel.Citation78-Citation80 This channel was suggested to conduct a calcium-selective store-operated current, ISOC, in endothelia; ISOC is small (approximately 1.5 pA/pF), inwardly rectifying and possesses a reversal potential near +40 mV.Citation80-Citation83 ISOC is activated by thapsigargin, inositol 1,4,5-trisphosphate and TPEN, all of which deplete endoplasmic reticulum calcium. Questions remain as to whether ISOC is the same as the calcium-release activated calcium current, ICRAC, which displays similar biophysical properties to ISOC, although ICRAC is thought to be more highly calcium selective.Citation84-Citation86 Orai1 is a 4 transmembrane spanning domain protein that forms a pore through the coordination of four subunits and conducts ICRAC.Citation87-Citation91 Endothelial cells express Orai1.Citation86,Citation92,Citation93 Thapsigargin activates both ISOC and ICRAC in endothelial cells. Furthermore, ISOC controls permeability, whereas ICRAC regulates proliferation, migration and angiogenesis.Citation86 TRPC1 and TRPC4 can interact with STIM1 to form functional store-operated Ca2+-entry channels, which are essential for mediating the Ca2+-entry-dependent disruption of the endothelial barrier via thrombin.Citation94 To determine whether Orai1 may be an essential subunit of TRPC channels that is required for TRPC proteins to sense calcium store depletion and hence to be store-operated, Cioffi et al.Citation95 developed a means to enrich for the endogenous TRPC4 channel from endothelial cell membrane fractions, using protein 4.1 as bait. Using purified fractions, they probed for intermolecular interactions by Foerster resonance energy transfer (FRET). Their results indicated that Orai1 constitutively interacts with TRPC4 in endothelial cells and with TRPC1 after calcium store depletion. Furthermore, Orai1 increased the probability that TRPC4 will be activated after calcium store depletion, promoted the calcium selectivity of the TRPC4 channel and critically controlled the transmembrane calcium flux that serves as a proximal signal for endothelial cell barrier disruption.Citation95
Although the role for TRPC channels in SOCE is still a matter of controversy, data demonstrating that TRPCs mediate receptor-operated Ca2+ entry pathways are quite conclusive. All of the TRPC channels, except TRPC1, show the typical doubly rectifying current-voltage (I-V) relationship regardless of the presence of a stimulator such as GPCR agonists (acetylcholine, thrombin, serotonin, histamine) or EGF. In contrast, the I-V relationships of the SOC channels, except the CRAC channel, are not clear. Furthermore, a calcium measurement method with calcium removal and addition was used to investigate the roles of SOC channels in endothelial cells. When we use calcium measurement methods to study of the role of TRPC channels, we should consider that other calcium pathways may contribute to the calcium signal. To us knowledge, there is no report to show typical double rectifying I-V curve of TRPC4 in endothelial cells, even the outwardly rectifying I-V curve of TRPC1/TRPC4 or TRPC1/TRPC5 heterodimer. It seems that it is difficult to record TRPC current in flat and thin endothelial cells with patch clamp technique or the current amplitude is too small to record contrary to those in smooth muscle cells. On the other hand, it is rather easy to record GPCR-induced currents showing clearly typical double rectifying I-V curve of TRPC4 from smooth muscle cells which express TRPC4 channels. In the future, careful experiments about TRPC4 or TRPC1/TRPC4 heterodimer are needed in endothelial cells with patch clamp technique clearly showing their typical I-V curve.
Summary
The debate about the activation mechanism of TRPC4 channels began with the TRPC4 knockout mice. The first results suggested that TRPC4 is essential for SOC or CRAC channels because those results showed an inwardly rectifying I-V curve; however, subsequent studies showed that neurotransmitter-induced ROC channels were impaired in TRPC4 knockout mice. There are still possibilities that the new candidates for the CRAC channel, STIM and ORAI, bind with TRPC1 or TRPC4 channels and act as a SOC channel. Initially, it was much more difficult for us to record the TRPC4 current than the TRPC5 current, which was easily activated by the multiple pathways mentioned early in this review (). Based on the previous results from native GI tissue, we conducted the experiments with heterotrimeric G proteins and found that Gαi/o proteins are essential for the activation of TRPC4 and TRPC5. TRPC4 channels are responsible for the nonselective cation currents activated by muscarinic stimulation and act as a ROC channel in the GI tract. Even in endothelial cells, TRPC4 was responsible for acetylcholine-induced vasorelaxation, which in turn suggests that TRPC4 might be a ROC in endothelial cells. When we use calcium measurement methods to study of the role of TRPC channels, we should consider that other calcium pathways may contribute to the calcium signal. Because the experimental results that showed that TRPC4 is a SOC channel usually used calcium measurement methods, we should also record the TRPC4 currents with a patch clamp technique to confirm that TRPC4 acts as a SOC channel in endothelial cells. Importantly, two conserved acidic amino acids (DD) in the C-terminus of TRPCs have been identified as essential for STIM1-mediated gating of the channels,Citation96 which are localized within the putative C-terminal caveolin1(Cav1)-binding domain in TRPC4.Citation97 Thus, STIM1 may functionally regulate the activity of TRPC4 channels by preferentially modulating its interaction with Cav1 in lipid rafts. In aggregate, SOC channels are perhaps more complexly regulated; major proteins (TRPC1, TRPC4, Orai1, STIM1 and Cav1) are localized into specialized lipid raft compartments wherein their dynamic assembly and molecular rearrangements underlie one of the fundamental aspects of cellular Ca2+ signaling. We require more data that demonstrates whether the co-expression of TRPC4 with STIM or ORAI will show the expected doubly rectifying I-V curve, whether Gαi2 protein can activate TRPC4 and STIM complex and whether STIM and ORAI supply calcium for the activation of TRPC4 as they do for TRPC5 (). It will also be important to test whether TRPC4 currents can be recorded simultaneously with intracellular calcium concentration. Finally, as was done for GIRK,Citation98 we should study further the binding site for intracellular calcium itself, the effect of polyamine and Mg2+ on TRPC4 in the inside-out patch mode, the roles of PtdIns(4,5)P2 and PKC in the desensitization and the role of G proteins in channel assembly and trafficking in addition to their known role in cellular signaling.
Acknowledgments
We acknowledge the roles of the Biomedical Imaging Center at the Seoul National University College of Medicine, Seoul, Korea. This study was supported by a grant from the Korea Health 21 R&D project, Ministry of Health, Welfare and Family Affairs, Republic of Korea (A100079) and by the National Research Foundation of Korea funded by the Korean government (MEST) (2008-2005948, 2010-0019472 and 2012R1A2A1A01003073). C. Hong, J. P. Jeon, J. Wie and S.Y. Kim were supported by the graduate program of BK21 project from the Ministry of Education, Science and Technology (MEST).
References
- Clapham DE, Runnels LW, Strübing C. The TRP ion channel family. Nat Rev Neurosci 2001; 2:387 - 96; http://dx.doi.org/10.1038/35077544; PMID: 11389472
- Wu LJ, Sweet TB, Clapham DE. International Union of Basic and Clinical Pharmacology. LXXVI. Current progress in the mammalian TRP ion channel family. Pharmacol Rev 2010; 62:381 - 404; http://dx.doi.org/10.1124/pr.110.002725; PMID: 20716668
- Nilius B, Owsianik G. The transient receptor potential family of ion channels. Genome Biol 2011; 12:218; http://dx.doi.org/10.1186/gb-2011-12-3-218; PMID: 21401968
- Nilius B, Owsianik G. Transient receptor potential channelopathies. Pflugers Arch 2010; 460:437 - 50; http://dx.doi.org/10.1007/s00424-010-0788-2; PMID: 20127491
- Okada T, Shimizu S, Wakamori M, Maeda A, Kurosaki T, Takada N, et al. Molecular cloning and functional characterization of a novel receptor-activated TRP Ca2+ channel from mouse brain. J Biol Chem 1998; 273:10279 - 87; http://dx.doi.org/10.1074/jbc.273.17.10279; PMID: 9553080
- Schaefer M, Plant TD, Obukhov AG, Hofmann T, Gudermann T, Schultz G. Receptor-mediated regulation of the nonselective cation channels TRPC4 and TRPC5. J Biol Chem 2000; 275:17517 - 26; http://dx.doi.org/10.1074/jbc.275.23.17517; PMID: 10837492
- Lee YM, Kim BJ, Kim HJ, Yang DK, Zhu MH, Lee KP, et al. TRPC5 as a candidate for the nonselective cation channel activated by muscarinic stimulation in murine stomach. Am J Physiol Gastrointest Liver Physiol 2003; 284:G604 - 16; PMID: 12631560
- Kang TM, Kim YC, Sim JH, Rhee JC, Kim SJ, Uhm DY, et al. The properties of carbachol-activated nonselective cation channels at the single channel level in guinea pig gastric myocytes. Jpn J Pharmacol 2001; 85:291 - 8; http://dx.doi.org/10.1254/jjp.85.291; PMID: 11325022
- Strübing C, Krapivinsky G, Krapivinsky L, Clapham DE. TRPC1 and TRPC5 form a novel cation channel in mammalian brain. Neuron 2001; 29:645 - 55; http://dx.doi.org/10.1016/S0896-6273(01)00240-9; PMID: 11301024
- Kim MJ, Jeon JP, Kim HJ, Kim BJ, Lee YM, Choe H, et al. Molecular determinant of sensing extracellular pH in classical transient receptor potential channel 5. Biochem Biophys Res Commun 2008; 365:239 - 45; http://dx.doi.org/10.1016/j.bbrc.2007.10.154; PMID: 17981154
- Hong C, Kim J, Jeon JP, Wie J, Kwak M, Ha K, et al. Gs cascade regulates canonical transient receptor potential 5 (TRPC5) through cAMP mediated intracellular Ca2+ release and ion channel trafficking. Biochem Biophys Res Commun 2012; 421:105 - 11; http://dx.doi.org/10.1016/j.bbrc.2012.03.123; PMID: 22490661
- Xu SZ, Sukumar P, Zeng F, Li J, Jairaman A, English A, et al. TRPC channel activation by extracellular thioredoxin. Nature 2008; 451:69 - 72; http://dx.doi.org/10.1038/nature06414; PMID: 18172497
- Yoshida T, Inoue R, Morii T, Takahashi N, Yamamoto S, Hara Y, et al. Nitric oxide activates TRP channels by cysteine S-nitrosylation. Nat Chem Biol 2006; 2:596 - 607; http://dx.doi.org/10.1038/nchembio821; PMID: 16998480
- Sukumar P, Beech DJ. Stimulation of TRPC5 cationic channels by low micromolar concentrations of lead ions (Pb2+). Biochem Biophys Res Commun 2010; 393:50 - 4; http://dx.doi.org/10.1016/j.bbrc.2010.01.074; PMID: 20100462
- Xu SZ, Zeng B, Daskoulidou N, Chen GL, Atkin SL, Lukhele B. Activation of TRPC cationic channels by mercurial compounds confers the cytotoxicity of mercury exposure. Toxicol Sci 2012; 125:56 - 68; http://dx.doi.org/10.1093/toxsci/kfr268; PMID: 21984481
- Flemming PK, Dedman AM, Xu SZ, Li J, Zeng F, Naylor J, et al. Sensing of lysophospholipids by TRPC5 calcium channel. J Biol Chem 2006; 281:4977 - 82; http://dx.doi.org/10.1074/jbc.M510301200; PMID: 16368680
- Al-Shawaf E, Naylor J, Taylor H, Riches K, Milligan CJ, O’Regan D, et al. Short-term stimulation of calcium-permeable transient receptor potential canonical 5-containing channels by oxidized phospholipids. Arterioscler Thromb Vasc Biol 2010; 30:1453 - 9; http://dx.doi.org/10.1161/ATVBAHA.110.205666; PMID: 20378846
- Obukhov AG, Nowycky MC. TRPC5 channels undergo changes in gating properties during the activation-deactivation cycle. J Cell Physiol 2008; 216:162 - 71; http://dx.doi.org/10.1002/jcp.21388; PMID: 18247362
- Venkatachalam K, Zheng F, Gill DL. Regulation of canonical transient receptor potential (TRPC) channel function by diacylglycerol and protein kinase C. J Biol Chem 2003; 278:29031 - 40; http://dx.doi.org/10.1074/jbc.M302751200; PMID: 12721302
- Zhu MH, Chae M, Kim HJ, Lee YM, Kim MJ, Jin NG, et al. Desensitization of canonical transient receptor potential channel 5 by protein kinase C. Am J Physiol Cell Physiol 2005; 289:C591 - 600; http://dx.doi.org/10.1152/ajpcell.00440.2004; PMID: 15843439
- Wang X, Pluznick JL, Settles DC, Sansom SC. Association of VASP with TRPC4 in PKG-mediated inhibition of the store-operated calcium response in mesangial cells. Am J Physiol Renal Physiol 2007; 293:F1768 - 76; http://dx.doi.org/10.1152/ajprenal.00365.2007; PMID: 17913834
- Bezzerides VJ, Ramsey IS, Kotecha S, Greka A, Clapham DE. Rapid vesicular translocation and insertion of TRP channels. Nat Cell Biol 2004; 6:709 - 20; http://dx.doi.org/10.1038/ncb1150; PMID: 15258588
- Kim MT, Kim BJ, Lee JH, Kwon SC, Yeon DS, Yang DK, et al. Involvement of calmodulin and myosin light chain kinase in activation of mTRPC5 expressed in HEK cells. Am J Physiol Cell Physiol 2006; 290:C1031 - 40; http://dx.doi.org/10.1152/ajpcell.00602.2004; PMID: 16306123
- Ordaz B, Tang J, Xiao R, Salgado A, Sampieri A, Zhu MX, et al. Calmodulin and calcium interplay in the modulation of TRPC5 channel activity. Identification of a novel C-terminal domain for calcium/calmodulin-mediated facilitation. J Biol Chem 2005; 280:30788 - 96; http://dx.doi.org/10.1074/jbc.M504745200; PMID: 15987684
- Tang Y, Tang J, Chen Z, Trost C, Flockerzi V, Li M, et al. Association of mammalian trp4 and phospholipase C isozymes with a PDZ domain-containing protein, NHERF. J Biol Chem 2000; 275:37559 - 64; http://dx.doi.org/10.1074/jbc.M006635200; PMID: 10980202
- Mery L, Strauss B, Dufour JF, Krause KH, Hoth M. The PDZ-interacting domain of TRPC4 controls its localization and surface expression in HEK293 cells. J Cell Sci 2002; 115:3497 - 508; PMID: 12154080
- Obukhov AG, Nowycky MC. TRPC5 activation kinetics are modulated by the scaffolding protein ezrin/radixin/moesin-binding phosphoprotein-50 (EBP50). J Cell Physiol 2004; 201:227 - 35; http://dx.doi.org/10.1002/jcp.20057; PMID: 15334657
- Freichel M, Suh SH, Pfeifer A, Schweig U, Trost C, Weissgerber P, et al. Lack of an endothelial store-operated Ca2+ current impairs agonist-dependent vasorelaxation in TRP4-/- mice. Nat Cell Biol 2001; 3:121 - 7; http://dx.doi.org/10.1038/35055019; PMID: 11175743
- Tiruppathi C, Freichel M, Vogel SM, Paria BC, Mehta D, Flockerzi V, et al. Impairment of store-operated Ca2+ entry in TRPC4(-/-) mice interferes with increase in lung microvascular permeability. Circ Res 2002; 91:70 - 6; http://dx.doi.org/10.1161/01.RES.0000023391.40106.A8; PMID: 12114324
- Sánchez-González P, Jellali K, Villalobo A. Calmodulin-mediated regulation of the epidermal growth factor receptor. FEBS J 2010; 277:327 - 42; http://dx.doi.org/10.1111/j.1742-4658.2009.07469.x; PMID: 19951361
- Yang H, Mergler S, Sun X, Wang Z, Lu L, Bonanno JA, et al. TRPC4 knockdown suppresses epidermal growth factor-induced store-operated channel activation and growth in human corneal epithelial cells. J Biol Chem 2005; 280:32230 - 7; http://dx.doi.org/10.1074/jbc.M504553200; PMID: 16033767
- Odell AF, Scott JL, Van Helden DF. Epidermal growth factor induces tyrosine phosphorylation, membrane insertion, and activation of transient receptor potential channel 4. J Biol Chem 2005; 280:37974 - 87; http://dx.doi.org/10.1074/jbc.M503646200; PMID: 16144838
- Odell AF, Van Helden DF, Scott JL. The spectrin cytoskeleton influences the surface expression and activation of human transient receptor potential channel 4 channels. J Biol Chem 2008; 283:4395 - 407; http://dx.doi.org/10.1074/jbc.M709729200; PMID: 18048348
- Jeon JP, Hong C, Park EJ, Jeon JH, Cho NH, Kim IG, et al. Selective Gαi subunits as novel direct activators of TRPC4 and TRPC5 channels. J Biol Chem 2012; 287:17029 - 39; http://dx.doi.org/10.1074/jbc.M111.326553; PMID: 22457348
- Wegierski T, Hill K, Schaefer M, Walz G. The HECT ubiquitin ligase AIP4 regulates the cell surface expression of select TRP channels. EMBO J 2006; 25:5659 - 69; http://dx.doi.org/10.1038/sj.emboj.7601429; PMID: 17110928
- Jeon JP, Lee KP, Park EJ, Sung TS, Kim BJ, Jeon JH, et al. The specific activation of TRPC4 by Gi protein subtype. Biochem Biophys Res Commun 2008; 377:538 - 43; http://dx.doi.org/10.1016/j.bbrc.2008.10.012; PMID: 18854172
- Otsuguro K, Tang J, Tang Y, Xiao R, Freichel M, Tsvilovskyy V, et al. Isoform-specific inhibition of TRPC4 channel by phosphatidylinositol 4,5-bisphosphate. J Biol Chem 2008; 283:10026 - 36; http://dx.doi.org/10.1074/jbc.M707306200; PMID: 18230622
- Kim BJ, Kim MT, Jeon JH, Kim SJ, So I. Involvement of phosphatidylinositol 4,5-bisphosphate in the desensitization of canonical transient receptor potential 5. Biol Pharm Bull 2008; 31:1733 - 8; http://dx.doi.org/10.1248/bpb.31.1733; PMID: 18758068
- Sung TS, Jeon JP, Kim BJ, Hong C, Kim SY, Kim J, et al. Molecular determinants of PKA-dependent inhibition of TRPC5 channel. Am J Physiol Cell Physiol 2011; 301:C823 - 32; http://dx.doi.org/10.1152/ajpcell.00351.2010; PMID: 21734191
- Zhang S, Remillard CV, Fantozzi I, Yuan JX. ATP-induced mitogenesis is mediated by cyclic AMP response element-binding protein-enhanced TRPC4 expression and activity in human pulmonary artery smooth muscle cells. Am J Physiol Cell Physiol 2004; 287:C1192 - 201; http://dx.doi.org/10.1152/ajpcell.00158.2004; PMID: 15229105
- Blair NT, Kaczmarek JS, Clapham DE. Intracellular calcium strongly potentiates agonist-activated TRPC5 channels. J Gen Physiol 2009; 133:525 - 46; http://dx.doi.org/10.1085/jgp.200810153; PMID: 19398778
- Gross SA, Guzmán GA, Wissenbach U, Philipp SE, Zhu MX, Bruns D, et al. TRPC5 is a Ca2+-activated channel functionally coupled to Ca2+-selective ion channels. J Biol Chem 2009; 284:34423 - 32; http://dx.doi.org/10.1074/jbc.M109.018192; PMID: 19815560
- Cao C, Huang X, Han Y, Wan Y, Birnbaumer L, Feng GS, et al. Galpha(i1) and Galpha(i3) are required for epidermal growth factor-mediated activation of the Akt-mTORC1 pathway. Sci Signal 2009; 2:ra17; http://dx.doi.org/10.1126/scisignal.2000118; PMID: 19401591
- Bolton TB, Prestwich SA, Zholos AV, Gordienko DV. Excitation-contraction coupling in gastrointestinal and other smooth muscles. Annu Rev Physiol 1999; 61:85 - 115; http://dx.doi.org/10.1146/annurev.physiol.61.1.85; PMID: 10099683
- Unno T, Matsuyama H, Okamoto H, Sakamoto T, Yamamoto M, Tanahashi Y, et al. Muscarinic cationic current in gastrointestinal smooth muscles: signal transduction and role in contraction. Auton Autacoid Pharmacol 2006; 26:203 - 17; http://dx.doi.org/10.1111/j.1474-8673.2006.00366.x; PMID: 16879487
- Kim BJ, So I, Kim KW. The relationship of TRP channels to the pacemaker activity of interstitial cells of Cajal in the gastrointestinal tract. J Smooth Muscle Res 2006; 42:1 - 7; http://dx.doi.org/10.1540/jsmr.42.1; PMID: 16702759
- Inoue R, Isenberg G. Acetylcholine activates nonselective cation channels in guinea pig ileum through a G protein. Am J Physiol 1990; 258:C1173 - 8; PMID: 1694399
- Zholos AV, Bolton TB. Muscarinic receptor subtypes controlling the cationic current in guinea-pig ileal smooth muscle. Br J Pharmacol 1997; 122:885 - 93; http://dx.doi.org/10.1038/sj.bjp.0701438; PMID: 9384504
- Kim SJ, Ahn SC, So I, Kim KW. Quinidine blockade of the carbachol-activated nonselective cationic current in guinea-pig gastric myocytes. Br J Pharmacol 1995; 115:1407 - 14; PMID: 8564199
- Kim SJ, Ahn SC, So I, Kim KW. Role of calmodulin in the activation of carbachol-activated cationic current in guinea-pig gastric antral myocytes. Pflugers Arch 1995; 430:757 - 62; http://dx.doi.org/10.1007/BF00386173; PMID: 7478930
- Kim YC, Kim SJ, Sim JH, Jun JY, Kang TM, Suh SH, et al. Protein kinase C mediates the desensitization of CCh-activated nonselective cationic current in guinea-pig gastric myocytes. Pflugers Arch 1998; 436:1 - 8; http://dx.doi.org/10.1007/s004240050597; PMID: 9560440
- Kim YC, Kim SJ, Sim JH, Cho CH, Juhnn YS, Suh SH, et al. Suppression of the carbachol-activated nonselective cationic current by antibody against alpha subunit of Go protein in guinea-pig gastric myocytes. Pflugers Arch 1998; 436:494 - 6; http://dx.doi.org/10.1007/s004240050663; PMID: 9644236
- Kim SJ, Koh EM, Kang TM, Kim YC, So I, Isenberg G, et al. Ca2+ influx through carbachol-activated non-selective cation channels in guinea-pig gastric myocytes. J Physiol 1998; 513:749 - 60; http://dx.doi.org/10.1111/j.1469-7793.1998.749ba.x; PMID: 9824715
- Beech DJ, Muraki K, Flemming R. Non-selective cationic channels of smooth muscle and the mammalian homologues of Drosophila TRP. J Physiol 2004; 559:685 - 706; PMID: 15272031
- Venkatachalam K, Montell C. TRP channels. Annu Rev Biochem 2007; 76:387 - 417; http://dx.doi.org/10.1146/annurev.biochem.75.103004.142819; PMID: 17579562
- Plant TD, Schaefer M. TRPC4 and TRPC5: receptor-operated Ca2+-permeable nonselective cation channels. Cell Calcium 2003; 33:441 - 50; http://dx.doi.org/10.1016/S0143-4160(03)00055-1; PMID: 12765689
- Zholos AV, Zholos AA, Bolton TB. G-protein-gated TRP-like cationic channel activated by muscarinic receptors: effect of potential on single-channel gating. J Gen Physiol 2004; 123:581 - 98; http://dx.doi.org/10.1085/jgp.200309002; PMID: 15111646
- Lee KP, Jun JY, Chang IY, Suh SH, So I, Kim KW. TRPC4 is an essential component of the nonselective cation channel activated by muscarinic stimulation in mouse visceral smooth muscle cells. Mol Cells 2005; 20:435 - 41; PMID: 16404161
- Tsvilovskyy VV, Zholos AV, Aberle T, Philipp SE, Dietrich A, Zhu MX, et al. Deletion of TRPC4 and TRPC6 in mice impairs smooth muscle contraction and intestinal motility in vivo. Gastroenterology 2009; 137:1415 - 24; http://dx.doi.org/10.1053/j.gastro.2009.06.046; PMID: 19549525
- Hofmann T, Obukhov AG, Schaefer M, Harteneck C, Gudermann T, Schultz G. Direct activation of human TRPC6 and TRPC3 channels by diacylglycerol. Nature 1999; 397:259 - 63; http://dx.doi.org/10.1038/16711; PMID: 9930701
- Kenton M. Sanders, Mei Hong Zhu, Fiona Britton, Sang Don Koh and Sean M.Ward. Anoctamins and gastrointestinal smooth muscle excitability. Exp Physiol 2012;97.2:200–206, 2012.
- Ward SM, Beckett EAH, Wang X-Y, Baker F, Khoyi M, Sanders KM. Interstitial cells of Cajal mediate cholinergic neurotransmission from enteric motor neurons. J Neurosci 2000; 20:1393 - 403; PMID: 10662830
- Sanders KM, Hwang SJ, Ward SM. Neuroeffector apparatus in gastrointestinal smooth muscle organs. J Physiol 2010; 588:4621 - 39; http://dx.doi.org/10.1113/jphysiol.2010.196030; PMID: 20921202
- Zhu MH, Sung IK, Zheng H, Sung TS, Britton FC, O’Driscoll K, et al. Muscarinic activation of Ca2+-activated Cl- current in interstitial cells of Cajal. J Physiol 2011; 589:4565 - 82; PMID: 21768263
- Zhu MH, Kim TW, Ro S, Yan W, Ward SM, Koh SD, et al. A Ca(2+)-activated Cl(-) conductance in interstitial cells of Cajal linked to slow wave currents and pacemaker activity. J Physiol 2009; 587:4905 - 18; http://dx.doi.org/10.1113/jphysiol.2009.176206; PMID: 19703958
- Hwang SJ, Blair PJ, Britton FC, O’Driscoll KE, Hennig G, Bayguinov YR, et al. Expression of anoctamin 1/TMEM16A by interstitial cells of Cajal is fundamental for slow wave activity in gastrointestinal muscles. J Physiol 2009; 587:4887 - 904; http://dx.doi.org/10.1113/jphysiol.2009.176198; PMID: 19687122
- Corada M, Liao F, Lindgren M, Lampugnani MG, Breviario F, Frank R, et al. Monoclonal antibodies directed to different regions of vascular endothelial cadherin extracellular domain affect adhesion and clustering of the protein and modulate endothelial permeability. Blood 2001; 97:1679 - 84; http://dx.doi.org/10.1182/blood.V97.6.1679; PMID: 11238107
- Lampugnani MG, Dejana E. Interendothelial junctions: structure, signalling and functional roles. Curr Opin Cell Biol 1997; 9:674 - 82; http://dx.doi.org/10.1016/S0955-0674(97)80121-4; PMID: 9330871
- Dejana E, Orsenigo F, Molendini C, Baluk P, McDonald DM. Organization and signaling of endothelial cell-to-cell junctions in various regions of the blood and lymphatic vascular trees. Cell Tissue Res 2009; 335:17 - 25; http://dx.doi.org/10.1007/s00441-008-0694-5; PMID: 18855014
- Navarro P, Caveda L, Breviario F, Mândoteanu I, Lampugnani MG, Dejana E. Catenin-dependent and -independent functions of vascular endothelial cadherin. J Biol Chem 1995; 270:30965 - 72; http://dx.doi.org/10.1074/jbc.270.52.30965; PMID: 8537353
- Vestweber D. Molecular mechanisms that control endothelial cell contacts. J Pathol 2000; 190:281 - 91; http://dx.doi.org/10.1002/(SICI)1096-9896(200002)190:3<281::AID-PATH527>3.0.CO;2-Z; PMID: 10685062
- Vestweber D. VE-cadherin: the major endothelial adhesion molecule controlling cellular junctions and blood vessel formation. Arterioscler Thromb Vasc Biol 2008; 28:223 - 32; http://dx.doi.org/10.1161/ATVBAHA.107.158014; PMID: 18162609
- Gonzalez-Cobos JC, Trebak M. TRPC channels in smooth muscle cells. Front Biosci 2010; 15:1023 - 39; http://dx.doi.org/10.2741/3660; PMID: 20515740
- Wakabayashi I, Poteser M, Groschner K. Intracellular pH as a determinant of vascular smooth muscle function. J Vasc Res 2006; 43:238 - 50; http://dx.doi.org/10.1159/000091235; PMID: 16449818
- Cioffi DL, Stevens T. Regulation of endothelial cell barrier function by store-operated calcium entry. Microcirculation 2006; 13:709 - 23; http://dx.doi.org/10.1080/10739680600930354; PMID: 17085429
- Abdullaev IF, Bisaillon JM, Potier M, Gonzalez JC, Motiani RK, Trebak M. Stim1 and Orai1 mediate CRAC currents and store-operated calcium entry important for endothelial cell proliferation. Circ Res 2008; 103:1289 - 99; http://dx.doi.org/10.1161/01.RES.0000338496.95579.56; PMID: 18845811
- Graziani A, Poteser M, Heupel WM, Schleifer H, Krenn M, Drenckhahn D, et al. Cell-cell contact formation governs Ca2+ signaling by TRPC4 in the vascular endothelium: evidence for a regulatory TRPC4-beta-catenin interaction. J Biol Chem 2010; 285:4213 - 23; http://dx.doi.org/10.1074/jbc.M109.060301; PMID: 19996314
- Brough GH, Wu S, Cioffi D, Moore TM, Li M, Dean N, et al. Contribution of endogenously expressed Trp1 to a Ca2+-selective, store-operated Ca2+ entry pathway. FASEB J 2001; 15:1727 - 38; http://dx.doi.org/10.1096/fj.01-0108com; PMID: 11481220
- Hofmann T, Schaefer M, Schultz G, Gudermann T. Subunit composition of mammalian transient receptor potential channels in living cells. Proc Natl Acad Sci U S A 2002; 99:7461 - 6; http://dx.doi.org/10.1073/pnas.102596199; PMID: 12032305
- Cioffi DL, Wu S, Alexeyev M, Goodman SR, Zhu MX, Stevens T. Activation of the endothelial store-operated ISOC Ca2+ channel requires interaction of protein 4.1 with TRPC4. Circ Res 2005; 97:1164 - 72; http://dx.doi.org/10.1161/01.RES.0000193597.65217.00; PMID: 16254212
- Wu S, Sangerman J, Li M, Brough GH, Goodman SR, Stevens T. Essential control of an endothelial cell ISOC by the spectrin membrane skeleton. J Cell Biol 2001; 154:1225 - 33; http://dx.doi.org/10.1083/jcb.200106156; PMID: 11564759
- Wu S, Cioffi EA, Alvarez D, Sayner SL, Chen H, Cioffi DL, et al. Essential role of a Ca2+-selective, store-operated current (ISOC) in endothelial cell permeability: determinants of the vascular leak site. Circ Res 2005; 96:856 - 63; http://dx.doi.org/10.1161/01.RES.0000163632.67282.1f; PMID: 15790951
- Wu S, Chen H, Alexeyev MF, King JA, Moore TM, Stevens T, et al. Microtubule motors regulate ISOC activation necessary to increase endothelial cell permeability. J Biol Chem 2007; 282:34801 - 8; http://dx.doi.org/10.1074/jbc.M704522200; PMID: 17921144
- Fasolato C, Nilius B. Store depletion triggers the calcium release-activated calcium current (ICRAC) in macrovascular endothelial cells: a comparison with Jurkat and embryonic kidney cell lines. Pflugers Arch 1998; 436:69 - 74; http://dx.doi.org/10.1007/s004240050605; PMID: 9560448
- Fierro L, Lund PE, Parekh AB. Comparison of the activation of the Ca2+ release-activated Ca2+ current ICRAC to InsP3 in Jurkat T-lymphocytes, pulmonary artery endothelia and RBL-1 cells. Pflugers Arch 2000; 440:580 - 7; http://dx.doi.org/10.1007/s004240000336; PMID: 10958342
- Abdullaev IF, Bisaillon JM, Potier M, Gonzalez JC, Motiani RK, Trebak M. Stim1 and Orai1 mediate CRAC currents and store-operated calcium entry important for endothelial cell proliferation. Circ Res 2008; 103:1289 - 99; http://dx.doi.org/10.1161/01.RES.0000338496.95579.56; PMID: 18845811
- Vig M, Peinelt C, Beck A, Koomoa DL, Rabah D, Koblan-Huberson M, et al. CRACM1 is a plasma membrane protein essential for store-operated Ca2+ entry. Science 2006; 312:1220 - 3; http://dx.doi.org/10.1126/science.1127883; PMID: 16645049
- Feske S, Gwack Y, Prakriya M, Srikanth S, Puppel SH, Tanasa B, et al. A mutation in Orai1 causes immune deficiency by abrogating CRAC channel function. Nature 2006; 441:179 - 85; http://dx.doi.org/10.1038/nature04702; PMID: 16582901
- Zhang SL, Yeromin AV, Zhang XH, Yu Y, Safrina O, Penna A, et al. Genome-wide RNAi screen of Ca(2+) influx identifies genes that regulate Ca(2+) release-activated Ca(2+) channel activity. Proc Natl Acad Sci U S A 2006; 103:9357 - 62; http://dx.doi.org/10.1073/pnas.0603161103; PMID: 16751269
- Prakriya M, Feske S, Gwack Y, Srikanth S, Rao A, Hogan PG. Orai1 is an essential pore subunit of the CRAC channel. Nature 2006; 443:230 - 3; http://dx.doi.org/10.1038/nature05122; PMID: 16921383
- Vig M, Beck A, Billingsley JM, Lis A, Parvez S, Peinelt C, et al. CRACM1 multimers form the ion-selective pore of the CRAC channel. Curr Biol 2006; 16:2073 - 9; http://dx.doi.org/10.1016/j.cub.2006.08.085; PMID: 16978865
- Li J, Cubbon RM, Wilson LA, Amer MS, McKeown L, Hou B, et al. Orai1 and CRAC channel dependence of VEGF-activated Ca2+ entry and endothelial tube formation. Circ Res 2011; 108:1190 - 8; http://dx.doi.org/10.1161/CIRCRESAHA.111.243352; PMID: 21441136
- Cioffi DL, Barry C, Stevens T. Store-operated calcium entry channels in pulmonary endothelium: the emerging story of TRPCS and Orai1. Adv Exp Med Biol 2010; 661:137 - 54; http://dx.doi.org/10.1007/978-1-60761-500-2_9; PMID: 20204728
- Sundivakkam PC, Freichel M, Singh V, Yuan JP, Vogel SM, Flockerzi V, et al. The Ca(2+) sensor stromal interaction molecule 1 (STIM1) is necessary and sufficient for the store-operated Ca(2+) entry function of transient receptor potential canonical (TRPC) 1 and 4 channels in endothelial cells. Mol Pharmacol 2012; 81:510 - 26; http://dx.doi.org/10.1124/mol.111.074658; PMID: 22210847
- Cioffi DL, Wu S, Chen H, Alexeyev M, St Croix CM, Pitt BR, Uhlig S, Stevens T. Orai1 Determines Calcium Selectivity of an Endogenous TRPC Heterotetramer Channel. Circ Res. 2012 Apr 24. [Epub ahead of print] PubMed PMID: 22534489.
- Zeng W, Yuan JP, Kim MS, Choi YJ, Huang GN, Worley PF, et al. STIM1 gates TRPC channels, but not Orai1, by electrostatic interaction. Mol Cell 2008; 32:439 - 48; http://dx.doi.org/10.1016/j.molcel.2008.09.020; PMID: 18995841
- Pani B, Singh BB. Lipid rafts/caveolae as microdomains of calcium signaling. Cell Calcium 2009; 45:625 - 33; http://dx.doi.org/10.1016/j.ceca.2009.02.009; PMID: 19324409
- Zylbergold P, Ramakrishnan N, Hebert T. The role of G proteins in assembly and function of Kir3 inwardly rectifying potassium channels. Channels (Austin) 2010; 4:411 - 21; http://dx.doi.org/10.4161/chan.4.5.13327; PMID: 20855978