Abstract
The development of bilateral symmetry during the evolution of species probably 600 million years ago brought about several important innovations: It fostered efficient locomotion, streamlining, and favored the development of a central nervous system through cephalization. However, to increase their functional capacities, many organisms exhibit chirality by breaking their superficial left-right (l-r) symmetry, which manifests in the lateralization of the nervous system or the l-r asymmetry of internal organs. In most bilateria, the mechanisms that maintain consistent l-r asymmetry throughout development are poorly understood. This review highlights insights into mechanisms that couple early embryonic l-r symmetry breaking to subsequent l-r patterning in the roundworm Caenorhabditis elegans. A recently identified strategy for l-r patterning in the early C. elegans embryo is discussed, the spatial separation of midline and anteroposterior axis, which relies on a rotational cellular rearrangement and non-canonical Wnt signaling. Evidence for a general relevance of rotational/torsional rearrangements during organismal l-r patterning and for non-canonical Wnt signaling/planar cell polarity as a common signaling mechanism to maintain l-r asymmetry is presented.
Introduction
Most bilaterian animals appear l-r symmetric from the outside. However, they are mostly l-r asymmetric on the inside: Their internal structures show chirality or handedness such that the organism adopts either a right- or a left-handed form.Citation1–Citation4 In order to properly characterize these l-r asymmetries, two fundamental distinctions are important: (1) At the level of an individual organism, primary and secondary l-r asymmetries have to be distinguished. Primary l-r asymmetry arises during early developmentCitation5,Citation6 and determines the l-r asymmetry of internal organs, e.g., coiling of gut and genitalia, shape and position of the heart or laterality of the nervous system (). Secondary l-r asymmetry arises during later development, is not restricted to internal structures and forms independently of the primary. A prominent example is the dramatic difference in claw size of fiddler crabs or the l-r asymmetries in human faces. (2) At the level of a population three major forms of l-r asymmetry can be discerned: Directional l-r asymmetry, enantiomorphy and fluctuating l-r asymmetry. If a species shows directional l-r asymmetry it means that all individuals show the same chirality.Citation7 In the case of enantiomorphy both types of chirality can be found,Citation8 whereas fluctuating asymmetries refer to random handedness and degree of a specific asymmetric trait in a population.Citation9
This review focuses on primary l-r asymmetry and on mechanisms of directional l-r asymmetry. In most invertebrateCitation6 (e.g., snails, nematodes, Drosophila) and vertebrateCitation10 (e.g., Xenopus, zebrafish, mouse, rat) model organisms and ourselves an invariant form of primary l-r asymmetry exists. On the one hand this is surprising since there is no obvious functional difference and thus also no evolutionary selective pressure favoring either a right- or a left-handed form. This is especially apparent in the natural occurrence of rare individuals in species with invariant primary l-r asymmetry that are mirror-symmetric to the rest of the population (situs inversus totalis).Citation11,Citation12 These ‘reversed’ organisms are however functionally equivalent and phenotypically asymptomatic, although they may show only partial reversal of behavioral l-r asymmetries.Citation12 On the other hand, the development of a consistent chirality where all individual l-r asymmetric organs are oriented with respect to the global l-r polarization is essential for an organism to function properly: If local deviations from the global l-r direction occur during development, they often lead to severe phenotypic abnormalities as inconsistent individual l-r asymmetries can no longer integrate into a coherent body plan.Citation13–Citation15
The emergence of primary l-r asymmetry during early embryonic development has been noticed more than a century ago.Citation16 Ultimate validation that early l-r symmetry breaking determines all later directional asymmetries was obtained much later from micromanipulation experiments, first performed in C. elegans embryosCitation11 and more recently also in embryos of the snail Lymnaea stagnalisCitation17 (): In both cases, a chiral arrangement of blastomeres with a unique handedness is apparent at the six- or eight-cell stage embryo, respectively. When this invariant handedness is inverted by the application of external force, organisms develop that are mirror-symmetric in all anatomical aspects as compared to their non-manipulated wild-type counterparts; they exhibit a situs inversus totalis through mechanical manipulation.Citation11,Citation17 These reversal experiments reveal the logic of l-r symmetry breaking:Citation1,Citation5,Citation18 The decision on an organism's primary l-r asymmetry can be thought of as crossing a critical point early in development at which the system's chiral fate is determined by choosing only one of the branches of a bifurcation. The current paradigm for further l-r patterning is that, after crossing this initial bifurcation point, fields of asymmetric gene expression are established. In these fields, asymmetric cellular behaviors emerge that eventually lead to asymmetric morphogenesis. Both the theoretical logic and the actual reversal experiments indicate that in order to develop consistent directional l-r asymmetry, the initial chirality decision has to be propagated effectively.
With the frequent lack of clear morphological analogy between species,Citation6,Citation19 only a combination of morphological characterization and cellular/molecular analyses can reveal whether comparable mechanisms determine and propagate primary l-r asymmetries. Of particular current interest are genetic and morphogenetic pathways that mediate these subsequent l-r patterning events so that they align with the global direction of l-r asymmetry. These will be discussed in the following section for the roundworm C. elegans.
Establishing and Maintaining Directional L-R Asymmetry in C. elegans
As in any other organism, the unequivocally first symmetry breaking event in C. elegans is not known. However, as indicated above, the first apparent and functionally relevant sign of l-r asymmetry corresponds to the l-r asymmetric placement of blastomeres at the six-cell stage,Citation20,Citation21 where the left pair of ectodermal founder cells (ABal/ABpl) is invariantly located more anteriorly than the equivalent pair on the right (ABar/ABpr) (). This configuration is forced by external constraints: The cells start to divide perfectly orthogonal to the anteroposterior (a-p) and dorsoventral axis, their spindles can however not elongate freely and skew into an oblique orientation as space along l-r is limited by the incompressible egg shell.Citation22 Notably, this external constraint is at best only a partial guidance cue for the l-r bifurcation, as embryos without an egg shell exhibit only 5% reversals.Citation7,Citation22 Moreover, another nematode species, Acrobeloides bodenheimeri Steiner, has been describedCitation23 that shows reversed handedness as compared to all other known nematodes of the Rhabditia orderCitation24 (to which also C. elegans belongs), yet it develops in an egg shell with a comparable shape. Thus, the handedness of the ABa/p spindle skew seems to originate from an intrinsic l-r bias. It has been proposedCitation7 that a reversal must be mechanistically constrained similarly to what has been shown for the only other phylum that besides nematodes exhibits naturally occurring reversals, the Limnea and Physa snails, where sinistral forms are due to a recessive maternal-effect mutation.Citation6,Citation25,Citation26
How is the spindle skew regulated to yield an invariant primary l-r asymmetry, and what could be the intrinsic l-r cue? Generally, spindle orientation in C. elegans is controlled by polarity cues provided by PAR proteins and heterotrimeric G-protein-signaling activated by a receptor-independent pathway, whereby both the PAR and the G-protein systems reside in the cortex.Citation27,Citation28 Together with dynein motor complexes, these systems can generate differential pulling forces on astral microtubules ( and right part). It has been demonstrated that a temperature-sensitive mutation in the Gα(i) gene gpa-16 leads to randomization of the ABa/p spindle skew handedness.Citation29 GPA-16 acts in association with RIC-8 and GPR-1/2, required for cortical recruitment and activation of GPA-16, respectively, while both are generally required for pulling force generation.Citation30,Citation31 More recently it has been shown that gpa-16 mutant embryos exhibit markedly diminished and symmetric pulling forces during divisions that would be asymmetric in wild type embryos.Citation32 Taken together, these findings show that proper attachment of the spindle to force generators in the cortex is required for the formation of the l-r axis in C. elegans. However, these findings do not yet fully explain the invariant handedness of the skew.
Further insight into the handedness problem comes from recent work which revealed a dynamic interplay between mitotic spindles and the actomyosin network at sites where microtubule tips contact the cortex.Citation33,Citation34 Interestingly, searching for l-r asymmetry cues in C. elegans that might precede the spindle skew at the six-cell stage, Wood and coworkers found that prior to the initiation of the first cell division, the entire one-cell embryo rotates by 120°, whereby the direction of rotation is invariant relative to the a-p axis, indicating an intrinsic chirality.Citation35 They also found that inhibition of actomyosin or microtubule functions block the skew. Additionally, it is known that (1) the remnant of the ABa-ABp cytokinesis, the midbody, is invariably found on the future right side, and that (2) cytokinesis is intrinsically asymmetric in C. elegans.Citation36 These interesting observations suggest that a chiral structure exists at the nexus of the actin and the microtubule cytoskeleton and that during cytokinesis—by unknown mechanisms—intrinsic chirality is translated into directional morphological l-r asymmetry. Strikingly, in the egg of Xenopus laevis, which is radially symmetric initially, Danilchik and colleagues have demonstrated an invariant chirality of the actin cytoskeleton that depends on the polarized orientation of preexisting F-actin fibers.Citation37 They suggest that this chiral structure is maternally inherited, which implies a similar genetic pathway as found for chirality in snails (see above). Moreover, the integrity of the actomyosin cortex is crucial for proper development of later l-r asymmetries e.g., internal organs handedness. Hence, an early determination of the primary l-r asymmetry that directs all later l-r patterning events is also found in vertebrates. Unfortunately, similar early aspects of l-r axis formation in other vertebrates have not been studied so far.
Until recently, the subsequent l-r patterning steps that immediately follow the initial l-r decision were unknown in C. elegans. Pohl and Bao have recently shown that right after the ABa/ABp spindle skew occurs cells in the C. elegans embryo undergo l-r asymmetric morphogenetic movements.Citation38 They termed this morphogenetic program chiral morphogenesis, as it comprises an invariant collective l-r asymmetric rearrangement that is highly robust (). The cell movements during chiral morphogenesis achieve a stereotypical configuration of blastomeres that provides the blueprint for the bilateral body plan in C. elegans: The l-r asymmetry generated by the spindle skew is reinforced through movements so that a midline is generated. Moreover, during its formation, the midline is uncoupled from the a-p axis, tilted to the right. According to the invariant cell lineage and fates, the cells on the midline generate a bilaterally symmetric structure and contribute equally to the left and right sides of the organism. Furthermore, the ABal blastomere, which does not have a bilateral counterpart in the cell lineage, is segregated to the left side of the tilted midline with no cell mirroring it on the right. Thus, the eight cells become organized into an inherently asymmetric bilateral body plan, with more cells on the left side of the midline. This represents an unusual literal interpretation of the logic of l-r patterning which requires that a midline must be established and, subsequently, the left and right sides must be made different from one another. Pohl and Bao found that in C. elegans the left and right sides are not necessarily qualitatively different but, surprisingly, quantitatively different as they contain different numbers of cells.
Importantly, it has been established through early work on the C. elegans lineage that the sister cells which are symmetric to the tilted midline present at the stage of chiral morphogenesis are equivalent in their cell fate.Citation39–Citation42 This is in contrast to higher organisms where the current paradigm for l-r patterning necessitates the establishment of a restricted expression domain of the TGFβ family factor Nodal to the left side of the midline.Citation43 A Nodal pathway is however not conserved in ecdysozoaCitation44 (e.g., nematodes and flies) and thus it could be argued that C. elegans is simply a special case due to the loss of the pathway. Remarkably, this apparent discrepancy has been resolved recently: Snail embryos, which establish their primary l-r asymmetry through a chiral morphogenesis comparable to C. elegans (see above), n evertheless use the Nodal pathway for downstream l-r patterning.Citation17,Citation45 Importantly, reversion of the primary l-r asymmetry also leads to a reversion in the Nodal expression domain, clearly demonstrating that the primary l-r asymmetry is upstream and independent of Nodal.Citation17 Additionally, the pathways that lead to the restricted expression of Nodal in different species vary substantially.Citation46–Citation48 Thus, it will be interesting to test whether earlier chiromorphogenetic symmetry breaking events could generally act upstream of l-r differential gene expression.
Furthermore, given the fate equivalence of l-r symmetric pairs, Pohl and Bao investigated the cause for l-r asymmetry during chiral morphogenesis.Citation38 As indicated by the reversal experiments (see above), they reasoned that l-r asymmetry might lie in mechanical l-r asymmetries originating from differential regulation of the cytoskeleton. Indeed, although the l-r sisters ABpl and ABpr are equivalent fate-wise, they exhibit strong asymmetries in cellular behaviors: ABpl undergoes dramatic shape changes by forming dorsal and ventral lamellipodia, anterior filopodia and translocates anteroventrally. In contrast, ABpr shows only very transient protrusive activity and does not move. They could furthermore establish that these l-r asymmetries originate from the actomyosin cortex through (1) differential regulation of contractility and (2) l-r differential balance of branched and unbranched actin polymerization mediated by WAVE-Arp2/3 or CYK-1/formin, respectively.
Pohl and Bao also revisited cases of l-r reversal that had been previously reported:Citation22 They cultivated worms at low temperature, which induces reversal of the ABa/ABp spindle skew in a fraction of embryos by impairing an unknown maternal contribution (). Consistent with the idea that chiral morphogenesis represents an integral part of the l-r patterning pathway that maintains the direction of primary l-r asymmetry, they find that a reversed spindle skew is always associated with a mirror-image chiral morphogenesis.Citation38 Thus, the ABa/ABp spindle skew is either upstream of handedness choice or—which seems to align better with their WAVE-Arp2/3 RNAi experiments (see above)—the two are parallel events that respond to a common intrinsic l-r asymmetry cue.
Strikingly, Pohl and Bao also show that the timing of protrusion formation appears to be guided by the division of the neighboring EMS cell:Citation38 The extension of the ventral protrusion of the ABpl cell coincides with the EMS cell forming its contractile ring. Additionally, they present evidence of direct causality by delaying the EMS division through laser irradiation. Interestingly, they also find that EMS cytokinesis has an invariant directionality similar to the ABa/p cytokinesis with the contractile ring also invariantly appearing on the right side. Although they could not establish a direct causality between the directionality of contractile ring closure and handedness of chiral morphogenesis, their findings underscore the importance of cytokinesis in the spatiotemporal coordination of morphogenesis. Thus, these finding unexpectedly point to signaling mechanisms, yet to be fully explored, that mediate communication between neighboring cells.
These novel findings close a gap in the understanding of l-r patterning in C. elegans: Elegant work from the SchnabelCitation49,Citation50 and Priess laboratoriesCitation51 has shown that l-r patterning from the 12-cell stage onwards is achieved by l-r differential Notch inductions (). However, as these inductions rely on direct cell-cell contacts, they necessitate an elaborate l-r asymmetric environment. With the discovery of the formation of an l-r asymmetric body plan through chiral morphogenesis,Citation38 the origin of this particular environment has been revealed. During the first Notch induction, which occurs shortly after chiral morphogenesis is completed, MS induces ABara—to produce pharyngeal cells instead of neuronal tissue when not induced—and ABalp—to produce neurons instead of hypodermis when not induced.Citation50 A second and third l-r Notch induction follow at the 24-cell stage, a major induction originating from ABalap and a minor induction likely from MSap.Citation50 Another induction that induces l-r asymmetry during intestinal organogenesis derives from descendants of MSap.Citation51
Taken together, investigation of l-r patterning in C. elegans has shown that very likely all directional l-r asymmetries that affect the construction of the basic bilateral body plan originate from the primary l-r decision.Citation11 Nonetheless, it could be conceived that directional l-r asymmetries emerging very late in development, e.g., lateralization of the nervous system, might originate independently of initial l-r symmetry breaking through local competition (e.g., stochastic lateral inhibition) or other mechanisms of local symmetry breaking.Citation52 However, an important observation has been made that supports the notion that the primary l-r asymmetry also influences developmentally late neuronal l-r asymmetry: Poole and Hobert demonstrated that both the handedness of the chiral arrangement of blastomeres at the six-cell stage and the subsequent Notch inductions determine functional l-r asymmetry of a bilateral neuron pair, ASEL/ASER.Citation53 The authors propose that these early events leave ‘asymmetry marks’ that are read only much later, when cells have to decide on their final l or r fate. These findings present another interesting starting point for the future discovery of molecular mechanisms that transduce l-r asymmetry information.
Rotational/Torsional Rearrangements during L-R Patterning in Other Organisms
As mentioned above, chiromorphogenesis in the early C. elegans embryo occurs through a rotational rearrangement of cells (). Remarkably, this is by no means an exceptional mechanism as rotational rearrangements have been implicated in l-r symmetry breaking events in other organisms: During Drosophila larval development for example, genitalia undergo a dextral circumrotation leading to a coiling of the spermiduct around the hindgut ( and middle part). A recent study by Suzanne et al. (review in ref. Citation54) shows that the genital plate serves as l-r organizer during this process. Interestingly, similar to C. elegans chiromorphogenesis, the rotational rearrangement of the genital plate relies on forces generated by actomyosin. Two studies by the Noselli and Matsuno labs have shown a requirement for MyoIA/MyoID-type myosins in controlling l-r choice whereby mutants show a reversal of coiling.Citation55,Citation56 Furthermore, a recent study has also shown that non-muscle myosin II is required for the l-r asymmetric development of the embryonic anterior midgut in Drosophila.Citation57 Thus, forces from actomyosin seem to represent a central aspect of l-r patterning in both worms and flies.
In the chick embryo, it has been noted earlierCitation58 that l-r differential gene expression is preceded by an asymmetric morphology of the organizer. Two recent studies have shown that this l-r asymmetry arises through cell rearrangements that create a leftward flow of cells around the node.Citation59,Citation60 Similar to worms and flies, this process depends on actomyosin contractility.
Heart looping in vertebrates represents another well-studied example of a torsional morphogenesis resulting in l-r asymmetry. During looping, the initially straight heart tube undergoes a torsional rightward deformation into a curved tube ( and right part), creating the basic pattern of the adult heart. In the chick embryo, inhibition of actomyosin activity suppressed heart looping, suggesting that also here actomyosin contractility represents the key force-producing system.Citation61
Wnt/Planar Cell Polarity Pathways and L-R Asymmetry
Although rotational rearrangements seem to present a common morphogenetic mechanism to achieve l-r asymmetries, it is important to ask whether commonalities in l-r patterning might also extend to shared signaling pathways. In their recent study, Pohl and Bao demonstrate that Wnt signaling is required to activate l-r asymmetrical cortical dynamics:Citation38 disrupting Wnt signaling by RNAi leads to a general loss of cortical contractility and protrusive activity in the embryo and a complete failure to undergo chiral morphogenesis. Interestingly, the specific type of Wnt signaling does not depend on a transcriptional response. This pathway has been referred to as non-canonical and is known to mediate spindle orientation in the early C. elegans embryo.Citation62,Citation63 It is also required during later embryonic development, where it was shown to activate actomyosin contractility during gastrulation.Citation64 Another major role for Wnt signaling during later development is to maintain a-p polarized tissue architecture.Citation65,Citation66 This role is reminiscent of non-canonical Wnt/planar cell polarity (PCP) pathways in higher organisms, e.g., Drosophila or vertebrates.Citation67–Citation69 Although C. elegans very likely lacks PCP in a truly canonical form, Langenhan and colleagues have recently demonstrated that a homolog of vertebrate latrophilins, lat-1, plays an essential role in the establishment of tissue polarity in C. elegans.Citation70 lat-1 is structurally related to flamingo/CELSR, an essential component of the canonical PCP in other organisms. Moreover, lat-1 genetically interacts with Wnt pathway genes and shows a strong l-r asymmetry during epidermal morphogenesis. Hence, the findings of Langenhan et al. and Pohl and Bao suggest that a PCP-like pathway establishes tissue polarity required for l-r patterning and epithelial morphogenesis.Citation38,Citation70 In the light of the l-r asymmetric body plan, it will be interesting to test whether direct links between early non-canonical Wnt and lat-1/Wnt signaling exist.
Importantly, several recent studies have established that the Wnt/PCP pathway is the major regulator that determines l-r patterning in vertebrates.Citation71–Citation73 In most vertebrates leftward movement of fluid at the ventral node mediates l-r symmetry breaking. The flow is generated by the rotation of posteriorly tilted cilia which unidirectionally transport so-called nodal vesicular parcels resulting in l-r differential gene expression. Until recently, it was unclear how posterior tilting of nodal cilia is accomplished. Notably, the fact that all cilia are localized to the posterior of cells indicates that embryonic a-p polarity is used to break l-r symmetry. The Hamada, Ciruna and Yang laboratories have now demonstrated that the posterior tilt depends on the homologs of Drosophila disheveled, the GTPase Rac1 and the homologs of the Drosophila Strabismus/van Gogh transmembrane protein, Vangl1/Vangl2, all components non-canonical Wnt signaling.Citation71–Citation73 Although theoretically proposed,Citation74 these findings are somewhat unexpected, in that they clearly show that a-p polarization is translated into l-r polarization through a PCP mechanism that acts before unidirectional nodal flow is established. Hence, l-r symmetry breaking in vertebrates occurs much earlier than previously assumed and seems to rely on a signaling mechanism which is also deployed for l-r patterning in the invertebrate C.-elegans.
Taken together, Wnt/PCP signaling constitutes a major developmental pathway to establish l-r symmetry breaking across the invertebrate-vertebrate boundary. It will be interesting to examine whether Wnt/PCP dependent l-r symmetry breaking mechanisms represent a common trigger for rotational tissue rearrangements and whether rotational extracellular flow can be considered a ‘especial case’ for rotational rearrangements. Thus, the recent discoveries in the field of l-r patterning have opened a door to search for commonalities of l-r patterning between invertebrates and vertebrates.
Abbreviations
l-r | = | left-right |
a-p | = | anteroposterior |
C. elegans | = | Caenorhabditis elegans |
PCP | = | planar cell polarity |
PAR | = | embryonic partitioning of cytoplasm |
Figures and Tables
Figure 1 L-R Asymmetry of internal organs in Caenorhabditis elegans, Drosophila melanogaster and Homo sapiens. Selected organs are shown for each organism, endodermal/intestinal organs are shown in red. Lower right of each part: Schematized topology of selected asymmetries for each organism. Arrows indicate tissue movements that lead to coiling of the respective tissue.
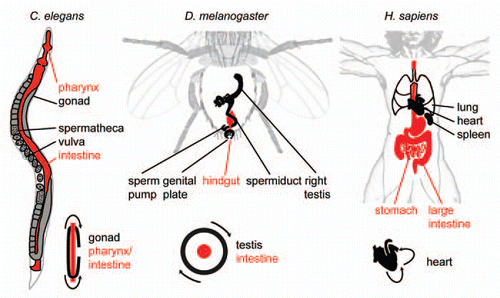
Figure 2 The sequence of l-r symmetry breaking events during C. elegans embryogenesis. Cells actively involved in morphogenetic events or inductions are highlighted in red. (A) Left: Spindle skew during the ABa/p divisions that leads to the first morphological l-r asymmetry. Dorsal views, anterior is to the left, posterior to the right. White lines connect daughter nuclei, arrows indicate the direction of spindle skewing. Right: The force-generating machinery that links the spindle to the cortex. Gα (GPA-16 and GOA-1, see text for details) recruit the dynein complex, a minus-end directed microtubule motor, through interactions with GPR-1/2 and the coiled-coil protein LIN-5. MT, microtubules. (B) Chiral morphogenesis at the 8-cell stage. Left side views, anterior is to the left. Midline (red), a-p axis (black) and l-r axis (blue) are shown. The dashed circle on the right indicates the direction of the rotational rearrangement. (c) Notch inductions that follow chiral morphogenesis on the left side of the embryo. Embryos are oriented as in b. Inducing cells are shown in green, receiving cells in red. For simplicity, lateral cells were obmitted in the case of the Notch induction from MSapxx, as this induction takes place in the center of the embryo.
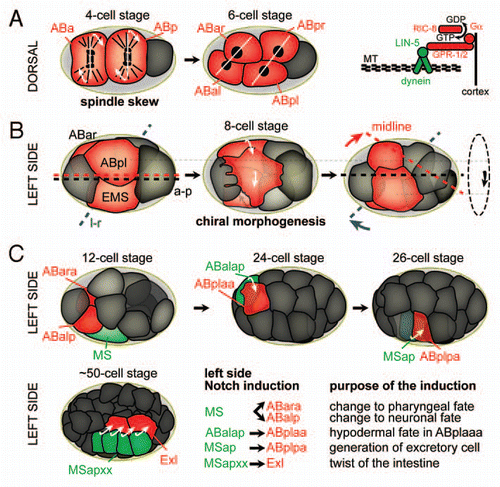
Acknowledgements
The author would like to thank Zhirong Bao for continuous support and Antony Santella for critical reading of the manuscript. The author is supported by a long-term fellowship from the Human Frontier in Science Program Organization.
References
- Brown NA, Wolpert L. The development of handedness in left/right asymmetry. Development 1990; 109:1 - 9
- Levin M, Mercola M. The compulsion of chirality: toward an understanding of left-right asymmetry. Genes Dev 1998; 12:763 - 769
- Wood WB. The left-right polarity puzzle: determining embryonic handedness. PLoS Biol 2005; 3:292
- Cintas P. Chirality of living systems: a helping hand from crystals and oligopeptides. Angew Chem Int Ed Engl 2002; 41:1139 - 1145
- Levin M. Left-right asymmetry in embryonic development: a comprehensive review. Mech Dev 2005; 122:3 - 25
- Okumura T, Utsuno H, Kuroda J, Gittenberger E, Asami T, Matsuno K. The development and evolution of left-right asymmetry in invertebrates: lessons from Drosophila and snails. Dev Dyn 2008; 237:3497 - 3515
- Wood WB. Handed asymmetry in nematodes. Semin Cell Dev Biol 1998; 9:53 - 60
- Asami T, Gittenberger E, Falkner G. Whole-body enantiomorphy and maternal inheritance of chiral reversal in the pond snail Lymnaea stagnalis. J Hered 2008; 99:552 - 557
- Dongen SV. Fluctuating asymmetry and developmental instability in evolutionary biology: past, present and future. J Evol Biol 2006; 19:1727 - 1743
- Mercola M, Levin M. Left-right asymmetry determination in vertebrates. Annu Rev Cell Dev Biol 2001; 17:779 - 805
- Wood WB. Evidence from reversal of handedness in C. elegans embryos for early cell interactions determining cell fates. Nature 1991; 349:536 - 538
- McManus C. Reversed bodies, reversed brains and (some) reversed behaviors: of zebrafish and men. Dev Cell 2005; 8:796 - 797
- Sutherland MJ, Ware SM. Disorders of left-right asymmetry: heterotaxy and situs inversus. Am J Med Genet C Semin Med Genet 2009; 151:307 - 317
- Tamura K, Yonei-Tamura S, Izpisua Belmonte JC. Molecular basis of left-right asymmetry. Dev Growth Differ 1999; 41:645 - 656
- Yost HJ. Coordinating the development of bilateral symmetry and left-right asymmetry. Semin Cell Dev Biol 2009; 20:455
- Crampton H. Reversal of cleavage in a sinistral gastropod. Ann NY Acad Sci 1894; 8:167 - 170
- Kuroda R, Endo B, Abe M, Shimizu M. Chiral blastomere arrangement dictates zygotic left-right asymmetry pathway in snails. Nature 2009; 462:790 - 794
- Palmer AR. Symmetry breaking and the evolution of development. Science 2004; 306:828 - 833
- Speder P, Petzoldt A, Suzanne M, Noselli S. Strategies to establish left/right asymmetry in vertebrates and invertebrates. Curr Opin Genet Dev 2007; 17:351 - 358
- Deppe U, Schierenberg E, Cole T, Krieg C, Schmitt D, Yoder B, von Ehrenstein G. Cell lineages of the embryo of the nematode Caenorhabditis elegans. Proc Natl Acad Sci USA 1978; 75:376 - 380
- Sulston JE, Schierenberg E, White JG, Thomson JN. The embryonic cell lineage of the nematode Caenorhabditis elegans. Dev Biol 1983; 100:64 - 119
- Wood WB, Bergmann D, Florance A. Maternal effect of low temperature on handedness determination in C. elegans embryos. Dev Genet 1996; 19:222 - 230
- Steiner G. Opuscula miscellanea nematologica, IV. Proc Heminthol Soc Wash 1936; 2:74 - 80
- Felix MA, Sternberg PW, De Ley P. Sinistral nematode population. Nature 1996; 381:122
- Sturtevant AH. Inheritance of the direction of coiling in Limnea. Science 1923; 58:269 - 270
- Shibazaki Y, Shimizu M, Kuroda R. Body handedness is directed by genetically determined cytoskeletal dynamics in the early embryo. Curr Biol 2004; 14:1462 - 1467
- Goldstein B, Macara IG. The PAR proteins: fundamental players in animal cell polarization. Dev Cell 2007; 13:609 - 622
- Willard FS, Kimple RJ, Siderovski DP. Return of the GDI: the GoLoco motif in cell division. Annu Rev Biochem 2004; 73:925 - 951
- Bergmann DC, Lee M, Robertson B, Tsou MF, Rose LS, Wood WB. Embryonic handedness choice in C. elegans involves the Galpha protein GPA-16. Development 2003; 130:5731 - 5740
- Afshar K, Willard FS, Colombo K, Johnston CA, McCudden CR, Siderovski DP, Gonczy P. RIC-8 is required for GPR-1/2-dependent Galpha function during asymmetric division of C. elegans embryos. Cell 2004; 119:219 - 230
- Afshar K, Willard FS, Colombo K, Siderovski DP, Gonczy P. Cortical localization of the Galpha protein GPA-16 requires RIC-8 function during C. elegans asymmetric cell division. Development 2005; 132:4449 - 4459
- Johnston CA, Afshar K, Snyder JT, Tall GG, Gonczy P, Siderovski DP, Willard FS. Structural determinants underlying the temperature-sensitive nature of a Galpha mutant in asymmetric cell division of Caenorhabditis elegans. J Biol Chem 2008; 283:21550 - 21558
- Kozlowski C, Srayko M, Nedelec F. Cortical microtubule contacts position the spindle in C. elegans embryos. Cell 2007; 129:499 - 510
- Minc N, Bratman SV, Basu R, Chang F. Establishing new sites of polarization by microtubules. Curr Biol 2009; 19:83 - 94
- Wood WB, Schonegg S. Rotation of 1-cell C. elegans embryos inside the egg shell reveals an early step in establishment of left-right polarity and embryonic handedness. International Worm Meeting 2005; Abstract110; http://www.wormbase.org/db/misc/paper?name=WBPaper00025920;class=Paper
- Maddox AS, Lewellyn L, Desai A, Oegema K. Anillin and the septins promote asymmetric ingression of the cytokinetic furrow. Dev Cell 2007; 12:827 - 835
- Danilchik MV, Brown EE, Riegert K. Intrinsic chiral properties of the Xenopus egg cortex: an early indicator of left-right asymmetry?. Development 2006; 133:4517 - 4526
- Pohl C, Bao Z. Chiral forces organize left-right patterning in C. elegans by uncoupling midline and anteroposterior axis. Dev Cell 2010; 19:402 - 412
- Priess JR, Schnabel H, Schnabel R. The glp-1 locus and cellular interactions in early C. elegans embryos. Cell 1987; 51:601 - 611
- Mello CC, Draper BW, Priess JR. The maternal genes apx-1 and glp-1 and establishment of dorsal-ventral polarity in the early C. elegans embryo. Cell 1994; 77:95 - 106
- Mickey KM, Mello CC, Montgomery MK, Fire A, Priess JR. An inductive interaction in 4-cell stage C. elegans embryos involves APX-1 expression in the signalling cell. Development 1996; 122:1791 - 1798
- Priess JR. Establishment of initial asymmetry in early Caenorhabditis elegans embryos. Curr OpinGenet Dev 1994; 4:563 - 568
- Levin M, Johnson RL, Stern CD, Kuehn M, Tabin C. A molecular pathway determining left-right asymmetry in chick embryogenesis. Cell 1995; 82:803 - 814
- Grande C, Patel NH. Lophotrochozoa get into the game: the nodal pathway and left/right asymmetry in bilateria. Cold Spring Harb Symp Quant Biol 2009; 74:281 - 287
- Grande C, Patel NH. Nodal signalling is involved in left-right asymmetry in snails. Nature 2009; 457:1007 - 1011
- Collignon J, Varlet I, Robertson EJ. Relationship between asymmetric nodal expression and the direction of embryonic turning. Nature 1996; 381:155 - 158
- Lowe LA, Supp DM, Sampath K, Yokoyama T, Wright CV, Potter SS, Overbeek P, Kuehn MR. Conserved left-right asymmetry of nodal expression and alterations in murine situs inversus. Nature 1996; 381:158 - 161
- Meno C, Shimono A, Saijoh Y, Yashiro K, Mochida K, Ohishi S, et al. lefty-1 is required for left-right determination as a regulator of lefty-2 and nodal. Cell 1998; 94:287 - 297
- Hutter H, Schnabel R. glp-1 and inductions establishing embryonic axes in C. elegans. Development 1994; 120:2051 - 2064
- Hutter H, Schnabel R. Establishment of left-right asymmetry in the Caenorhabditis elegans embryo: a multistep process involving a series of inductive events. Development 1995; 121:3417 - 3424
- Hermann GJ, Leung B, Priess JR. Left-right asymmetry in C. elegans intestine organogenesis involves a LIN-12/Notch signaling pathway. Development 2000; 127:3429 - 3440
- Hobert O, Johnston RJ Jr, Chang S. Left-right asymmetry in the nervous system: the Caenorhabditis elegans model. Nature Rev Neurosci 2002; 3:629 - 640
- Poole RJ, Hobert O. Early embryonic programming of neuronal left/right asymmetry in C. elegans. Curr Biol 2006; 16:2279 - 2292
- Suzanne M, Petzoldt AG, Speder P, Coutelis JB, Steller H, Noselli S. Coupling of apoptosis and L/R patterning controls stepwise organ looping. Curr Biol 2010; 20:1773 - 1778
- Hozumi S, Maeda R, Taniguchi K, Kanai M, Shirakabe S, Sasamura T, et al. An unconventional myosin in Drosophila reverses the default handedness in visceral organs. Nature 2006; 440:798 - 802
- Speder P, Adam G, Noselli S. Type ID unconventional myosin controls left-right asymmetry in Drosophila. Nature 2006; 440:803 - 807
- Okumura T, Fujiwara H, Taniguchi K, Kuroda J, Nakazawa N, Nakamura M, et al. Left-right asymmetric morphogenesis of the anterior midgut depends on the activation of a non-muscle myosin II in Drosophila. Dev Biol 2010; 344:693 - 706
- Dathe V, Gamel A, Manner J, Brand-Saberi B, Christ B. Morphological left-right asymmetry of Hensen's node precedes the asymmetric expression of Shh and Fgf8 in the chick embryo. Anatomy and embryology 2002; 205:343 - 354
- Gros J, Feistel K, Viebahn C, Blum M, Tabin CJ. Cell movements at Hensen's node establish left/right asymmetric gene expression in the chick. Science 2009; 324:941 - 944
- Cui C, Little CD, Rongish BJ. Rotation of organizer tissue contributes to left-right asymmetry. Anat Rec 2009; 292:557 - 561
- Nerurkar NL, Ramasubramanian A, Taber LA. Morphogenetic adaptation of the looping embryonic heart to altered mechanical loads. Dev Dyn 2006; 235:1822 - 1829
- Schlesinger A, Shelton CA, Maloof JN, Meneghini M, Bowerman B. Wnt pathway components orient a mitotic spindle in the early Caenorhabditis elegans embryo without requiring gene transcription in the responding cell. Genes Dev 1999; 13:2028 - 2038
- Walston T, Tuskey C, Edgar L, Hawkins N, Ellis G, Bowerman B, et al. Multiple Wnt signaling pathways converge to orient the mitotic spindle in early C. elegans embryos. Dev Cell 2004; 7:831 - 841
- Lee JY, Marston DJ, Walston T, Hardin J, Halberstadt A, Goldstein B. Wnt/Frizzled signaling controls C. elegans gastrulation by activating actomyosin contractility. Curr Biol 2006; 16:1986 - 1997
- Bischoff M, Schnabel R. A posterior centre establishes and maintains polarity of the Caenorhabditis elegans embryo by a Wnt-dependent relay mechanism. PLoS Biol 2006; 4:396
- Cabello J, Neukomm LJ, Gunesdogan U, Burkart K, Charette SJ, Lochnit G, et al. The Wnt pathway controls cell death engulfment, spindle orientation and migration through CED-10/Rac. PLoS Biol 2010; 8:1000297
- Segalen M, Bellaiche Y. Cell division orientation and planar cell polarity pathways. Semin Cell Dev Biol 2009; 20:972 - 977
- Zallen JA. Planar polarity and tissue morphogenesis. Cell 2007; 129:1051 - 1063
- Wang Y, Nathans J. Tissue/planar cell polarity in vertebrates: new insights and new questions. Development 2007; 134:647 - 658
- Langenhan T, Promel S, Mestek L, Esmaeili B, Waller-Evans H, Hennig C, et al. Latrophilin signaling links anterior-posterior tissue polarity and oriented cell divisions in the C. elegans embryo. Dev Cell 2009; 17:494 - 504
- Borovina A, Superina S, Voskas D, Ciruna B. Vangl2 directs the posterior tilting and asymmetric localization of motile primary cilia. Nat Cell Biol 2010; 12:407 - 412
- Hashimoto M, Shinohara K, Wang J, Ikeuchi S, Yoshiba S, Meno C, et al. Planar polarization of node cells determines the rotational axis of node cilia. Nat Cell Biol 2010; 12:170 - 176
- Song H, Hu J, Chen W, Elliott G, Andre P, Gao B, Yang Y. Planar cell polarity breaks bilateral symmetry by controlling ciliary positioning. Nature 2010; 466:378 - 382
- Aw S, Levin M. Is left-right asymmetry a form of planar cell polarity?. Development 2009; 136:355 - 366