Abstract
Our previous studies demonstrated that the key gluconeogenic enzyme fructose-1,6-bisphosphatase is secreted when Saccharomyces cerevisiae are starved of glucose for a prolonged period of time. In this study, we showed that malate dehydrogenase, isocitrate lyase, phosphoenolpyruvate carboxykinase, glyceraldehyde-3-phosphate dehydrogenase, and cyclophilin A are also secreted in glucose-starved cells. Thus, both gluconeogenic and non-gluconeogenic enzymes are secreted via the non-classical pathway.
When Saccharomyces cerevisiae are grown in media containing low glucose, gluconeogenic enzymes are induced.Citation1-Citation9 However, when glucose is added to prolonged-starved cells, these enzymes are inactivated and degraded in the vacuole via the Vid (vacuole import and degradation) pathway.Citation10-Citation24 These enzymes include fructose-1,6-bisphosphatase (FBPase), malate dehydrogenase (MDH2), isocitrate lyase (Icl1p), and phosphoenolpyruvate carboxykinase (Pck1p).Citation10-Citation21,Citation25,Citation26 Recent evidence indicates that the key gluconeogenic enzyme FBPase is secreted when cells were starved of glucose for three days.Citation22-Citation24 Since other gluconeogenic enzymes MDH2, Icl1p, and Pck1p are also degraded in the vacuole via the same Vid pathway,Citation16,Citation25 they should show distribution patterns similar to those of FBPase. As such, they should also be secreted in glucose-starved cells. To test this, we used immuno-TEM techniques to determine the distribution of these enzymes at the ultra-structural level. To confirm our immune-TEM studies, we used an extraction procedure that extracts extracellular proteins from whole cells.Citation22,Citation24 We also determined whether or not non-gluconeogenic enzymes such as glyceraldehyde-3-phosphate dehydrogenase (GAPDH) and cyclophilin A (Cpr1p) were secreted in glucose-starved cells. GAPDH is a cell surface protein,Citation27,Citation28 whereas Cpr1p has been identified in the culture media in Saccharomyces cerevisiae in large-scale secretomic/extraction studies.Citation29,Citation30 Our results indicated that approximately 33.7–76.7% of these enzymes were secreted in glucose-starved cells. Given that these proteins do not contain typical ER signal sequence, they are secreted via the non-classical pathway. Thus, gluconeogenic as well as non-gluconeogenic enzymes utilize the un-conventional pathway to be secreted during prolonged starvation.
FBPase, MDH2, Icl1p, Pck1p, GAPDH, and Cpr1p are secreted in glucose-starved cells
We first used immuno-TEM to examine the distribution of FBPase, MDH2, Icl1p, Pck1p, GAPDH, and Cpr1p at the ultra-structural level. For localization studies, FBPase has previously been shown to be secreted into the extracellular spaceCitation22,Citation24 and was used as a positive control. Under the same experimental conditions, the coatomer subunit Sec28p is distributed mainly in the intracellular fraction as shown previouslyCitation22,Citation24 and was used as a negative control. Because antibodies against Pck1p and Icl1p were not available, we produced a wild-type strain expressing Icl1p tagged with HA and Pck1p-tagged with Myc. We then used HA and Myc antibodies to detect these tagged proteins. For these experiments, wild-type cells co-expressing Icl1p-HA and Pck1p-Myc were grown in YPKG media containing low glucose for three days and processed for immuno-TEM studies. First, we used western blotting to examine whether or not these antibodies recognized proteins at the expected molecular weights in total lysates. As is shown in , polyclonal antibodies against Sec28p, FBPase, MDH2, HA, Myc, GAPDH, and Cpr1p all reacted with a single protein band at the predicted molecular weights.
Figure 1. Immuno-TEM analysis of the distribution of FBPase, MDH2, Icl1p, Pck1p, GAPDH, and Cpr1p in glucose-starved wild-type cells. (A), wild-type cells expressing Icl1p-HA and Pck1p-Myc were lysed and total lysates were examined by western blotting using antibodies directed against Sec28p, FBPase, MDH2, HA, Myc, GAPDH, and Cpr1p. (B), wild-type cells expressing Icl1p-HA and Pck1p-Myc were starved of glucose for 3 d and fixed. Cells were processed and embedded. Thin sections (10 nm) were incubated in the absence or presence of primary antibodies directed against Sec28p, FBPase, MDH2, HA, Myc, GAPDH, and Cpr1p followed by secondary antibodies conjugated with 10 nm gold particles. Enlargements of the periplasm in sections of the whole cells were shown. CW:cell wall. PM:plasma membrane. Bars: 200 nm.
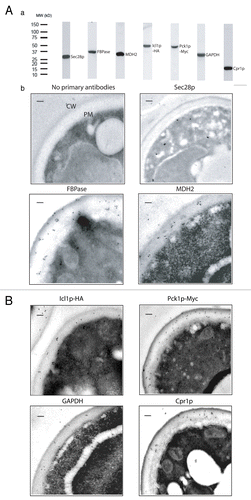
We next examined the distribution of these proteins by incubating thin sections of glucose-starved wild-type cells in the absence or presence of purified primary antibodies followed by secondary antibodies conjugated with 10 nm gold particles (). When thin sections of cells were incubated in the absence of primary antibodies, there were very few gold particles observed between the cell wall and the plasma membrane. In a similar manner, low levels of Sec28p were detected in the extracellular space. In contrast, substantial amounts of the positive control protein FBPase were present in areas between the cell wall and the plasma membrane. Likewise, significant amounts of MDH2, Icl1p-HA, Pck1p-Myc, GAPDH, and Cpr1p were also found in the extracellular space in cells that were grown in low glucose for a prolonged period of time. Quantification of immunogold particles indicated that approximately 76.7% of FBPase, 36.9% of MDH2, 46.9% of Icl1p, 57.6% of Pck1p, 33.7% of GAPDH, and 42.7% of Cpr1p were in the extracellular space in glucose-starved cells ().
Table 1. Quantification of the number of gold particles per cell in the I and E fractions
We next used the extraction procedure to confirm the presence of these proteins in the extracellular fraction.Citation22,Citation24 Wild-type cells expressing Icl1p-HA and Pck1p-Myc were grown in low-glucose media for three days and subjected to the extraction procedure. Following the extraction protocol, proteins were separated into the intracellular and extracellular fractions. The distribution of these proteins in these fractions was then examined by western blotting (). Scw4p is the soluble cell wall proteinCitation31 and was used as a control for proteins that reside in the extracellular fraction. Not surprisingly, the majority of Scw4p was in the extracellular fraction. In contrast, most of the Sec28p was distributed in the intracellular fraction. As expected, significant amounts of FBPase were in the extracellular fraction. In a similar manner, MDH2, Icl1p-HA, Pck1p-Myc, GAPDH, and Cpr1p were all found in the extracellular fraction. Taken together, our results indicate that FBPase, MDH2, Icl1p-HA, Pck1p-Myc, GAPDH, and Cpr1p are secreted when cells were grown in media containing low glucose. Because these enzymes do not contain typical ER signal sequence, they are secreted by the non-conventional pathway.
Figure 2. . FBPase, MDH2, Icl1p, Pck1p, GAPDH, and Cpr1p are in the extracellular fraction in glucose-starved cells. Wild-type cells expressing Scw4p-GFP and wild-type cells co-expressing Icl1p-HA and Pck1p-Myc were grown in YPKG for three days and subjected to the extraction protocol. The distribution of Scw4p-GFP in the intracellular and extracellular fractions was examined by anti-GFP antibodies. The distribution of Sec28p, FBPase, MDH2, Icl1p-HA, Pck1p-Myc, GAPDH, and Cpr1p in the intracellular and extracellular fractions was examined using western blotting.
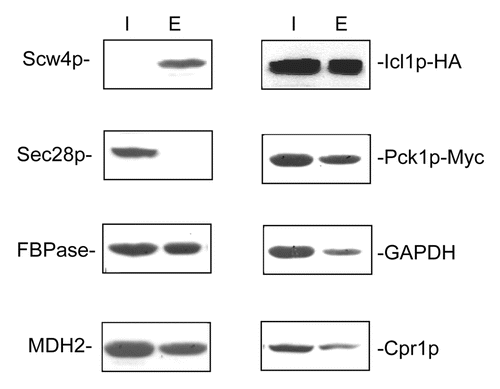
Secretion of signal-less proteins in extracellular vesicles has been observed in different fungi including Cryptococcus neoformans, Candida parapsilosis, Candida albicans, Histoplasma capsulatum, Paracoccidiodes brasiliensis, Sporothrix schenckii, Saccharomyces cerevisiae, and Malassezia sympodialis.Citation30,Citation32-Citation40 Extracellular vesicles isolated from different species showed differences in their sizes. For example, 20–400 nm vesicles were observed in C. neoformans, while 10–350 nm vesicles and 20–200 nm vesicles were found in H. capsulatum and in P. brasiliensis respectively.Citation36-Citation38 Extracellular vesicles of 50- 250 nm were also detected in culture media from S. cerevisiae.Citation36 Interestingly, FBPase, MDH2, Icl1p, Pck1p, GAPDH, and Cpr1p were identified in extracellular vesicles isolated from Histoplasma capsulatum.Citation36 We have shown previously that FBPase is associated with small vesicles (Vid vesicles) in the cytoplasm in S. cerevisiae.Citation26 These vesicles exist in at least two forms. Free vesicles are 30–50 nm in diameter.Citation26 Vid vesicles can also aggregate to form large clusters in the cytoplasm.Citation15 Given that substantial amounts of gluconeogenic enzymes are secreted in glucose-starved cells, these proteins may also be secreted in vesicles. Future experiments will be needed to elucidate the molecular mechanisms responsible for the secretion of signal-less proteins and vesicles across the plasma membrane and the cell wall.
Methods
Cell Culture, Media and Antibodies
Yeast strains used in this study included wild-type (BY4742, MATα his3Δ1 leu2Δ0 lys2Δ0 ura3Δ0), wild-type tagged with Icl1p-HA and Pck1p-Myc (MATα his3Δ1 leu2Δ0 lys2Δ0 ura3Δ0 trp1–1 Icl1p-HA-TRP1 Pck1p-Myc-HIS3), and wild-type expressing Scw4p-GFP (MATα his3Δ1 leu2Δ0 lys2Δ0 ura3Δ0 Scw4p-GFP::HIS3). Cells were first grown in YPKG (1% yeast extract, 2% peptone, 1% potassium acetate and 0.5% glucose) for three days and processed. Antigen affinity purified anti-GAPDH polyclonal antibodies were purchased from Protein Tech Group. Affinity purified anti-HA polyclonal antibodies were purchased from Immunology Consultant Laboratory. Affinity purified polyclonal anti-Myc antibodies were obtained from Santa Cruz Biotechnology. Wild-type cells expressing Scw4p-GFP were purchased from Invitrogen. Anti-GFP polyclonal antibodies were purchased from Abcam.
Immuno-TEM
Immuno-TEM was performed as described.Citation22 After three days in YPKG media, cells were fixed with 3% paraformaldehyde and 0.2% glutaraldehyde overnight at room temperature. Cells were serially dehydrated and embedded in LR White. Embedded samples were sectioned and placed onto Formvar-carbon-coated nickel grids. Grids containing cell sections were incubated on a droplet containing 0.1% BSA/PBS for 30 min. Next, the grids were incubated with or without primary antibody (Sec28p:10 x, FBPase: 50 x, MDH2: 20 x, pHA: 20 x, pMyc: 20 x, GAPDH: 10 x, and Cpr1p: 20 x) diluted in 1% BSA/PBS for 2 h. Grids were then washed and incubated in 50 x dilution of goat anti-rabbit IgG conjugated with 10 nm immunogold particles (Ted Pella). After washing, grids were stained with 2% uranyl acetate for 1 min and viewed on a JEOL JEM-1400 electron microscope with an Orius SC1000 CCD camera.
Extraction and Western Blotting
Extraction was performed as described.Citation22,Citation24 Cells were grown in 2 ml YPKG for three days and harvested. Cells (OD600 = 10/ml) were incubated with 100 μl of extraction buffer containing 0.1 M Tris, pH 9.4 and 10 mM β-mercaptoethanol in a 37°c shaker for 15 min. Following incubation, cells were pelleted at 3,000 x g for 5 min. The supernatant fraction was precipitated with 15% TCA, washed and resuspended in SDS-PAGE buffer. The distribution of proteins in the extracellular and intracellular fractions was examined using polyclonal antibodies directed against Sec28p, FBPase, MDH2, HA, Myc, GAPDH, and Cpr1p followed by peroxidase-conjugated donkey anti-rabbit IgG diluted 1:10,000 (GE Healthcare). The distribution of Scw4p-GFP was examined using anti-GFP antibodies. Bands were developed using Western Lighting Plus ECL (Perkin Elmer).
Abbreviations: | ||
fructose-1,6-bisphosphatase | = | FBPase |
malate dehydrogenase | = | MDH2 |
isocitrate lyase | = | Icl1p |
phosphoenolpyruvate carboxykinase | = | Pck1p |
glyceraldehyde-3-phosphate dehydrogenase | = | GAPDH |
cyclophilin A | = | Cpr1p |
vacuole import and degradation | = | Vid |
Disclosure of Potential Conflicts of Interest
No potential conflicts of interest were disclosed.
Acknowledgments
Immuno-TEM was performed using the EM Core Facility at Penn State College of Medicine. This work was supported by Penn State Bridge Fund to Hui-Ling Chiang.
References
- Giardina BJ, Stanley BA, Chiang HL. Comparative proteomic analysis of transition of saccharomyces cerevisiae from glucose-deficient medium to glucose-rich medium. Proteome Sci 2012; 10:40; http://dx.doi.org/10.1186/1477-5956-10-40; PMID: 22691627
- Carlson M. Regulation of glucose utilization in yeast. Curr Opin Genet Dev 1998; 8:560 - 4; http://dx.doi.org/10.1016/S0959-437X(98)80011-7; PMID: 9794821
- Carlson M. Glucose repression in yeast. Curr Opin Microbiol 1999; 2:202 - 7; http://dx.doi.org/10.1016/S1369-5274(99)80035-6; PMID: 10322167
- Gancedo C. Inactivation of fructose-1,6-diphosphatase by glucose in yeast. J Bacteriol 1971; 107:401 - 5; PMID: 4329729
- Gancedo JM. Yeast carbon catabolite repression. Microbiol Mol Biol Rev 1998; 62:334 - 61; PMID: 9618445
- Gancedo JM. The early steps of glucose signalling in yeast. FEMS Microbiol Rev 2008; 32:673 - 704; http://dx.doi.org/10.1111/j.1574-6976.2008.00117.x; PMID: 18559076
- Holzer H. Proteolytic catabolite inactivation in Saccharomyces cerevisiae. Revis Biol Celular 1989; 21:305 - 19; PMID: 2561496
- Schork SM, Thumm M, Wolf DH. Catabolite inactivation of fructose-1,6-bisphosphatase of Saccharomyces cerevisiae. Degradation occurs via the ubiquitin pathway. J Biol Chem 1995; 270:26446 - 50; http://dx.doi.org/10.1074/jbc.270.44.26446; PMID: 7592860
- Schüle T, Rose M, Entian KD, Thumm M, Wolf DH. Ubc8p functions in catabolite degradation of fructose-1, 6-bisphosphatase in yeast. EMBO J 2000; 19:2161 - 7; http://dx.doi.org/10.1093/emboj/19.10.2161; PMID: 10811607
- Alibhoy AA, Chiang HL. The TOR complex 1 is required for the interaction of multiple cargo proteins selected for the vacuole import and degradation pathway. Commun Integr Biol 2010; 3:594 - 6; http://dx.doi.org/10.4161/cib.3.6.13241; PMID: 21331250
- Alibhoy AA, Chiang HL. Vacuole import and degradation pathway: Insights into a specialized autophagy pathway. World J Biol Chem 2011; 2:239 - 45; http://dx.doi.org/10.4331/wjbc.v2.i11.239; PMID: 22125667
- Alibhoy AA, Giardina BJ, Dunton DD, Chiang HL. Vid30 is required for the association of Vid vesicles and actin patches in the vacuole import and degradation pathway. Autophagy 2012; 8:29 - 46; http://dx.doi.org/10.4161/auto.8.1.18104; PMID: 22082961
- Brown CR, Chiang HL. A selective autophagy pathway that degrades gluconeogenic enzymes during catabolite inactivation. Commun Integr Biol 2009; 2:177 - 83; PMID: 19513275
- Brown CR, Cui DY, Hung GG, Chiang HL. Cyclophilin A mediates Vid22p function in the import of fructose-1,6-bisphosphatase into Vid vesicles. J Biol Chem 2001; 276:48017 - 26; PMID: 11641409
- Brown CR, Dunton D, Chiang HL. The vacuole import and degradation pathway utilizes early steps of endocytosis and actin polymerization to deliver cargo proteins to the vacuole for degradation. J Biol Chem 2010; 285:1516 - 28; http://dx.doi.org/10.1074/jbc.M109.028241; PMID: 19892709
- Brown CR, Hung GC, Dunton D, Chiang HL. The TOR complex 1 is distributed in endosomes and in retrograde vesicles that form from the vacuole membrane and plays an important role in the vacuole import and degradation pathway. J Biol Chem 2010; 285:23359 - 70; http://dx.doi.org/10.1074/jbc.M109.075143; PMID: 20457600
- Brown CR, McCann JA, Chiang HL. The heat shock protein Ssa2p is required for import of fructose-1, 6-bisphosphatase into Vid vesicles. J Cell Biol 2000; 150:65 - 76; http://dx.doi.org/10.1083/jcb.150.1.65; PMID: 10893257
- Brown CR, McCann JA, Hung GG, Elco CP, Chiang HL. Vid22p, a novel plasma membrane protein, is required for the fructose-1,6-bisphosphatase degradation pathway. J Cell Sci 2002; 115:655 - 66; PMID: 11861771
- Brown CR, Wolfe AB, Cui D, Chiang HL. The vacuolar import and degradation pathway merges with the endocytic pathway to deliver fructose-1,6-bisphosphatase to the vacuole for degradation. J Biol Chem 2008; 283:26116 - 27; http://dx.doi.org/10.1074/jbc.M709922200; PMID: 18660504
- Chiang HL, Schekman R. Regulated import and degradation of a cytosolic protein in the yeast vacuole. Nature 1991; 350:313 - 8; http://dx.doi.org/10.1038/350313a0; PMID: 1848921
- Chiang HL, Schekman R, Hamamoto S. Selective uptake of cytosolic, peroxisomal, and plasma membrane proteins into the yeast lysosome for degradation. J Biol Chem 1996; 271:9934 - 41; http://dx.doi.org/10.1074/jbc.271.17.9934; PMID: 8626630
- Alibhoy AA, Giardina BJ, Dunton DD, Chiang HL. Vps34p is required for the decline of extracellular fructose-1,6-bisphosphatase in the vacuole import and degradation pathway. J Biol Chem 2012; 287:33080 - 93; http://dx.doi.org/10.1074/jbc.M112.360412; PMID: 22833678
- Giardina BJ, Chiang HL. The key gluconeogenic enzyme fructose-1,6-bisphosphatase is secreted during prolonged glucose starvation and is internalized following glucose re-feeding via the non-classical secretory and internalizing pathways in Saccharomyces cerevisiae. Plant Signal Behav 2013; 8:8; http://dx.doi.org/10.4161/psb.24936; PMID: 23673352
- Giardina BJ, Dunton D, Chiang HL. Vid28 protein is required for the association of vacuole import and degradation (Vid) vesicles with actin patches and the retention of Vid vesicle proteins in the intracellular fraction. J Biol Chem 2013; 288:11636 - 48; http://dx.doi.org/10.1074/jbc.M112.419895; PMID: 23393132
- Hung GC, Brown CR, Wolfe AB, Liu J, Chiang HL. Degradation of the gluconeogenic enzymes fructose-1,6-bisphosphatase and malate dehydrogenase is mediated by distinct proteolytic pathways and signaling events. J Biol Chem 2004; 279:49138 - 50; http://dx.doi.org/10.1074/jbc.M404544200; PMID: 15358789
- Huang PH, Chiang HL. Identification of novel vesicles in the cytosol to vacuole protein degradation pathway. J Cell Biol 1997; 136:803 - 10; http://dx.doi.org/10.1083/jcb.136.4.803; PMID: 9049246
- Delgado ML, Gil ML, Gozalbo D. Starvation and temperature upshift cause an increase in the enzymatically active cell wall-associated glyceraldehyde-3-phosphate dehydrogenase protein in yeast. FEMS Yeast Res 2003; 4:297 - 303; http://dx.doi.org/10.1016/S1567-1356(03)00159-4; PMID: 14654434
- Delgado ML, O’Connor JE, Azorín I, Renau-Piqueras J, Gil ML, Gozalbo D. The glyceraldehyde-3-phosphate dehydrogenase polypeptides encoded by the Saccharomyces cerevisiae TDH1, TDH2 and TDH3 genes are also cell wall proteins. Microbiology 2001; 147:411 - 7; PMID: 11158358
- Rowe JD, Harbertson JF, Osborne JP, Freitag M, Lim J, Bakalinsky AT. Systematic identification of yeast proteins extracted into model wine during aging on the yeast lees. J Agric Food Chem 2010; 58:2337 - 46; http://dx.doi.org/10.1021/jf903660a; PMID: 20108898
- Oliveira DL, Nakayasu ES, Joffe LS, Guimarães AJ, Sobreira TJ, Nosanchuk JD, Cordero RJ, Frases S, Casadevall A, Almeida IC, et al. Characterization of yeast extracellular vesicles: evidence for the participation of different pathways of cellular traffic in vesicle biogenesis. PLoS One 2010; 5:e11113; http://dx.doi.org/10.1371/journal.pone.0011113; PMID: 20559436
- Cappellaro C, Mrsa V, Tanner W. New potential cell wall glucanases of Saccharomyces cerevisiae and their involvement in mating. J Bacteriol 1998; 180:5030 - 7; PMID: 9748433
- Rodrigues ML, Franzen AJ, Nimrichter L, Miranda K. Vesicular mechanisms of traffic of fungal molecules to the extracellular space. Curr Opin Microbiol 2013; 16:414 - 20; http://dx.doi.org/10.1016/j.mib.2013.04.002; PMID: 23628115
- Rodrigues ML, Nakayasu ES, Almeida IC, Nimrichter L. The impact of proteomics on the understanding of functions and biogenesis of fungal extracellular vesicles. J Proteomics 2013; http://dx.doi.org/10.1016/j.jprot.2013.04.001; PMID: 23583696
- Rodrigues ML, Nakayasu ES, Oliveira DL, Nimrichter L, Nosanchuk JD, Almeida IC, Casadevall A. Extracellular vesicles produced by Cryptococcus neoformans contain protein components associated with virulence. Eukaryot Cell 2008; 7:58 - 67; http://dx.doi.org/10.1128/EC.00370-07; PMID: 18039940
- Rodrigues ML, Nimrichter L, Oliveira DL, Frases S, Miranda K, Zaragoza O, Alvarez M, Nakouzi A, Feldmesser M, Casadevall A. Vesicular polysaccharide export in Cryptococcus neoformans is a eukaryotic solution to the problem of fungal trans-cell wall transport. Eukaryot Cell 2007; 6:48 - 59; http://dx.doi.org/10.1128/EC.00318-06; PMID: 17114598
- Albuquerque PC, Nakayasu ES, Rodrigues ML, Frases S, Casadevall A, Zancope-Oliveira RM, Almeida IC, Nosanchuk JD. Vesicular transport in Histoplasma capsulatum: an effective mechanism for trans-cell wall transfer of proteins and lipids in ascomycetes. Cell Microbiol 2008; 10:1695 - 710; http://dx.doi.org/10.1111/j.1462-5822.2008.01160.x; PMID: 18419773
- Vallejo MC, Matsuo AL, Ganiko L, Medeiros LC, Miranda K, Silva LS, Freymüller-Haapalainen E, Sinigaglia-Coimbra R, Almeida IC, Puccia R. The pathogenic fungus Paracoccidioides brasiliensis exports extracellular vesicles containing highly immunogenic α-Galactosyl epitopes. Eukaryot Cell 2011; 10:343 - 51; http://dx.doi.org/10.1128/EC.00227-10; PMID: 21216942
- Vallejo MC, Nakayasu ES, Longo LV, Ganiko L, Lopes FG, Matsuo AL, Almeida IC, Puccia R. Lipidomic analysis of extracellular vesicles from the pathogenic phase of Paracoccidioides brasiliensis. PLoS One 2012; 7:e39463; http://dx.doi.org/10.1371/journal.pone.0039463; PMID: 22745761
- Oliveira DL, Nakayasu ES, Joffe LS, Guimarães AJ, Sobreira TJ, Nosanchuk JD, Cordero RJ, Frases S, Casadevall A, Almeida IC, et al. Biogenesis of extracellular vesicles in yeast: Many questions with few answers. Commun Integr Biol 2010; 3:533 - 5; http://dx.doi.org/10.4161/cib.3.6.12756; PMID: 21331232
- Gehrmann U, Qazi KR, Johansson C, Hultenby K, Karlsson M, Lundeberg L, Gabrielsson S, Scheynius A. Nanovesicles from Malassezia sympodialis and host exosomes induce cytokine responses--novel mechanisms for host-microbe interactions in atopic eczema. PLoS One 2011; 6:e21480; http://dx.doi.org/10.1371/journal.pone.0021480; PMID: 21799736