Abstract
Lung infection by Gram-negative bacteria is a major cause of morbidity and mortality in humans. Lipopolysaccharide (LPS), located in the outer membrane of the Gram-negative bacterial cell wall, is a highly potent stimulus of immune and structural cells via the TLR4/MD2 complex whose function is sequentially regulated by defined subsets of adaptor proteins. Regulatory mechanisms of lung-specific defense pathways point at the crucial role of resident alveolar macrophages, alveolar epithelial cells, the TLR4 receptor pathway, and lung surfactant in shaping the innate immune response to Gram-negative bacteria and LPS. During the past decade intracellular spatiotemporal localization of TLR4 emerged as a key feature of TLR4 function. Here, we briefly review lung cell type- and compartment-specific mechanisms of LPS-induced TLR4 regulation with a focus on primary resident hematopoietic and structural cells as well as modifying microenvironmental factors involved.
Introduction
Due to the unique condition of being constantly exposed to air that contains pathogens, allergens, and pollutants, the lung has developed a highly specific local microenvironment where the combined actions of the epithelial barrier, innate defense molecules, and responses of both, the epithelium and professional phagocytes, help to maintain biophysical and immune homeostasis. Key cells mediating lung innate immune responses in the proximal respiratory tract include ciliated cells, goblet cells, Clara cells, and submucosal glands that together transport particles trapped in secreted mucus from more distally located airways toward proximal, a defense meachnisms known as mucociliary escalator. At the alveolar site pulmonary innate immunity is ensured by airway epithelial cells, alveolar epithelial cells (AEC) type I and II and alveolar macrophages that cofunction with surfactant-associated proteins belonging to the C-type lectin superfamily as well as antimicrobial peptides. Type II AEC synthesize, secrete and reuptake pulmonary surfactant, a lipoprotein complex that reduces surface tension at the air-liquid interface of the lung, thereby allowing normal breathing, and protects the lung from continuous environmental exposures to pathogens and allergens.Citation1-Citation4 Surfactant is composed of 90% phopholipids (dipalmitoylphosphatidylcholine) and 10% of proteins (SP) SP-A, SP-B, SP-C, and SP-D.Citation2 The collectins SP-A, the most abundant one in the lung, and SP-D are both soluble pattern recognition receptors and sensing molecules not based on pattern recognition with important functions on lung immune homeostasis in vivo.Citation1,Citation4 The small hydrophobic proteins SP-B and SP-C are essential for the functional structure of the surfactant lipid film at the air-liquid interphase.Citation3 However, increasing evidence indicates that all surfactant proteins and distinct surfactant lipids regulate innate immune responses of the lung,Citation5 partly by interacting with alveolar macrophages. Under physiological conditions, resident alveolar macrophages account for approximately 95% of airspace leukocytes, constituting the sentinel phagocytic cell of the innate immune system in the lung. The natural microenvironment of alveolar macrophages is pulmonary surfactant whose lipid and protein elements modulate their functional phenotype in humans, rats and mice in vivo.Citation1,Citation4,Citation6
Cell surface and endosomal TLRs constitute one of the major cell receptors involved in innate immunity of the lung mediating immediate immune responses against microbial pathogens. TLR4, one of the most extensively studied TLR, is a type I transmembrane protein with an extracellular domain of leucine-rich repeats that provides, dependent on MD2, the recognition of LPS, the invariant virulence factor of Gram-negative bacteria. In recent years some unique regulatory mechanisms of defense pathways intrinsic to the lung have been identified which point at the critical role of resident alveolar macrophages, alveolar epithelial cells, the canonical TLR4 receptor pathway and pulmonary surfactant in initiating and modulating initial immune responses to Gram-negative bacteria and LPS. These normal lung defense mechanisms ensure that most host-microbe interactions do not lead to persistent pathological consequences. However, dependent on pathogen exposure time, load, virulence as well as age- and immune status-related specific factors of individuals being at risk, pneumonia may develop. Pneumonia caused by Gram-negative bacteria is a major cause of morbidity and mortality in humans with an increasing prevalence of community-acquired and early-onset ventilator-associated pneumonia.Citation7
Although lung cell-specific regulatory mechanisms of TLR4 signaling are largely unknown, they are expected to maintain defense homeostasis of the lung. LPS-induced cell responses are tightly regulated via distinct pathways including subcellular TLR4 localization and thus ligand sensing, but the role of TLR4 localization specific for the lung is only beginning to be experimentally addressed. In the lung, TLR4 and TLR4 adaptors are expressed by all immune and structural cell types. The purpose of this article is to review lung cell- and context-specific mechanisms of TLR4 regulation with a focus on primary resident hematopoietic and structural cells, represented by alveolar macrophages and alveolar epithelial cells, respectively.
TLR4 signaling in gram-negative respiratory infections
The identification of TLR4 as signaling receptor for LPS is based on studies showing that LPS-resistant C3H/HeJ and C57BL/10ScCr mice have a mutation in the Tlr4 gene and was confirmed by data showing that cells isolated from TLR4-deficient knockout mice are hyporesponsive to LPS.Citation8,Citation9 Subsequent in vivo studies in TLR4-deficient mice revealed impaired survival associated with higher bacterial loads, reduced activation of gene expression and diminished production of inflammatory mediators indicating that TLR4 signaling is required to induce a protective pulmonary immune response against common Gram-negative respiratory pathogens, including Klebsiella pneumoniae,Citation10-Citation12 Pseudomonas aeruginosa,Citation13 and Hemophilus influenza.Citation14 Of note, mice lacking the adaptor protein MyD88, TIRAP, or TRIF also showed strongly reduced clearance of bacteria from the lung due to impaired induction of early immune responses with a much more remarkable phenotype in MyD88-deficient mice infected with K. pneumoniae,Citation15,Citation16 P. aeruginosa,Citation17,Citation18 or Hemophilus influenzaCitation19 indicating that MyD88-dependent signaling is especially important for mounting the initial host response to Gram-negative infections. Additionally, TIRAP has been shown to be critically involved in early lung immune responses against E. coli LPS and viable E. coli.Citation20 By adoptive transfer of bone marrow cells from TLR4-deficient mice to wild type mice, it was demonstrated that TLR4 signaling in cells of hematopoietic origin, like lymphocytes and/or macrophages plays an important role in early host defense against K. pneumoniae.Citation21 The combined studies clearly reveal the complexity and redundancy of the TLR4 signaling pathway in inducing initial pulmonary immune responses toward Gram-negative pathogens and suggest a primary role of hematopoietic cells.
Cell compartment-specific regulation of TLR4 signaling
First evidence for the mechanistic link between LPS recognition and intracellular transport of LPS from the plasma membrane to intracellular sites of accumulation as well as the dependence of this traffic on the LPSd gene was demonstrated by Wright and colleagues.Citation22-Citation24 Mechanisms of how TLR4 signaling is integrated into the cellular infrastructure have been uncovered in cell line-based studies, murine bone marrow derived macrophages and dendritic cells, murine splenic B-cells and dendritic cells, murine embryonic fibroblasts, as well as human monocytes, neutrophils, and peripheral blood mononuclear cells. In the past decade it has become increasingly evident that subcellular localization of TLR4 and TLR4 adaptors is an important regulatory mode of TLR4 signaling.Citation25,Citation26
Under resting conditions, TLR4 cycles between the Golgi and at the plasma membrane and translocates to the cell surface upon LPS exposure.Citation27 Trafficking of TLR4 from the Golgi to the plasma membrane is regulated by the small TLR4 associated glycoprotein MD2.Citation28 At the plasma membrane, TLR4 and its co-receptor CD14 are recruited to phosphatidylinositol 4,5-bisphosphate (PIP2)-rich microdomains.Citation29,Citation30 Within these PIP2-rich microdomains TLR4 oligomerizes and engages MyD88 that is recruited by the sorting adaptor TIRAP.Citation31 In addition to the MyD88-dependent pathway that leads to early NF-κB activation and production of pro-inflammatory cytokines, TLR4 subsequently activates a second pathway via interaction with TRAM, the sorting adaptor for TRIF. This second pathway is initiated upon receptor endocytosis and, from the endosomal compartment, triggers nuclear translocation of IRF3 and delayed activation of NF-κB and AP-1.Citation32,Citation33 Both clathrin-mediated endocytosis as well as caveolae and lipid raft-mediated endocytosis of TLR4 are involved in TLR4 signalingCitation34 and are required for TRIF-dependent interferon expression.Citation32 From early endosomes, TLR4 is sorted and targeted to a trans-Golgi network pathwayCitation35 and/or to a lysosomal-degradation pathway,Citation34 required for resolution of the inflammatory response (). Thus, LPS responsiveness is fine-tuned by the levels of TLR4 present on the cell surface membrane which is in turn determined by the amount of TLR4 trafficking from the Golgi to the plasma membrane and the amount of TLR4 internalized into endosomes.Citation26 Minimal perturbation of any of these steps causes an abnormal inflammatory response. Regulation of the fine-tuning of TLR4-induced signaling by endocytosis and the factors that restrict these processes are only starting to be elucidated. A recent study described an LPS-induced endocytic process that is CD14-dependent but TLR4 signaling independent and involves the tyrosine kinase Syk and its downstream effector PLCγ2.Citation36 In that study, cell type-specific responses to LPS are conferred by cell type-specific coexpression of TLR4 and CD14.Citation36 The p110δ isoform of phosphatidylinositol-3-kinase has been ascribed to have an important regulatory function in TLR4 endocytosis by promoting the transfer of TLR4 to endosomes thereby controlling the balance between TIRAP-MyD88-dependent pro-inflammatory and TRIF-dependent anti-inflammatory cytokine expression.Citation37 Besides spatiotemporal localization of TLR4 itself, signaling is also dictated by subcellular localization of the sorting adaptors TIRAP and TRAM. An example for how adaptor localization can regulate TLR4 signaling was provided by recent data showing that TAG, a splice variant of TRAM, is directed to late endosomes, unlike TRAM that localizes to early endosomes, and thus, in association with a recently identified protein called TMED7, negatively regulates TLR4-induced IFN production.Citation38,Citation39 These seminal cell biological studies have revolutionized the understanding of compartmentalized TLR4 signaling and will pave the way for increasing the knowledge on regulation of TLR4 signaling in primary immune cells and identifying cell- and context-specific factors involved.
Figure 1. Simplified overview of TLR4 signaling in primary lung cells. Within the alveolus LPS is recognized by the TLR4 receptor complex that is constitutively expressed by primary alveolar macrophages and epithelial cells. Upon receptor binding TLR4 sequentially activates the MyD88-dependent pathway from the plasma membrane resulting in NF-kB activation and the TRAM/TRIF-dependent pathway from the endosomal compartment leading to activation of IRF3. Cell type-specific regulation of LPS-induced TLR4 signaling in the lung is modified by microenvironmental factors, including the pulmonary surfactant lipids and proteins that partly regulate signaling through modulation of subcellular TLR4 localization. AECI, type I alveolar epithelial cells; AECII, type II alveolar epithelial cells; IFN, interferon; IL, interleukin; IRAK, interleukin-1 receptor-associated kinase; IRF, interferon regulatory factor; LPS, lipopolysaccharide; MyD88, myeloid differentiation primary response gene 88; NF-κB, nuclear factor κB; SP-A, surfactant protein A; SP-D, surfactant protein D; TIRAP, toll-interleukin 1 receptor (TIR) domain containing adaptor protein; TLR4, Toll-like receptor 4; TNF-α, tumor necrosis factor-α; TRAM, TRIF-related adaptor molecule; TRIF, toll/Il-1R-domain-containing adaptor protein inducing interferon-β.
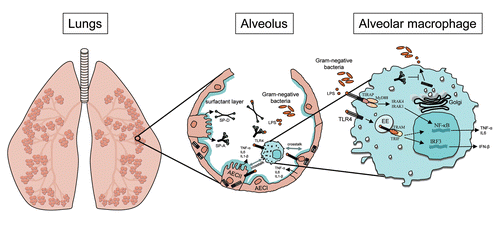
Rab-regulated TLR4 trafficking
Only recently, Rab (Ras related in brain) GTPases, known as pivotal regulators of phagocytic, endocytic, and exocytic trafficking in eukaryotic cellsCitation40 have been described to regulate receptor rafficking and thus signaling. Rab GTPases involved in regulating subcellular TLR4 localization are Rab7b, Rab10, and Rab11. Rab7b controls trafficking between endosomes and the TGNCitation41 and has been suggested to negatively regulate TLR4 signaling by promoting TLR4 lysosomal degradation in RAW264.7 cells.Citation42 Rab10 is essential for optimal macrophage activation upon LPS stimulation by promoting continuous replenishment of TLR4 to the plasma membrane of RAW264.7 cells and, when overexpressed in macrophages, enhances LPS-induced acute lung injury in mice.Citation43 Rab11a is essential for the trafficking of TLR4 and TRAM to Escherichia coli-enriched phagosomes in human monocytes and thereby controls IRF3 activation from this compartment.Citation44 The regulation of Rab-mediated TLR4 trafficking in primary lung-specific cells is only beginning to be understood.
Emerging evidence indicates that lung-specific microenvironmental factors such as surfactant play a critical role in regulating subcellular membrane trafficking. In 2006 Ferguson et al. were the first demonstrating that pulmonary C-type lectins can modulate Rab-regulated intracellular membrane trafficking. In that study, coating of Mycobacterium tuberculosis with SP-D modified phagosome-lysosome fusion in human monocyte-derived macrophages.Citation45 Furthermore, both SP-A and SP-D significantly increase the number of Legionella pneumophilia co-localized with lysosome-associated membrane protein-1in THP-1 cells.Citation46 Using primary rat alveolar macrophages, we could show that SP-A specifically and transiently modulates endocytic/phagocytic membrane trafficking via regulation of Rab GTPases thereby functionally enhancing the lysosomal delivery of GFP-labeled Escherichia coli in these cells.Citation47 Together, these studies provide evidence for lung-specific mechanisms in modulating Rab-regulated receptor trafficking.
Constitutive and LPS-modulated TLR4 gene and protein expression in primary alveolar macrophages
TLR4 signaling outcomes are partly generated through differences in TLR4 expression patterns by distinct cells. LPS-induced cytokine release by primary murine alveolar macrophages depends on TLR4, MyD88, and TRIF.Citation48 Constitutively expressed TLR4 mRNA and protein by primary murine and rat alveolar macrophages are significantly and transiently regulated by LPS treatment in vitro and in vivo intranasal, inhalative, or intratracheal challenge depending on LPS dose and exposure time.Citation49-Citation53 Using chimeric mice separately expressing TLR4 on hematopoietic or structural lung cells, Hollingsworth et al. demonstrated a critical role of TLR4 expression on specifically alveolar macrophages for the biological response to inhaled LPS.Citation54 Since the expression of TLR4 on structural lung cells is essential for neutrophil recruitment after systemic LPS exposure, the authors suggested the existence of lung-specific mechanisms for inhaled but not systemic exposure to LPS.Citation54 Furthermore, the inflammatory trafficking of monocytes into the alveolar space is associated with a significantly increased expression of TLR4 and CD14 mRNA supporting the assumption that freshly recruited alveolar phagocytes substantially contribute to acute immune responses of the lung.Citation55 By comparing the constitutive and ligand-induced expression of TLR4 on human alveolar macrophages and autologous blood monocytes, it was demonstrated that the constitutive cell surface expression on alveolar macrophages is either significantly lower than on monocytesCitation56 or equally low on both cells types.Citation57 Comparably, the constitutive TLR4 mRNA expression is lower in alveolar macrophages than in autologous monocytes.Citation57 Taken together, the TLR4 expression profile of autologous human alveolar macrophages and monocytes is not identical and may thus provide specificity of immune responses to TLR4 ligation by LPS both in the lung and systemically. Exposure to LPS enhances TLR4 surface expression already after 10 min and TLR4 mRNA after 1 h on both cell types with a subsequent decrease of TLR4 mRNA in both cell types after 24 h.Citation57 Similarly, the low constitutive TLR4 cell surface expression on human alveolar macrophages is significantly increased after LPS treatment at the same concentration with staining of TLR4 being most distinct at the cell surface after 30 min and located more intracellularly after 3 h as shown by confocal microscopy.Citation58 The combined data demonstrate that constitutive TLR4 expression in freshly isolated primary human alveolar macrophages is low, but quickly and transiently upregulated at the gene and protein level by LPS in vitro. Inhalation of LPS by healthy humans decreases TLR4 mRNA expression in alveolar macrophages after 6 h,Citation59 whereas lung subsegmental instillation of LPS in healthy humans does not influence the cell surface expression of TLR4 or CD14 on alveolar macrophages recovered after the same time,Citation60 suggesting that LPS application procedures in humans differentially affect TLR4 abundancy in alveolar macrophages.
Constitutive and LPS-modulated TLR4 gene and protein expression in human type I and type II alveolar epithelial cells (AECI and AECII)
Together with alveolar macrophages, alveolar epithelial cells are the first to encounter LPS. Recently, distinct roles of AECI and AECII in immunomodulation begin to emerge and additionally point to positive or negative impacts of both alveolar macrophages and surfactant on the functional status of AECs. Primary rat AECI, which have been shown to express TLR4,Citation61 produce more pro-inflammatory cytokines upon LPS treatment than AECII and, equally important, AECI-released cytokines are significantly enhanced by co-cultured alveolar macrophages and decreased by co-incubation with surfactant.Citation62 Confirming and extending previous data,Citation63,Citation64 Guillot et al. demonstrated the constitutive expression of TLR4 mRNA and protein in human alveolar (A549) and bronchial (BRAS-2B, CFT-2, and NT-1) epithelial cell lines as well as MD2 mRNA expression in A549 and BEAS-2B cellsCitation65 and provided first evidence for an intracellular compartmentalization of TLR4 in lung epithelial cells. LPS treatment does not alter TLR4 mRNA or protein expression or localization in BEAS-2B cells though it induces a strong cytokine release.Citation65 The constitutive expression of TLR4 mRNA and protein by primary human AECII is significantly upregulated by LPS treatment accompanied by an enhanced cytokine release.Citation66 Likewise, LPS induces a marked increase in TLR4 protein expression in primary human AECII after 30 min which increases further after 3 h of exposure.Citation58 However, in contrast to the staining pattern of TLR4 in autologous primary alveolar macrophages, TLR4 staining in AECII is most prominent intracellulary. LPS-induced increases of TLR4 expression are functionally relevant as determined by enhanced TNFα release by both cell types. Thorley et al. suggested that this differential expression of TLR4 by alveolar epithelium and macrophages is important in coordinated responses to inhaled pathogens.Citation58 In addition, the LPS co-receptor CD14, that facilitates TLR4 responsiveness to very low levels (< 1 ng/ml) of LPSCitation67 and controls the LPS-induced endocytosis of TLR4,Citation36 is constitutively expressed on the cell surface of primary human AECII and immortalized AECI.Citation58
Lung cell-specific microenvironmental regulation of TLR4 receptor and adaptor localization
In general, the role of lung cell-specific microenvironmental factors in regulating expression and intracellular TLR4 localization in response to LPS is only beginning to be experimentally addressed. The so far best-studied microenvironmental factor modulating TLR4 signaling in the lung is pulmonary surfactant. Pulmonary SP-A modulates the alveolar macrophage threshold of LPS activity both in vitro and in vivo through various mechanisms. SP-A does not affect constitutive TLR4 surface expression on human monocyte-derived macrophages as determined by flow cytometry.Citation68 Likewise under basal conditions, SP-A has very little effect on TLR4 mRNA expression during monocyte differentiation into macrophages.Citation68 We observed that SP-A alone transiently decreases TLR4 staining at early time points (15 min and 30 min) of exposure and antagonizes LPS-enhanced TLR4 protein expression in primary rat alveolar macrophages.Citation53 The combined data suggest that SP-A, under resting conditions, only transiently affects TLR4 abundancy, but can persistently decrease TLR4 expression levels in the presence of LPS. First evidence for specifically coordinated TLR4 positioning and thus functioning through surfactant protein-mediated alterations in membrane trafficking was provided by a study showing that Survanta, a clinical surfactant extract that contains SP-C, inhibits LPS signaling in vitro by blocking translocation of TLR4 to lipid rafts in A549 cells.Citation69 Data from our group demonstrated that SP-A modulates the spatiotemporal compartmentalization of LPS-induced TLR4 in primary alveolar macrophages in vitro and in a mouse model of intratracheal LPS challenge. SP-A reduces the LPS-induced colocalization of TLR4 with early endosomes and promotes TLR4 localization with the Golgi thereby inhibiting LPS-induced TLR4 signaling.Citation53 Furthermore, the lack of direct SP-A/TLR4 co-localization, the SP-A-mediated upregulation of β-arrestin 2 protein expression and the SP-A-enhanced β-arrestin 2/TLR4 interaction suggest that SP-A-modulated cellular distribution of TLR4 in primary alveolar macrophages is mediated indirectly by integrating β-arrestin 2 scaffolding interactions. However, the underlying mechanisms are unknown but one could speculate that SP-A either decelerates the LPS-induced transport of TLR4 from the Golgi to the plasma membrane or accelerates the retrograde transport of TLR4 from endosomes to the Golgi.Citation53 The combined studies provide first evidence for a critical role of surfactant lipids and proteins in regulating TLR4 subcellular localization thereby fine tuning the quantity of LPS-induced immune responses of the lung. Among numerous negative TLR4 regulators is IL-1R-associated kinase-M (IRAK-M) that is highly expressed in resting human alveolar macrophages.Citation70 IRAK-M inhibits TLR-induced NF-κB activity by binding to MyD88 and TRAFCitation71 and can suppress sepsis-induced lung innate immunity.Citation72 Both SP-A and surfactant lipids (Survanta) upregulate IRAK-M expression in human alveolar macrophages over 24 h leading to reduced LPS-stimulated cytokine release.Citation73 The host defense peptide cathelicidin LL37 inhibits TLR4 responses in myeoloid cellsCitation74 but enhances the LPS-induced activation of primary human bronchial epithelial cells by facilitating the delivery of LPS to TLR4-containing intracellular compartments.Citation75 The combined data provide evidence for lung cell type-specific effects of LL37 on TLR4 activation. Likewise, the host defense peptide CLP-19 inhibits LPS-induced microtubule-dependent translocation of TLR4 from the endoplasmic reticulum to the cell surface in the mouse macrophage cell line RAW264.7 functionally inhibiting TNF release and phosphorylation of IκB-α.Citation76 The tetraspanin CD9 prevents LPS-induced TLR4 localization at lipid-enriched membrane microdomains in RAW264.7 cells and pro-inflammatory cytokine release by alveolar macrophages after in vivo LPS challenge thereby preventing LPS-induced lung inflammation.Citation77 In vivo oxidative stress induces the formation of a surface TLR4 receptor complex within lipid rafts of rat alveolar macrophages resulting in an augmented responsiveness to LPS.Citation78 This was the first study showing that oxidative stress alters subcellular TLR4 distribution in vivo. Actin depolymerization is required for multiple steps of endocytic receptor trafficking.Citation79 The actin depolymerization protein glia maturation factor-γ (GMFG) is highly expressed in endothelial cells derived from human lung.Citation80 Recent data demonstrated that GMFG functions as a negative regulator of LPS-induced TLR4 signaling by promoting TLR4 trafficking from early endosomes to late endosomes in primary human macrophages without affecting TLR4 expression levels.Citation81 Activated adenylyl cyclases mediate the conversion of ATP to cAMP whose compartmentalized signaling regulates central cellular processes in the lung.Citation82 The AC6 isoform, expressed in lung structural and immune cells,Citation82 promotes a shift of TLR4 endocytosis via the clathrin-dependent pathway toward the lipid-raft-mediated pathway resulting in an accelerated degradation of TLR4 with subsequently suppressed downstream signaling in RAW264.7 cells and bone-marrow-derived macrophages.Citation83 The combined studies provide evidence for a critical role of lung-specific microenvironmental factors in TLR4 trafficking and thus signaling.
Dysregulation of TLR4 spatiotemporal localization associated with Gram-negative human lung diseases
Two frequently occurringCitation84 single nucleotide polymorphisms (SNPs) in the human TLR4 ecto-domain, the missense mutations Asp299Gly (D299G) and Thr399Ile (T399I), are associated with LPS hyporesponsiveness and enhanced susceptibility to infection with Gram-negative bacteria.Citation85 Another study on human individual genetic variations revealed a combination of D299G and T399I within the TLR4 and Ser180Leu within the TIRAP/MAL gene is associated with a significantly higher risk for developing sepsis and pneumonia.Citation86 Recent data provide structural and biological evidence of the functionality of the mutant TLR4 carrying T399I and D299G, respectively.Citation87,Citation88 First evidence for a mechanism by which a TLR4 polymorphism alters TLR4 signaling was provided by Figueroa et al. demonstrating that the D299G polymorphism impairs the recruitment of MyD88 and TRIF to TLR4 and subsequent pathway-specific signaling.Citation88
Studies on TLR4 subcellular localization and signaling employing primary alveolar macrophages from patients suffering from lung diseases induced by or associated with Gram-negative infection are limited. One disease, for which first data relevant to this subject are provided, is cystic fibrosis (CF). Uncontrolled inflammatory response to infection with Pseudomonas aeruginosa is associated with morbidity and mortality in patients with CF.Citation89 Abnormal trafficking and degradation of TLR4 in macrophages results in elevated inflammatory responses in both murine CF models and patients suffering from CF.Citation90 Untreated CFTR−/− bone-marrow derived macrophages display significantly higher levels of plasma membrane-associated TLR4 than wild-type macrophages.Citation90 Upon LPS stimulation, the functionally hyperresponsive phenotype of CFTR−/− macrophages is associated with a prolonged retention of TLR4 in early endosomes.Citation90 Similarly, differentiated human monocytes from CF patients are hyperresponsive to LPS.Citation90 Kelly et al. examined the intracellular trafficking of TLR4 in primary nasal epithelial cells from non-CF controls and CF patients after LPS challenge. They found a sustained gene expression of Rab10 in CF epithelium and an abolished TLR4 targeting to the lysosome in CF airway epithelial cells.Citation91 The combined data imply that in pulmonary and immune cells from CF patients TLR4 spends a prolonged time in the early endosome resulting in enhanced signaling.
Because pneumonia induced by Gram-negative bacteria frequently develops postoperatively,Citation92 Chalk et al. recently started a pilot study to characterize lung-cell specific alterations of the immune profile after cardiac surgery.Citation93 Patients who postoperatively developed pneumonia revealed a stronger reduction of TLR4 expression on alveolar macrophages than patients who did notCitation93 suggesting that a local cell-mediated immunosuppression in the lung might be a risk factor for postoperative pneumonia. The expression of TLR4 on alveolar macrophages from patients with ARDS is suppressed and, compared with control cells, does not change after ex vivo stimulation of the cells with LPS.Citation94 Comparably, though employing blood monocytes, decreased TLR4 expression on these cells is associated with mortality in elderly patients with severe pneumonia.Citation95 Together, these studies suggest that TLR4 expression profiles on hematopoietic cells are linked to pulmonary diseases induced by or associated with Gram-negative bacteria.
Conclusions
Emerging evidence demonstrates the functional significance of subcellular TLR4 receptor and adaptor localization in regulating cellular responses to LPS. It is, however, unknown whether cell type-specific differences in TLR4 compartmentalization are associated with individual responses to TLR4 ligation by LPS and thus LPS signaling specific for the lung is only beginning to be experimentally addressed. Pulmonary surfactant-modulated trafficking of the TLR4 receptor in primary alveolar macrophages provides first evidence for lung-specific regulation of TLR4 signaling. Future studies are likely to focus increasingly on whether defined surfactant compositions can switch subcellular TLR4 localization in response to LPS. Further investigations on lung cell type-specific TLR4 responses and the impact of unique pulmonary microenvironmental factors on intracellular TLR4 positioning and thus ligand sensing will help to potentially improve the qualitative and quantitative outcome of innate immune responses of the lung to Gram-negative bacteria.
Abbreviations: | ||
AECI | = | type I alveolar epithelial cells |
AECII | = | type II alveolar epithelial cells |
IFN | = | interferon |
IL | = | interleukin |
IRAK | = | interleukin-1 receptor-associated kinase |
IRF | = | interferon regulatory factor |
LPS | = | lipopolysaccharide |
MyD88 | = | myeloid differentiation primary response gene 88 |
NF-κB | = | nuclear factor κB |
SP-A | = | surfactant protein A |
SP-D | = | surfactant protein D |
TIRAP | = | toll-interleukin 1 receptor (TIR) domain containing adaptor protein |
TLR4 | = | Toll-like receptor 4 |
TNF-α | = | tumor necrosis factor-α |
TRAM | = | TRIF-related adaptor molecule |
TRIF | = | toll/Il-1R-domain-containing adaptor protein inducing interferon-β |
Disclosure of Potential Conflicts of Interest
No potential conflicts of interest were disclosed.
Acknowledgments
We thank Birgitta Henriques-Normark and Staffan Normark for critical reading of the manuscript. Work done in the author’s laboratory was supported by grants from the German Research Council (DFG, Grant 609/1-4 and 609/2–1 to C.S.).
References
- Wright JR. Immunoregulatory functions of surfactant proteins. Nat Rev Immunol 2005; 5:58 - 68; http://dx.doi.org/10.1513/pats.200701-018AW; PMID: 17607008
- Goerke J. Pulmonary surfactant: functions and molecular composition. Biochim Biophys Acta 1998; 1408:79 - 89; http://dx.doi.org/10.1016/S0925-4439(98)00060-X; PMID: 9813251
- Pérez-Gil J. Structure of pulmonary surfactant membranes and films: the role of proteins and lipid-protein interactions. Biochim Biophys Acta 2008; 1778:1676 - 95; http://dx.doi.org/10.1016/j.bbamem.2008.05.003; PMID: 18515069
- LeVine AM, Whitsett JA. Pulmonary collectins and innate host defense of the lung. Microbes Infect 2001; 3:161 - 6; http://dx.doi.org/10.4049/jimmunol.181.1.621; PMID: 18566429
- Glasser SW, Maxfield MD, Ruetschilling TL, Akinbi HT, Baatz JE, Kitzmiller JA, Page K, Xu Y, Bao EL, Korfhagen TR. Persistence of LPS-induced lung inflammation in surfactant protein-C-deficient mice. Am J Respir Cell Mol Biol 2013; 49:845 - 54; http://dx.doi.org/10.1165/rcmb.2012-0374OC; PMID: 23795648
- Whitsett JA. Review: The intersection of surfactant homeostasis and innate host defense of the lung: lessons from newborn infants. Innate Immun 2010; 16:138 - 42; http://dx.doi.org/10.1177/1753425910366879; PMID: 20351134
- Koulenti D, Rello J. Gram-negative bacterial pneumonia: aetiology and management. Curr Opin Pulm Med 2006; 12:198 - 204; PMID: 16582675
- Poltorak A, He X, Smirnova I, Liu MY, Van Huffel C, Du X, Birdwell D, Alejos E, Silva M, Galanos C, et al. Defective LPS signaling in C3H/HeJ and C57BL/10ScCr mice: mutations in Tlr4 gene. Science 1998; 282:2085 - 8; http://dx.doi.org/10.1126/science.282.5396.2085; PMID: 9851930
- Hoshino K, Takeuchi O, Kawai T, Sanjo H, Ogawa T, Takeda Y, Takeda K, Akira S. Cutting edge: Toll-like receptor 4 (TLR4)-deficient mice are hyporesponsive to lipopolysaccharide: evidence for TLR4 as the Lps gene product. J Immunol 1999; 162:3749 - 52; PMID: 10201887
- Branger J, Knapp S, Weijer S, Leemans JC, Pater JM, Speelman P, Florquin S, van der Poll T. Role of Toll-like receptor 4 in gram-positive and gram-negative pneumonia in mice. Infect Immun 2004; 72:788 - 94; http://dx.doi.org/10.1128/IAI.72.2.788-794.2004; PMID: 14742522
- Schurr JR, Young E, Byrne P, Steele C, Shellito JE, Kolls JK. Central role of toll-like receptor 4 signaling and host defense in experimental pneumonia caused by Gram-negative bacteria. Infect Immun 2005; 73:532 - 45; http://dx.doi.org/10.1128/IAI.73.1.532-545.2005; PMID: 15618193
- Standiford LR, Standiford TJ, Newstead MJ, Zeng X, Ballinger MN, Kovach MA, Reka AK, Bhan U. TLR4-dependent GM-CSF protects against lung injury in Gram-negative bacterial pneumonia. Am J Physiol Lung Cell Mol Physiol 2012; 302:L447 - 54; http://dx.doi.org/10.1152/ajplung.00415.2010; PMID: 22160309
- Power MR, Peng Y, Maydanski E, Marshall JS, Lin TJ. The development of early host response to Pseudomonas aeruginosa lung infection is critically dependent on myeloid differentiation factor 88 in mice. J Biol Chem 2004; 279:49315 - 22; http://dx.doi.org/10.1074/jbc.M402111200; PMID: 15375173
- Wang X, Moser C, Louboutin JP, Lysenko ES, Weiner DJ, Weiser JN, Wilson JM. Toll-like receptor 4 mediates innate immune responses to Haemophilus influenzae infection in mouse lung. J Immunol 2002; 168:810 - 5; http://dx.doi.org/10.4049/jimmunol.168.2.810; PMID: 11777976
- Jeyaseelan S, Young SK, Yamamoto M, Arndt PG, Akira S, Kolls JK, Worthen GS. Toll/IL-1R domain-containing adaptor protein (TIRAP) is a critical mediator of antibacterial defense in the lung against Klebsiella pneumoniae but not Pseudomonas aeruginosa. J Immunol 2006; 177:538 - 47; http://dx.doi.org/10.4049/jimmunol.177.1.538; PMID: 16785551
- Cai S, Batra S, Shen L, Wakamatsu N, Jeyaseelan S. Both TRIF- and MyD88-dependent signaling contribute to host defense against pulmonary Klebsiella infection. J Immunol 2009; 183:6629 - 38; http://dx.doi.org/10.4049/jimmunol.0901033; PMID: 19846873
- Skerrett SJ, Liggitt HD, Hajjar AM, Wilson CB. Cutting edge: myeloid differentiation factor 88 is essential for pulmonary host defense against Pseudomonas aeruginosa but not Staphylococcus aureus. J Immunol 2004; 172:3377 - 81; http://dx.doi.org/10.4049/jimmunol.172.6.3377; PMID: 15004134
- Power MR, Li B, Yamamoto M, Akira S, Lin TJ. A role of Toll-IL-1 receptor domain-containing adaptor-inducing IFN-beta in the host response to Pseudomonas aeruginosa lung infection in mice. J Immunol 2007; 178:3170 - 6; http://dx.doi.org/10.4049/jimmunol.178.5.3170; PMID: 17312165
- Wieland CW, Florquin S, Maris NA, Hoebe K, Beutler B, Takeda K, Akira S, van der Poll T. The MyD88-dependent, but not the MyD88-independent, pathway of TLR4 signaling is important in clearing nontypeable Haemophilus influenzae from the mouse lung. J Immunol 2005; 175:6042 - 9; http://dx.doi.org/10.4049/jimmunol.175.9.6042; PMID: 16237099
- Jeyaseelan S, Manzer R, Young SK, Yamamoto M, Akira S, Mason RJ, Worthen GS. Toll-IL-1 receptor domain-containing adaptor protein is critical for early lung immune responses against Escherichia coli lipopolysaccharide and viable Escherichia coli. J Immunol 2005; 175:7484 - 95; http://dx.doi.org/10.4049/jimmunol.175.11.7484; PMID: 16301656
- Wieland CW, van Lieshout MH, Hoogendijk AJ, van der Poll T. Host defence during Klebsiella pneumonia relies on haematopoietic-expressed Toll-like receptors 4 and 2. Eur Respir J 2011; 37:848 - 57; http://dx.doi.org/10.1183/09031936.00076510; PMID: 20650991
- Detmers PA, Thieblemont N, Vasselon T, Pironkova R, Miller DS, Wright SD. Potential role of membrane internalization and vesicle fusion in adhesion of neutrophils in response to lipopolysaccharide and TNF. J Immunol 1996; 157:5589 - 96; PMID: 8955211
- Thiéblemont N, Wright SD. Mice genetically hyporesponsive to lipopolysaccharide (LPS) exhibit a defect in endocytic uptake of LPS and ceramide. J Exp Med 1997; 185:2095 - 100; http://dx.doi.org/10.1084/jem.185.12.2095; PMID: 9182681
- Thieblemont N, Thieringer R, Wright SD. Innate immune recognition of bacterial lipopolysaccharide: dependence on interactions with membrane lipids and endocytic movement. Immunity 1998; 8:771 - 7; http://dx.doi.org/10.1016/S1074-7613(00)80582-8; PMID: 9655491
- Barton GM, Kagan JC. A cell biological view of Toll-like receptor function: regulation through compartmentalization. Nat Rev Immunol 2009; 9:535 - 42; http://dx.doi.org/10.1038/nri2587; PMID: 19556980
- McGettrick AF, O’Neill LA. Localisation and trafficking of Toll-like receptors: an important mode of regulation. Curr Opin Immunol 2010; 22:20 - 7; http://dx.doi.org/10.1016/j.coi.2009.12.002; PMID: 20060278
- Latz E, Visintin A, Lien E, Fitzgerald KA, Monks BG, Kurt-Jones EA, Golenbock DT, Espevik T. Lipopolysaccharide rapidly traffics to and from the Golgi apparatus with the toll-like receptor 4-MD-2-CD14 complex in a process that is distinct from the initiation of signal transduction. J Biol Chem 2002; 277:47834 - 43; http://dx.doi.org/10.1074/jbc.M207873200; PMID: 12324469
- Nagai Y, Akashi S, Nagafuku M, Ogata M, Iwakura Y, Akira S, Kitamura T, Kosugi A, Kimoto M, Miyake K. Essential role of MD-2 in LPS responsiveness and TLR4 distribution. Nat Immunol 2002; 3:667 - 72; PMID: 12055629
- Triantafilou M, Miyake K, Golenbock DT, Triantafilou K. Mediators of innate immune recognition of bacteria concentrate in lipid rafts and facilitate lipopolysaccharide-induced cell activation. J Cell Sci 2002; 115:2603 - 11; PMID: 12045230
- Triantafilou M, Morath S, Mackie A, Hartung T, Triantafilou K. Lateral diffusion of Toll-like receptors reveals that they are transiently confined within lipid rafts on the plasma membrane. J Cell Sci 2004; 117:4007 - 14; http://dx.doi.org/10.1242/jcs.01270; PMID: 15286178
- Kagan JC, Medzhitov R. Phosphoinositide-mediated adaptor recruitment controls Toll-like receptor signaling. Cell 2006; 125:943 - 55; http://dx.doi.org/10.1016/j.cell.2006.03.047; PMID: 16751103
- Kagan JC, Su T, Horng T, Chow A, Akira S, Medzhitov R. TRAM couples endocytosis of Toll-like receptor 4 to the induction of interferon-beta. Nat Immunol 2008; 9:361 - 8; http://dx.doi.org/10.1038/ni1569; PMID: 18297073
- Tanimura N, Saitoh S, Matsumoto F, Akashi-Takamura S, Miyake K. Roles for LPS-dependent interaction and relocation of TLR4 and TRAM in TRIF-signaling. Biochem Biophys Res Commun 2008; 368:94 - 9; http://dx.doi.org/10.1016/j.bbrc.2008.01.061; PMID: 18222170
- Husebye H, Halaas Ø, Stenmark H, Tunheim G, Sandanger Ø, Bogen B, Brech A, Latz E, Espevik T. Endocytic pathways regulate Toll-like receptor 4 signaling and link innate and adaptive immunity. EMBO J 2006; 25:683 - 92; http://dx.doi.org/10.1038/sj.emboj.7600991; PMID: 16467847
- Thieblemont N, Wright SD. Transport of bacterial lipopolysaccharide to the golgi apparatus. J Exp Med 1999; 190:523 - 34; http://dx.doi.org/10.1084/jem.190.4.523; PMID: 10449523
- Zanoni I, Ostuni R, Marek LR, Barresi S, Barbalat R, Barton GM, Granucci F, Kagan JC. CD14 controls the LPS-induced endocytosis of Toll-like receptor 4. Cell 2011; 147:868 - 80; http://dx.doi.org/10.1016/j.cell.2011.09.051; PMID: 22078883
- Aksoy E, Taboubi S, Torres D, Delbauve S, Hachani A, Whitehead MA, Pearce WP, Berenjeno IM, Nock G, Filloux A, et al. The p110δ isoform of the kinase PI(3)K controls the subcellular compartmentalization of TLR4 signaling and protects from endotoxic shock. Nat Immunol 2012; 13:1045 - 54; http://dx.doi.org/10.1038/ni.2426; PMID: 23023391
- Palsson-McDermott EM, Doyle SL, McGettrick AF, Hardy M, Husebye H, Banahan K, Gong M, Golenbock D, Espevik T, O’Neill LA. TAG, a splice variant of the adaptor TRAM, negatively regulates the adaptor MyD88-independent TLR4 pathway. Nat Immunol 2009; 10:579 - 86; http://dx.doi.org/10.1038/ni.1727; PMID: 19412184
- Doyle SL, Husebye H, Connolly DJ, Espevik T, O’Neill LA, McGettrick AF. The GOLD domain-containing protein TMED7 inhibits TLR4 signalling from the endosome upon LPS stimulation. Nat Commun 2012; 3:707; http://dx.doi.org/10.1038/ncomms1706; PMID: 22426228
- Stenmark H. Rab GTPases as coordinators of vesicle traffic. Nat Rev Mol Cell Biol 2009; 10:513 - 25; http://dx.doi.org/10.1038/nrm2728; PMID: 19603039
- Progida C, Cogli L, Piro F, De Luca A, Bakke O, Bucci C. Rab7b controls trafficking from endosomes to the TGN. J Cell Sci 2010; 123:1480 - 91; http://dx.doi.org/10.1242/jcs.051474; PMID: 20375062
- Wang Y, Chen T, Han C, He D, Liu H, An H, Cai Z, Cao X. Lysosome-associated small Rab GTPase Rab7b negatively regulates TLR4 signaling in macrophages by promoting lysosomal degradation of TLR4. Blood 2007; 110:962 - 71; http://dx.doi.org/10.1182/blood-2007-01-066027; PMID: 17395780
- Wang D, Lou J, Ouyang C, Chen W, Liu Y, Liu X, Cao X, Wang J, Lu L. Ras-related protein Rab10 facilitates TLR4 signaling by promoting replenishment of TLR4 onto the plasma membrane. Proc Natl Acad Sci U S A 2010; 107:13806 - 11; http://dx.doi.org/10.1073/pnas.1009428107; PMID: 20643919
- Husebye H, Aune MH, Stenvik J, Samstad E, Skjeldal F, Halaas O, Nilsen NJ, Stenmark H, Latz E, Lien E, et al. The Rab11a GTPase controls Toll-like receptor 4-induced activation of interferon regulatory factor-3 on phagosomes. Immunity 2010; 33:583 - 96; http://dx.doi.org/10.1016/j.immuni.2010.09.010; PMID: 20933442
- Ferguson JS, Martin JL, Azad AK, McCarthy TR, Kang PB, Voelker DR, Crouch EC, Schlesinger LS. Surfactant protein D increases fusion of Mycobacterium tuberculosis-containing phagosomes with lysosomes in human macrophages. Infect Immun 2006; 74:7005 - 9; http://dx.doi.org/10.1128/IAI.01402-06; PMID: 17030585
- Sawada K, Ariki S, Kojima T, Saito A, Yamazoe M, Nishitani C, Shimizu T, Takahashi M, Mitsuzawa H, Yokota S, et al. Pulmonary collectins protect macrophages against pore-forming activity of Legionella pneumophila and suppress its intracellular growth. J Biol Chem 2010; 285:8434 - 43; http://dx.doi.org/10.1074/jbc.M109.074765; PMID: 20056602
- Sender V, Moulakakis C, Stamme C. Pulmonary surfactant protein A enhances endolysosomal trafficking in alveolar macrophages through regulation of Rab7. J Immunol 2011; 186:2397 - 411; http://dx.doi.org/10.4049/jimmunol.1002446; PMID: 21248257
- Imai Y, Kuba K, Neely GG, Yaghubian-Malhami R, Perkmann T, van Loo G, Ermolaeva M, Veldhuizen R, Leung YH, Wang H, et al. Identification of oxidative stress and Toll-like receptor 4 signaling as a key pathway of acute lung injury. Cell 2008; 133:235 - 49; http://dx.doi.org/10.1016/j.cell.2008.02.043; PMID: 18423196
- Fan J, Kapus A, Marsden PA, Li YH, Oreopoulos G, Marshall JC, Frantz S, Kelly RA, Medzhitov R, Rotstein OD. Regulation of Toll-like receptor 4 expression in the lung following hemorrhagic shock and lipopolysaccharide. J Immunol 2002; 168:5252 - 9; http://dx.doi.org/10.4049/jimmunol.168.10.5252; PMID: 11994482
- Oshikawa K, Sugiyama Y. Gene expression of Toll-like receptors and associated molecules induced by inflammatory stimuli in the primary alveolar macrophage. Biochem Biophys Res Commun 2003; 305:649 - 55; http://dx.doi.org/10.1016/S0006-291X(03)00837-4; PMID: 12763043
- Punturieri A, Alviani RS, Polak T, Copper P, Sonstein J, Curtis JL. Specific engagement of TLR4 or TLR3 does not lead to IFN-beta-mediated innate signal amplification and STAT1 phosphorylation in resident murine alveolar macrophages. J Immunol 2004; 173:1033 - 42; http://dx.doi.org/10.4049/jimmunol.173.2.1033; PMID: 15240691
- Saito T, Yamamoto T, Kazawa T, Gejyo H, Naito M. Expression of toll-like receptor 2 and 4 in lipopolysaccharide-induced lung injury in mouse. Cell Tissue Res 2005; 321:75 - 88; http://dx.doi.org/10.1007/s00441-005-1113-9; PMID: 15902499
- Sender V, Lang L, Stamme C. Surfactant protein-A modulates LPS-induced TLR4 localization and signaling via β-arrestin 2. PLoS One 2013; 8:e59896; http://dx.doi.org/10.1371/journal.pone.0059896; PMID: 23536892
- Hollingsworth JW, Chen BJ, Brass DM, Berman K, Gunn MD, Cook DN, Schwartz DA. The critical role of hematopoietic cells in lipopolysaccharide-induced airway inflammation. Am J Respir Crit Care Med 2005; 171:806 - 13; http://dx.doi.org/10.1164/rccm.200407-953OC; PMID: 15618460
- Srivastava M, Jung S, Wilhelm J, Fink L, Bühling F, Welte T, Bohle RM, Seeger W, Lohmeyer J, Maus UA. The inflammatory versus constitutive trafficking of mononuclear phagocytes into the alveolar space of mice is associated with drastic changes in their gene expression profiles. J Immunol 2005; 175:1884 - 93; http://dx.doi.org/10.4049/jimmunol.175.3.1884; PMID: 16034132
- Droemann D, Goldmann T, Tiedje T, Zabel P, Dalhoff K, Schaaf B. Toll-like receptor 2 expression is decreased on alveolar macrophages in cigarette smokers and COPD patients. Respir Res 2005; 6:68; http://dx.doi.org/10.1186/1465-9921-6-68; PMID: 16004610
- Juarez E, Nuñez C, Sada E, Ellner JJ, Schwander SK, Torres M. Differential expression of Toll-like receptors on human alveolar macrophages and autologous peripheral monocytes. Respir Res 2010; 11:2; http://dx.doi.org/10.1186/1465-9921-11-2; PMID: 20051129
- Thorley AJ, Grandolfo D, Lim E, Goldstraw P, Young A, Tetley TD. Innate immune responses to bacterial ligands in the peripheral human lung--role of alveolar epithelial TLR expression and signalling. PLoS One 2011; 6:e21827; http://dx.doi.org/10.1371/journal.pone.0021827; PMID: 21789185
- Maris NA, Dessing MC, de Vos AF, Bresser P, van der Zee JS, Jansen HM, Spek CA, van der Poll T. Toll-like receptor mRNA levels in alveolar macrophages after inhalation of endotoxin. Eur Respir J 2006; 28:622 - 6; http://dx.doi.org/10.1183/09031936.06.00010806; PMID: 16946093
- Hoogerwerf JJ, de Vos AF, van’t Veer C, Bresser P, de Boer A, Tanck MW, Draing C, van der Zee JS, van der Poll T. Priming of alveolar macrophages upon instillation of lipopolysaccharide in the human lung. Am J Respir Cell Mol Biol 2010; 42:349 - 56; http://dx.doi.org/10.1165/rcmb.2008-0362OC; PMID: 19448156
- Wong MH, Chapin OC, Johnson MD. LPS-stimulated cytokine production in type I cells is modulated by the renin-angiotensin system. Am J Respir Cell Mol Biol 2012; 46:641 - 50; http://dx.doi.org/10.1165/rcmb.2011-0289OC; PMID: 22205632
- Wong MH, Johnson MD. Differential response of primary alveolar type I and type II cells to LPS stimulation. PLoS One 2013; 8:e55545; http://dx.doi.org/10.1371/journal.pone.0055545; PMID: 23383221
- Schulz C, Farkas L, Wolf K, Kratzel K, Eissner G, Pfeifer M. Differences in LPS-induced activation of bronchial epithelial cells (BEAS-2B) and type II-like pneumocytes (A-549). Scand J Immunol 2002; 56:294 - 302; http://dx.doi.org/10.1046/j.1365-3083.2002.01137.x; PMID: 12193231
- Becker MN, Diamond G, Verghese MW, Randell SH. CD14-dependent lipopolysaccharide-induced beta-defensin-2 expression in human tracheobronchial epithelium. J Biol Chem 2000; 275:29731 - 6; http://dx.doi.org/10.1074/jbc.M000184200; PMID: 10882713
- Guillot L, Medjane S, Le-Barillec K, Balloy V, Danel C, Chignard M, Si-Tahar M. Response of human pulmonary epithelial cells to lipopolysaccharide involves Toll-like receptor 4 (TLR4)-dependent signaling pathways: evidence for an intracellular compartmentalization of TLR4. J Biol Chem 2004; 279:2712 - 8; http://dx.doi.org/10.1074/jbc.M305790200; PMID: 14600154
- Armstrong L, Medford AR, Uppington KM, Robertson J, Witherden IR, Tetley TD, Millar AB. Expression of functional toll-like receptor-2 and -4 on alveolar epithelial cells. Am J Respir Cell Mol Biol 2004; 31:241 - 5; http://dx.doi.org/10.1165/rcmb.2004-0078OC; PMID: 15044215
- Frey EA, Miller DS, Jahr TG, Sundan A, Bazil V, Espevik T, Finlay BB, Wright SD. Soluble CD14 participates in the response of cells to lipopolysaccharide. J Exp Med 1992; 176:1665 - 71; http://dx.doi.org/10.1084/jem.176.6.1665; PMID: 1281215
- Henning LN, Azad AK, Parsa KV, Crowther JE, Tridandapani S, Schlesinger LS. Pulmonary surfactant protein A regulates TLR expression and activity in human macrophages. J Immunol 2008; 180:7847 - 58; http://dx.doi.org/10.4049/jimmunol.180.12.7847; PMID: 18523248
- Abate W, Alghaithy AA, Parton J, Jones KP, Jackson SK. Surfactant lipids regulate LPS-induced interleukin-8 production in A549 lung epithelial cells by inhibiting translocation of TLR4 into lipid raft domains. J Lipid Res 2010; 51:334 - 44; http://dx.doi.org/10.1194/jlr.M000513; PMID: 19648651
- Kobayashi H, Nolan A, Naveed B, Hoshino Y, Segal LN, Fujita Y, Rom WN, Weiden MD. Neutrophils activate alveolar macrophages by producing caspase-6-mediated cleavage of IL-1 receptor-associated kinase-M. J Immunol 2011; 186:403 - 10; http://dx.doi.org/10.4049/jimmunol.1001906; PMID: 21098228
- Ye H, Arron JR, Lamothe B, Cirilli M, Kobayashi T, Shevde NK, Segal D, Dzivenu OK, Vologodskaia M, Yim M, et al. Distinct molecular mechanism for initiating TRAF6 signalling. Nature 2002; 418:443 - 7; http://dx.doi.org/10.1038/nature00888; PMID: 12140561
- Deng JC, Cheng G, Newstead MW, Zeng X, Kobayashi K, Flavell RA, Standiford TJ. Sepsis-induced suppression of lung innate immunity is mediated by IRAK-M. J Clin Invest 2006; 116:2532 - 42; PMID: 16917541
- Nguyen HA, Rajaram MV, Meyer DA, Schlesinger LS. Pulmonary surfactant protein A and surfactant lipids upregulate IRAK-M, a negative regulator of TLR-mediated inflammation in human macrophages. Am J Physiol Lung Cell Mol Physiol 2012; 303:L608 - 16; http://dx.doi.org/10.1152/ajplung.00067.2012; PMID: 22886503
- Mookherjee N, Brown KL, Bowdish DM, Doria S, Falsafi R, Hokamp K, Roche FM, Mu R, Doho GH, Pistolic J, et al. Modulation of the TLR-mediated inflammatory response by the endogenous human host defense peptide LL-37. J Immunol 2006; 176:2455 - 64; http://dx.doi.org/10.4049/jimmunol.176.4.2455; PMID: 16456005
- Shaykhiev R, Sierigk J, Herr C, Krasteva G, Kummer W, Bals R. The antimicrobial peptide cathelicidin enhances activation of lung epithelial cells by LPS. FASEB J 2010; 24:4756 - 66; http://dx.doi.org/10.1096/fj.09-151332; PMID: 20696857
- Li D, Liu Y, Yang Y, Chen JH, Yang J, Zou LY, Tian ZQ, Lv J, Xia PY. Looped host defense peptide CLP-19 binds to microtubules and inhibits surface expression of TLR4 on mouse macrophages. J Immunol 2013; 190:6083 - 92; http://dx.doi.org/10.4049/jimmunol.1203167; PMID: 23667111
- Suzuki M, Tachibana I, Takeda Y, He P, Minami S, Iwasaki T, Kida H, Goya S, Kijima T, Yoshida M, et al. Tetraspanin CD9 negatively regulates lipopolysaccharide-induced macrophage activation and lung inflammation. J Immunol 2009; 182:6485 - 93; http://dx.doi.org/10.4049/jimmunol.0802797; PMID: 19414803
- Powers KA, Szászi K, Khadaroo RG, Tawadros PS, Marshall JC, Kapus A, Rotstein OD. Oxidative stress generated by hemorrhagic shock recruits Toll-like receptor 4 to the plasma membrane in macrophages. J Exp Med 2006; 203:1951 - 61; http://dx.doi.org/10.1084/jem.20060943; PMID: 16847070
- Kaksonen M, Toret CP, Drubin DG. Harnessing actin dynamics for clathrin-mediated endocytosis. Nat Rev Mol Cell Biol 2006; 7:404 - 14; http://dx.doi.org/10.1038/nrm1940; PMID: 16723976
- Ikeda K, Kundu RK, Ikeda S, Kobara M, Matsubara H, Quertermous T. Glia maturation factor-gamma is preferentially expressed in microvascular endothelial and inflammatory cells and modulates actin cytoskeleton reorganization. Circ Res 2006; 99:424 - 33; http://dx.doi.org/10.1161/01.RES.0000237662.23539.0b; PMID: 16873721
- Aerbajinai W, Lee K, Chin K, Rodgers GP. Glia maturation factor-γ negatively modulates TLR4 signaling by facilitating TLR4 endocytic trafficking in macrophages. J Immunol 2013; 190:6093 - 103; http://dx.doi.org/10.4049/jimmunol.1203048; PMID: 23677465
- Oldenburger A, Maarsingh H, Schmidt M. Multiple facets of cAMP signalling and physiological impact: cAMP compartmentalization in the lung. Pharmaceuticals (Basel) 2012; 5:1291 - 331; http://dx.doi.org/10.3390/ph5121291; PMID: 24281338
- Cai W, Du A, Feng K, Zhao X, Qian L, Ostrom RS, Xu C. Adenylyl cyclase 6 activation negatively regulates TLR4 signaling through lipid raft-mediated endocytosis. J Immunol 2013; 191:6093 - 100; http://dx.doi.org/10.4049/jimmunol.1301912; PMID: 24218452
- Ferwerda B, McCall MB, Alonso S, Giamarellos-Bourboulis EJ, Mouktaroudi M, Izagirre N, Syafruddin D, Kibiki G, Cristea T, Hijmans A, et al. TLR4 polymorphisms, infectious diseases, and evolutionary pressure during migration of modern humans. Proc Natl Acad Sci U S A 2007; 104:16645 - 50; http://dx.doi.org/10.1073/pnas.0704828104; PMID: 17925445
- Arbour NC, Lorenz E, Schutte BC, Zabner J, Kline JN, Jones M, Frees K, Watt JL, Schwartz DA. TLR4 mutations are associated with endotoxin hyporesponsiveness in humans. Nat Genet 2000; 25:187 - 91; http://dx.doi.org/10.1038/76048; PMID: 10835634
- Kumpf O, Giamarellos-Bourboulis EJ, Koch A, Hamann L, Mouktaroudi M, Oh DY, Latz E, Lorenz E, Schwartz DA, Ferwerda B, et al. Influence of genetic variations in TLR4 and TIRAP/Mal on the course of sepsis and pneumonia and cytokine release: an observational study in three cohorts. Crit Care 2010; 14:R103; http://dx.doi.org/10.1186/cc9047; PMID: 20525286
- Ohto U, Yamakawa N, Akashi-Takamura S, Miyake K, Shimizu T. Structural analyses of human Toll-like receptor 4 polymorphisms D299G and T399I. J Biol Chem 2012; 287:40611 - 7; http://dx.doi.org/10.1074/jbc.M112.404608; PMID: 23055527
- Figueroa L, Xiong Y, Song C, Piao W, Vogel SN, Medvedev AE. The Asp299Gly polymorphism alters TLR4 signaling by interfering with recruitment of MyD88 and TRIF. J Immunol 2012; 188:4506 - 15; http://dx.doi.org/10.4049/jimmunol.1200202; PMID: 22474023
- Campodónico VL, Gadjeva M, Paradis-Bleau C, Uluer A, Pier GB. Airway epithelial control of Pseudomonas aeruginosa infection in cystic fibrosis. Trends Mol Med 2008; 14:120 - 33; http://dx.doi.org/10.1016/j.molmed.2008.01.002; PMID: 18262467
- Bruscia EM, Zhang PX, Satoh A, Caputo C, Medzhitov R, Shenoy A, Egan ME, Krause DS. Abnormal trafficking and degradation of TLR4 underlie the elevated inflammatory response in cystic fibrosis. J Immunol 2011; 186:6990 - 8; http://dx.doi.org/10.4049/jimmunol.1100396; PMID: 21593379
- Kelly C, Canning P, Buchanan PJ, Williams MT, Brown V, Gruenert DC, Elborn JS, Ennis M, Schock BC. Toll-like receptor 4 is not targeted to the lysosome in cystic fibrosis airway epithelial cells. Am J Physiol Lung Cell Mol Physiol 2013; 304:L371 - 82; http://dx.doi.org/10.1152/ajplung.00372.2011; PMID: 23316065
- Hortal J, Muñoz P, Cuerpo G, Litvan H, Rosseel PM, Bouza E, European Study Group on Nosocomial Infections, European Workgroup of Cardiothoracic Intensivists. Ventilator-associated pneumonia in patients undergoing major heart surgery: an incidence study in Europe. Crit Care 2009; 13:R80; http://dx.doi.org/10.1186/cc7896; PMID: 19463176
- Chalk K, Meisel C, Spies C, Volk T, Thuenemann K, Linneweber J, Wernecke KD, Sander M. Dysfunction of alveolar macrophages after 3 cardiac surgery and postoperative pneumonia? - an 5 observational study. Crit Care 2013; 17:R285; http://dx.doi.org/10.1186/cc13148; PMID: 24321282
- Hatzidaki E, Nakos G, Galiatsou E, Lekka ME. Impaired phospholipases A₂production by stimulated macrophages from patients with acute respiratory distress syndrome. Biochim Biophys Acta 2010; 1802:986 - 94; http://dx.doi.org/10.1016/j.bbadis.2010.06.008; PMID: 20600872
- Tang L, Li Q, Bai J, Zhang H, Lu Y, Ma S. Severe pneumonia mortality in elderly patients is associated with downregulation of Toll-like receptors 2 and 4 on monocytes. Am J Med Sci 2014; 347:34 - 41; http://dx.doi.org/10.1097/MAJ.0b013e3182798583; PMID: 23406892