Abstract
Triglycerides and phospholipids play an important role in epidermal permability barrier formation and function. They are synthesized de novo in the epidermis via the glycerol-3-phosphate pathway, catalyzed sequentially by a group of enzymes that have multiple isoforms including glycerol-3-phosphate acyltransferase (GPAT), 1-acylglycerol-3-phosphate acyltransferase (AGPAT), Lipin, and diacylglycerol acyltransferase (DGAT). Here we review the current knowledge of GPAT, AGPAT, Lipin, and DGAT enzymes in keratinocytes/epidermis focusing on the expression levels of the various isoforms and their localization in mouse epidermis. Additionally, the factors regulating their gene expression, including calcium induced differentiation, PPAR and LXR activators, and the effect of acute permeability barrier disruption will be discussed.
Introduction
The principal function of the skin is to provide a protective barrier against the transcutaneous loss of water and electrolytes, which is essential for the survival of a mammal in a terrestrial environment. This epidermal permeability barrier is localized to the stratum corneum, the outermost layer of the skin.Citation1 Stratum corneum consists of corneocytes, terminally differentiated keratinocytes which provide the mechanical strength of the skin, and a hydrophobic, lipid-enriched extracellular matrix, which provides the barrier to the movement of water and electrolytes. These extracellular lipids are primarily composed of cholesterol, ceramides and free fatty acids. On a total lipid mass basis, human stratum corneum contains 50% ceramides, 25% cholesterol and 15% free fatty acids.Citation1 These lipids are delivered to the extracellular spaces of the stratum corneum by the secretion of lamellar body contents by differentiated keratinocytes.Citation1 Lamellar bodies contain cholesterol, glucosylceramides and phospholipids, and following lamellar body secretion the glucosylceramides are metabolized to ceramides, a reaction catalyzed by β-glucocerebrosidase, and the phospholipids are metabolized to free fatty acids, a reaction catalyzed by secretory phospholipases.Citation1–Citation4 Inhibition of cholesterol, fatty acid, ceramide or glucosylceramide synthesis in the epidermis results in abnormal lamellar body formation indicating that the synthesis of sufficient quantities of these lipids (ceramide, glucosylceramide, cholesterol, fatty acids) is required for normal permability barrier homeostasis.Citation1
The free fatty acids produced in the epidermis serve as precursors for both phospholipids and ceramides, which are essential components for lamellar body formation. In human stratum corneum, fatty acids are predominantly straight chained, with C22 and C24 chain lengths being the most abundant.Citation5 These fatty acids include the essential fatty acid linoleate, which is present in acylceramides, and the absence of linoleate (in essential fatty acid deficiency animals) leads to abnormal structure and function of the epidermal permability barrier,Citation6–Citation9 indicating that the essential fatty acids are required for maintaining permeability barrier homeostasis. Recently, mice deficient in comparative gene identification-58 (CGI-58), a lipid droplet associated protein that facilities triglyceride hydrolysis, have been shown to develop a severe permeability barrier defect.Citation10 This along with studies in DGAT-2 deficient mice (see below) indicates that the synthesis and breakdown of triglycerides is also required for permeability barrier homeostasis.
Whereas much is known about the enzymes of sphingolipid and cholesterol synthesis in the epidermis/keratinocytes, little is known about the enzymes required for triglyceride and phospholipid synthesis. In this review, the focus will be on the expression levels and factors that regulate the key enzymes responsible for triglyceride and phospholipid biosynthesis in keratinocytes/epidermis.
Biosynthetic Pathway of Triglycerides and Phospholipids
In mammals, triglycerides are synthesized through two major pathways, the glycerolphosphate (Kennedy) pathway and the monoacylglycerol pathway while phospholipids are synthesized via the glycerolphosphate (Kennedy) pathway.Citation11,Citation12 The glycerol phosphate pathway is responsible for the majority of de novo biosynthesis of triglycerides in most cell types, while the monoacylglycerol pathway is very important in triglyceride synthesis in the small intestine.Citation11,Citation12 Here we focus on the glycerol phosphate pathway, in which the acylation of glycerol 3-phosphate occurs through a stepwise addition of activated fatty acyl groups, each of which is catalyzed by distinct enzymes ().
The initial committed step in triglyceride/phospholipid synthesis via the glycerol phosphate pathway is the acylation of glycerol-3-phosphate by glycerol-3-phosphate acyltransferase (GPAT, EC 2.3.1.15).Citation13–Citation15 The resulting product lysophosphatidic acid is further acylated by 1-acylglycerol-3-phosphate acyltransferase (AGPAT, EC 2.3.1.51) to produce phosphatidate.Citation13,Citation16 Phosphatidate is a branch point in lipid synthesis: it can serve as a precursor for either acidic phospholipids (PI, PG, CL) or diacylglycerol biosynthesis. The conversion of phosphatidate to diacylglycerol is catalyzed by lipins, a group of hydrolyases which have phosphatidate phosphatase (PAP1) enzyme activity. Finally, diacylglycerol is converted to triacylglycerol through the action of diacylglycerol acyltransferase (DGAT), or serves as precursor for phospholipid (PC, PS, PE) synthesis.Citation17,Citation18
The Expression and Regulation of Key Enzymes in the Biosynthetic Pathway of Triglyceride/Phospholipid in Keratinocytes/Epidermis
GPATs.
In eukaryotic cells, several GPAT isoforms exist that differ in subcellular localization, sensitivity to N-ethylmaleimide (NEM) inactivation and substrate preferences.Citation12–Citation14 So far, four GPAT genes (GPAT-1, -2, -3 and -4) have been cloned and their products demonstrated to have GPAT activities.Citation13,Citation14 GPAT-1 encodes a NEM-resistant enzyme, which is located to the outer membrane of mitochondria with a substrate preference for saturated palmitoyl-CoA. GPAT-2 encodes a NEM-sensitive mitochondrial isoform, but it is expressed in limited tissues and to date only has been found in testis and liver. Both GPAT-3 and GPAT-4 are ER-associated, NEM-sensitive enzymes, which utilize a broad range of long-chain fatty acyl-CoAs, including both saturated and unsaturated species, as substrates.Citation12–Citation14
In both cultured human keratinocytes and mouse epidermis, GPAT-1, -3 and -4 mRNAs can be detected using real-time PCR, with CT values of ∼24–25 for GPAT-1, ∼23–24 for GPAT-3, and ∼27–28 for GPAT-4 (). In contrast, under the same experimental conditions, GPAT-2 is not detected in either human keratinocytes or in mouse epidermis (but is found in mouse liver),Citation19 (). GPAT-3 and -4 are mainly localized to the upper epidermis (stratum corneum/granulosum/spinosum) and GPAT-1 is found in both the upper and lower (stratum basale) epidermis in mice.Citation19
In fetal rat, epidermal stratification begins on day 17, a burst of lipid synthesis occurs on day 19, a multilayered stratum corneum with a competent barrier to transepidermal water loss is formed between day 19 and 21, and the rat is born with a competent barrier on day 22.Citation20,Citation21 Similar to cultured human keratinocytes and mouse epidermis, GPAT-2 mRNA is not detected in fetal rat epidermis.Citation19 The mRNA levels of GPAT-1 and GPAT-3 are found to increase on day 19, reaching a peak on day 20, and declining thereafter. GPAT-4 mRNA levels also change from days 17–22, but to lesser extent.Citation19 These results indicate that the expression of GPAT-1 and GPAT-3 significantly increases during the late stage of fetal epidermal development, concurrent with the formation of lamellar bodies and SC extracellular lipid membranes.Citation21
It is well recognized that high calcium induces keratinocyte differentiation. In response to high calcium, GPAT-3 mRNA levels markedly increase whereas both GPAT-1 and GPAT-4 mRNA levels decrease by ∼50% at most time points in primarily cultured human keratinocytes.Citation19 In parallel, total GPAT activity increases (two-fold) in keratinocytes cultured in high calcium medium versus that in low calcium control. Further analysis reveals that NEM-sensitive GPAT activity, which is encoded by GPAT-3, increases about three-fold, with no alteration in NEM-resistent activity, which is encoded by GPAT-1.Citation19 Together, these studies demonstrate that GPAT isoforms are differentially regulated during keratinocyte differentiation, with GPAT-3 expression increasing, leading to an increase in total GPAT activity, attributable to the GPAT-3-encoded NEM-sensitive GPAT activity.
PPAR-α, -δ, γ- and LXR-α, -β, are expressed in keratinocytes/epidermis and activation of these nuclear hormone receptors has numerous effects on keratinocyte function.Citation22 When keratinocytes are treated with PPAR and LXR activators, GPAT-3 gene expression increases significantly, with PPAR-γ > PPAR-δ > LXR > PPAR-α.Citation19 Activators of RXR also slightly increase GPAT-3 mRNA levels. In contrast, GPAT-1 and GPAT-4 mRNA levels are not altered by PPAR or LXR activators.Citation19 The increase in GPAT-3 gene expression by PPAR-γ or PPAR-δ activators occurs at the level of transcription since treatment of keratinocytes with either PPAR-γ or PPAR-δ activators does not alter GPAT-3 mRNA stability. However, PPAR activators increase the expression of GPAT-3 promoter constructs.Citation19 Finally, the increase in GPAT-3 gene expression by PPAR activators leads to a significant increase in microsomal GPAT activity (which is consistent with an increase in GPAT-3), resulting in an increase in glycerolipid synthesis.Citation19 Thus, PPAR or LXR activators specifically upregulate GPAT-3 expression but not other GPAT isoforms in cultured human keratinocytes.
Acute permeability barrier disruption by either tape-stripping or acetone treatment did not alter GPAT in mouse epidermis.
AGPATs.
The end product of GPAT action is lysophosphatidic acid, which is further acylated to phosphatidic acid by adding an acyl group to the sn-2 position of the glycerol backbone, catalyzed by AGPAT enzymes (). AGPAT is also known as lysophosphatidic acid acyltransferase. Like GPAT, multiple AGPAT isoforms have been identified in mammals, each encoded by a distinct gene.Citation13,Citation23,Citation24 These AGPAT isoforms display tissue-specific variation in expression and activity as well as different substrate preferences, suggesting tissue-specific functions. New AGPAT isoforms have been identified recently and the current understanding of the biological role of AGPATs is far from complete. Originally, five isoforms were found in mice and six in human, with mouse AGPAT-1-5 having validated enzyme activity.Citation25 Additionally, mouse AGPAT-2 has two-fold higher enzyme activity than AGPAT-3, -4 and -5 in an in vitro system by direct comparison.Citation24 Recently, AGPAT6-10 has been reported. Since AGPAT-6 has microsomal GPAT activity and is localized to the ER, it is now renamed as GPAT-4.Citation26 AGPAT-8, which is highly related to AGPAT-5, has been shown to have acyl-CoA: lysocardiolipin acyltransferase activityCitation27,Citation28 and AGPAT activity.Citation27 AGPAT-7 and AGPAT-9 are reported as members of the lysophosphatidylcholine acyltransferase family, with only weak AGPAT activity.Citation29–Citation31 Finally, AGPAT-10, which is identical in sequence to GPAT-3 and localized to the ER, has been reported to have both AGPATCitation32 and GPAT activity.Citation33
In mouse epidermis, AGPAT-3, -4 and -5 have relatively high constitutive expression levels, while AGPAT-1 and -2 have low constitutive expression levels.Citation23 Localization studies demonstrated that all five isoforms of AGPAT are expressed in all nucleated layers of mouse epidermis. Of note is in other tissues, including brain, heart, lung, liver, kidney and spleen, both AGPAT-1 and -2 are expressed at relatively high levels.Citation24 Similarly, all five AGPATs are expressed in cultured human keratinocytes. AGPAT-3, -4, and -5 are expressed at high constitutive levels while AGPAT-1 and -2 expression levels are relatively low.Citation23 Like GPATs, the physiological role of these different isoforms of AGPAT in keratinocytes/epidermis remains unclear.
The expression pattern of AGPATs during fetal rat skin barrier development has been determined. The mRNA levels for both AGPAT-2 and -5 increase progressively in the epidermis at days 17–22, peaking on day 19, while mRNA levels for AGPAT-1, -3 and -4 remain unchanged.Citation23 There is a parallel increase in the total AGPAT activity on day 19, which may be due to the increased expression of AGPAT-2 and -5.Citation23
Unlike GPAT3, which is induced during keratinocytes differentiation, AGPAT expression is not altered by exposure to high calcium treatment for 6–10 days. Additionally, treatment with PPAR or LXR activators also had a minimal effect on AGPAT expression.
Acute disruption of the permeability barrier by tape-stripping results in a rapid increase in the mRNA levels of AGPAT-1, -2 and -3.Citation23 AGPAT-5 mRNA also increases after tape-stripping but to a lesser extent. In contrast, the mRNA levels of AGPAT-4 remain unchanged.Citation23 These observations were verified by using another method (acetone) to disrupt the barrier, and similarly, the mRNA levels of AGPAT-1, -2, -3 and -5 increase by 1 hour after acetone treatment with no change in the mRNA levels of AGPAT-4.Citation23 In parallel with the increase in mRNA levels, there is a corresponding increase in total AGPAT enzyme activity in mouse epidermis following tape-stripping.Citation23 Thus, acute disruption of the permeability barrier stimulates an increase in both mRNA levels and enzyme activity of AGPATs in mouse epidermis. Since upregulation of AGPAT mRNAs after tape-stripping can be partially reversed by artifical restoration of the permeability barrier by occlusion with an impermeable membrane, it can be postulated that the increase in the expression of AGPAT is linked to permeability barrier requirements.Citation23
Lipins.
At the third step of triglyceride/phospholipid biosynthesis, phosphatidic acid, produced by the action of AGPAT enzymes, is dephosphorylated to form diacylglycerol for the synthesis of triglycerides and phospholipids (PC, PE and PS). This dephoshorylation process is catalyzed by phosphatidate phosphatase (PAP1), which is localized in the cytosol and transiently translocates to the ER membrane to encounter phosphatidic acid substrate for performing the phosphatase reaction. PAP1 enzymes are Mg2+-dependent, NEM sensitive and are responsible for most of PAP activity involved in the glycerol phosphate biosynthetic pathway.Citation13 (Of note PAP2 has lipid phosphate phosphatase activity that is Mg2+-independent, NEM resistent, localized to the plasma membrane and is not involved in triglyceride synthesis through glycerol phosphate pathway).Citation34 The PAP catalytic motif is present in the carboxyl-terminal lipin domain (C-LIP) of lipin proteins.Citation35,Citation36 This motif is critical for PAP function, as conversion of aspartate residue to glutamate in this motif completely abolishes PAP activity.Citation37 In addition, a naturally occurring glycine to arginine mutation in amino-terminal lipin domain (N-LIP) dramatically reduces PAP activity in mouse, indicating the importance of N-LIP domain as well.Citation38,Citation39 Finally, the PAP1 family is composed of three members, Lipin-1, -2 and -3.Citation13,Citation40,Citation41 In addition to its enzyme activity Lipin-1 serves as a transcriptional co-activator interacting with PPARα, PPARδ, PPARγ, hepatocyte nuclear factor 4α, and the glucocorticoid receptor to enhance transcriptional activity.Citation37 Human lipin-1 and mouse lipin-1, lipin-2 and lipin-3 all have PAP activity, which is specific for phosphatidic acid and no activity for other related lipid phosphate substrates, including LPA, sphingosine phosphate, ceramide-1-phosphate.Citation35,Citation36 Furthermore, while lipin genes are conserved across a broad range of eukaryotes, including vertebrates, fly, nematodes and yeast, lipin orthologs in all species have conserved regions of both C-LIP and N-LIP.Citation42 This evolutionary conservation of lipin proteins indicates the important role of lipin proteins in cellular function.
There is a distinct tissue distribution of the three lipin family members. Lipin-1 is mainly found in adipose tissue and muscle, whereas lipin-2 is expressed at high levels in liver, brain, and kidney, and lipin-3 is detected at low levels in several visceral tissues, including small intestine and liver.Citation36 In human keratinocytes under proliferating conditions (maintained in low calcium medium), lipin-1 expression is relatively high (CT: 23∼24), followed by lipin-3 (CT: 25∼27) and lipin-2 (CT: 27∼28) (). In mouse epidermis, however, lipin-3 expression is relatively abundant (CT: 25∼26), while lipin-1 and -2 expression levels are in similar ranges (CT: 28∼29). All three lipin mRNAs are localized to the upper epidermis, while lipin-2 mRNA is also found in the lower epidermis.
Acute permeability barrier disruption by tape stripping results in an increase in Lipin 1 mRNA levels as early as 1 hour after barrier disruption by tape stripping with an ∼5.5-fold increase at 3 hours, returning towards baseline levels at later times (). Similar changes occur in lipin-2 mRNA levels after permeability barrier disruption, albeit the magnitude of the increase is smaller (). In contrast, no alteration occurs in lipin-3 mRNA levels (). To confirm these observations, acetone treatment was employed to disrupt permability barrier function. As shown in , 3 hours after acetone treatment, both lipin-1 and -2 mRNA levels increase, with no change in lipin-3 mRNA levels. However, occlusion following permeability barrier disruption does not block the increases in lipin-1 and -2 expression, suggesting that the upregulation of lipin gene expression may be due to an injury reaction rather than being mediated by the loss of permeability barrier function.
Calcium induces differentiation, and treatment of keratinocytes with either high calcium, or PPAR/LXR activators has a limited effect on the expression of all three lipin isoforms in keratinocytes.
When skin structure and function were examined in the lipin-1 deficient mice,Citation43 neither epidermal morphology, surface pH, SC hydration, basal trans-epidermal water loss (a sensitive marker of permeability barrier function), or the recovery of permeability barrier function following acute barrier disruption were altered in the lipin-1 deficient mice compared to wild type controls (data not shown). Thus, epidermal structure and function is not abnormal in lipin-1 deficient mice, presumably due to compensation by lipin-2 and -3.
DGAT.
In the final step of triglyceride biosynthesis, diacylglycerol is converted to triglyceride, a reaction catalyzed by diacylglycerol acyltransferase (DGAT).Citation44 In mammals, there are two DGAT isoforms, DGAT-1 and DGAT-2. DGAT-1 has 6–12 putative trans-membrane domains and is a member of the mammalian acyl-CoA: cholesterol acyltransferase gene family, whereas DGAT-2 has two trans-membrane domains and is a member of a gene family that includes DGAT-2 and three monoacylglycerol acyltransferase isoforms.Citation11,Citation18 Both DGAT-1 and DGAT-2 recognize DAG and monoacylglycerol as substrates for triglyceride synthesis.Citation45,Citation46 However, they bear little sequence similarity and there are functional differences in their catalytic properties, subcellular localization, physiological function and regulation.Citation18,Citation45,Citation47,Citation48
Both DGAT-1 and -2 are widely expressed in mammalian tissues, including small intestine, liver, adipose tissue, mammary gland, skeletal muscle, heart, spleen and pancreas.Citation49,Citation50 In neonatal mouse skin, DGAT-2 is expressed at higher levels in the epidermis than dermis.Citation51 DGAT-1 is barely detectable in both epidermis and dermis of neonatal mice skin.Citation51 Using RT-PCR, DGAT-1 and 2 mRNA can be detected in adult mouse epidermis but DGAT-2 expression is much more robust than DGAT-1 (). The lower epidermis (basal layer) accounts for the majority of DGAT-2 expression (). While DGAT-1 is not highly expressed in adult mouse epidermis it is very abundantly expressed in sebaceous glands.Citation52
In cultured human keratinocytes the expression levels of DGAT-1 is as expected modest (). However, very surprisingly, in cultured human keratinocytes the expression level of DGAT-2 is very low (CT = 34–35) compared with that in mouse epidermis (CT = 23–25) (). That the low abundance of DGAT-2 mRNA in human keratinocytes is not a technical problem is shown by the ability to detect significant amounts (CT = 29–30) of DGAT-2 mRNA in Hep3G cells, a human liver cell line. Why the level of expression of DGAT-2 is different between human keratinocytes and mouse epidermis is unclear. It could be a species difference or perhaps due to the procedures used to grow human keratinocytes. It would be of interest to measure DGAT-2 mRNA in human epidermis.
Treatment of keratinocytes with either high calcium, or PPAR/LXR activators did not alter the expression of DGAT-1 or -2 in keratinocytes. Additionally, neither DGAT-1 nor DGAT-2 expression changed following acute barrier disruption by tape-stripping in hairless mouse epidermis, indicating that basal DGAT expression suffices to meet the permeability barrier requirement in adult mice.
The skin of Dgat2-deficient mice lacks elasticity and is shining, dry and cracked shortly after birth.Citation51 Dgat2-deficient mice rapidly loss weight from dehydration owing to impaired permeability barrier function and increased transepidermal water loss.Citation51 Structurally, light microscopy of Dgat2-/- skin reveals compact hyperkeratosis of affected stratum corneum, thinning of the epidermis and flattened of the dermo-epidermal interface owing to the effacement of the epidermal rete ridges/papillary projections.Citation51 Electron microscopy of Dgat2-/- skin further reveals a reduced number of lamellar membranes and lamellar bodies with decreased lipid content, reflecting abnormalities in the lamellar body secretory system.Citation51 A 96% reduction in triglyceride content is found in Dgat2-/- skin, with normal levels of other lipids.Citation51 Further analysis reveals a drastically reduction (>90%) of linoleic acid content in triglycerides and free fatty acids. Most interestingly, there is a marked reduction (>60%) in the content of acylceramide, a skin lipid containing linoleic acid that is critical for skin barrier maintenance.Citation53 Thus, the lack of DGAT2 results in a decrease in triglyceride synthesis leading to abnormal lamellar body formation and secretion, and ultimately impaired skin permability barrier function.
Furthermore, there are striking differences in the phenotype of DGAT-1 and DGAT-2 knock-out mice: Dgat2-deficient mice die shortly after birth, owing to severe abnormalities in both energy metabolism and skin permeability barrier function.Citation51 In contrast, Dgat1-deficient mice are viable and healthy, but have significant changes in lipid metabolism in several tissues including white adipose tissue and skin, particularly the sebaceous glands.Citation52 Dgat1-deficient mice developed dry fur and hair loss after puberty and exhibited impaired water repulsion and hypothermia due to the defective production of fur lipids, in particular type II wax diesters.Citation52 These changes are accounted for by abnormalities in sebaceous gland function. Dgat1-deficient mice do not have epidermal abnormalities and permeability barrier function is normal.
Conclusion
The biosynthesis of triglycerides and phospholipids requires multiple steps each of which are catalyzed by several different enzyme isoforms. Studies have shown that the epidermis expresses three GPAT isoforms, at least five AGPAT isoforms, three lipin isoforms, and two DGATs (in the mouse DGAT-2 is predominant with relatively low expression of DGAT-1). Why the epidermis expresses multiple isoforms is unclear but there are a number of possible explanations. First, having multiple different enzymes ensures that a mutation in a single enzyme is not likely to significantly compromise triglyceride or phospholipid synthesis, which is essential to form a functional permeability barrier. The exception to this is DGAT, where mice deficient in DGAT-2 die shortly after birth, in part due to an abnormality in permeability barrier function. Second, the epidermis is structurally very complex with undifferentiated proliferating cells in the basal layer and markedly differentiated cells in the outer layers. It is possible that expression of different isoforms differs for each cell type. Third, each isoform may localize to a different site within the cell. For example GPAT-1 is located in the mitochondria whereas GPAT-3 is located in the endoplasmic reticulum, which could result in different functional roles. Fourth, keratinocyte have a wide variety of fatty acids, including very long chain fatty acids, and it is possible that different isoforms have specificity for certain fatty acids. To utilize the diverse variety of fatty acids in keratinocytes may require different isoforms. Finally, the factors that regulate the expression or catalytic activity of different isoforms could differ allowing for a more precise regulation of triglyceride and phospholipid synthesis in keratinocytes. Future studies will need to address the roles of these various isoenzymes in epidermal metabolism.
Abbreviations
AGPAT | = | 1-acylglycerol-3-phosphate acyltransferase |
CL | = | cardiolipin |
DAG | = | diacylglycerol |
DGAT | = | diacylglycerol acyltransferase |
G-3-P | = | glycerol-3-phosphate |
GPAT | = | glycerol-3-phosphate acyltransferase |
LPA | = | lysophosphatidic acid |
NEM | = | N-ethylmaleimide |
PA | = | phosphatidic acid |
PAP | = | phosphatidate phosphatase |
PC | = | phosphatidylcholine |
PE | = | phosphatidylethanolamine |
PG | = | phosphatidylglycerol |
PI | = | phosphoinositol |
PS | = | phosphatidylserine |
TAG | = | triacylglyerol |
Figures and Tables
Figure 1 The glycerol phosphate pathway for de novo phospholipid/triacylglcerol synthesis in keratinocytes/epidermis.
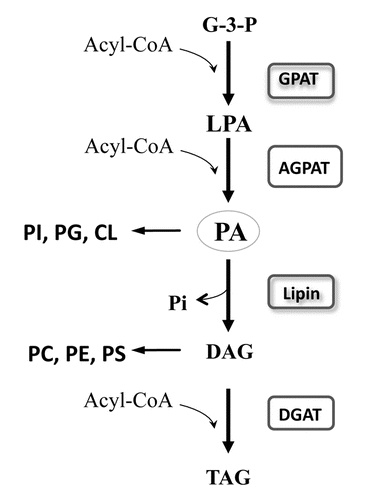
Figure 2 The time course of lipin gene expression in mouse epidermis after acute barrier disruption by tape stripping. Skin samples were collected from mice at 0, 1, 3, 6 and 24 hours after tape stripping and from control mice as described previously in reference Citation54. Epidermis was isolated and total RNA was prepared for real-time PCR analysis. Relative mRNA levels of lipin-1, -2 and -3 (36B4 as internal control) were determined by RT-PCR. Data are expressed as percentage of control (100%) for each time point and presented as mean ± SEM (n = 6). The experiment was repeated once using a different batch of mice with similar results. *p < 0.05; **p < 0.01.
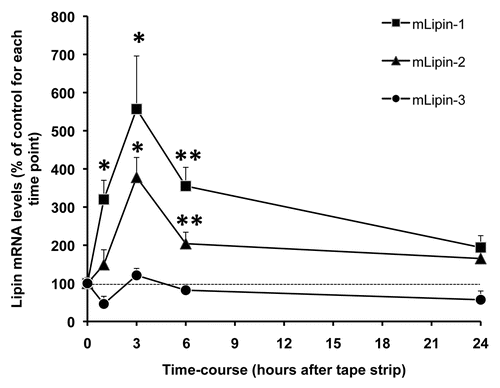
Figure 3 Lipin gene expression in mouse epidermis after acetone treatment. Mouse skin was treated with acetone (filled bar) or phosphate buffered saline (as control) (empty bar). Three hours after treatment, skin samples were collected and epidermis was prepared. Total RNA was prepared for real-time PCR analysis, and the relative mRNA levels of lipin-1, -2 and -3 (36B4 as internal control) were determined. Data are expressed as a percentage of phosphate buffered saline control (100%) and presented as mean ± SEM (n = 4). The experiment was repeated once using a different batch of mice with similar results. **p < 0.01, *p < 0.05.
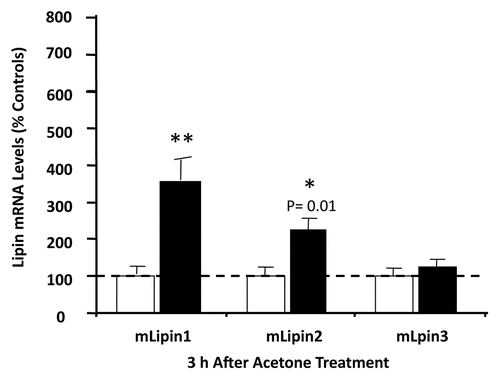
Figure 4 Localization of DGAT-2 in mouse epidermis. The upper and lower epidermis was prepared and the relative mRNA expression levels of DGAT-2 (36B4 as internal control) were determined. Results are expressed as a percentage of the upper epidermis (100%) and presented as mean ± SEM (n = 7). The experiment was repeated once using a different batch of mice with similar results. *p < 0.05.
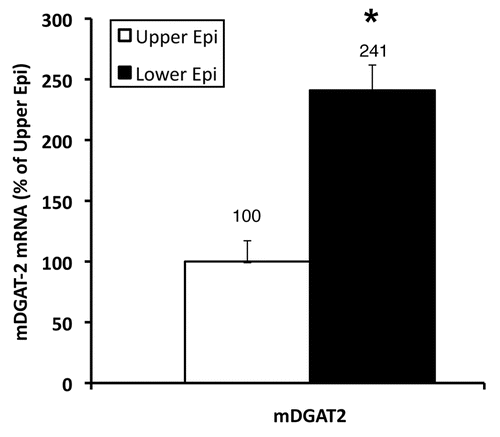
Table 1 CT values of GPAT, Lipin and DGAT isoforms in human keratinocytes and mouse epidermis.
Acknowledgements
The authors appreciate the excellent technical assistance of Peggy Kim, Sally Pennypacker, Judy Shigenaga and Art Moser.
References
- Feingold KR. Thematic review series: skin lipids. The role of epidermal lipids in cutaneous permeability barrier homeostasis. J Lipid Res 2007; 48:2531 - 2546
- Grayson S, Johnson-Winegar AG, Wintroub BU, Isseroff RR, Epstein E Jr, Elias PM. Lamellar body-enriched fractions from neonatal mice: preparative techniques and partial characterization. J Invest Dermatol 1985; 85:289 - 294
- Schurer NY, Elias PM. The biochemistry and function of stratum corneum lipids. Adv Lipid Res 1991; 24:27 - 56
- Holleran WM, Takagi Y, Uchida Y. Epidermal sphingolipids: metabolism, function and roles in skin disorders. FEBS Lett 2006; 580:5456 - 5466
- Wertz PW. Feingold PEK. Biochemistry of human stratum corneum. Skin Barrier 2006; New York Taylor & Francis 33 - 42
- Elias PM, Brown BE. The mammalian cutaneous permeability barrier: defective barrier function is essential fatty acid deficiency correlates with abnormal intercellular lipid deposition. Lab Invest 1978; 39:574 - 583
- Hansen HS, Jensen B. Essential function of linoleic acid esterified in acylglucosylceramide and acylceramide in maintaining the epidermal water permeability barrier. Evidence from feeding studies with oleate, linoleate, arachidonate, columbinate and alpha-linolenate. Biochim Biophys Acta 1985; 834:357 - 363
- Melton JL, Wertz PW, Swartzendruber DC, Downing DT. Effects of essential fatty acid deficiency on epidermal O-acylsphingolipids and transepidermal water loss in young pigs. Biochim Biophys Acta 1987; 921:191 - 197
- Wertz PW, Cho ES, Downing DT. Effect of essential fatty acid deficiency on the epidermal sphingolipids of the rat. Biochim Biophys Acta 1983; 753:350 - 355
- Radner FP, Streith IE, Schoiswohl G, Schweiger M, Kumari M, Eichmann TO, et al. Growth retardation, impaired triacylglycerol catabolism, hepatic steatosis and lethal skin barrier defect in mice lacking comparative gene identification-58 (CGI-58). J Biol Chem 2010; 285:7300 - 7311
- Shi Y, Cheng D. Beyond triglyceride synthesis: the dynamic functional roles of MGAT and DGAT enzymes in energy metabolism. Am J Physiol 2009; 297:10 - 18
- Wendel AA, Lewin TM, Coleman RA. Glycerol-3-phosphate acyltransferases: rate limiting enzymes of triacylglycerol biosynthesis. Biochim Biophys Acta 2009; 1791:501 - 506
- Takeuchi K, Reue K. Biochemistry, physiology and genetics of GPAT, AGPAT and lipin enzymes in triglyceride synthesis. Am J Physiol 2009; 296:1195 - 1209
- Gimeno RE, Cao J. Thematic review series: glycerolipids. Mammalian glycerol-3-phosphate acyltransferases: new genes for an old activity. J Lipid Res 2008; 49:2079 - 2088
- Gonzalez-Baro MR, Lewin TM, Coleman RA. Regulation of Triglyceride Metabolism II. Function of mitochondrial GPAT1 in the regulation of triacylglycerol biosynthesis and insulin action. Am J Physiol Gastrointest Liver Physiol 2007; 292:1195 - 1199
- Shindou H, Hishikawa D, Harayama T, Yuki K, Shimizu T. Recent progress on acyl CoA: lysophospholipid acyltransferase research. J Lipid Res 2009; 50:46 - 51
- Turkish A, Sturley SL. Regulation of triglyceride metabolism. I. Eukaryotic neutral lipid synthesis: “Many ways to skin ACAT or a DGAT”. Am J Physiol Gastrointest Liver Physiol 2007; 292:953 - 957
- Yen CL, Stone SJ, Koliwad S, Harris C, Farese R Jr. Thematic review series: glycerolipids. DGAT enzymes and triacylglycerol biosynthesis. J Lipid Res 2008; 49:2283 - 2301
- Lu B, Jiang YJ, Kim P, Moser A, Elias PM, Grunfeld C, Feingold KR. Expression and regulation of GPAT isoforms in cultured human keratinocytes and rodent epidermis. J Lipid Res 2010; 51:3207 - 3216
- Hurt CM, Hanley K, Williams ML, Feingold KR. Cutaneous lipid synthesis during late fetal development in the rat. Arch Dermatol Res 1995; 287:754 - 760
- Aszterbaum M, Menon GK, Feingold KR, Williams ML. Ontogeny of the epidermal barrier to water loss in the rat: correlation of function with stratum corneum structure and lipid content. Pediatr Res 1992; 31:308 - 317
- Schmuth M, Jiang YJ, Dubrac S, Elias PM, Feingold KR. Thematic review series: skin lipids. Peroxisome proliferator-activated receptors and liver X receptors in epidermal biology. J Lipid Res 2008; 49:499 - 509
- Lu B, Jiang YJ, Man MQ, Brown B, Elias PM, Feingold KR. Expression and regulation of 1-acyl-sn-glycerol-3-phosphate acyltransferases in the epidermis. J Lipid Res 2005; 46:2448 - 2457
- Lu B, Jiang YJ, Zhou Y, Xu FY, Hatch GM, Choy PC. Cloning and characterization of murine 1-acyl-sn-glycerol-3-phosphate acyltransferases and their regulation by PPARalpha in murine heart. Biochem J 2005; 385:469 - 477
- Leung DW. The structure and functions of human lysophosphatidic acid acyltransferases. Front Biosci 2001; 6:944 - 953
- Chen YQ, Kuo MS, Li S, Bui HH, Peake DA, Sanders PE, et al. AGPAT6 is a novel microsomal glycerol-3-phosphate acyltransferase. J Biol Chem 2008; 283:10048 - 10057
- Agarwal AK, Barnes RI, Garg A. Functional characterization of human 1-acylglycerol-3-phosphate acyltransferase isoform 8: cloning, tissue distribution, gene structure and enzymatic activity. Arch Biochem Biophys 2006; 449:64 - 76
- Cao J, Liu Y, Lockwood J, Burn P, Shi Y. A novel cardiolipin-remodeling pathway revealed by a gene encoding an endoplasmic reticulum-associated acyl-CoA:lysocardiolipin acyltransferase (ALCAT1) in mouse. J Biol Chem 2004; 279:31727 - 31734
- Agarwal AK, Sukumaran S, Bartz R, Barnes RI, Garg A. Functional characterization of human 1-acylglycerol-3-phosphate-O-acyltransferase isoform 9: cloning, tissue distribution, gene structure and enzymatic activity. J Endocrinol 2007; 193:445 - 457
- Soupene E, Fyrst H, Kuypers FA. Mammalian acyl-CoA:lysophosphatidylcholine acyltransferase enzymes. Proc Natl Acad Sci USA 2008; 105:88 - 93
- Ye GM, Chen C, Huang S, Han DD, Guo JH, Wan B, Yu L. Cloning and characterization a novel human 1-acyl-sn-glycerol-3-phosphate acyltransferase gene AGPAT7. DNA Seq 2005; 16:386 - 390
- Sukumaran S, Barnes RI, Garg A, Agarwal AK. Functional characterization of the human 1-acylglycerol-3-phosphate-O-acyltransferase isoform 10/glycerol-3-phosphate acyltransferase isoform 3. J Mol Endocrinol 2009; 42:469 - 478
- Cao J, Li JL, Li D, Tobin JF, Gimeno RE. Molecular identification of microsomal acyl-CoA:glycerol-3-phosphate acyltransferase, a key enzyme in de novo triacylglycerol synthesis. Proc Natl Acad Sci USA 2006; 103:19695 - 19700
- Brindley DN. Lipid phosphate phosphatases and related proteins: signaling functions in development, cell division and cancer. J Cell Biochem 2004; 92:900 - 912
- Han GS, Wu WI, Carman GM. The Saccharomyces cerevisiae Lipin homolog is a Mg2+-dependent phosphatidate phosphatase enzyme. J Biol Chem 2006; 281:9210 - 9218
- Donkor J, Sariahmetoglu M, Dewald J, Brindley DN, Reue K. Three mammalian lipins act as phosphatidate phosphatases with distinct tissue expression patterns. J Biol Chem 2007; 282:3450 - 3457
- Finck BN, Gropler MC, Chen Z, Leone TC, Croce MA, Harris TE, et al. Lipin 1 is an inducible amplifier of the hepatic PGC-1alpha/PPARalpha regulatory pathway. Cell Metab 2006; 4:199 - 210
- Han GS, Siniossoglou S, Carman GM. The cellular functions of the yeast lipin homolog PAH1p are dependent on its phosphatidate phosphatase activity. J Biol Chem 2007; 282:37026 - 37035
- Harris TE, Huffman TA, Chi A, Shabanowitz J, Hunt DF, Kumar A, et al. Insulin controls subcellular localization and multisite phosphorylation of the phosphatidic acid phosphatase, lipin 1. J Biol Chem 2007; 282:277 - 286
- Carman GM, Han GS. Phosphatidic acid phosphatase, a key enzyme in the regulation of lipid synthesis. J Biol Chem 2009; 284:2593 - 2597
- Carman GM, Han GS. Roles of phosphatidate phosphatase enzymes in lipid metabolism. Trends Biochem Sci 2006; 31:694 - 699
- Peterfy M, Phan J, Xu P, Reue K. Lipodystrophy in the fld mouse results from mutation of a new gene encoding a nuclear protein, lipin. Nat Genet 2001; 27:121 - 124
- Phan J, Reue K. Lipin, a lipodystrophy and obesity gene. Cell Metab 2005; 1:73 - 83
- Coleman RA, Lee DP. Enzymes of triacylglycerol synthesis and their regulation. Prog Lipid Res 2004; 43:134 - 176
- Cheng D, Iqbal J, Devenny J, Chu CH, Chen L, Dong J, et al. Acylation of acylglycerols by acyl coenzyme A:diacylglycerol acyltransferase 1 (DGAT1). Functional importance of DGAT1 in the intestinal fat absorption. J Biol Chem 2008; 283:29802 - 29811
- Yen CL, Monetti M, Burri BJ, Farese R Jr. The triacylglycerol synthesis enzyme DGAT1 also catalyzes the synthesis of diacylglycerols, waxes and retinyl esters. J Lipid Res 2005; 46:1502 - 1511
- Stone SJ, Levin MC, Zhou P, Han J, Walther TC, Farese R Jr. The endoplasmic reticulum enzyme DGAT2 is found in mitochondria-associated membranes and has a mitochondrial targeting signal that promotes its association with mitochondria. J Biol Chem 2009; 284:5352 - 5361
- Meegalla RL, Billheimer JT, Cheng D. Concerted elevation of acyl-coenzyme A:diacylglycerol acyltransferase (DGAT) activity through independent stimulation of mRNA expression of DGAT1 and DGAT2 by carbohydrate and insulin. Biochem Biophys Res Commun 2002; 298:317 - 323
- Cases S, Smith SJ, Zheng YW, Myers HM, Lear SR, Sande E, et al. Identification of a gene encoding an acyl CoA:diacylglycerol acyltransferase, a key enzyme in triacylglycerol synthesis. Proc Natl Acad Sci USA 1998; 95:13018 - 13023
- Cases S, Stone SJ, Zhou P, Yen E, Tow B, Lardizabal KD, et al. Cloning of DGAT2, a second mammalian diacylglycerol acyltransferase, and related family members. J Biol Chem 2001; 276:38870 - 38876
- Stone SJ, Myers HM, Watkins SM, Brown BE, Feingold KR, Elias PM, et al. Lipopenia and skin barrier abnormalities in DGAT2-deficient mice. J Biol Chem 2004; 279:11767 - 11776
- Chen HC, Smith SJ, Tow B, Elias PM, Farese R Jr. Leptin modulates the effects of acyl CoA:diacylglycerol acyltransferase deficiency on murine fur and sebaceous glands. J Clin Invest 2002; 109:175 - 181
- Uchida Y, Holleran WM. Omega-O-acylceramide, a lipid essential for mammalian survival. J Dermatol Sci 2008; 51:77 - 87
- Jackson SM, Wood LC, Lauer S, Taylor JM, Cooper AD, Elias PM, et al. Effect of cutaneous permeability barrier disruption on HMG-CoA reductase, LDL receptor and apolipoprotein E mRNA levels in the epidermis of hairless mice. J Lipid Res 1992; 33:1307 - 1314