Abstract
The Defense Threat Reduction Agency’s Joint Science and Technology Office manages the Chemical and Biological Defense Program’s Science and Technology portfolio. The Joint Science and Technology Office’s mission is to invest in transformational ideas, innovative people and actionable technology development for Chemical and Biological Defense solutions, with the primary goal to deliver Science and Technology products and capabilities to the warfighter and civilian population that outpace the threat. This commentary focuses on one thrust area within this mission: the Vaccine program of the Joint Science and Technology Office’s Translational Medical Division. Here, we will describe candidate vaccines currently in the S&T pipeline, enabling technologies that should facilitate advanced development of these candidates into FDA licensed vaccines, and how the ever-changing biological threat landscape impacts the future of biodefense vaccines.
Joint Science and Technology Office Overview
The Defense Threat Reduction Agency’s (DTRA) Joint Science and Technology Office for Chemical and Biological Defense (JSTO-CBD), established in 2003, integrates and manages the Science and Technology (S&T) portfolio of the Chemical and Biological Defense Program (CBDP). The mission of the CBDP is to ensure that the US. Military has the capability to operate effectively and decisively in the face of chemical or biological warfare threats at home or abroad. To this end, JSTO’s Translational Medical Division (CBM) invests in science and technology aimed at generating candidate medical countermeasures (MCMs) that -following advanced development into licensed products- can be used to protect and sustain the Warfighter against biological threats. Within the critical path of vaccine research and development, JSTO investments span from early discovery through IND enabling studies, and in some cases early clinical (phase I) development. JSTO transitions candidate MCMs to the Joint Program Executive Office (JPEO) for Chemical and Biological Defense, which as the advanced developer, is responsible for progressing the candidate MCMs through the regulatory path and obtaining FDA approval (). JSTO and JPEO investments are driven by requirements (). The Joint Requirements Office (JRO) is responsible for coordinating and integrating requirements and capability needs identified via input from the Services, the Joint Staff-led Capabilities Based Assessments and Combatant Commands for the CBDP (). Although highlights the role of JSTO within a requirements-driven “technological pull,” it is important to note that parts of the JSTO portfolio are dedicated to an internal “technological push” with a goal of providing revolutionary Warfighter capabilities in the long-term.
Figure 1. Depiction of JSTO role within the CBDP. JSTO invests in science and technology to address an internal technological push, and the requirements-driven pull from the JRO. Mature technologies are provided to the JPEO for advanced development. JPEO provides bio-defense capabilities to the Warfighter to meet the requirements that have been communicated to the JRO.
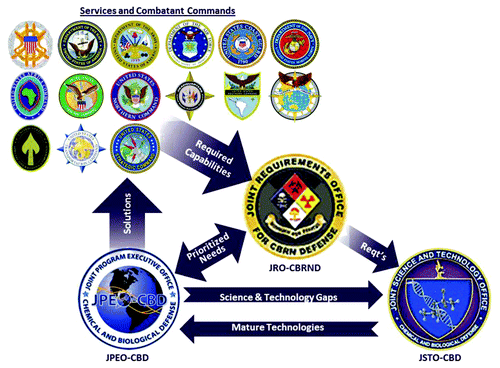
JSTOs Vaccine Strategy
To accelerate the delivery of candidate vaccines which defeat biological threats to human health, JSTO has adopted translational product and research principles as the foundation of its S&T efforts. This includes utilizing translational teams between the S&T base and advanced developer in the management of the discovery, development, manufacturing, acquisition and fielding phases of the S&T programs. Exploiting translational research and development principles and integrated team structures enables the earlier identification and removal of programs that are unlikely to succeed, the amplification of successful programs, and thus increases the efficiency of candidates transitioning from discovery to advanced development.
The potential for exposure of DoD personnel to not only known, but also new or emerging biological threats also demands that our investment strategy for vaccines embraces agility and adaptability. This requires an approach that exploits innovative platforms that can be easily modified to create vaccines as new threats are identified. In addition, rapid threat identification, antigen/target selection and lead candidate development must be strategically integrated. Moreover, a paradigm shift is required to enable the rapid, reliable and cost-effective sustainable access to biodefense vaccines and other countermeasures in sufficient quantities to treat affected populations. Past models involving the construction and operation of facilities dedicated to the production of a single vaccine product utilizing traditional expression technologies, such as prokaryotic and egg-based platforms, are not sustainable for biodefense, and thus an alternative approach is needed. To this end, and in response to key strategic direction from The White House through Homeland Security Presidential Directives, Executive Orders and other correspondences, the DoD initiated the Medical Countermeasure Initiative (MCMI). The goal of the MCMI is to provide the innovative S&T base and critical flexible and agile biomanufacturing capability required to ensure a rapid response to multiple and/or changing threats for both the military and civilian populations. JSTO is responsible for the S&T mission of the MCMI, and is thus establishing new investments in vaccine prototypes that leverage adaptable antigen expression platforms and flexible and agile biomanufacturing technologies that utilize scalable, single-use/disposable systems. Borrowing from enabling investments in flexible manufacturing, JSTO is seeking to enhance the CBDP’s ability to adaptively manufacture countermeasures through new expression vehicles such as plants, cells and de novo synthetic platforms. Methods to demonstrate and develop such platforms toward new capabilities that can integrate into a multi-vaccine product advanced development facility and large-scale production are being explored.
The need to create flexible and adaptive countermeasure strategies requires development of new regulatory paradigms that go beyond the “Animal Rule.” Thus, JSTO is investing in regulatory sciences and technologies to increase the likelihood of success and facilitate the advancement of MCMs for biodefense along the regulatory path. These investments include advanced animal models for threat agent diseases and validation of biomarkers, ex vivo technologies to predict/determine human safety and efficacy of MCMs (discussed below), as well as downstream processing methods and analytical tools to provide novel methods of assessment.
Basic Research
Basic research is essential to guide the rationale design of efficacious vaccines for biodefense. JSTO investments seek to tease out molecular mechanisms of pathogenesis of priority biothreats and to better understand the interaction of these pathogens with the host, in order to identify potential targets for exploitation as immunogens or therapeutic intervention. Our insufficient understanding of the basic biology of biodefense pathogens has hindered the development of successful vaccines, which is exemplified in attempts to develop countermeasures against Burkholderia species. B. mallei and B. pseudomallei are able to evade the host immune response,Citation1,Citation2 leading to chronic infections that can resurge in many casesCitation3 and making vaccine design a difficult challenge. Elucidating the mechanisms by which Burkholderia species evade an adaptive immune response is necessary to identify protective antigens for the design of efficacious vaccines as well as immune correlates. The capsular polysaccharide and lipopolysaccharide of B. mallei and B. pseudomallei were shown to induce robust antibody responses in animal models and clinical samples, with some evidence of protection associated with this response.Citation4 Recent work identified secretion system-related virulence factors such as Hcp1, BopE and BimA that are involved in evading the innate immune response,Citation5,Citation6 and suggested that TssM modulates the adaptive immune response.Citation1 Additionally, cellular immunity including TNF-α and IFN-γ responses correlated with protection in animal models.Citation7 Together, these findings will impact the choice of antigens and adjuvants, as well as the vaccine platform itself.
Ex vivo Mimetics
Human clinical trials to determine medical countermeasure efficacy against the majority of high priority biothreats cannot be conducted because there are too few cases, the occurrence is too sporadic, and the severity of the disease is too great. Instead, licensure in the United States for these MCMs will only be possible using the “Animal Rule” of the FDA, which allows for a demonstration of efficacy in one or more well characterized animal models which are considered relevant to the disease in humans. Thus, JSTO is investing in the development of animal models for many of the top biothreats. The challenge lies in developing models that truly recapitulate human disease. Again, insufficient understanding of biothreat disease pathogenesis in humans, immune correlates, strain selection and natural history studies make identifying the appropriate animal model difficult. Moreover, the threat of biological weapons for the military would likely be in the form of an aerosol, and aerosol transmission is not thought to be a major factor in natural outbreaks involving the majority of biothreats.
Prior to first in human clinical trials, studies to determine pre-clinical MCM safety and efficacy are lengthy and rely heavily on in vitro and animal models that frequently have a limited ability to extrapolate to human safety, efficacy and tolerability. JSTO is thus exploiting advances in technologies utilizing primary human cells organized in a physiological relevant manner to develop more reliable ex vivo models that could better predict the safety, efficacy as well as pharmacodynamics in humans prior to entry into phase I clinical studies.
Three-dimensional, organotypic in vitro constructs are being investigated as tools to reasonably reproduce the human condition.Citation8,Citation9 Surrogate human immune systems such as the Modular IMmune In vitro Construct (MIMICTM) provide a three-dimensional tissue model that is intended to reliably reproduce the human humoral and cell-mediated immune responses.Citation10 The MIMICTM platform consists of three different modules: the Peripheral Tissue Equivalent, the Lymphoid Tissue Equivalent and a Functional Assay or Disease Module. Combined, these modules measure the effect of a test antigen (protein or infectious/killed pathogen) on the induction of innate and adaptive immune responses. Recent studies in which human donors also received the 2009–2010 seasonal influenza vaccine showed that HAI titers increased both on the MIMICTM platform and in sera samples, with similar patterns following vaccination with the seasonal influenza vaccination.Citation10 Thus, these technologies can streamline the discovery stage of vaccines and provide additional validation of results obtained in animal models.
The respiratory mucosa is a critical exposure portal for natural and bioengineered pathogens and an attractive drug delivery route. Ex vivo models of the mucosa provide novel technologies for studying mucosal immunology. For example, an improved in vitro three-dimensional (3-D) Fluidic-Enhanced Airway Model is being pursued that reproduces not only epithelial function, but also encompasses the integrated epithelial-interstitial-microvasculature structure of the human respiratory air-blood barrier. This model will employ primary human lung cells in a self-contained engineered fluidic chamber enabling independent control and access to the three cell types (i.e., bronchial epithelial cells, fibroblasts and microvascular endothelial cells). These respiratory models offer a tool to study the impact of biological threats, and vaccines against them, at a likely site of exposure.
In vitro platforms of human organ constructs that could accurately predict human safety, efficacy and pharmacokinetics of candidate medical countermeasures are a long-term prospect for ex vivo technologies. The ultimate goal of this research would be to reduce the overall burden of in vivo testing in the development of products for human use. A platform comprised of in vitro human organ constructs in communication with each other (i.e., liver, lung, heart, kidney, vasculature and blood brain barrier with neuronal component) could accurately assess efficacy, toxicity and pharmacokinetics of drugs in a way that is relevant to humans. Development of a platform that is both reliable and accessible to the scientific community at a reasonable cost would greatly facilitate medical countermeasure development.
Vaccines against Viral Threats
Viral hemorrhagic fevers caused by filoviruses (i.e., Ebola Zaire, Ebola Sudan and Marburg strains) and viral encephalitis caused by alphaviruses (i.e., VEE, EEE and WEE) pose two major challenges to the biodefense community (). Although the required immunogens to develop a protective immune response to both filoviruses and alphaviruses have been identified and incorporated into multiple distinct vaccine candidates that were shown to provide protection to animals, the advancement of a filovirus vaccine or an alphavirus vaccine toward licensure has been slow. Again, the ‘stumbling block’ lies in the development and validation of appropriate animal models and identification of immune correlates of protection necessary for advancement and licensure.
Figure 2. Current snapshot of Biodefense vaccines. Current vaccine candidates against the filoviruses and alphaviruses are undergoing pre-clinical testing. Vaccines against ricin toxin and anthrax have undergone phase I clinical safety testing. Antigen and platform discovery is being pursued for tularemia, melioidosis and glanders vaccines.
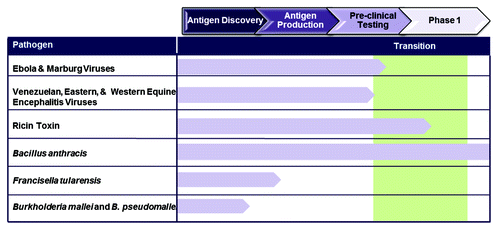
Over seven distinct vaccine platforms have been characterized that confer protection to nonhuman primates (NHPs) from Ebola (Ebola Zaire and Ebola Sudan) and Marburg (all Marburg strains tested) challenge. Each platform is based on generating immune responses against the filovirus glycoprotein, GP, with the aim of eliciting protective neutralizing antibodies. The leading viral replicon-based vaccine directed against filoviruses is based on an alphavirus replicon vector technology derived from an attenuated strain of Venezuelan equine encephalitis (VEE) virus. The attenuation is derived from site-directed mutagenesis of envelope glycoproteins E1 and E2.Citation11 This attenuated variant of VEE does not cause illness or significant viremia in animals, but does induce protective immunity against subsequent virulent VEE challenge in mice, horses and primates.Citation11 Foreign genes can be inserted in place of the VEE structural protein gene region in the cDNA plasmid, resulting in expression of the heterologous genes. In the case of filovirus vaccines, the self-amplifying replicon RNA directs the synthesis of large amounts filovirus-derived GP. This results in a GP-specific immune response and protection against an aerosol challenge with filoviruses.Citation12 The VRP expressing Marburg GP was shown to provide cross-protection against all members of the Marburgvirus family (Ci67, Musoke and RAVN), while the VRP expressing GP derived from Ebolaviruses (Zaire or Sudan) does not appear to protect against heterologous challenge of Ebolaviruses.Citation12 Additional work toward dose optimization, adjuvant formulation and manufacturing process optimization may further improve the efficacy and cross-reactivity of these vaccine constructs.
Virus-like particles (VLPs) expressing three filovirus antigens: GP, a matrix protein (VP40) and a nucleoprotein (NP); also achieved promising results. Filovirus VLPs potently stimulated functional maturation and activation of dendritic cells in vitro, which is likely responsible for activation of both humoral and cellular immune responses.Citation13 Similar to the viral replicon, additional efforts in optimizing the formulation of a VLP-based vaccine may shed light on strategies to induce effective cross-protection. The final product may be a tri-component vaccine consisting of separate VLPs for Marburg virus, Ebola Virus Zaire and Ebola Virus Sudan, but could be flexible to incorporate emerging strains as well. Both the VRP-based and VLP-based filovirus vaccines are in the early stages of advanced development, with the ultimate goal of providing a single vaccine that protects against all members of the Filoviridae family.
Vaccines against the encephalitic alphaviruses (Venezuelan, Eastern and Western Equine Encephalitis Viruses) also remain an emphasis of the Biodefense community. Similar to filoviruses, efforts are targeting a single vaccine with multivalent protective efficacy against all three species. There are current investments in a small range of candidate vaccines that have demonstrated efficacy in non-human primate studies (unpublished data),Citation14,Citation15 as well as the initiation of back-up candidates should more mature technologies prove to be unsuccessful.
Some of the leading candidates include DNA-based vaccines, virus-like particles, viral replicons and inactivated viruses. The DNA-based vaccine encodes the E1 and E2 envelope proteins of Venezuelan Equine Encephalitis Virus and is administered via intramuscular electroporation.Citation14 This vaccine induced robust neutralizing antibody titers and eliminated viremia in a cynomolgous macaque infection model. Preliminary data with a trivalent vaccine preparation suggested effective multivalent protection as well.Citation14 Similarly, efficacy was observed and associated with neutralizing antibody titers for the virus-like particle, viral replicon and inactivated virus candidates (unpublished data).Citation16 It is important to note that although the current dogma with alphavirus immunity emphasizes neutralizing antibody titers as a correlate of protection, the impact of T cell responses on the course of infection is less clear. In this light, a more thoroughly defined non-human primate model of infection, and the elucidation of other key immune correlates have potential to facilitate vaccine discovery and evaluation.
Vaccines against Bacterial and Toxin Threats
Vaccines effective against bacterial and toxin threats remain elusive (). The natural prevalence of disease or toxicity associated with these agents is either very low, or non-existent, making approval via the animal rule a necessity in many cases. This also has hindered the ability to use clinical cases to identify the most promising protective antigens for some organisms. However, advances in bioinformatics, in vitro technologies and animal models will continue to facilitate the discovery and development of effective vaccines.
Beans of the castor plant are the source of ricin toxin, a highly potent A-B toxin against which vaccines are currently being developed. This includes an E. coli-produced recombinant A chain of ricin toxin (RV EcTM), comprised of amino acids 1–33 and 44–198 of the A chain and adjuvanted with alhydrogel.Citation17 Both this candidate and RiVax have demonstrated protective efficacy in animal models of ricin toxicityCitation18 and are currently in phase I clinical trials. Similarly, the protein toxin based vaccines against anthrax are fairly mature, with recombinant protective antigen (PA)-based vaccines currently in clinical trials.Citation19 However, PA-independent vaccines are also being pursued as a means to mitigate the potential risk of escape variants.
Tularemia, melioidosis and glanders vaccines also comprise major Biodefense vaccine efforts, but are at earlier stages in the development pipeline. For Francisella tularensis, the discovery of protective antigens and adaptation to various delivery platforms remain to be key focuses given regulatory issues with the live vaccine strain (LVS). One such platform, a Listeria monocytogenes-based approach, showed promising efficacy in a rat model of inhalational tularemia.Citation20 The backbone consists of an ActA-deficient strain of L. monocytogenes. ActA is critical for intracellular movement, and intercellular spread of L. monocytogenes, and strains deficient in this protein are cleared from the body within about seven days.Citation21 This strain was engineered to express F. tularensis IglC, which is immunogenic and is essential for survival within macrophages.Citation22 At this stage of maturity, this and other platforms would still benefit from the discovery and validation of alternative protective antigens in the pursuit of a tularemia vaccine.
As noted above, vaccines against B. mallei and B. pseudomallei, the causative agents of glanders and melioidosis, are being pursued but remain a major challenge. In addition to the issues associated with mechanisms of immune evasion, vaccines against Burkholderia species also face two major difficulties; broad efficacy across all strains and selection of the most relevant animal model(s). B. pseudomallei is an incredibly plastic organism in terms of its genome, undergoing genomic recombination at levels much higher than most bacterial pathogens.Citation23 The diversity of pathogenicity islands across a range of B. pseudomallei isolates could hinder the ability to develop a protein-based vaccine for melioidosis. The lipopolysaccharide and capsular polysaccharide of Burkholderia species was shown to provide protection,Citation4 but would likely require formulation with, or conjugation to, other protein antigens in order to induce a robust T cell response. Animal model selection is critical given that licensure will ultimately require approval via the animal rule. The Balb/c and C57BL/6 murine models are considered to be reasonable small animal models of acute and chronic Burkholderia infection,Citation24,Citation25 however, which is more representative of the human condition for initial vaccine testing remains unclear. Several large animal models are currently under investigation to include goats and multiple non-human primate species,Citation26,Citation27 and continued investments in animal models to select that which most closely replicates the human condition is critical.
Future of Biodefense Vaccines
Challenge of evolving biological threats
The future of biological defense vaccines is inevitably tied to the evolving threat. The biological threat has changed dramatically since the passing of the Biological and Toxins Weapons Convention went into force in 1975 as a result of asymmetrical warfare, emerging infectious diseases and advances in biotechnology. The adversary has changed; the focus has shifted in large part from state-run biological weapons programs to biological terrorism. This in itself impacts the breadth of biological agents for which we need medical countermeasures.
An increasing awareness of emerging infectious diseases also continues to shape our picture of the biological threat. For example, since the discovery of Ebola virus in 1976, four other Ebola species have been identifiedCitation28 which has impacted the experimental design when seeking a “pan-Ebola virus” vaccine. As biosurveillance efforts continue to expand globally and encompass both clinical and agricultural studies, newly emerging threats will inevitably be found. The recent discovery of a novel rhabdovirus, Bas-Congo virus, as the etiological agent of a 2009 viral hemorrhagic fever outbreak in the Democratic Republic of the CongoCitation29 is a prime example of how the combination of increasing surveillance with technological advances will likely increase the rate at which new pathogens are discovered.
While technological advances increase our ability to detect and diagnose new pathogens, they also bring the potential for new threats. Genetic engineering is common practice in microbiology labs world-wide to tease out biological functions. Combined with an increasing understanding of how given biological functions and networks impact a system as a whole, the creation of synthetic viruses and bacteria has become a reality.Citation30,Citation31 The 2010 Presidential Commission for the Study of Bioethical Issues concluded that synthetic biology was not a threat at the time.Citation32 However, the field of synthetic biology will need to be monitored for advances that could lead to new threats, or perhaps more importantly, new opportunities for biological defense capabilities.
Streamlining vaccine development
The development of vaccines against traditional biological threats for which the CBDP needs vaccines remains a challenge. The evolving threat landscape further complicates this scenario, providing a moving target that necessitates the development/utilization of adaptable, ‘plug-and-play’ vaccine platforms exploiting flexible and agile manufacturing technologies. Negotiating the critical path for biodefense vaccine development in a timely manner requires the implementation of translational medicine principals aimed at product delivery. Advancements in regulatory sciences and technologies are needed to increase the likelihood of success and facilitate the progression of MCMs for biodefense along the regulatory path. Novel in vitro assays and ex vivo human mimetics (as well as in silico approaches) for the rapid screening of vaccine candidates should enable the ability to better predict the efficacy, potential toxicity and immune response in humans prior to entry into clinical trials. Investments aimed at understanding the molecular mechanisms of pathogenesis and protective immunity in humans for both traditional and emerging threats will continue to be required to facilitate not only vaccine candidate discovery but subsequent advanced development. JSTO will continue to combine technological advances with an understanding of biological threats to provide timely and effective vaccine candidates for the Warfighter.
Abbreviations: | ||
JSTO | = | Joint Science and Technology Office |
DTRA | = | Defense Threat Reduction Agency |
CBDP | = | Chemical and Biological Defense Program |
JRO | = | Joint Requirements Office |
JPEO | = | Joint Program Executive Office |
MIMIC® | = | Modular Immune in vitro Construct |
Acknowledgments
This manuscript represents the opinion of the authors, and not necessarily that of the Defense Threat Reduction Agency and the Joint Science and Technology Office. The manuscript has been approved for public release (PA-13–034).
Disclosure of Potential Conflicts of Interest
No potential conflicts of interest were disclosed.
References
- Tan KS, Chen Y, Lim YC, Tan GY, Liu Y, Lim YT, et al. Suppression of host innate immune response by Burkholderia pseudomallei through the virulence factor TssM. J Immunol 2010; 184:5160 - 71; http://dx.doi.org/10.4049/jimmunol.0902663; PMID: 20335533
- Galyov EE, Brett PJ, DeShazer D. Molecular insights into Burkholderia pseudomallei and Burkholderia mallei pathogenesis. Annu Rev Microbiol 2010; 64:495 - 517; http://dx.doi.org/10.1146/annurev.micro.112408.134030; PMID: 20528691
- Peacock SJ. Melioidosis. Curr Opin Infect Dis 2006; 19:421 - 8; http://dx.doi.org/10.1097/01.qco.0000244046.31135.b3; PMID: 16940864
- AuCoin DP, Reed DE, Marlenee NL, Bowen RA, Thorkildson P, Judy BM, et al. Polysaccharide specific monoclonal antibodies provide passive protection against intranasal challenge with Burkholderia pseudomallei. PLoS One 2012; 7:e35386; http://dx.doi.org/10.1371/journal.pone.0035386; PMID: 22530013
- Burtnick MN, Brett PJ, Harding SV, Ngugi SA, Ribot WJ, Chantratita N, et al. The cluster 1 type VI secretion system is a major virulence determinant in Burkholderia pseudomallei. Infect Immun 2011; 79:1512 - 25; http://dx.doi.org/10.1128/IAI.01218-10; PMID: 21300775
- Sitthidet C, Korbsrisate S, Layton AN, Field TR, Stevens MP, Stevens JM. Identification of motifs of Burkholderia pseudomallei BimA required for intracellular motility, actin binding, and actin polymerization. J Bacteriol 2011; 193:1901 - 10; http://dx.doi.org/10.1128/JB.01455-10; PMID: 21335455
- Utaisincharoen P, Anuntagool N, Limposuwan K, Chaisuriya P, Sirisinha S. Involvement of beta interferon in enhancing inducible nitric oxide synthase production and antimicrobial activity of Burkholderia pseudomallei-infected macrophages. Infect Immun 2003; 71:3053 - 7; http://dx.doi.org/10.1128/IAI.71.6.3053-3057.2003; PMID: 12761082
- Yan Y, Wang X, Pan Y, Liu H, Cheng J, Xiong Z, et al. Fabrication of viable tissue-engineered constructs with 3D cell-assembly technique. Biomaterials 2005; 26:5864 - 71; http://dx.doi.org/10.1016/j.biomaterials.2005.02.027; PMID: 15949552
- Chang R, Nam J, Sun W. Direct cell writing of 3D microorgan for in vitro pharmacokinetic model. Tissue Eng Part C Methods 2008; 14:157 - 66; http://dx.doi.org/10.1089/ten.tec.2007.0392; PMID: 18544030
- Byers AM, Tapia TM, Sassano ER, Wittman V. In vitro antibody response to tetanus in the MIMIC system is a representative measure of vaccine immunogenicity. Biologicals 2009; 37:148 - 51; http://dx.doi.org/10.1016/j.biologicals.2009.02.018; PMID: 19272794
- Geisbert TW, Jahrling PB. Towards a vaccine against Ebola virus. Expert Rev Vaccines 2003; 2:777 - 89; http://dx.doi.org/10.1586/14760584.2.6.777; PMID: 14711361
- Daddario-DiCaprio KM, Geisbert TW, Geisbert JB, Ströher U, Hensley LE, Grolla A, et al. Cross-protection against Marburg virus strains by using a live, attenuated recombinant vaccine. J Virol 2006; 80:9659 - 66; http://dx.doi.org/10.1128/JVI.00959-06; PMID: 16973570
- Warfield KL, Swenson DL, Olinger GG, Kalina WV, Aman MJ, Bavari S. Ebola virus-like particle-based vaccine protects nonhuman primates against lethal Ebola virus challenge. J Infect Dis 2007; 196:Suppl 2 S430 - 7; http://dx.doi.org/10.1086/520583; PMID: 17940980
- Dupuy LC, Richards MJ, Ellefsen B, Chau L, Luxembourg A, Hannaman D, et al. A DNA vaccine for venezuelan equine encephalitis virus delivered by intramuscular electroporation elicits high levels of neutralizing antibodies in multiple animal models and provides protective immunity to mice and nonhuman primates. Clin Vaccine Immunol 2011; 18:707 - 16; http://dx.doi.org/10.1128/CVI.00030-11; PMID: 21450977
- Paessler S, Weaver SC. Vaccines for Venezuelan equine encephalitis. Vaccine 2009; 27:Suppl 4 D80 - 5; http://dx.doi.org/10.1016/j.vaccine.2009.07.095; PMID: 19837294
- Martin SS, Bakken RR, Lind CM, Garcia P, Jenkins E, Glass PJ, et al. Comparison of the immunological responses and efficacy of gamma-irradiated V3526 vaccine formulations against subcutaneous and aerosol challenge with Venezuelan equine encephalitis virus subtype IAB. Vaccine 2010; 28:1031 - 40; http://dx.doi.org/10.1016/j.vaccine.2009.10.126; PMID: 19914193
- Carra JH, Wannemacher RW, Tammariello RF, Lindsey CY, Dinterman RE, Schokman RD, et al. Improved formulation of a recombinant ricin A-chain vaccine increases its stability and effective antigenicity. Vaccine 2007; 25:4149 - 58; http://dx.doi.org/10.1016/j.vaccine.2007.03.011; PMID: 17408819
- Smallshaw JE, Vitetta ES. Ricin vaccine development. Curr Top Microbiol Immunol 2012; 357:259 - 72; http://dx.doi.org/10.1007/82_2011_156; PMID: 21805396
- Bellanti JA, Lin FY, Chu C, Shiloach J, Leppla SH, Benavides GA, et al. Phase 1 study of a recombinant mutant protective antigen of Bacillus anthracis. Clin Vaccine Immunol 2012; 19:140 - 5; http://dx.doi.org/10.1128/CVI.05556-11; PMID: 22190398
- Jia Q, Lee BY, Clemens DL, Bowen RA, Horwitz MA. Recombinant attenuated Listeria monocytogenes vaccine expressing Francisella tularensis IglC induces protection in mice against aerosolized Type A F. tularensis. Vaccine 2009; 27:1216 - 29; http://dx.doi.org/10.1016/j.vaccine.2008.12.014; PMID: 19126421
- Brockstedt DG, Giedlin MA, Leong ML, Bahjat KS, Gao Y, Luckett W, et al. Listeria-based cancer vaccines that segregate immunogenicity from toxicity. Proc Natl Acad Sci U S A 2004; 101:13832 - 7; http://dx.doi.org/10.1073/pnas.0406035101; PMID: 15365184
- Lai XH, Golovliov I, Sjöstedt A. Expression of IglC is necessary for intracellular growth and induction of apoptosis in murine macrophages by Francisella tularensis. Microb Pathog 2004; 37:225 - 30; http://dx.doi.org/10.1016/j.micpath.2004.07.002; PMID: 15519043
- Pearson T, Giffard P, Beckstrom-Sternberg S, Auerbach R, Hornstra H, Tuanyok A, et al. Phylogeographic reconstruction of a bacterial species with high levels of lateral gene transfer. BMC Biol 2009; 7:78 - 91; http://dx.doi.org/10.1186/1741-7007-7-78; PMID: 19922616
- Srisurat N, Sermswan RW, Tatawasart U, Wongratanacheewin S. Bacterial loads and antibody responses in BALB/c mice infected with low and high doses of Burkholderia pseudomallei. Am J Trop Med Hyg 2010; 82:1102 - 5; http://dx.doi.org/10.4269/ajtmh.2010.09-0567; PMID: 20519607
- Conejero L, Patel N, de Reynal M, Oberdorf S, Prior J, Felgner PL, et al. Low-dose exposure of C57BL/6 mice to burkholderia pseudomallei mimics chronic human melioidosis. Am J Pathol 2011; 179:270 - 80; http://dx.doi.org/10.1016/j.ajpath.2011.03.031; PMID: 21703409
- Soffler C, Bosco-Lauth AM, Aboellail TA, Marolf AJ, Bowen RA. Development and characterization of a caprine aerosol infection model of melioidosis. PLoS One 2012; 7:e43207; http://dx.doi.org/10.1371/journal.pone.0043207; PMID: 22916225
- Yeager JJ, Facemire P, Dabisch PA, Robinson CG, Nyakiti D, Beck K, et al. Natural history of inhalation melioidosis in rhesus macaques (Macaca mulatta) and African green monkeys (Chlorocebus aethiops). Infect Immun 2012; 80:3332 - 40; http://dx.doi.org/10.1128/IAI.00675-12; PMID: 22778104
- Wamala JF, Lukwago L, Malimbo M, Nguku P, Yoti Z, Musenero M, et al. Ebola hemorrhagic fever associated with novel virus strain, Uganda, 2007-2008. Emerg Infect Dis 2010; 16:1087 - 92; http://dx.doi.org/10.3201/eid1607.091525; PMID: 20587179
- Grard G, Fair JN, Lee D, Slikas E, Steffen I, Muyembe JJ, et al. A novel rhabdovirus associated with acute hemorrhagic fever in central Africa. PLoS Pathog 2012; 8:e1002924; http://dx.doi.org/10.1371/journal.ppat.1002924; PMID: 23028323
- Smith HO, Hutchison CA 3rd, Pfannkoch C, Venter JC. Generating a synthetic genome by whole genome assembly: phiX174 bacteriophage from synthetic oligonucleotides. Proc Natl Acad Sci U S A 2003; 100:15440 - 5; http://dx.doi.org/10.1073/pnas.2237126100; PMID: 14657399
- Gibson DG, Benders GA, Andrews-Pfannkoch C, Denisova EA, Baden-Tillson H, Zaveri J, et al. Complete chemical synthesis, assembly, and cloning of a Mycoplasma genitalium genome. Science 2008; 319:1215 - 20; http://dx.doi.org/10.1126/science.1151721; PMID: 18218864
- Presidential Commission for the Study of Bioethical Issues. New Directions: The Ethics of Synthetic Biology and Emerging Technologies. Washington, DC. 2010. Retrieved from http://www.bioethics.gov/documents/synthetic-biology/PCSBI-Synthetic-Biology-Report-12.16.10.pdf