Abstract
Oral vaccination is the most challenging vaccination method due to the administration route. However, oral vaccination has socio-economic benefits and provides the possibility of stimulating both humoral and cellular immune responses at systemic and mucosal sites. Despite the advantages of oral vaccination, only a limited number of oral vaccines are currently approved for human use. During the last decade, extensive research regarding antigen-based oral vaccination methods have improved immunogenicity and induced desired immunological outcomes. Nevertheless, several factors such as the harsh gastro-intestinal environment and oral tolerance impede the clinical application of oral delivery systems. To date, human clinical trials investigating the efficacy of these systems are still lacking. This review addresses the rationale and key biological and physicochemical aspects of oral vaccine design and highlights the use of yeast-derived β-glucan microparticles as an oral vaccine delivery platform.
Introduction
The mucosal epithelium is the major entry site for oral pathogens, which creates the need for proper induction of local immune response to prevent infectious disease. Diarrheal disease is the second leading cause of death in children under 5-y-old, killing around 760 000 children annually.Citation1 Both respiratory and gastro-intestinal infections kill approximately 5 million children under the age of 5 in developing countries and cause more than 10 billion disease episodes annually.Citation2 Oral vaccination appears to be the only route that protects against enteric pathogens. If oral vaccination were used to prophylactically treat susceptible human populations at risk of enteric pathogens, it could prove to be the most cost-effective and efficient manner to reduce morbidity and mortality against gastro-intestinal infections.Citation3,Citation4 Successful oral vaccination will extend effective immunity to the small intestine, ascending colon, salivary and mammary glands, due to the homing of activated antigen-specific lymphocytes to remote mucosal effector sites.Citation5
Currently, most oral vaccines are based on heat-killed or attenuated pathogens. The downside to these whole pathogen-based vaccines is that they do not provoke a sufficient immune response, which consequently results in pathogen persistence and disease progression in immunocompromised individuals. For example, in 2000, outbreaks of poliomyelitis were reported in Egypt, Haiti, the Philippines, and the Dominican Republic.Citation6,Citation7 These outbreaks of polio were caused by live attenuated polio vaccine (OPV) that was orally administered. The cause of this polio outbreak was due to a reversion of the attenuated virus back to the infectious wild type strain resulting in increased neurovirulence and thus leading to infections among immunocompromised individuals.Citation8 A safer alternative might be a protein subunit vaccine, which is composed of specific antigens found within the pathogen. However, soluble antigens do not penetrate the mucosal lining of the intestine and are consequently less efficiently presented by antigen presenting cells (APCs).Citation9 These problems prevent an efficient mucosal and/or systemic immune response following oral vaccination and inhibit the ability to successfully develop new oral-based vaccines.
Undoubtedly, vaccination constitutes one of the major breakthroughs in human medicine, which has allowed the eradication of numerous infectious diseases.Citation10 Oral vaccination provides both social and economic advantages, especially in developing countries. The use of needle-free vaccine administration eliminates the risk of transmitting blood-borne pathogens and can be performed by health workers without any medical training. Furthermore, oral vaccination increases patient compliance because the pain and discomfort from a needle-stick is avoided. This ultimately results in an increased adherence to vaccination schemes. In addition, since the gut is already heavily colonized by microbiota, oral vaccine formulations do not require extensive antigen purification, simplifying the overall manufacturing process. Taken together, the simplified production, storage, and administration method for oral vaccination might preferentially favor it over conventional needle methods during pandemic situations.Citation11
Biodegradable and biocompatible microparticulate antigen carriers are novel and promising vaccination methods that may act synergistically as a delivery vehicle and an adjuvant to ensure the proper induction of both cellular and humoral immune responses.Citation12,Citation13 Microparticulate encapsulation strategies should ideally protect the antigen from degradation and increase the concentration of antigen in the vicinity of mucosal tissue for better absorption. Additionally, microparticulate antigen carriers can selectively target the gut-associated lymphoid tissue (GALT), Peyer’s patches (PP) and intestinal APCs to initiate immune responses.Citation14 The categories of particulate antigen delivery systems, including immune stimulating complexes (ISCOMs), liposomes, virus-like particles, micro- and nanoparticles have been widely reviewed in recent literature.Citation15-Citation18 In this review, we discuss the challenges regarding the development and design of oral vaccine formulations, focusing on the documented effects of yeast-derived β-glucan microparticles as an oral delivery platform.
Challenges in the Development of Oral Vaccine Formulations
The oral delivery route is the most challenging route in mucosal vaccination and many factors influence the uptake of particulate antigen carriers by the intestinal epithelium. The physiology of the gastro-intestinal tract, which is reinforced with various defense and clearance mechanisms, contributes to the equilibrium between immunity and oral tolerance in the gut. Gastric antigen degradation can be circumvented by using pH-sensitive polymers, (e.g., methacrylic acid,Citation19 hydroxypropyl methylcellulose phthalateCitation20) that protect antigens at acidic pH and respond to the slightly basic pH in the intestine due to their intrinsic ionizable functional groups leading to swelling or dissolution of the vehicle and site-specific antigen release. In contrast, other types of pH-sensitive polymers such as polymersomes dissolve under acidic conditions, which are particularly suitable for targeting tumors and endolysomoses.Citation21,Citation22 Currently, enteric coating based on Eudragit (poly (methacrylic acid-co-ethylacrylate) copolymers) formulations, which remain impermeable at the low gastric pH and dissolve upon alkaline pH in the small intestine, are extensively employed.Citation23 Combinations of Eudragit L100–55 or S-100 with other polymers have shown to improve the oral bioavailability and the controlled release of drugs.Citation24-Citation27 Shastri et al.Citation28 reported enhanced antigen-specific IgG1 and IgG2a antibodies and protection against live influenza viral challenge after 2 oral immunizations with microparticles containing inactivated influenza A/PR/34/8 H1N1 virus with Eudragit S100 and trehalose.
In addition to the wide pH gradients in the gastro-intestinal tract, the antigen is subjected to different mechanical features such as the intestinal peristaltic movement, capture by mucus and glycocalyx and attack by digestive enzymes (proteases, lipases, nucleases, bile, lactoferrin, and peroxidases), which results in antigen dilution and degradation. In this regard, an innovative approach based on particle surface modification with bio-adhesive molecules like plant lectins, microbial adhesins, specific antibodies, or Toll-Like Receptor (TLR) ligands may target the epithelial barrier through carbohydrate or receptor binding. Lectins, such as Aleuria aurantia lectin, wheat germ agglutinin or Ulex europaeus agglutinin-1 (UEA-1), are promising targeting moieties that result in M-cell-specific adherence. Several studies have shown that UEA-1-coated particles selectively bind to M-cells in murine PP upon oral gavage or injection into ligated intestinal loops.Citation29-Citation31 In humans, few M-cell markers have been reported, cathepsin E,Citation32 sialyl Lewis A antigenCitation33 and clusterinCitation34 however, their real potential as human M-cell targeting molecule awaits further investigation. An area gaining more importance is targeting vaccines to DCs together with TLR agonists, for instance, TLR9 ligands or CpG-containing oligodeoxynucleotides (CpG-ODNs). A study by Hunter et al.Citation35 showed that oral/nasal/vaginal immunization with PLG microparticles containing group B Streptococcus antigen and CpG-ODN, elicited both IgG and secretory IgA antibodies in blood and vaginal washes in mice. Multiple studies demonstrate that soluble antigen mixed with free CpG failed to induce a potent antigen-specific immune response. This contrasts with PLG particles containing CpG absorbed onto the particle surface or PLG particles containing CpG co-encapsulated with antigen inside of particles, which generate potent immune responses.Citation36,Citation37
Antigen transport is restricted by epithelial barriers created by tight cellular junctions. Smith et al.Citation38 showed that the mucoadhesive molecule chitosan reorganized the structure of the tight junction proteins occludin and zonula occludin protein-1 in Caco-2 cells, enhancing its permeation and prolonging its residence time. However, chitosan is insoluble in water at physiological pH, but its quaternized derivative N-trimethyl-chitosan (TMC) exerts high solubility over a wide pH range, which makes it favorable. In addition, TMC possesses intrinsic adjuvanticity.Citation39-Citation41
Another major challenge in oral vaccine development is oral tolerance, which downregulates cell-mediated and humoral immune responses in the gastro-intestinal tract to ensure local homeostasis. As the mucosal absorption of soluble antigens is generally very low, preferential induction of tolerance occurs in the absence of adjuvants. However, particulate antigens are more effective at stimulating protective immunity and cross-presentation due to their adjuvant properties discussed later. Many particulate vaccine strategies including β-glucan microparticles,Citation42 PLGA,Citation43 exosomes, virus-like particlesCitation44 and spray-dried polyelectrolyte microspheresCitation45 have been reported to exert efficient cross-presentation at up to 1000-fold lower antigen doses. It has also been reported by De Koker et al.Citation46 that OVA-loaded polyelectrolyte capsules strongly enhance antigen presentation to both CD4+ OT-I and CD8+ OT-II cells, allowing cross-presentation at a 50-fold lower antigen dose when compared with soluble OVA alone.
A second group of factors affecting antigen processing and efficiency of antigen uptake are associated with the host itself such as species, age, genetic nature, and nutritional and health status. Oral vaccines against rotavirus, V. cholerae and Escherichia coli (ETEC) have been found to be less effective in developing countries, ascribed to the differences in nutritional status, natural/maternal antibodies, poor sanitation, and gut microflora like ongoing persistent infections with helminths and parasites.Citation47 Nutrition-related factors such as malnutrition, vitamin A or zinc deficiency, and malabsorption of nutrients as a consequence of intestinal inflammation, may all result in diminished mucosal immunity in response to oral vaccination.Citation48,Citation49 Data with regard to the impact of vitamin A deficiency on the immunogenicity and protective vaccine efficacy are conflicting. Although most studies could not demonstrate an effect of vitamin A deficiency or supplementation on antibody levels in response to vaccination,Citation50 some clinical and animal studies showed diminished antibody responses.Citation51,Citation52 Jaensson-Gyllenback et al.Citation53 described that vitamin A deficiency directly impairs intestinal CD103+ dendritic cell function. Retinoic acid (RA), a vitamin A metabolite, has been shown to be a crucial regulator in intestinal immunity and homeostasis by promoting IgA class switch recombination, plasma cell differentiation, and development of regulatory T-cells.Citation54 Furthermore, antibodies from breast milk or the maternal placenta may also impede mucosal vaccine immunogenicity.
Finally, the factors associated with physicochemical properties of antigen delivery vehicles, such as surface charge, size, hydrophobicity/hydrophilicity balance, antigen dose, and total number of particles per dose, all need to be considered in the design of safe and efficacious oral vaccines.
Vaccine Design
The development of an effective oral vaccine delivery system requires careful consideration of its physicochemical properties. Ideally, these systems should (1) be safe, (2) overcome the harsh gastro-intestinal environment, (3) target the immune inductive sites, and (4) induce long lasting immunological memory. Particle size is of utmost importance for the in vivo distribution, fate, and targeting ability of the oral carrier. It is generally known that nanoparticles are better absorbed than microparticles.Citation55 For instance, according to Desai et al.,Citation56 100 nm nanoparticles in rats were 15- to 250-fold better absorbed than 500 nm and 10 µm particles, respectively. Nevertheless, many reports describe that both APCs and PP M-cells are able to internalize particles up to 10 µm through phagocytosis or macropinocytosis.Citation57-Citation60 Indeed, particulate vaccines typically within the 0.1–10 μm range, resemble the dimensions of common bacteria and viruses, and therefore are recognized by the immune system and readily phagocytosed by APCs. Moreover, small particle internalization occurs through receptor-mediated endocytosis in the PP. Small particles are subsequently disseminated to the mesenteric lymph nodes, blood circulation, and spleen, whereas larger particles are retained in the lymphoid tissue.Citation61,Citation62 In addition, particle size also plays a role in antigen loading, antigen release, and particulate carrier stability. Nano- and submicron-sized particles exhibit a large surface area, which accelerates their carrier dissolution and antigen release. In contrast, larger particles allow higher antigen encapsulation and slower antigen release.
Surface hydrophobicity and surface charge are likewise contributing factors in the efficiency of particle uptake in the gastro-intestinal tract. More hydrophobic and polymeric particles, e.g., polystyrene and PLGA particles show a strong affinity to PP M-cells due to increased permeability through mucins and appear to recruit phagocytic cells more efficiently.Citation63,Citation64 On the other hand, hydrophilic particles like cellulose and cellulose acetate are preferably translocated to intracellular compartments. Although positively charged particles interact more efficiently with the negatively charged mucins and cell membranes, Shakweh and Jung reported that negatively charged particles and neutral particles exhibited a greater affinity to PP over positively charged nanoparticles.Citation57,Citation65 In turn, Nakanishi et al.Citation66 demonstrated that positively charged particles induced a more efficient cytotoxic T-cell response in mice. Other advantages of particulate delivery systems are the possibility for high antigen encapsulation, depot formation, and co-delivery of antigens and adjuvants.
Beta-Glucans
Beta-glucans are carbohydrate polymers found in the cell walls of fungi, yeast, plants and bacteria, but also in cereals like oat and barley. Depending on the source, β-glucans contain primary structural variations and physicochemical parameters (solubility, primary structure, molecular weight, and branching), which may influence their physiological functions. Βeta-glucans have been shown to elicit a broad range of functions.Citation67 First of all, β-glucans are known to be potent activators of the innate immune system. Several in vitro studies have demonstrated the intrinsic adjuvant capacity of β-1,3-D-glucans from yeast and fungi in enhancing the functional activity of neutrophils, macrophages, DCs, and epithelial cells. For instance, Saccharomyces cerevisiae glucan increased TNF-α production in rat alveolar macrophages in vitro.Citation68 Incubation of human whole blood with PGG-glucan (a soluble β-1,3-glucan) enhanced the oxidative burst by leukocytes and increased their antimicrobial activity, all in the absence of inflammatory cytokine production.Citation69 Second, β-1,3-D-glucans exert beneficial effects on T- and B-cell responses. Beta-glucan binding to dectin-1 on DCs and macrophages triggers their activation and maturation and enhances the production of pro-inflammatory cytokines such as TNF-α, IL-6, IL-2, IL-10, and IL-23. The aforementioned cytokines are able to prime CD4+ T-cells and instruct their differentiation into Th1 and Th17 T-helper cells.Citation70 Additionally, dectin-1-mediated recognition of β-glucan induces the activation of antigen-specific CD8+ cytotoxic T-lymphocytes (CTL).Citation71 Third, β-1,3-D-glucans possess anti-infective activity (stimulates innate immune responses) against various bacterial, viral, protozoan, and fungal diseases. Beta-glucans may act as an immunotherapeutic agent in the treatment of a number of diseases. For instance, lentinin (from shiitake mushrooms) enhanced host resistance against Mycobacterium tuberculosis and Streptococcus pneumoniae in mice and oat β-glucan had potent activity against HIV-1 in human peripheral blood mononuclear cells.Citation72-Citation74 The Ostroff group showed that orally administered yeast β-glucan particles increased survival time in mice challenged with the anthrax bacteria.Citation75 Finally, it has been shown that β-glucans have immunomodulatory and cytotoxic effects in cancer by inhibiting tumor growth and increasing survival. Supplementing chemotherapy treatment with β-glucans from Grifola frondosa-extracted Maitake D-Fraction resulted in decreased lung, liver, and breast tumor sizes in 60% of the patients as compared with chemotherapy alone.Citation76 Similarly, combining Schizophyllan (derived from Schizophyllan commune) with conventional chemotherapy improved the long-term survival rate in patients with ovarian cancer.Citation77
Yeast-Derived β-Glucan Microparticles as a Delivery Platform
The β-glucan particle (GP) delivery platform features efficient antigen loading and receptor-targeted uptake in APCs combined with the inherent adjuvant function. GPs are highly purified, hollow, porous 3–4 micron cell wall shells () manufactured by treating baker's yeast S. cerevisiae with a series of alkaline, acid, and solvent extractions. S. cerevisiae glucan consists of β-1,3-linked glycopyranosyl residues with smaller numbers of β-1,6-linked branches (). After alkali and acidic treatment, these hollow yeast shells are composed primarily of β-1,3-glucan (>85%) chitin/chitosan (2%) and are devoid of proteins, lipids (1%), and mannans.Citation78
Figure 1. Transmission electron micrograph (A) and scanning electron microscopy (B) images of ovalbumin-loaded β-glucan particles (De Smet et al.Citation60).
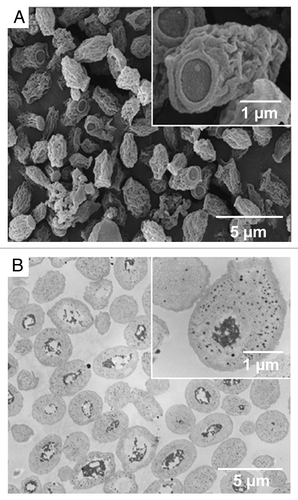
Figure 2. Structure of yeast β-glucan (adapted from Volman et al.Citation112). Polymer of β-(1–3)-D-glycopyranosyl units with branching at β-(1–6)-D-glycopyranosyl units. S. cerevisiae structure consists of β-1–3 and small numbers of β-1–6 branches and β-1–6 linkages.
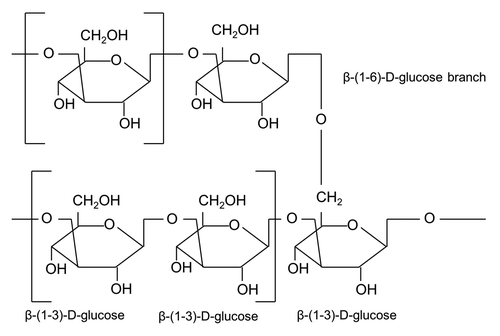
CR3 and dectin-1 are 2 membrane β-1,3-glucan receptors that have been characterized at the molecular level. GPs are predominantly recognized by dectin-1 (also known as CLEC7A), a C-type lectin receptor that is highly expressed on cells of myeloid origin, including DCs, macrophages, monocytes, and neutrophils.Citation79,Citation80 The second receptor is the iC3b-complement receptor 3 (CR3, also known as Mac-1, CD11b/CD18, or αMβ2 integrin), which is highly expressed on neutrophils, monocytes, macrophages, and natural killer cells.Citation81,Citation82 CR3 is involved in the anti-tumor properties of β-glucans as studies by Xia et al.Citation82,Citation83 have shown that β-glucan-CR3-mediated binding triggered cytotoxic degranulation on iC3b-coated tumor cells. In addition to dectin-1 and CR3, other receptors have been reported to bind β-glucans, including lactosylceramide, scavenger receptors, and CD5.Citation84,Citation85 GPs are also potent activators of the alternative complement pathway, which leads to deposition of opsonic C3 fragments on the surface of GPs. GPs stimulate DCs to produce cytokines such as IFN-γ and IL-17, which are associated with beneficial responses in vaccine models of pathogen protection. Moreover, synergistic DC cytokine responses are seen when GPs are combined with TLR agonists.
Microparticulate β-Glucans Stimulate Innate Immunity
A number of groups studied the innate immunostimulatory activity of both soluble and particulate β-glucan formulations from S. cerevisiae.Citation86-Citation90 The group of Hunter gave mice daily oral doses of 0.1 mg kg−1 of microparticulate β-glucan preparations for 2 wk, which significantly increased the phagocytic activity of mouse peritoneal macrophages.Citation87 In-depth in vitrostudies of β-glucan microparticulate-treated peritoneal macrophages by Hunter et al.Citation88 showed enhanced B7.1 and B7.2 co-stimulatory molecule expression, which are mandatory as a co-signal in T-cell activation and proliferation. Berner et al.Citation89,Citation90 demonstrated that β-glucan microparticulates are rapidly phagocytized by peritoneal macrophages and induced the upregulation and secretion of the pro-inflammatory cytokines TNF-α, IL-6, and IL-1β. This response was even more enhanced upon IFN-γ priming. Previous reports by Hoffman et al.Citation91 and by Abel and CzopCitation92 described similar cytokine profiles in rat alveolar macrophages and human monocytes. In accordance, a recent study by Huang et al.Citation93 described likewise a strong TNF-α production by IFN-γ primed human DCs upon yeast glucan particle stimulation. This striking increase of cytokine production is caused by IFN-γ priming, which is believed to lower the response threshold of innate cells to microparticulate β-glucans.
Use of Innate Immunity Against Cancer and Infectious Disease Challenge
Several groups made beneficial use of the innate immunomodulatory activity of these β-glucan formulations to protect against cancer and infectious disease challenge. Kournikakis et al.Citation75 reported that subcutaneous injection or oral administration of yeast β-glucan particles prior to anthrax challenge in mice, increased the survival rate, reduced pulmonary bacterial load and increased the proportion of bacteria-free animals.
Hurtgen and colleagues performed an intranasal challenge with Coccidioides spores in mice, after the subcutaneous vaccination with yeast glucan particles loaded with an epitope-based vaccine.Citation94 Vaccinated mice exhibited an adequate protection against Coccidioidomycosis as featured by lung infiltration of activated Th1, Th2, and Th17 cells, significant reduction of colony forming units and increase of survival compared with non-vaccinated mice.
Two studies by Hong elaborate on the impact of the delivery of whole β-glucan particles in different murine tumor models.Citation95,Citation96 Orally delivered β-glucan particles are preferentially internalized by macrophages, which migrate to the spleen, lymph nodes, and bone marrow.
Upon processing, small fragments of β-glucan particles bind to the CR3-receptor of granulocytes that trigger cytotoxicity of iC3b-opsonized tumor cells. The combined treatment of β-glucans and antitumor antibodies induced significant tumor regression. Also Li et al.Citation97 evidenced reduced tumor burden upon oral particulate β-glucan administration in mice and CR3-dependent neutrophil induced cytotoxicity. In addition, β-glucan particles interacted with DCs and augmented antitumor CD4+ and CD8+ T-cell responses.
β-glucan Particles as Orally Delivery of Nucleic Acids (siRNA, DNA)
Importantly, GPs have been effectively used for both oral- and parenteral-targeted delivery of a wide range of drug classes (small molecules, siRNA/DNA, peptide/protein antigens, and nanoparticles) in preclinical animal models.Citation93,Citation94,Citation98-Citation103
Based on the in situ layer-by-layer synthesis through electrostatic interactions, the group of Soto initially engineered yeast cell wall particles for DNA delivery.Citation104 This encapsulation strategy enables a high nucleic acid capacity and delivery, in addition to the oral bioavailability of β-glucan particles and their inherent β-glucan receptor targeting capacity toward APCs. However, a disadvantage associated with the sequential adsorption of polymers in particular of polyethylenimine (PEI) is that high concentration ranges are cytotoxic. Huang et al.Citation102 eliminated this PEI-related toxicity, by using alternating hydration and lyophilization steps in the loading process of antigen inside the GP shells. Nonetheless, using this nanomaterial engineering approach, the GP delivery technology platform has been expanded for co-formulating TLR adjuvants and/or siRNAs in discrete layers around the antigen core providing for multiplexed co-delivery to augment vaccine efficacy and safety.
The group of Aouadi designed β-1,3-D-glucan-loaded siRNA particles for oral targeting of M-cells and oral siRNA delivery to mouse macrophages.Citation105 Oral gavage with glucan-encapsulated siRNA particles once daily for 8 consecutive days, resulted in internalization by GALT macrophages, in which siRNA-mediated gene silencing occurred and subsequently migrated to spleen, liver, and lung. Also Tesz and colleagues combined this β-glucan receptor targeting and high RNA encapsulation capacity of glucan shells to obtain gene silencing in phagocytic cells, following intraperitoneal administration.Citation106
Microparticulate β-Glucan Sampling Across the Intestinal Mucosae
Besides their application as DNA delivery system, the use of β-glucan microparticles as oral vaccine delivery platform has recently gained increased interest in the pharmaco-medical field. introduces the pathways of β-glucan microparticle internalization in the GALT, in which M-cells, epithelial cells, and dendritic cells are suggested as pivotal players in translocation across the intestinal epithelium.
Figure 3. Schematic representation of the pathways of β-glucan microparticle uptake. Beta-glucan microparticulate antigen uptake in mucosal inductive sites may occur through 3 different pathways: M-cells, intestinal epithelial cells, and dendritic cells. (Route 1) Non-migratory gut-resident CX3CR1-expressing dendritic cells protrude dendrites through epithelial tight junctions into the lumen to directly sample luminal antigen. (Route 2) Transcellular uptake of β-glucan microparticles across enterocytes by endocytosis. (Route 3) M-cells are specialized in antigen uptake because of their sparse glycocalyx, limited microvillus border, reduced enzymatic activity, and active transcytotic pathway. Transcytosis is a particular process by which M-cells endocytose antigens at their apical membrane, resulting in antigen transport into endosomal tubules, vesicles and large multivesicular bodies and finally exocytosis into the basolateral pocket. FAE, follicle-associated epithelium; SED, subepithelial dome; IFR, interfollicular region; HEV, high endothelial venule.
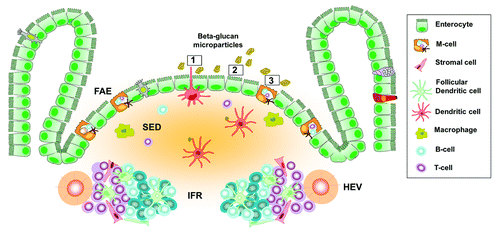
A variety of in vitro and in vivo models were used to study the interaction between β-glucan-based carrier systems and the intestinal epithelium and are predominantly focused on M-cells. Ahmad et al.Citation107 addressed the uptake and translocation of yeast-derived glucan microparticles across an in vitro M-cell-like model of human intestinal PP, based on the co-culture of Caco-2 cells and Raji B lymphocytes. Surface binding and transport of yeast-derived glucan particles occurred more efficiently across co-cultures vs. mono-cultures. In contrast, β-glucan microparticles (GP) were efficiently and rapidly internalized by Caco-2 and HT-29 cells, modeling the human small and large intestinal epithelial cells respectively. Cellular uptake of GP increased with incubation time and was dose-dependent.Citation60
In 1998, Beier and Gebert investigated the kinetics of S. cerevisiae in PP of minipigs by means of intestinal loops. Yeast particle uptake in the gut of minipigs takes about 1 h of transport from the gut to the underlying immune cells.Citation108 After 4 h, the majority of the yeast cells were present in phagocytes populating the subepithelial lymphoid tissue. A 24 h incubation period yielded no detectable yeast particles in the PP domes, which is suggestive of biodegradation by phagocytes that migrated to the mesenterial lymph nodes. TEM imaging of murine PP following GP inoculation for 1 h in intestinal ligated loops by De Smet et al.Citation60 confirmed endocytosis and transport of GP by M-cells occurred through transcellular pathways. Overall, these studies suggest that M-cell internalization and transcytosis of β-glucan particles are rapidly advancing processes and therefore the most principal gateways in PP.
Oral Immunization with Microparticulate β-Glucans
Another focus is the impact of oral vaccination with antigens and β-glucans as antigen delivery platform to promote enhanced adaptive immune responses. Two groups investigated oral immunization with β-glucan microparticles carrying model antigens such as OVA and BSA. A study by Berner demonstrated that microparticulate β-glucan conjugated to BSA was able to initiate an enhanced IgG response against BSA in both intradermal and oral immunizations.Citation90 Food pellets coated with MG/F-BSA conjugates were orally administered to mice as primary immunization and booster doses after 24 d. This MG/F-BSA conjugate induced a significant adaptive immune response, characterized by elevated IgG titers on day 29 and up to a 100-fold increase on day 35 in comparison with other groups.
De Smet et al.Citation60 investigated the effect of oral microparticulate OVA-loaded glucan particles (GP-OVA) on local and systemic humoral responses in mice. Animals were sensitized for 3 consecutive days with either 100 µg or 300 µg OVA-containing formulations and boosted on days 14 and 28. Oral administration of GP-OVA induced increased OVA-specific IgA, secretory-IgA and secretory component production in intestinal fluids. Moreover, adoptive transfer experiments in OT-II transgenic mice showed proliferating OVA-specific CD4+ T-cells mainly in the spleens of GP-OVA-fed mice. This OT-II proliferation is accompanied by an increased IL-17 production and a tendency toward increased levels of IFN-γ, characteristic for a mixed Th17/Th1 response. The most crucial cellular players, antibody and T-cell mediated responses upon β-glucan microparticulate internalization are depicted in . This is in agreement with a study performed by Huang et al.Citation109 wherein subcutaneous administrations of GP formulations elicited strong antigen-specific antibody and T-cell responses, including the production of IFN-γ and IL17a by CD4+ Th1 and Th17 cells.
Figure 4. (A) Activated dendritic cells may migrate to the mesenteric lymph nodes and process the β-glucan particles into peptides that are presented to T-cells. Presentation of β-glucan microparticulate antigen by dendritic cells via MHC class II molecules to CD4+ helper T-cells, leads to the activation, proliferation, and differentiation of antigen-specific Th17 and Th1 cells. Furthermore, dendritic cells and the intestinal epithelium produces cytokines such as BAFF, APRIL, and TGF-β1 that trigger the process of isotype switching and differentiation of IgA-committed B cells to IgA-producing plasma cells, which produce dimeric IgA. (B) Mature APCs and antigen-primed lymphoid cells will travel along the mesenteric lymph nodes, through the thoracic duct into the blood stream and end up at mucosal effector sites such as epithelia and lamina propria, where a cellular (effector T-lymphocytes) and humoral (secretory IgA-production by plasma cells) immune response is generated. CCR9 or CCR10 is expressed on gut-homing B-cells and T-cells which interact with CCL25 or CCL28 on the epithelium of the small or large intestine, respectively. IgA, immunoglobulin A; SC, secretory component; S-IgA, secretory IgA.
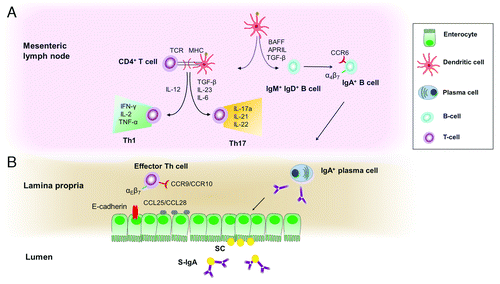
Conclusions and Perspectives
Contemporary developments in the use of delivery systems and adjuvants have undeniably improved the effectiveness of oral vaccination in animal models. However, knowledge obtained from animal models has not been extrapolated to human beings. The use of particulate systems to deliver clinical relevant antigens has yet to be established in humans. In particular, the differences in microbiome, physiology, immunogenicity, and genetic diversity between animals and humans, may affect vaccine safety and effectiveness. To address the gaps in our understanding of the human mucosal immune system, a full investigation of the unique properties of mucosal surfaces (e.g., gastrointestinal tract) are indispensable. Currently, all licensed oral vaccines are composed of attenuated or inactivated forms of disease-causing pathogens. However, these types of vaccines have been shown to have safety concerns as discussed earlier for polio. In addition, soluble antigens do not sufficiently penetrate the mucus layer of the intestine, are taken up less by APCs and will rather generate tolerance since the host strives to maintain mucosal homeostasis by responding to mucosal antigens with tolerance. For these reasons, currently no subunit vaccines are listed among those approved for oral vaccination.
An area requiring additional effort and consideration is the identification, exploration, and use of targeting molecules such as lectins, antibodies, and TLR ligands to target M-cells and DCs, or immune-inductive sites (e.g., Peyer’s patches). Surface modification of particulate carriers with targeting molecules may enhance carrier uptake and promote optimal immune activation without the induction of adverse reactions. A deeper understanding of ligand-receptor interactions and an in-depth investigation of both the biological and technological aspects relating to these vaccine tailored formulations may contribute to the translational research into humans.
Furthermore, the pharmaceutical industry is charged with many constraints on costs, formulation stability, shelf-life, chemical and physical processes that might cause antigen damage and cold-chain-constrained settings regarding oral vaccine design. Newer generation oral vaccines must comply with high safety, stability, and immunogenicity standards and must generate protective and therapeutic immune responses.
Particulate β-glucans may serve as a suitable vaccine delivery platform because they are FDA approved as GRAS (generally recognized as safe) since 2007 and established in large animals and humans. In addition to their cost-effectiveness in large-scale manufacturing, they exert intrinsic adjuvanticity and are potent activators of the innate immune system suitable for both immunocompetent and immunocompromised subjects.Citation110,Citation111 As vaccine delivery platform, β-glucan microparticles have a large antigen payload and antigen sparing capacity with the opportunity of co-delivery of both antigen and secondary adjuvant. Their β-1,3-D-glucan composition along with their 3–4 micron size enables receptor-targeted uptake by M-cells and APCs. However, several issues concerning the switch from liquid to dry formulations, the use of a relevant cancer or infectious disease antigen that provides protection in a mammalian system, still awaits further investigation.
In conclusion, the impact of particle characteristics on the human intestinal epithelium and lymphoid tissue along with the role of cytokines in the development of immunity and tolerance still needs to be elucidated. Therefore, a tight network between preclinical research and clinical vaccine development may provide new vaccine options for both human and animal health worldwide.
Abbreviations: | ||
DC | = | dendritic cell |
APC | = | antigen presenting cell |
GALT | = | gut-associated lymphoid tissue |
PP | = | Peyer’s patches |
M-cell | = | microfold cell |
OVA | = | ovalbumin |
GP | = | beta-glucan particle |
TLR | = | Toll-like receptor |
Acknowledgments
R.D.S. is supported by a doctoral grant from the Concerted Research Action of the University of Ghent (BOF10/GOA/21, project number: 01GC2110W; Ghent, Belgium). L.A. is supported by a doctoral grant from the Special Research Fund of Ghent University (01D41012).
References
- World Health Organization. Diarrhoeal disease, fact sheet number 330. 2013. Available from: http://www.who.int/mediacentre/factsheets/fs330/en/
- Black RE, Cousens S, Johnson HL, Lawn JE, Rudan I, Bassani DG, Jha P, Campbell H, Walker CF, Cibulskis R, et al, Child Health Epidemiology Reference Group of WHO and UNICEF. Global, regional, and national causes of child mortality in 2008: a systematic analysis. Lancet 2010; 375:1969 - 87; http://dx.doi.org/10.1016/S0140-6736(10)60549-1; PMID: 20466419
- Levine MM. Immunization against bacterial diseases of the intestine. J Pediatr Gastroenterol Nutr 2000; 31:336 - 55; http://dx.doi.org/10.1097/00005176-200010000-00003; PMID: 11045827
- Brandtzaeg P. Induction of secretory immunity and memory at mucosal surfaces. Vaccine 2007; 25:5467 - 84; http://dx.doi.org/10.1016/j.vaccine.2006.12.001; PMID: 17227687
- Holmgren J, Czerkinsky C. Mucosal immunity and vaccines. Nat Med 2005; 11:Suppl S45 - 53; http://dx.doi.org/10.1038/nm1213; PMID: 15812489
- From the Centers for Disease Control and Prevention. Acute flaccid paralysis associated with circulating vaccine-derived poliovirus--Philippines, 2001. JAMA 2002; 287:311
- Centers for Disease Control and Prevention (CDC). Update: Outbreak of poliomyelitis--Dominican Republic and Haiti, 2000-2001. MMWR Morb Mortal Wkly Rep 2001; 50:855 - 6; PMID: 11665846
- Greensfelder L. Infectious diseases. Polio outbreak raises questions about vaccine. Science 2000; 290:1867 - 9; http://dx.doi.org/10.1126/science.290.5498.1867b; PMID: 11187033
- Mayer L, Shao L. Therapeutic potential of oral tolerance. Nat Rev Immunol 2004; 4:407 - 19; http://dx.doi.org/10.1038/nri1370; PMID: 15173830
- O’Hagan DT, Valiante NM. Recent advances in the discovery and delivery of vaccine adjuvants. Nat Rev Drug Discov 2003; 2:727 - 35; http://dx.doi.org/10.1038/nrd1176; PMID: 12951579
- Holmgren J, Czerkinsky C, Eriksson K, Mharandi A. Mucosal immunisation and adjuvants: a brief overview of recent advances and challenges. Vaccine 2003; 21:Suppl 2 S89 - 95; http://dx.doi.org/10.1016/S0264-410X(03)00206-8; PMID: 12763689
- McNeela EA, Lavelle EC. Recent advances in microparticle and nanoparticle delivery vehicles for mucosal vaccination. Curr Top Microbiol Immunol 2012; 354:75 - 99; http://dx.doi.org/10.1007/82_2011_140; PMID: 21904984
- Mann JFS, Acevedo R, Campo JD, Pérez O, Ferro VA. Delivery systems: a vaccine strategy for overcoming mucosal tolerance?. Expert Rev Vaccines 2009; 8:103 - 12; http://dx.doi.org/10.1586/14760584.8.1.103; PMID: 19093777
- Brandtzaeg P. Function of mucosa-associated lymphoid tissue in antibody formation. Immunol Invest 2010; 39:303 - 55; http://dx.doi.org/10.3109/08820131003680369; PMID: 20450282
- Barr IG, Mitchell GF. ISCOMs (immunostimulating complexes): the first decade. Immunol Cell Biol 1996; 74:8 - 25; http://dx.doi.org/10.1038/icb.1996.2; PMID: 8934649
- Shi Y, Huang GH. Recent developments of biodegradable and biocompatible materials based micro/nanoparticles for delivering macromolecular therapeutics. Crit Rev Ther Drug Carrier Syst 2009; 26:29 - 84; http://dx.doi.org/10.1615/CritRevTherDrugCarrierSyst.v26.i1.20; PMID: 19496747
- Perrie Y, Obrenovic M, McCarthy D, Gregoriadis G. Liposome (Lipodine)-mediated DNA vaccination by the oral route. J Liposome Res 2002; 12:185 - 97; http://dx.doi.org/10.1081/LPR-120004792; PMID: 12604053
- Ludwig C, Wagner R. Virus-like particles-universal molecular toolboxes. Curr Opin Biotechnol 2007; 18:537 - 45; http://dx.doi.org/10.1016/j.copbio.2007.10.013; PMID: 18083549
- Jones MC, Ranger M, Leroux JC. pH-sensitive unimolecular polymeric micelles: synthesis of a novel drug carrier. Bioconjug Chem 2003; 14:774 - 81; http://dx.doi.org/10.1021/bc020041f; PMID: 12862430
- Wang XQ, Dai JD, Chen Z, Zhang T, Xia GM, Nagai T, Zhang Q. Bioavailability and pharmacokinetics of cyclosporine A-loaded pH-sensitive nanoparticles for oral administration. J Control Release 2004; 97:421 - 9; http://dx.doi.org/10.1016/j.jconrel.2004.03.003; PMID: 15212874
- Shen Y, Tang H, Radosz M, Van Kirk E, Murdoch WJ. pH-responsive nanoparticles for cancer drug delivery. Methods Mol Biol 2008; 437:183 - 216; http://dx.doi.org/10.1007/978-1-59745-210-6_10; PMID: 18369970
- Lomas H, Canton I, MacNeil S, Du J, Armes SP, Ryan AJ, Lewis AL, Battaglia G. Biomimetic pH sensitive polymersomes for efficient DNA encapsulation and delivery. Adv Mater 2007; 19:4238; http://dx.doi.org/10.1002/adma.200700941
- De Jaeghere F, Allémann E, Kubel F, Galli B, Cozens R, Doelker E, Gurny R. Oral bioavailability of a poorly water soluble HIV-1 protease inhibitor incorporated into pH-sensitive particles: effect of the particle size and nutritional state. J Control Release 2000; 68:291 - 8; http://dx.doi.org/10.1016/S0168-3659(00)00272-8; PMID: 10925137
- Dai J, Nagai T, Wang X, Zhang T, Meng M, Zhang Q. pH-sensitive nanoparticles for improving the oral bioavailability of cyclosporine A. Int J Pharm 2004; 280:229 - 40; http://dx.doi.org/10.1016/j.ijpharm.2004.05.006; PMID: 15265562
- Makhlof A, Tozuka Y, Takeuchi H. pH-Sensitive nanospheres for colon-specific drug delivery in experimentally induced colitis rat model. Eur J Pharm Biopharm 2009; 72:1 - 8; http://dx.doi.org/10.1016/j.ejpb.2008.12.013; PMID: 19348015
- Yoo JW, Giri N, Lee CH. pH-sensitive Eudragit nanoparticles for mucosal drug delivery. Int J Pharm 2011; 403:262 - 7; http://dx.doi.org/10.1016/j.ijpharm.2010.10.032; PMID: 20971177
- Sajeesh S, Sharma CP. Novel pH responsive polymethacrylic acid-chitosan-polyethylene glycol nanoparticles for oral peptide delivery. J Biomed Mater Res B Appl Biomater 2006; 76:298 - 305; http://dx.doi.org/10.1002/jbm.b.30372; PMID: 16130147
- Shastri PN, Kim MC, Quan FS, D’Souza MJ, Kang SM. Immunogenicity and protection of oral influenza vaccines formulated into microparticles. J Pharm Sci 2012; 101:3623 - 35; http://dx.doi.org/10.1002/jps.23220; PMID: 22711602
- Foster N, Clark MA, Jepson MA, Hirst BH. Ulex europaeus 1 lectin targets microspheres to mouse Peyer’s patch M-cells in vivo. Vaccine 1998; 16:536 - 41; http://dx.doi.org/10.1016/S0264-410X(97)00222-3; PMID: 9491509
- Clark MA, Blair H, Liang L, Brey RN, Brayden D, Hirst BH. Targeting polymerised liposome vaccine carriers to intestinal M cells. Vaccine 2001; 20:208 - 17; http://dx.doi.org/10.1016/S0264-410X(01)00258-4; PMID: 11567766
- Gupta PN, Khatri K, Goyal AK, Mishra N, Vyas SP. M-cell targeted biodegradable PLGA nanoparticles for oral immunization against hepatitis B. J Drug Target 2007; 15:701 - 13; http://dx.doi.org/10.1080/10611860701637982; PMID: 18041638
- Finzi G, Cornaggia M, Capella C, Fiocca R, Bosi F, Solcia E, Samloff IM. Cathepsin E in follicle associated epithelium of intestine and tonsils: localization to M cells and possible role in antigen processing. Histochemistry 1993; 99:201 - 11; http://dx.doi.org/10.1007/BF00269138; PMID: 8491674
- Giannasca PJ, Giannasca KT, Leichtner AM, Neutra MR. Human intestinal M cells display the sialyl Lewis A antigen. Infect Immun 1999; 67:946 - 53; PMID: 9916113
- Verbrugghe P, Kujala P, Waelput W, Peters PJ, Cuvelier CA. Clusterin in human gut-associated lymphoid tissue, tonsils, and adenoids: localization to M cells and follicular dendritic cells. Histochem Cell Biol 2008; 129:311 - 20; http://dx.doi.org/10.1007/s00418-007-0369-4; PMID: 18097679
- Hunter SK, Andracki ME, Krieg AM. Biodegradable microspheres containing group B Streptococcus vaccine: immune response in mice. Am J Obstet Gynecol 2001; 185:1174 - 9; http://dx.doi.org/10.1067/mob.2001.117658; PMID: 11717653
- Singh M, Ott G, Kazzaz J, Ugozzoli M, Briones M, Donnelly J, O’Hagan DT. Cationic microparticles are an effective delivery system for immune stimulatory cpG DNA. Pharm Res 2001; 18:1476 - 9; http://dx.doi.org/10.1023/A:1012269226066; PMID: 11697476
- Schlosser E, Mueller M, Fischer S, Basta S, Busch DH, Gander B, Groettrup M. TLR ligands and antigen need to be coencapsulated into the same biodegradable microsphere for the generation of potent cytotoxic T lymphocyte responses. Vaccine 2008; 26:1626 - 37; http://dx.doi.org/10.1016/j.vaccine.2008.01.030; PMID: 18295941
- Smith J, Wood E, Dornish M. Effect of chitosan on epithelial cell tight junctions. Pharm Res 2004; 21:43 - 9; http://dx.doi.org/10.1023/B:PHAM.0000012150.60180.e3; PMID: 14984256
- Bal SM, Slütter B, van Riet E, Kruithof AC, Ding Z, Kersten GF, Jiskoot W, Bouwstra JA. Efficient induction of immune responses through intradermal vaccination with N-trimethyl chitosan containing antigen formulations. J Control Release 2010; 142:374 - 83; http://dx.doi.org/10.1016/j.jconrel.2009.11.018; PMID: 19932723
- Baudner BC, Morandi M, Giuliani MM, Verhoef JC, Junginger HE, Costantino P, Rappuoli R, Del Giudice G. Modulation of immune response to group C meningococcal conjugate vaccine given intranasally to mice together with the LTK63 mucosal adjuvant and the trimethyl chitosan delivery system. J Infect Dis 2004; 189:828 - 32; http://dx.doi.org/10.1086/381708; PMID: 14976599
- Suksamran T, Ngawhirunpat T, Rojanarata T, Sajomsang W, Pitaksuteepong T, Opanasopit P. Methylated N-(4-N,N-dimethylaminocinnamyl) chitosan-coated electrospray OVA-loaded microparticles for oral vaccination. Int J Pharm 2013; 448:19 - 27; http://dx.doi.org/10.1016/j.ijpharm.2013.03.015; PMID: 23524125
- Huang HB, Ostroff GR, Lee CK, Specht CA, Levitz SM. Robust Stimulation of Humoral and Cellular Immune Responses following Vaccination with Antigen-Loaded beta-Glucan Particles. Mbio 2010; 1.
- Shen H, Ackerman AL, Cody V, Giodini A, Hinson ER, Cresswell P, Edelson RL, Saltzman WM, Hanlon DJ. Enhanced and prolonged cross-presentation following endosomal escape of exogenous antigens encapsulated in biodegradable nanoparticles. Immunology 2006; 117:78 - 88; http://dx.doi.org/10.1111/j.1365-2567.2005.02268.x; PMID: 16423043
- Lattanzi L, Federico M. A strategy of antigen incorporation into exosomes: comparing cross-presentation levels of antigens delivered by engineered exosomes and by lentiviral virus-like particles. Vaccine 2012; 30:7229 - 37; http://dx.doi.org/10.1016/j.vaccine.2012.10.010; PMID: 23099330
- Dierendonck M, De Koker S, De Rycke R, Bogaert P, Grooten J, Vervaet C, Remon JP, De Geest BG. Single-step formation of degradable intracellular biomolecule microreactors. ACS Nano 2011; 5:6886 - 93; http://dx.doi.org/10.1021/nn200901g; PMID: 21866940
- De Koker S, De Geest BG, Singh SK, De Rycke R, Naessens T, Van Kooyk Y, Demeester J, De Smedt SC, Grooten J. Polyelectrolyte microcapsules as antigen delivery vehicles to dendritic cells: uptake, processing, and cross-presentation of encapsulated antigens. Angew Chem Int Ed Engl 2009; 48:8485 - 9; http://dx.doi.org/10.1002/anie.200903769; PMID: 19810070
- Madhi SA, Cunliffe NA, Steele D, Witte D, Kirsten M, Louw C, Ngwira B, Victor JC, Gillard PH, Cheuvart BB, et al. Effect of human rotavirus vaccine on severe diarrhea in African infants. N Engl J Med 2010; 362:289 - 98; http://dx.doi.org/10.1056/NEJMoa0904797; PMID: 20107214
- Walker RI. Considerations for development of whole cell bacterial vaccines to prevent diarrheal diseases in children in developing countries. Vaccine 2005; 23:3369 - 85; http://dx.doi.org/10.1016/j.vaccine.2004.12.029; PMID: 15837361
- Jaensson-Gyllenbäck E, Kotarsky K, Zapata F, Persson EK, Gundersen TE, Blomhoff R, Agace WW. Bile retinoids imprint intestinal CD103+ dendritic cells with the ability to generate gut-tropic T cells. Mucosal Immunol 2011; 4:438 - 47; http://dx.doi.org/10.1038/mi.2010.91; PMID: 21289617
- Villamor E, Fawzi WW. Effects of vitamin a supplementation on immune responses and correlation with clinical outcomes. Clin Microbiol Rev 2005; 18:446 - 64; http://dx.doi.org/10.1128/CMR.18.3.446-464.2005; PMID: 16020684
- Rahman MM, Mahalanabis D, Hossain S, Wahed MA, Alvarez JO, Siber GR, Thompson C, Santosham M, Fuchs GJ. Simultaneous vitamin A administration at routine immunization contact enhances antibody response to diphtheria vaccine in infants younger than six months. J Nutr 1999; 129:2192 - 5; PMID: 10573548
- Bhaskaram P, Rao KV. Enhancement in seroconversion to measles vaccine with simultaneous administration of vitamin A in 9-months-old Indian infants. Indian J Pediatr 1997; 64:503 - 9; http://dx.doi.org/10.1007/BF02737757; PMID: 10771879
- Jaensson-Gyllenbäck E, Kotarsky K, Zapata F, Persson EK, Gundersen TE, Blomhoff R, Agace WW. Bile retinoids imprint intestinal CD103+ dendritic cells with the ability to generate gut-tropic T cells. Mucosal Immunol 2011; 4:438 - 47; http://dx.doi.org/10.1038/mi.2010.91; PMID: 21289617
- Coombes JL, Siddiqui KR, Arancibia-Cárcamo CV, Hall J, Sun CM, Belkaid Y, Powrie F. A functionally specialized population of mucosal CD103+ DCs induces Foxp3+ regulatory T cells via a TGF-beta and retinoic acid-dependent mechanism. J Exp Med 2007; 204:1757 - 64; http://dx.doi.org/10.1084/jem.20070590; PMID: 17620361
- Jani PU, Mccarthy DE, Florence AT. Nanosphere and Microsphere Uptake Via Peyer Patches - Observation of the Rate of Uptake in the Rat after a Single Oral Dose. Int J Pharm 1992; 86:239 - 46; http://dx.doi.org/10.1016/0378-5173(92)90202-D
- Desai MP, Labhasetwar V, Amidon GL, Levy RJ. Gastrointestinal uptake of biodegradable microparticles: effect of particle size. Pharm Res 1996; 13:1838 - 45; http://dx.doi.org/10.1023/A:1016085108889; PMID: 8987081
- Shakweh M, Besnard M, Nicolas V, Fattal E. Poly (lactide-co-glycolide) particles of different physicochemical properties and their uptake by peyer’s patches in mice. Eur J Pharm Biopharm 2005; 61:1 - 13; http://dx.doi.org/10.1016/j.ejpb.2005.04.006; PMID: 16005619
- Newman KD, Elamanchili P, Kwon GS, Samuel J. Uptake of poly(D,L-lactic-co-glycolic acid) microspheres by antigen-presenting cells in vivo. J Biomed Mater Res 2002; 60:480 - 6; http://dx.doi.org/10.1002/jbm.10019; PMID: 11920673
- Dierendonck M, De Koker S, Cuvelier C, Grooten J, Vervaet C, Remon JP, De Geest BG. Facile two-step synthesis of porous antigen-loaded degradable polyelectrolyte microspheres. Angew Chem Int Ed Engl 2010; 49:8620 - 4; http://dx.doi.org/10.1002/anie.201001046; PMID: 20922728
- De Smet R, Demoor T, Verschuere S, Dullaers M, Ostroff GR, Leclercq G, Allais L, Pilette C, Dierendonck M, De Geest BG, et al. β-Glucan microparticles are good candidates for mucosal antigen delivery in oral vaccination. J Control Release 2013; 172:671 - 8; http://dx.doi.org/10.1016/j.jconrel.2013.09.007; PMID: 24041710
- Shakweh M, Ponchel G, Fattal E. Particle uptake by Peyer’s patches: a pathway for drug and vaccine delivery. Expert Opin Drug Deliv 2004; 1:141 - 63; http://dx.doi.org/10.1517/17425247.1.1.141; PMID: 16296726
- Florence AT. Nanoparticle uptake by the oral route: fulfilling its potential?. Drug Discov Today Technol 2005; 2:75 - 81; http://dx.doi.org/10.1016/j.ddtec.2005.05.019
- Eldridge JH, Hammond CJ, Meulbroek JA, Staas JK, Gilley RM, Tice TR. Controlled Vaccine Release in the Gut-Associated Lymphoid-Tissues. 1. Orally-Administered Biodegradable Microspheres Target the Peyers Patches. J Control Release 1990; 11:205 - 14; http://dx.doi.org/10.1016/0168-3659(90)90133-E
- Tabata Y, Ikada Y. Phagocytosis of Polymer Microspheres by Macrophages. Adv Polym Sci 1990; 94:107 - 41; http://dx.doi.org/10.1007/BFb0043062
- Jung T, Kamm W, Breitenbach A, Kaiserling E, Xiao JX, Kissel T. Biodegradable nanoparticles for oral delivery of peptides: is there a role for polymers to affect mucosal uptake?. Eur J Pharm Biopharm 2000; 50:147 - 60; http://dx.doi.org/10.1016/S0939-6411(00)00084-9; PMID: 10840198
- Nakanishi T, Kunisawa J, Hayashi A, Tsutsumi Y, Kubo K, Nakagawa S, Nakanishi M, Tanaka K, Mayumi T. Positively charged liposome functions as an efficient immunoadjuvant in inducing cell-mediated immune response to soluble proteins. J Control Release 1999; 61:233 - 40; http://dx.doi.org/10.1016/S0168-3659(99)00097-8; PMID: 10469918
- Samuelsen AB, Schrezenmeir J, Knutsen SH. Effects of orally administered yeast-derived beta-glucans: A review. Mol Nutr Food Res 2013; 58:183 - 93; http://dx.doi.org/10.1002/mnfr.201300338; PMID: 24019098
- Olson EJ, Standing JE, Griego-Harper N, Hoffman OA, Limper AH. Fungal beta-glucan interacts with vitronectin and stimulates tumor necrosis factor alpha release from macrophages. Infect Immun 1996; 64:3548 - 54; PMID: 8751898
- Wakshull E, Brunke-Reese D, Lindermuth J, Fisette L, Nathans RS, Crowley JJ, Tufts JC, Zimmerman J, Mackin W, Adams DS. PGG-glucan, a soluble beta-(1,3)-glucan, enhances the oxidative burst response, microbicidal activity, and activates an NF-kappa B-like factor in human PMN: evidence for a glycosphingolipid beta-(1,3)-glucan receptor. Immunopharmacology 1999; 41:89 - 107; http://dx.doi.org/10.1016/S0162-3109(98)00059-9; PMID: 10102791
- LeibundGut-Landmann S, Gross O, Robinson MJ, Osorio F, Slack EC, Tsoni SV, Schweighoffer E, Tybulewicz V, Brown GD, Ruland J, et al. Syk- and CARD9-dependent coupling of innate immunity to the induction of T helper cells that produce interleukin 17. Nat Immunol 2007; 8:630 - 8; http://dx.doi.org/10.1038/ni1460; PMID: 17450144
- Leibundgut-Landmann S, Osorio F, Brown GD, Reis e Sousa C. Stimulation of dendritic cells via the dectin-1/Syk pathway allows priming of cytotoxic T-cell responses. Blood 2008; 112:4971 - 80; http://dx.doi.org/10.1182/blood-2008-05-158469; PMID: 18818389
- Markova N, Kussovski V, Drandarska I, Nikolaeva S, Georgieva N, Radoucheva T. Protective activity of Lentinan in experimental tuberculosis. Int Immunopharmacol 2003; 3:1557 - 62; http://dx.doi.org/10.1016/S1567-5769(03)00178-4; PMID: 12946453
- Hetland G, Ohno N, Aaberge IS, Lovik M. Protective effect of beta-glucan against systemic Streptococcus pneumoniae infection in mice. FEMS Immunol Med Microbiol 2000; 27:111 - 6; PMID: 10640605
- Wang SC, Bligh SWA, Zhu CL, Shi SS, Wang ZT, Hu ZB, Crowder J, Branford-White C, Vella C. Sulfated beta-glucan derived from oat bran with potent anti-HIV activity. J Agric Food Chem 2008; 56:2624 - 9; http://dx.doi.org/10.1021/jf072888h; PMID: 18376844
- Kournikakis B, Mandeville R, Brousseau P, Ostroff G. Anthrax-protective effects of yeast beta 1,3 glucans. MedGenMed 2003; 5:1; PMID: 12827062
- Kodama N, Komuta K, Nanba H. Effect of Maitake (Grifola frondosa) D-Fraction on the activation of NK cells in cancer patients. J Med Food 2003; 6:371 - 7; http://dx.doi.org/10.1089/109662003772519949; PMID: 14977447
- Inoue M, Tanaka Y, Sugita N, Yamasaki M, Yamanaka T, Minagawa J, Nakamuro K, Tani T, Okudaira Y, Karita T, et al. Improvement of long-term prognosis in patients with ovarian cancers by adjuvant sizofiran immunotherapy: a prospective randomized controlled study. Biotherapy 1993; 6:13 - 8; http://dx.doi.org/10.1007/BF01877381; PMID: 8507540
- Young SH, Ostroff GR, Zeidler-Erdely PC, Roberts JR, Antonini JM, Castranova V. A comparison of the pulmonary inflammatory potential of different components of yeast cell wall. J Toxicol Environ Health A 2007; 70:1116 - 24; http://dx.doi.org/10.1080/15287390701212224; PMID: 17558806
- Willment JA, Marshall ASJ, Reid DM, Williams DL, Wong SYC, Gordon S, Brown GD. The human beta-glucan receptor is widely expressed and functionally equivalent to murine Dectin-1 on primary cells. Eur J Immunol 2005; 35:1539 - 47; http://dx.doi.org/10.1002/eji.200425725; PMID: 15816015
- Taylor PR, Brown GD, Reid DM, Willment JA, Martinez-Pomares L, Gordon S, Wong SY. The beta-glucan receptor, dectin-1, is predominantly expressed on the surface of cells of the monocyte/macrophage and neutrophil lineages. J Immunol 2002; 169:3876 - 82; PMID: 12244185
- Thornton BP, Vĕtvicka V, Pitman M, Goldman RC, Ross GD. Analysis of the sugar specificity and molecular location of the beta-glucan-binding lectin site of complement receptor type 3 (CD11b/CD18). J Immunol 1996; 156:1235 - 46; PMID: 8558003
- Xia Y, Vetvicka V, Yan J, Hanikýrová M, Mayadas T, Ross GD. The beta-glucan-binding lectin site of mouse CR3 (CD11b/CD18) and its function in generating a primed state of the receptor that mediates cytotoxic activation in response to iC3b-opsonized target cells. J Immunol 1999; 162:2281 - 90; PMID: 9973505
- Xia Y, Ross GD. Generation of recombinant fragments of CD11b expressing the functional beta-glucan-binding lectin site of CR3 (CD11b/CD18). J Immunol 1999; 162:7285 - 93; PMID: 10358177
- Zimmerman JW, Lindermuth J, Fish PA, Palace GP, Stevenson TT, DeMong DE. A novel carbohydrate-glycosphingolipid interaction between a beta-(1-3)-glucan immunomodulator, PGG-glucan, and lactosylceramide of human leukocytes. J Biol Chem 1998; 273:22014 - 20; http://dx.doi.org/10.1074/jbc.273.34.22014; PMID: 9705343
- Vera J, Fenutría R, Cañadas O, Figueras M, Mota R, Sarrias MR, Williams DL, Casals C, Yelamos J, Lozano F. The CD5 ectodomain interacts with conserved fungal cell wall components and protects from zymosan-induced septic shock-like syndrome. Proc Natl Acad Sci U S A 2009; 106:1506 - 11; http://dx.doi.org/10.1073/pnas.0805846106; PMID: 19141631
- Gallin EK, Green SW, Patchen ML. Comparative effects of particulate and soluble glucan on macrophages of C3H/HeN and C3H/HeJ mice. Int J Immunopharmacol 1992; 14:173 - 83; http://dx.doi.org/10.1016/0192-0561(92)90028-J; PMID: 1320591
- Hunter KW Jr., Gault RA, Berner MD. Preparation of microparticulate beta-glucan from Saccharomyces cerevisiae for use in immune potentiation. Lett Appl Microbiol 2002; 35:267 - 71; http://dx.doi.org/10.1046/j.1472-765X.2002.01201.x; PMID: 12358685
- Hunter KW Jr., DuPre’ S, Redelman D. Microparticulate beta-glucan upregulates the expression of B7.1, B7.2, B7-H1, but not B7-DC on cultured murine peritoneal macrophages. Immunol Lett 2004; 93:71 - 8; http://dx.doi.org/10.1016/j.imlet.2004.02.006; PMID: 15134902
- Berner MD, Sura ME, Alves BN, Hunter KW Jr.. IFN-gamma primes macrophages for enhanced TNF-alpha expression in response to stimulatory and non-stimulatory amounts of microparticulate beta-glucan. Immunol Lett 2005; 98:115 - 22; http://dx.doi.org/10.1016/j.imlet.2004.10.020; PMID: 15790516
- Berner VK, Sura ME, Hunter KW Jr.. Conjugation of protein antigen to microparticulate beta-glucan from Saccharomyces cerevisiae: a new adjuvant for intradermal and oral immunizations. Appl Microbiol Biotechnol 2008; 80:1053 - 61; http://dx.doi.org/10.1007/s00253-008-1618-8; PMID: 18677470
- Hoffman OA, Olson EJ, Limper AH. Fungal beta-glucans modulate macrophage release of tumor necrosis factor-alpha in response to bacterial lipopolysaccharide. Immunol Lett 1993; 37:19 - 25; http://dx.doi.org/10.1016/0165-2478(93)90127-N; PMID: 8225403
- Abel G, Czop JK. Stimulation of human monocyte beta-glucan receptors by glucan particles induces production of TNF-alpha and IL-1 beta. Int J Immunopharmacol 1992; 14:1363 - 73; http://dx.doi.org/10.1016/0192-0561(92)90007-8; PMID: 1334474
- Huang H, Ostroff GR, Lee CK, Wang JP, Specht CA, Levitz SM. Distinct patterns of dendritic cell cytokine release stimulated by fungal beta-glucans and toll-like receptor agonists. Infect Immun 2009; 77:1774 - 81; http://dx.doi.org/10.1128/IAI.00086-09; PMID: 19273561
- Hurtgen BJ, Hung CY, Ostroff GR, Levitz SM, Cole GT. Construction and evaluation of a novel recombinant T cell epitope-based vaccine against Coccidioidomycosis. Infect Immun 2012; 80:3960 - 74; http://dx.doi.org/10.1128/IAI.00566-12; PMID: 22949556
- Hong F, Hansen RD, Yan J, Allendorf DJ, Baran JT, Ostroff GR, Ross GD. Beta-glucan functions as an adjuvant for monoclonal antibody immunotherapy by recruiting tumoricidal granulocytes as killer cells. Cancer Res 2003; 63:9023 - 31; PMID: 14695221
- Hong F, Yan J, Baran JT, Allendorf DJ, Hansen RD, Ostroff GR, Xing PX, Cheung NK, Ross GD. Mechanism by which orally administered beta-1,3-glucans enhance the tumoricidal activity of antitumor monoclonal antibodies in murine tumor models. J Immunol 2004; 173:797 - 806; PMID: 15240666
- Li B, Cai Y, Qi C, Hansen R, Ding C, Mitchell TC, Yan J. Orally administered particulate beta-glucan modulates tumor-capturing dendritic cells and improves antitumor T-cell responses in cancer. Clin Cancer Res 2010; 16:5153 - 64; http://dx.doi.org/10.1158/1078-0432.CCR-10-0820; PMID: 20855461
- Agarwal S, Specht CA, Haibin H, Ostroff GR, Ram S, Rice PA, Levitz SM. Linkage specificity and role of properdin in activation of the alternative complement pathway by fungal glycans. mBio 2011; 2.
- Huang H, Ostroff GR, Lee CK, Agarwal S, Ram S, Rice PA, Specht CA, Levitz SM. Relative contributions of dectin-1 and complement to immune responses to particulate β-glucans. J Immunol 2012; 189:312 - 7; http://dx.doi.org/10.4049/jimmunol.1200603; PMID: 22649195
- Aouadi M, Tesz GJ, Nicoloro SM, Wang M, Chouinard M, Soto E, Ostroff GR, Czech MP. Orally delivered siRNA targeting macrophage Map4k4 suppresses systemic inflammation. Nature 2009; 458:1180 - 4; http://dx.doi.org/10.1038/nature07774; PMID: 19407801
- Soto ER, Caras AC, Kut LC, Castle MK, Ostroff GR. Glucan particles for macrophage targeted delivery of nanoparticles. J Drug Deliv. 2012;2012:143524.
- Huang H, Ostroff GR, Lee CK, Specht CA, Levitz SM. Robust stimulation of humoral and cellular immune responses following vaccination with antigen-loaded beta-glucan particles. MBio 2010; 1:e00164 - 10; http://dx.doi.org/10.1128/mBio.00164-10; PMID: 20802824
- Tesz GJ, Aouadi M, Prot M, Nicoloro SM, Boutet E, Amano SU, Goller A, Wang M, Guo CA, Salomon WE, et al. Glucan particles for selective delivery of siRNA to phagocytic cells in mice. Biochem J 2011; 436:351 - 62; http://dx.doi.org/10.1042/BJ20110352; PMID: 21418037
- Soto ER, Ostroff GR. Characterization of multilayered nanoparticles encapsulated in yeast cell wall particles for DNA delivery. Bioconjug Chem 2008; 19:840 - 8; http://dx.doi.org/10.1021/bc700329p; PMID: 18376856
- Aouadi M, Tesz GJ, Nicoloro SM, Wang MX, Chouinard M, Soto E, Ostroff GR, Czech MP. Orally delivered siRNA targeting macrophage Map4k4 suppresses systemic inflammation. Nature 2009; 458:1180 - 4; http://dx.doi.org/10.1038/nature07774; PMID: 19407801
- Tesz GJ, Aouadi M, Prot M, Nicoloro SM, Boutet E, Amano SU, Goller A, Wang M, Guo CA, Salomon WE, et al. Glucan particles for selective delivery of siRNA to phagocytic cells in mice. Biochem J 2011; 436:351 - 62; http://dx.doi.org/10.1042/BJ20110352; PMID: 21418037
- Tauseef Ahmad. Michael P. Czech DJB. Uptake and translocation of yeast-derived glucan microparticles across an in vitro M-cell model. In: 37th Annual meeting and exposition of the controlled release society UCD, Ireland, Portland, Oregon, USA, 2010, pp. 40, ed., 2010.
- Beier R, Gebert A. Kinetics of particle uptake in the domes of Peyer’s patches. Am J Physiol 1998; 275:G130 - 7; PMID: 9655693
- Huang H, Ostroff GR, Lee CK, Specht CA, Levitz SM. Characterization and optimization of the glucan particle-based vaccine platform. Clin Vaccine Immunol 2013; 20:1585 - 91; http://dx.doi.org/10.1128/CVI.00463-13; PMID: 23945157
- Franzusoff A, Duke RC, King TH, Lu YN, Rodell TC. Yeasts encoding tumour antigens in cancer immunotherapy. Expert Opin Biol Ther 2005; 5:565 - 75; http://dx.doi.org/10.1517/14712598.5.4.565; PMID: 15934834
- Munson S, Parker J, King TH, Lu Y, Kelley V, Guo Z, Borges V, Franzusoff A. Coupling Innate and Adaptive Immunity with Yeast-Based Cancer Immunotherapy. In: eds R. Orentas JWHaBDJ, ed. Cancer Vaccines and Tumor Immunity: John Wiley & Sons, Inc., Hoboken, NJ, USA., 2007.
- Volman JJ, Ramakers JD, Plat J. Dietary modulation of immune function by beta-glucans. Physiol Behav 2008; 94:276 - 84; http://dx.doi.org/10.1016/j.physbeh.2007.11.045; PMID: 18222501