Abstract
Gene manipulation using Cre-loxP recombination has proven to be an important approach for studying the impact of gene expression on pancreatic β-cell biology. We report the generation of a transgenic mouse line that enables a highly specific system for conditional gene manipulation within β-cells and achieve tissue specific and temporally regulated deletion of the Ctnnb1 (β-catenin) gene in pancreatic β-cells. cDNA encoding Cre recombinase fused to modified estrogen receptor (CreERT) under control of mouse insulin 1 gene promoter (Ins1) was used to construct the mouse line Tg(Ins1-Cre/ERT)1Lphi, also termed MIP1-CreERT. In a cross of MIP1-CreERT with a ROSA26/LacZ reporter strain, tamoxifen [Tmx] - dependent β-galactosidase expression occurred within pancreatic β-cells but not in other organ systems. Intraperitoneal glucose tolerance tests and glucose-stimulated changes in β-cell cytoplasmic calcium concentration were not adversely affected in adult MIP1-CreERT. A mouse line with floxed Ctnnb1 gene (Ctnnb1f/f) was crossed with the MIP1-CreERT line to generate a mouse model for inducible β-cell specific deletion of β-catenin gene (Ctnnb1f/f:MIP1-CreERT). Ctnnb1f/f:MIP1-CreERT mice and Ctnnb1f/f littermate controls, were injected with Tmx as adults to knock down β-catenin production in the majority of pancreatic β-cells. These mice showed normal glucose tolerance, islet cyto-architecture and insulin secretion. A novel protein fraction of 50Kd, immunoreactive with anti-β-catenin was observed in islet extracts from Ctnnb1f/f:MIP1-CreERT[Tmx] mice but not MIP1-CreERT-negative Ctnnb1f/f[Tmx] controls, indicating possible presence of a cryptic protein product of recombined Ctnnb1 gene. The MIP1-CreERT mouse line is a powerful tool for conditional manipulation of gene expression in β-cells.
Introduction
Manipulation of gene expression through Cre/loxP recombination system is a powerful research tool to study the role of gene functionCitation1 and has contributed to understanding of pancreatic β-cell biology and pathophysiology. Expressing the Cre gene under control of a fragment of the rat insulin II promoterCitation2 or a partial Pdx1 gene promoterCitation3-Citation5 proved useful to direct Cre recombinase in mouse β-cells in vivo. On the downside, these promoters also direct Cre expression at various extra-pancreatic locations, including areas of the brain critical for regulation of metabolism.Citation6 To overcome potential limitations of existing approaches, we developed a transgenic mouse line Tg(Ins1-CreERT) (MIP1-CreERT) where the expression of Cre is targeted by β-cells-specific mouse insulin 1 gene promoter (Ins1, MIP1)Citation7,Citation8 and the inducible Cre-fused estrogen receptor (CreERT)-based activation system offers temporal control of Cre activity in the nucleus.Citation9,Citation10 Detailed examination of the resulting transgenic line demonstrated specific expression of CreERT in pancreatic islet β-cells and lack of expression in the brain.Citation11 Here we report a detailed characterization of this β-cell specific MIP1-CreERT mouse line and show that it has normal metabolism and β-cell function. Furthermore, by inactivating the β-catenin gene Ctnnb1 in pancreatic β-cells of adult mice we demonstrate that MIP1-CreERT can be successfully used to manipulate gene activity in pancreatic β-cells.
Despite its potential importance, the impact of Wnt/β-catenin signaling in β-cell function remains unclear. The role of β-catenin in the pancreas was probed in several loss-of-function studies by conditional knockout of the Ctnnb1 geneCitation12-Citation15 timed to early mouse development and involving the whole pancreas. Despite important data gained in these studies, effects of β-catenin knockout on insulin secretion in mature β-cells continue to be inconclusive. Here we attempted a conditional β-catenin deletion in pancreatic β-cells of adult mice, using the mouse line B6.129-Ctnnb1tm2Kem/KnwJ+/+ which contains the floxed gene for β-catenin, Ctnnb1 (Ctnnb1fl/fl) where exons 1 to 6 of the Ctnnb1 gene can be removed by Cre/lox recombination.Citation16 We crossed Ctnnb1fl/fl to MIP1-CreERT mice, thus generating a stable conditional, tissue- and time-specific knockout of β-catenin in pancreatic β-cells that can be activated by series of Tmx injections (Ctnnb1fl/fl:MIP1-CreERT[Tmx]). We then examined the effects of decreased expression of β catenin on glucose tolerance, islet cytoarchitecture and insulin secretion.
Results and Discussion
MIP1-CreERT expression is specific for pancreatic β-cells
An 8.3-kb fragment of mouse Insulin 1 (Ins1) gene promoter (MIP; nucleotides -8280 to +12 relative to the transcriptional start site),Citation7 was placed upstream of recombinant cDNA for Cre recombinase fused to hormone-binding domain of a mutant mouse estrogen receptor, (ERT),Citation10,Citation17 and a 2.1-kb fragment of the human growth hormone gene (hGH), which did not contain any coding DNA but was useful for providing the polyadenylation signal. Founders were identified by PCR of genomic DNA () and CreERT expression was determined by western blot of islet protein extracts (not shown).
Figure 1. Generation and testing of the MIP1-CreERT transgenic mouse. (A) Transgenic construct containing MIP promoter, CreER gene and hGH and agarose gel with genotyping products from negative (1,2,5,7) and positive (3,4,6) founder lines, as well as control DNA (8); Expression of β-galactosidase in the pancreas of ROSA-LacZ:MIP1-CreERT[Tmx]: (B) Frozen sections of the pancreas from ROSA-LacZ:MIP1-CreERT mice injected or not with tamoxifen, were stained for β-galactosidase activity (blue staining); (C) frozen sections of pancreas from ROSA-LacZ:MIP1-CreERT [Tmx] mice (injected with tamoxifen) were stained for β-galactosidase (GREEN), insulin (BLUE) and glucagon (RED) antibodies. Note almost complete overlap of β-galactosidase staining and insulin staining, and the absence of such overlap of β-galactosidase with glucagon staining. Corresponding image of a control islet ROSA-LacZ:MIP1-CreERT[oil] staining for β-galactosidase, insulin and glucagon shows lack of β-galactosidase staining in the islet; (D) MIP1-CreERT transgene expression is specific for pancreatic islets: RT-PCR amplification of Cre in cDNA obtained from various tissues of MIP1-CreERT mice. Densitometry of PCR fragment in an EtBr stained gel (n = 3). Control – actin (not shown).
![Figure 1. Generation and testing of the MIP1-CreERT transgenic mouse. (A) Transgenic construct containing MIP promoter, CreER gene and hGH and agarose gel with genotyping products from negative (1,2,5,7) and positive (3,4,6) founder lines, as well as control DNA (8); Expression of β-galactosidase in the pancreas of ROSA-LacZ:MIP1-CreERT[Tmx]: (B) Frozen sections of the pancreas from ROSA-LacZ:MIP1-CreERT mice injected or not with tamoxifen, were stained for β-galactosidase activity (blue staining); (C) frozen sections of pancreas from ROSA-LacZ:MIP1-CreERT [Tmx] mice (injected with tamoxifen) were stained for β-galactosidase (GREEN), insulin (BLUE) and glucagon (RED) antibodies. Note almost complete overlap of β-galactosidase staining and insulin staining, and the absence of such overlap of β-galactosidase with glucagon staining. Corresponding image of a control islet ROSA-LacZ:MIP1-CreERT[oil] staining for β-galactosidase, insulin and glucagon shows lack of β-galactosidase staining in the islet; (D) MIP1-CreERT transgene expression is specific for pancreatic islets: RT-PCR amplification of Cre in cDNA obtained from various tissues of MIP1-CreERT mice. Densitometry of PCR fragment in an EtBr stained gel (n = 3). Control – actin (not shown).](/cms/asset/e0e4fef0-afac-4f20-887d-5aacd0e4ca89/kisl_a_10927685_f0001.gif)
Tissue specificity of transgene expression was tested by crossing MIP1-CreERT mice with Rosa-LacZ strain and studying the double transgenic ROSA-LacZ:MIP1-CreERT mice, where activation of CreER with tamoxifen [Tmx] is expected to initiate expression of β-galactosidase. In these ROSA-LacZ:MIP1-CreERT[Tmx] mice, β-galactosidase staining was found only in pancreatic islets of Langerhans (), while other tissues studied were negative or not different from non-transgenic controls (brain, liver, fat, skeletal muscle, spleen, lungs, small intestine, large intestine, kidney, heart, eyes, testes, and ovaries; not shown). In our earlier paperCitation11 we provided an extensive probe of the MIP1-CreERT function in the brain of ROSA-LacZ:MIP1-CreERT[Tmx] mice and found that it was not active in the brain, in that it was not able to induce LacZ activation. Therefore, our current findings confirm and extend earlier published data.
Immunofluorescence was used to simultaneously detect β-galactosidase, insulin and glucagon in islets of ROSA-LacZ:MIP1-CreERT[Tmx] mice. Co-localization of anti-insulin and anti-β-galactosidase staining occurred in pancreas sections, while there was no co-localization between anti-β-galactosidase and anti-glucagon antibodies. This confirmed that β-galactosidase staining occurred in islet β-cells but not in α-cells (). At Tmx delivery of 100 mg/kg for 5 consecutive days, with post-injection waiting period of 1 wk, β-galactosidase staining was observed in 78% of islet insulin-positive cells while with 200 mg/kg it was observed in 92% of cells. In double-transgenic ROSA-LacZ:MIP1-CreERT[oil] () or in non-injected (not shown) mice, only 1–3% (avg. 1.69% in males) of β-cells were positive for β-galactosidase activity at 6 mo of age. This indicates that in Tmx-stimulated MIP1-CreERT hemizygotes, the Cre/lox recombination is active in the great majority of β-cells, but the leakiness of the transgene in the absence of Tmx is low.
To detect CreERT transcripts in organs, semi-quantitative RT-PCR was performed on RNA isolated from islets, whole pancreas, brain, liver, fat, skeletal muscle, spleen, lungs, small intestine, large intestine, kidney, heart, eyes, testes, and ovaries with Cre and actin primers (). The extra-pancreatic tissues produced negligible amounts of Cre transcript, which correlates with the lack of positive β-galactosidase staining. Thus, the previously published report showing the absence of Cre activity in the brain of ROSA-LacZ:MIP1-CreERT miceCitation11 was further confirmed.
To detect the function of MIP1-CreERT transgene in embryos, ROSA-LacZ:MIP1-CreERT females were crossed with ROSA-LacZ males. Pregnant females were injected once with 2 mg of Tmx. At P21, Rosa-LacZ:MIP1-CreERT pups demonstrated β-galactosidase expression in pancreatic islets, while ROSA-LacZ littermates did not have such expression (not shown). This confirmed that the activity of CreERT at embryonic stages resided in pancreatic β-cells.
MIP1-CreERT mice have functionally normal β-cells
Purified pancreatic islets from ROSA-LacZ:MIP1-CreERT mice were evaluated for intracellular free Ca2+ dynamics by performing real-time Fura-2 imaging during stimulation with 14 mM glucose () and muscarinic receptor stimulation with carbachol (not shown). Islets from both types of mice displayed the slow type of Ca2+ oscillations. The values for the maximal peak of glucose-stimulated Ca2+ increase and the period of Ca2+ oscillation in islets were not statistically different (3.1 ± 0.7-fold and 5.9 ± 1.6 min for ROSA-LacZ:MIP1-CreERT[Tmx] islets and 3.5 ± 0.7-fold and 6.7 ± 1.8 min for ROSA-LacZ:MIP1-CreERT[oil]; n = 20 islets in 2 separate experiments). After Ca2+ imaging experiments islets were stained for β-galactosidase expression showing that the Cre-directed recombination in the floxed LacZ gene had taken place (). The results show that glucose-stimulated and carbachol-stimulated increases in intracellular Ca2+ in Tmx treated ROSA-LacZ:MIP1-CreERT[Tmx] islets were not different from non-Tmx- treated ROSA-LacZ:MIP1-CreERT controls.
Figure 2. Calcium responses in ROSALacZ:MIP1-CreERT islets. Representative traces show changes in Fura 2 fluorescence, which reflects changes in intracellular calcium in isolated mouse islets: (A) ROSA-LacZ:MIP1-CreERT[oil] males; (B) ROSA-LacZ:MIP1-CreERT[Tmx] males. Period of Ca2+ oscillation in islets was 6.7 ± 1.8 min for [Tmx]-injected and 5.9 ± 1.6 min for [oil]-injected; n = 20 islets in each group; differences in Ca2+ oscillations were not statistically significant P = 0.67). (C) - islets from (A and B) stained for β-galactosidase activity (blue color) to show minimal leakiness of Cre activity in control (ARROW) compared with Tmx-treated mice.
![Figure 2. Calcium responses in ROSALacZ:MIP1-CreERT islets. Representative traces show changes in Fura 2 fluorescence, which reflects changes in intracellular calcium in isolated mouse islets: (A) ROSA-LacZ:MIP1-CreERT[oil] males; (B) ROSA-LacZ:MIP1-CreERT[Tmx] males. Period of Ca2+ oscillation in islets was 6.7 ± 1.8 min for [Tmx]-injected and 5.9 ± 1.6 min for [oil]-injected; n = 20 islets in each group; differences in Ca2+ oscillations were not statistically significant P = 0.67). (C) - islets from (A and B) stained for β-galactosidase activity (blue color) to show minimal leakiness of Cre activity in control (ARROW) compared with Tmx-treated mice.](/cms/asset/53b8f2c3-d157-4e33-be3e-9c7283493a1f/kisl_a_10927685_f0002.gif)
Pancreatic islet structure in transgenic animals was studied by double immunofluorescence with anti-insulin and anti-glucagon antibodies (). At 16 wk of age the pancreatic sections for MIP1-CreERT males (m) and females (f) were analyzed. The results for islet shape aspect ratio, 0.82 ± 0.2 (m) and 0.79 ± 0.2 (f), percentage of β-cells in an islet, 83 ± 4% (m) and 85 ± 2.2% (f), and the percentage of α-cells 14 ± 2.6% (m) and 16 ± 3.5% (f) were compared with those from controls. These features were not statistically different between MIP1-CreERT and the corresponding controls of either gender (corresponding P = 0.6, P = 0.58 and P = 0.45; n = 3 mice for each group, 3 sections scored per mouse). Intraperitoneal glucose tolerance test results (IPGTT) in MIP1-CreERT males and females at 12 wk of age were not significantly different from control wild-type littermates ().
Figure 3. Islet structure and physiological responses in transgenic MIP1-CreERT mice are preserved. Paraffin sections of pancreas were stained with anti-insulin (RED) and anti-glucagon (GREEN) antibodies using double immunofluorescence: (A) female non-transgenic; (B) female MIP1-CreERT+/−; (C) male non-transgenic; (D) male MIP1-CreERT+/−. Nuclei are stained with DAPI (BLUE); size bar in indicates 100µm for all panelsc (A-D). IPGTT performed on MIP1-CreERT+/− and non-transgenic littermate controls at 12 wk of age: (E) males and (F) females from each genotype were recorded separately. Each group consisted of 5–7 mice.
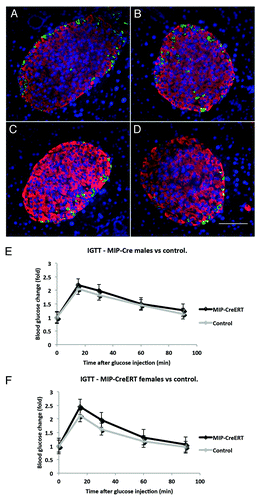
We then determined whether Tmx injection influences glucose metabolism in these transgenic mice. ROSA-LacZ:MIP1-CreERT males and females at 16 wk of age were injected for 5 consecutive days with 2 mg Tmx per day, while control mice were injected with corn oil (). The results of the IPGTT did not show statistically significant difference between these 2 groups of mice.
Figure 4. Tamoxifen [Tmx] injections do not alter glucose tolerance in double-transgenic ROSA-LacZ:MIP1-CreERT mice. IPGTT in 16 wk old ROSA26/LacZ:MIP1-CreERT males and females, before injection with tamoxifen and one week after a round of 5 injections (100mg/kg/day). Each group consisted of 5–7 mice.
![Figure 4. Tamoxifen [Tmx] injections do not alter glucose tolerance in double-transgenic ROSA-LacZ:MIP1-CreERT mice. IPGTT in 16 wk old ROSA26/LacZ:MIP1-CreERT males and females, before injection with tamoxifen and one week after a round of 5 injections (100mg/kg/day). Each group consisted of 5–7 mice.](/cms/asset/a2599f60-b1a2-4843-88f4-10178e2e6d94/kisl_a_10927685_f0004.gif)
MIP1-CreERT mice originally on a CD1 background were crossed for 10 generations to C57Bl/J strain, generating sub-line MIP1-CreERT/C57Bl which retained the ability of the transgene to facilitate Cre/lox recombination in ROSA-LacZ:MIP1-CreERT mice and normal IPGTT. MIP1-CreERT/C57Bl transgenic mice, activated or not, with Tmx, retain the metabolic characteristics of non-transgenic animals. The expression of CreERT was specific to pancreatic β-cells and did not detectably alter their physiology. We conclude that the MIP1-CreERT transgenic mouse line is a useful tool for conditional β-cell recombination in mice.
β-catenin gene knockout with MIP1-CreERT mice
MIP1-CreERT mice were then crossed to strain B6.129-Ctnnb1tm2Kem/KnwJ+/+ (Ctnnb1fl/fl:MIP1-CreERT), which contains loxP sites flanking exons 1–6 of the Ctnnb1 gene. To generate a mouse line with pancreatic β-cell specific knockout of β-catenin gene, Ctnnb1KO, Ctnnb1fl/fl:MIP1-CreERT (and control littermates Ctnnb1fl/fl) at 8 wk of age were injected with 2 mg/day of Tmx for 5 d. Four weeks after the conclusion of injections, Ctnnb1fl/fl:MIP1-CreERT[Tmx] and Ctnnb1fl/fl[Tmx] mice were assessed for islets structure, glucose tolerance, and in vitro insulin secretion from purified pancreatic islets.
Compared with controls, pancreatic sections of Ctnnb1fl/fl:MIP1-CreERT[Tmx] mice consistently demonstrated decreased β-catenin staining within the insulin-positive cell area () suggesting that efficient knockdown of β-catenin took place in β-cells. At the same time, general islet structure, islet size, and cellular composition were not perturbed. β-catenin positive staining was still present in other cell types of Ctnnb1fl/fl:MIP1-CreERT[Tmx] mice such as glucagon-positive α-cells and pancreatic acinar tissue ().
Figure 5. Deleting of Ctnnb1 gene in pancreatic β-cells (βCtnnb1KO) leads to elimination of β-catenin from insulin positive cells in islets of Langerhans. Pancreatic sections stained for - β-catenin (GREEN; A, D), insulin (RED; B, E) and glucagon (BLUE, C, F). Mouse samples: (A, B, C) control (Ctnnb1fl/fl[Tmx]); (D, E, F) double-transgenic Ctnnb1KO, (Ctnnb1fl/fl:MIP1-CreERT[Tmx]). Note the decreased β-catenin staining in the islet area of Ctnnb1KO pancreas despite the preserved β–catenin staining of surrounding acinar tissue. The graph under the images represents intensity of staining along the white line shown in the β-catenin-stained panels. Note the decreased level of β-catenin intensity under the area of insulin staining indicated by red bars.
![Figure 5. Deleting of Ctnnb1 gene in pancreatic β-cells (βCtnnb1KO) leads to elimination of β-catenin from insulin positive cells in islets of Langerhans. Pancreatic sections stained for - β-catenin (GREEN; A, D), insulin (RED; B, E) and glucagon (BLUE, C, F). Mouse samples: (A, B, C) control (Ctnnb1fl/fl[Tmx]); (D, E, F) double-transgenic Ctnnb1KO, (Ctnnb1fl/fl:MIP1-CreERT[Tmx]). Note the decreased β-catenin staining in the islet area of Ctnnb1KO pancreas despite the preserved β–catenin staining of surrounding acinar tissue. The graph under the images represents intensity of staining along the white line shown in the β-catenin-stained panels. Note the decreased level of β-catenin intensity under the area of insulin staining indicated by red bars.](/cms/asset/b1747381-eea5-45eb-8262-4d463b5049bb/kisl_a_10927685_f0005.gif)
Normal structure of the pancreas and similar sizes of islets in Ctnnb1fl/fl:MIP1-CreERT[Tmx] and control mice led us to assume that islets could be purified using conventional methods with collagenase digestion. However, in these isolations islets purified from Ctnnb1fl/fl:MIP1-CreERT[Tmx] mice were consistently smaller than the ones from controls, which could indicate increased breakdown during collagenase treatments of the pancreatic tissue. Staining of the purified islets with anti-β-catenin and anti-insulin antibodies demonstrated extensive elimination of β-catenin from insulin-positive cells (). PCR performed on genomic DNA extracted from islets of Ctnnb1fl/fl:MIP1-CreERT[Tmx] mice indicated that Cre-dependent recombination in β-catenin gene took place, while DNA extracted from tail of the same mice or from control islets did not show such recombination (not shown).
Figure 6. Effects of β-cell specific deletion Ctnnb1KO in mice include decreased expression of β-catenin in islets. Primary pancreatic islets purified from Ctnnb1KO,Ctnnb1fl/fl:MIP1-CreERT[Tmx] double-transgenic mice, and controlCtnnb1fl/fl[Tmx] mice, were stained for insulin (GREEN) and β-catenin (RED).
![Figure 6. Effects of β-cell specific deletion Ctnnb1KO in mice include decreased expression of β-catenin in islets. Primary pancreatic islets purified from Ctnnb1KO,Ctnnb1fl/fl:MIP1-CreERT[Tmx] double-transgenic mice, and controlCtnnb1fl/fl[Tmx] mice, were stained for insulin (GREEN) and β-catenin (RED).](/cms/asset/538a940f-d14e-4e22-a50b-3eb2f29c1b9e/kisl_a_10927685_f0006.gif)
IPGTT demonstrated that there was no significant difference between Ctnnb1fl/fl:MIP1-CreERT[Tmx] and control Ctnnb1fl/fl[Tmx] animals (). In vitro insulin secretion test was performed on islets purified from Ctnnb1fl/fl:MIP1-CreERT[Tmx] mice and Ctnnb1fl/fl[Tmx] control animals. Purified islets were first incubated in KRBH with low glucose concentration followed by high (16.7 mM) glucose and insulin content in the medium was determined with ELISA. The basal insulin secretion at 2.8 mM glucose-containing KRBH normalized for total insulin content was not different in the 2 group of islets (Ctnnb1fl/fl:MIP1-CreERT[Tmx] – 0.08% ± 0.015%; Ctnnb1fl/fl[Tmx] – 0.075% ± 0.025; P > 0.05; n = 5). The secretion Stimulation Index (SI, a ratio of insulin secreted in High glucose to that secreted in Low glucose) was calculated (). There was no statistically significant difference in SI between Ctnnb1fl/fl:MIP1-CreERT[Tmx] mice and Ctnnb1fl/fl[Tmx] control (P > 0.05; n = 5).
Figure 7. Effects of β-cell-specific Ctnnb1 deletion. (A) IPGTT performed 4 wk after TMX injection in male and female double-transgenic Ctnnb1KO, (Ctnnb1fl/fl:MIP1-CreERT[Tmx]) as well as control,Ctnnb1fl/fl[Tmx], show no statistically significant change (n = 5 in each group). (B) Insulin secretion stimulation index (amount of insulin secreted in KRB 16.7mM glucose divided by that secreted in KRB 2.8mM glucose) is unchanged in double-transgenic Ctnnb1KO compared with control littermates. No statistically significant differences between Ctnnb1KO and control mice were detected (n = 5 in each group). (C) western blots of islet extracts from Ctnnb1KO mice and controls stained with β-catenin and actin antibodies show depletion of 90 Kd β-catenin band in the brief exposure of the western blot (TOP PANEL), while longer exposure of the same blot (LOWER PANEL) shows the appearance of additional 50Kd band, as mentioned in the text (representative of 3 independent experiments).
![Figure 7. Effects of β-cell-specific Ctnnb1 deletion. (A) IPGTT performed 4 wk after TMX injection in male and female double-transgenic Ctnnb1KO, (Ctnnb1fl/fl:MIP1-CreERT[Tmx]) as well as control,Ctnnb1fl/fl[Tmx], show no statistically significant change (n = 5 in each group). (B) Insulin secretion stimulation index (amount of insulin secreted in KRB 16.7mM glucose divided by that secreted in KRB 2.8mM glucose) is unchanged in double-transgenic Ctnnb1KO compared with control littermates. No statistically significant differences between Ctnnb1KO and control mice were detected (n = 5 in each group). (C) western blots of islet extracts from Ctnnb1KO mice and controls stained with β-catenin and actin antibodies show depletion of 90 Kd β-catenin band in the brief exposure of the western blot (TOP PANEL), while longer exposure of the same blot (LOWER PANEL) shows the appearance of additional 50Kd band, as mentioned in the text (representative of 3 independent experiments).](/cms/asset/4520ccac-40e8-4573-bd57-d4ff4f296520/kisl_a_10927685_f0007.gif)
Protein extracts of isolated islets were analyzed by western blot with C-terminus-specific (aa 571–581) anti-β-catenin antibody staining (). Quantitation of β-catenin bands normalized to actin control indicated that islets from Ctnnb1fl/fl:MIP1-CreERT[Tmx] mice had only 18 ± 0.6% of full size (90Kd) β-catenin compared with control Ctnnb1fl/fl[Tmx] islets (P < 0.05, n = 3). The resultant β-catenin in these blots could represent the protein originating in non-β-cells of the islets, thus confirming the immunofluorescence data. These results in combination with genomic PCR suggest the successful excision of the “floxed” part of the Ctnnb1 gene took place in pancreatic islet β-cells.
Western blot staining of protein extracts also revealed that an additional β-catenin-specific protein fraction (50Kd) appeared exclusively in islets of Ctnnb1fl/fl:MIP1-CreERT[Tmx] but not in control Ctnnb1fl/fl[Tmx] islets (, lower panel). To confirm that the appearance of the 50Kd band was Tmx dependent, islets from Ctnnb1fl/fl:MIP1-CreERT mice (not previously treated with Tmx) were purified and treated overnight with 4-hydroxytamoxifen (4OH-Tmx; 25nM) or vehicle. After washing off 4OH-Tmx, islets were cultured for 48 h and extracts were studied by western blot. Results confirmed that the 50Kd band appeared exclusively in islets treated with 4OH-Tmx but not in the control group. This is consistent with the hypothesis that the Tmx-dependent expression of the shortened form of β-catenin represents a cryptic protein, which is synthesized after CreERT-dependent recombination at the Ctnnb1 locus. It is thus conceivable that despite the elimination of the majority of β-catenin, a minute quantity of a truncated protein might still be present. It is as yet unknown if the 50Kd band represents an active form of β-catenin.
The results demonstrate that almost complete elimination of β-catenin protein expression in pancreatic β-cells in Ctnnb1fl/fl:MIP1-CreERT(Tmx) mice did not result in a significant alteration of glucose metabolism or insulin secretion. This supports the data from the literature, which indicates that deleting the β-catenin gene early in pancreatic development did not lead to abnormalities of glucose metabolism.Citation12,Citation14 Our data demonstrate for the first time that selective decrease of β-catenin expression in insulin secreting cells of adult mice does not disrupt glucose tolerance in vivo and insulin secretion in vitro. At the same time, the finding of a cryptic form of β-catenin in islets of Ctnnb1fl/fl:MIP1-CreERT[Tmx] mice but not in islets from Ctnnb1fl/fl[Tmx] mice or Ctnnb1fl/fl:MIP1-CreERT mice not treated with Tmx, raises the possibility that functionally the knockout in Ctnnb1fl/fl:MIP1-CreERT[Tmx] mice may be incomplete. This is possible despite significant CreERT-dependent recombination at the Ctnnb1fl/fl locus, which eliminated of the bulk of β-catenin immunoreactivity on sections and western blot. Similar concerns were also expressed by Ahrens et al.Citation18 where these authors demonstrated that the recombined β-catenin locus, such as one in B6.129-Ctnnb1tm2Kem/KnwJ,Citation16 can produce a stable transcript that encodes a truncated 50Kd protein (likely produced using the methionine at aa328, in exon7, as an initiation codon) capable of interfering with diabetes-related transcription factor Tcf4 (Tcf7L2).Citation19 Because of these concerns we could not reach a definitive conclusion on the role of β-catenin in mouse pancreatic β-cells.
At the same time we are able to confidently conclude that the expression of CreERT under the control of the mouse Ins1 promoter is highly specific for pancreatic β-cells and can be efficiently regulated by Tmx injection. Therefore, MIP1-CreERT mouse line is a valuable tool for conditional Cre/loxP recombination in pancreatic β-cells that could be temporally controlled. This mouse tool makes it possible to probe gene expression in pancreatic β-cells at any stage without developmental effects.
Materials and Methods
Generation of Tg(Ins1-cre/ERT)1Lpi [alt. MIP1-CreERT] transgenic mice
The Tg(Ins1-cre/ERT) transgene () was assembled using an 8.3-kb fragment of mouse insulin I promoter (Ins1, MIP1; nucleotides -8,280 to +12 relative to the transcriptional start site),Citation7 a cDNA for Cre recombinase fused to hormone-binding domain of a mutant mouse estrogen receptor, ERT (provided by Dr A. McMahon),Citation10,Citation17 and a 2.1-kb fragment of the human growth hormone gene (hGH), which did not contain any coding DNA but was useful for transcription stop signal. The resulting 12.5-kb Tg(Ins1-cre/ERT) transgene was isolated from the vector by digestion with AscI and Sfi I, purified by agarose gel electrophoresis, and microinjected into the pronuclei of fertilized oocytes from CD1 mice by the Transgenic Mouse/ES Core Laboratory of the University of Chicago Diabetes Research and Training Center. Tail DNA was used to determine the presence of the transgene by PCR using forward and reverse primers 5′- CCTGGCGATCCCTGAACATGTCCT-3′ and 5′-TGGACTATAAAGCTGGTGGGCAT-3′, respectively (). The resulting transgenic mouse line was termed Tg(Ins1-cre/ERT)1Lphi, and is also known as MIP1-CreERT. The MIP1-CreERT line was further maintained with heterozygote breeding, but homozygotes are viable and reproduce successfully. No specific phenotypes associated with homozygous state were noted. Transgenic mice were housed under pathogen-free conditions with free access to food and water. Procedures involving mice were approved by the University of Chicago Institutional Animal Care and Use Committee. The transgenic mouse line Tg(Ins1-cre/ERT)1Lphi [alt. MIP1-CreERT] will be available to the research community.
Characterization of transgene expression
To assess the tissue distribution of MIP1-CreERT expression, MIP1-CreERT founder mice were crossed with ROSA26-LacZ reporter miceCitation20 obtained from Jackson Laboratory. Expression of the transgene in ROSA-LacZ+/−:MIP1-CreERT+/− (ROSA-LacZ:MIP1-CreERT) mice was assessed following intraperitoneal injections of tamoxifen (Tmx; Sigma-Aldrich Co.) in corn oil (100 mg/kg or 200 mg/kg, once per day for 5 consecutive days). In the text, mice injected with tamoxifen are indicated with adding [Tmx] to their name. Negative controls were ROSA-LacZ[Tmx] mice or ROSA-LacZ:MIP1-CreERT[oil], which received just injections of corn oil. Mice were sacrificed 1 wk after the last injection unless stated otherwise. Following euthanasia, mouse organs were dissected, fixed with 2% paraformaldehyde/0.2% glutaraldehyde for 10 min and stained for lacZ activity.Citation20 For identification of lacZ activity in specific cells, dissected tissues stained with X-Gal were post-fixed with 4% paraformaldehyde for 1 h, washed with 30% sucrose in phosphate-buffered saline, embedded in OCT TissueTek compound (Sakura), frozen, and sectioned using a cryostat. First strand cDNA synthesis for RT-PCR was performed from 1 µg total RNA from a variety of organs using Superscript III (Invitrogen). Thirty cycles of PCR from 1/10 of the cDNA was performed with primers for Cre recombinase (for – 5′-ACGCTGGTTAGCACCGCAGG-3′; rev – 5′-AGATGGCGCGGCAACACCATT-3′) and actin (for- 5′-CACGGTTGGCCTTAGGGTTCAG-3′, rev 5′-GCTGTATTCCCCTCCATCGTG-3′). The products were quantified after gel electrophoresis using ChemiDoc software (BioRad). Immunohistochemistry was performed on frozen or paraffin embedded tissue sections with anti-Cre, anti-β-galactosidase, anti-glucagon and anti-insulin antibodies (Millipore). Fluorescent donkey anti-Guinea pig and anti-Rabbit secondary antibodies were from (Jackson Immunoresearch). Microscopy was performed using Leica SP5 laser scanning confocal system with 63x objective. For islet structure analysis, 3 sections per mouse in 3 mice were analyzed. Aspect ratio was calculated for islets containing 50 or more cells. Image analysis was performed with Image J software (NIH).
Glucose tolerance tests
Glucose tolerance test on mice injected with tamoxifen was performed after 3 wk post-injection. Mice were fasted for 4 h with full access to water and intraperitoneal glucose tolerance tests were conducted as described.Citation21 Mice were injected intraperitoneally with 2 mg of glucose per g body weight and blood glucose concentration was measured 0, 15, 30, 60, and 90 min with a FreeStyle glucometer (Abbott Diabetes Care Inc.). Statistical analysis was performed using Student t test.
Measurement of cytoplasmic Ca2+ concentration
Islets of Langerhans were purified and maintained in culture as described.Citation22 For imaging dynamic changes in cytoplasmic Ca2+ concentration ([Ca2+]c), islets were loaded with Fura-2-acetoxymethyl ester (Invitrogen) and ratiometric dual-wavelength excitation microspectrophotometry conducted using a Nikon TE-2000U microscope equipped for epifluorescence with 10x objective (Nikon).Citation23 Islets were continuously superfused with a standard Krebs-Ringer buffer (KRB) and stimulated by increasing the concentration of D-glucose in the superfusate from 2 to 14 mM or with 100 µM carbachol containing solution. [Ca2+]c is expressed as the change in the ratio of Fura-2 fluorescence emission following excitation at 340 nm and 380 nm (R340/380).
Deletion of β-catenin gene in double-transgenic mice
MIP1-CreERT +/− were crossed with homozygous strain B6.129-Ctnnb1tm2Kem/KnwJ+/+Citation16 (Jackson Labs), which contains insertions of loxP within β-catenin gene (“floxed”). Genotyping primers β-cat For - AAG GTA GAG TGA TGA AAG TTG TT and β-cat Rev - CAC CAT GTC CTC TGT CTA TTC were used for PCR of genomic DNA. Anti-β catenin (Immunogen: Mouse β-Catenin aa. 571–781 at the C-terminus of β catenin; Cat. 610154; BD Biosciences), anti-actin (Roche) and donkey anti-mouse HRP-labeled (Jackson Immunoresearch) antibodies were used for protein detection by western blot. ChemiDoc software (BioRad) was used for quantitation of chemiluminescent signals on western blots. Insulin secretion was measured after static incubation in KRB with either 2.8 mM glucose or 16.7 mM glucose; insulin content was measured with Ultrasensitive Mouse Insulin ELISA (ALPCO).
Statistics
Statistical analysis was performed using Student t test and data presented as mean ± SEM.
Disclosure of Potential Conflicts of Interest
No potential conflicts of interest were disclosed.
Acknowledgments
We are grateful to Drs A McMahon, C Lobe, and M Hara for plasmids, to Felipe Mendez and Olivia Cochet for excellent technical assistance and to Drs Graeme Bell and Donald Steiner for helpful discussions. Institution at which the work was performed: University of Chicago; Chicago IL, USA.
Grant Support
DK063493 (L.H.P.), DK48494 (L.H.P.), DK074966 (M.W.R.), DK092616 (M.W.R.), and P60DK020595 from the National Institute of Diabetes and Digestive and Kidney Diseases.
References
- Mallo M. Controlled gene activation and inactivation in the mouse. Front Biosci 2006; 11:313 - 27; http://dx.doi.org/10.2741/1799; PMID: 16146733
- Postic C, Shiota M, Niswender KD, Jetton TL, Chen Y, Moates JM, Shelton KD, Lindner J, Cherrington AD, Magnuson MA. Dual roles for glucokinase in glucose homeostasis as determined by liver and pancreatic beta cell-specific gene knock-outs using Cre recombinase. J Biol Chem 1999; 274:305 - 15; http://dx.doi.org/10.1074/jbc.274.1.305; PMID: 9867845
- Zhang H, Fujitani Y, Wright CV, Gannon M. Efficient recombination in pancreatic islets by a tamoxifen-inducible Cre-recombinase. Genesis 2005; 42:210 - 7; http://dx.doi.org/10.1002/gene.20137; PMID: 15986486
- Gu G, Dubauskaite J, Melton DA. Direct evidence for the pancreatic lineage: NGN3+ cells are islet progenitors and are distinct from duct progenitors. Development 2002; 129:2447 - 57; PMID: 11973276
- Wiebe PO, Kormish JD, Roper VT, Fujitani Y, Alston NI, Zaret KS, Wright CV, Stein RW, Gannon M. Ptf1a binds to and activates area III, a highly conserved region of the Pdx1 promoter that mediates early pancreas-wide Pdx1 expression. Mol Cell Biol 2007; 27:4093 - 104; http://dx.doi.org/10.1128/MCB.01978-06; PMID: 17403901
- Mori H, Inoki K, Münzberg H, Opland D, Faouzi M, Villanueva EC, Ikenoue T, Kwiatkowski D, MacDougald OA, Myers MG Jr., et al. Critical role for hypothalamic mTOR activity in energy balance. Cell Metab 2009; 9:362 - 74; http://dx.doi.org/10.1016/j.cmet.2009.03.005; PMID: 19356717
- Hara M, Wang X, Kawamura T, Bindokas VP, Dizon RF, Alcoser SY, Magnuson MA, Bell GI. Transgenic mice with green fluorescent protein-labeled pancreatic beta -cells. Am J Physiol Endocrinol Metab 2003; 284:E177 - 83; PMID: 12388130
- Park SY, Wang X, Chen Z, Powers AC, Magnuson MA, Head WS, Piston DW, Bell GI. Optical imaging of pancreatic beta cells in living mice expressing a mouse insulin I promoter-firefly luciferase transgene. Genesis 2005; 43:80 - 6; http://dx.doi.org/10.1002/gene.20157; PMID: 16108006
- Feil R, Brocard J, Mascrez B, LeMeur M, Metzger D, Chambon P. Ligand-activated site-specific recombination in mice. Proc Natl Acad Sci U S A 1996; 93:10887 - 90; http://dx.doi.org/10.1073/pnas.93.20.10887; PMID: 8855277
- Hayashi S, McMahon AP. Efficient recombination in diverse tissues by a tamoxifen-inducible form of Cre: a tool for temporally regulated gene activation/inactivation in the mouse. Dev Biol 2002; 244:305 - 18; http://dx.doi.org/10.1006/dbio.2002.0597; PMID: 11944939
- Wicksteed B, Brissova M, Yan W, Opland DM, Plank JL, Reinert RB, Dickson LM, Tamarina NA, Philipson LH, Shostak A, et al. Conditional gene targeting in mouse pancreatic ß-Cells: analysis of ectopic Cre transgene expression in the brain. Diabetes 2010; 59:3090 - 8; http://dx.doi.org/10.2337/db10-0624; PMID: 20802254
- Dessimoz J, Bonnard C, Huelsken J, Grapin-Botton A. Pancreas-specific deletion of beta-catenin reveals Wnt-dependent and Wnt-independent functions during development. Curr Biol 2005; 15:1677 - 83; http://dx.doi.org/10.1016/j.cub.2005.08.037; PMID: 16169491
- Dabernat S, Secrest P, Peuchant E, Moreau-Gaudry F, Dubus P, Sarvetnick N. Lack of beta-catenin in early life induces abnormal glucose homeostasis in mice. Diabetologia 2009; 52:1608 - 17; http://dx.doi.org/10.1007/s00125-009-1411-y; PMID: 19513688
- Murtaugh LC, Law AC, Dor Y, Melton DA. Beta-catenin is essential for pancreatic acinar but not islet development. Development 2005; 132:4663 - 74; http://dx.doi.org/10.1242/dev.02063; PMID: 16192304
- Rulifson IC, Karnik SK, Heiser PW, ten Berge D, Chen H, Gu X, Taketo MM, Nusse R, Hebrok M, Kim SK. Wnt signaling regulates pancreatic beta cell proliferation. Proc Natl Acad Sci U S A 2007; 104:6247 - 52; http://dx.doi.org/10.1073/pnas.0701509104; PMID: 17404238
- Brault V, Moore R, Kutsch S, Ishibashi M, Rowitch DH, McMahon AP, Sommer L, Boussadia O, Kemler R. Inactivation of the beta-catenin gene by Wnt1-Cre-mediated deletion results in dramatic brain malformation and failure of craniofacial development. Development 2001; 128:1253 - 64; PMID: 11262227
- Danielian PS, Muccino D, Rowitch DH, Michael SK, McMahon AP. Modification of gene activity in mouse embryos in utero by a tamoxifen-inducible form of Cre recombinase. Curr Biol 1998; 8:1323 - 6; http://dx.doi.org/10.1016/S0960-9822(07)00562-3; PMID: 9843687
- Ahrens MJ, Romereim S, Dudley AT. A re-evaluation of two key reagents for in vivo studies of Wnt signaling. Dev Dyn 2011; 240:2060 - 8; http://dx.doi.org/10.1002/dvdy.22704; PMID: 21793100
- Peng S, Zhu Y, Lü B, Xu F, Li X, Lai M. TCF7L2 gene polymorphisms and type 2 diabetes risk: a comprehensive and updated meta-analysis involving 121,174 subjects. Mutagenesis 2013; 28:25 - 37; http://dx.doi.org/10.1093/mutage/ges048; PMID: 23188737
- Soriano P. Generalized lacZ expression with the ROSA26 Cre reporter strain. Nat Genet 1999; 21:70 - 1; http://dx.doi.org/10.1038/5007; PMID: 9916792
- Marshall BA, Tordjman K, Host HH, Ensor NJ, Kwon G, Marshall CA, Coleman T, McDaniel ML, Semenkovich CF. Relative hypoglycemia and hyperinsulinemia in mice with heterozygous lipoprotein lipase (LPL) deficiency. Islet LPL regulates insulin secretion. J Biol Chem 1999; 274:27426 - 32; http://dx.doi.org/10.1074/jbc.274.39.27426; PMID: 10488074
- Roe MW, Worley JF 3rd, Qian F, Tamarina N, Mittal AA, Dralyuk F, Blair NT, Mertz RJ, Philipson LH, Dukes ID. Characterization of a Ca2+ release-activated nonselective cation current regulating membrane potential and [Ca2+]i oscillations in transgenically derived beta-cells. J Biol Chem 1998; 273:10402 - 10; http://dx.doi.org/10.1074/jbc.273.17.10402; PMID: 9553098
- Tamarina NA, Kuznetsov A, Rhodes CJ, Bindokas VP, Philipson LH. Inositol (1,4,5)-trisphosphate dynamics and intracellular calcium oscillations in pancreatic beta-cells. Diabetes 2005; 54:3073 - 81; http://dx.doi.org/10.2337/diabetes.54.11.3073; PMID: 16249428