Abstract
Drug development from early discovery to late stage commercialization is a long arduous process where a number of factors are taken into consideration when deciding on a particular immunoglobulin isotype for a therapeutic purpose. There are no general rules for which isotype is selected; however, prior experiences, effector function and the specific therapy targeted, as well as extensive testing early in development help in paring the number of candidates. Over 20 monoclonal antibodies are FDA-approved, and most are IgG1 isotype, although a number of non-IgG1 molecules have been approved recently and the number in development is on the rise. Analytical techniques that examine the physicochemical properties of a molecule provide vital information on the stability and efficacy of candidate antibody therapeutics, but most of these studies are conducted using standard buffers and under well defined storage conditions. It has recently become apparent that analysis of antibody therapeutics recovered after circulation in blood show altered physicochemical characteristics, and in many instances therapeutic molecules recovered from serum show lower potency. This review examines some of these studies, with a focus on the physicochemical changes observed in the molecules. Technologies that can facilitate rapid screening of candidate antibody therapeutics directly from blood are highlighted. The facts indicate that antibody therapeutic development programs must incorporate understanding of the basic biology of the isotype and its stability in serum, which is the intended environment of the therapeutic.
Introduction
Antibodies (immunoglobulins, Ig) have emerged as an important class of therapeutics in oncology, chronic inflammation, cardiovascular, transplantation and infectious diseases. To date, over 20 antibody therapeutics have been approved, with numerous others at various stages of development.Citation1–Citation4 Antibodies are attractive as therapeutics due to their specificity and safety. This is reflected in their relatively high approval success rate (∼25% for humanized antibodies) compared with the ∼11% success rate for small molecule development.Citation4–Citation6 Other advantages of antibodies, in general, are that they are well tolerated, and the knowledge and experience gained from one antibody during development, manufacturing and clinical use has the potential to be readily transferred to other therapeutics in the pipeline of an organization.Citation4,Citation7 Two major disadvantages of antibody therapeutics are that targets are restricted to molecules in circulation or those expressed on the surface of cells and they are expensive, primarily due to high doses needed for treatments.Citation4,Citation8
The structure of antibodies, with complete amino acid content and disulfide bond pairing, was elucidated by Gerald Edelman and Rodney Porter in the early 1960s, and they were awarded the Nobel Prize in 1972 for this work.Citation9–Citation11 The Ig monomer is composed of two identical heavy chains (HC) and two identical light chains (LC) that are linked by disulfide bonds (inter-chain disulfides). Each of the HC and LC contain structural domains (Ig domains) that resemble immunoglobulin folds composed of two beta sheets linked by cysteine residues (intra-chain disulfides); the Ig domains are further classified into variable (V) or constant (C) domains depending on structure and function.Citation12,Citation13
There are five classes of immunoglobulins that are classified on the basis of the constant region of the heavy chain; they are IgA, IgD, IgE, IgG and IgM. The constant heavy region of IgA, IgD and IgG has three Ig domains and a hinge region to provide flexibility; whereas, the constant regions of IgE and IgM has four Ig domains. The IgG and IgA classes are further classified into six isotypes (IgG1, IgG2, IgG3, IgG4, IgA1 and IgA2). Despite the vast choice of immunoglobulin types to select for development of a therapeutic antibody, most antibody engineers have focused on the IgG class, although the IgG3 class is not used as a therapeutic candidate because it has a shorter half-life, a long hinge region that is easily accessible to proteolysis, and allotypic polymorphism.Citation1,Citation4,Citation5,Citation14
The intra-chain disulfides of the HC and LC immunoglobulin domains for the four IgG isotypes are similar; however, the inter-chain disulfide brides are different (see ). Differences in the inter-chain disulfide bridges between the HC is brought about by the amino acid composition of the hinge region and the number and position of the cysteine residues (). Both IgG1 and IgG4 have two disulfide linkages between their HC; whereas, IgG2 and IgG3 have four and eleven, respectively. The inter-chain linkage between the HC and LC of IgG1 is between the C-terminal cysteine residue of the LC and the first cysteine residue in the hinge region; whereas, the inter-chain linkage between the HC and LC of IgG2, IgG3 and IgG4 is between the terminal cysteine residue of the LC and the cysteine residue in Fab region (N-terminal of the CH1 domain).Citation15–Citation22
Protein therapeutics generally have a long half-life in blood and studies have revealed that the environment in blood can have a profound influence on the physicochemical properties of the molecule. Blood is composed of many types of cells, proteins and salts.Citation23 When the cells are removed without clotting, the liquid portion left behind is plasma; however, if cells are removed in the absence of anti-coagulants, the liquid portion is then called serum. Serum differs from plasma in that fibrin, as well as proteins that associate with fibrin, are removed; serum is estimated to have about 3–4% less protein than plasma.
Plasma typically contains about 22 proteins that make up 99% of the total protein content—they include albumin, total IgG, transferrin, fibrinogen, total IgA, alpha-2-macroglobulin, total IgM, alpha-1-anti-trypsin, C3 complement, haptoglobulin, alpha-1-acid glycoprotein, apolipoprotein-B, apolipoprotein-A1, lipoprotein (a), factor H, ceruloplasmin, C4 complement, complement factor B, pre-albumin, C9 complement, C1q complement and C8 complement.Citation23–Citation25 The remaining 1% of plasma is composed of hundreds of proteins that have been identified after removal of the most abundant proteins, using columns from Agilent, Sigma or GenWay Biotech, prior to MS analysis.Citation11,Citation23,Citation26–Citation28 Serum proteins thus present an extremely “crowded” environment where the excluded volume results in antibody therapeutics adopting a compact structure, as well as showing enhanced association with antigen in serum.Citation29 High levels of albumin, cysteine, cystine, glutathione, homocysteine and other small thiols also present a “redox” environment, outside the endoplasmic reticulum (ER), that facilitates rearrangement of disulfide bonds in antibody therapeutics recovered from serum.Citation30–Citation36 In circulation, the temperature of blood is at 37°C and antibody therapeutics are exposed for various lengths of time to receptors, as well as enzymes such as mannosidases. Due to these factors, serum has influences on the primary, secondary and higher order structures of antibody therapeutics.
Influence in Serum on Primary Structure of mAbs
Deamidation of asparagine residues in serum.
Deamidation of asparagine, a non-enzymatic process, is a common post-translational modification (PTM) seen in protein therapeutics and is considered one of its major degradation pathways. Deamidation of asparagine is spontaneous and takes place during storage or sample handling. Due to steric exposure, asparagine residues, followed by glycines (NG sites) in flexible regions of a molecule, are found to be most susceptible to deamidation; the first step involves a nucleophilic attack of an α-amino group, from the peptide bond, on the side chain carbonyl group of the asparagine residue, resulting in a cyclic succinimide intermediate (). The succinimide intermediate is then hydrolyzed at either the alpha or beta carbonyl group to give either aspartate or isoaspartate (beta aspartate) respectively; hydrolysis of the beta carbonyl linkage is generally favored due to the asymmetry of the intermediate.Citation37,Citation38 The Fc domain of the IgG1 and IgG2 isotypes share about 97% sequence homology.Citation39 Comparison of the rates of deamidation of 2 NG sites (Asn-315 and Asn-384) in the Fc domain revealed a slower rate of deamidation at Asn-315 (0.03% per day) compared to Asn-384 (0.63% per day).Citation40 An inspection of the two sites reveal the influence of secondary structure as hydrogen bonding of Gly-316 to Asp-312 reduced flexibility of the local region, thereby influencing the rate of deamidation of Asn-315.
A comprehensive analysis of sites of deamidation in murine mAbs (IgG1 and IgG2a) and recombinant human mAbs reveal that asparagine residues in the CH2 domain (WLN*GK) and CH3 domain WESN*GQPEN*NY were consistently found to undergo deamidation.Citation41–Citation43 Deamidation of asparagine residues in the CDR region was also observed in many of the molecules studied. The introduction of a net negative charge via deamidation is detrimental in many instances to protein function, as well as the pharmacokinetics (PK) of the molecule.Citation44 Loss of activity due to deamidation has been reported for two antibody therapeutics when it occurred in the CDR region.Citation44,Citation45 Other evidence links isoaspartyl PTM to altered immunogenicity.Citation46,Citation47 Consequently, long term stability studies at the recommended storage conditions of the protein therapeutic, as well as stressed stability studies done typically with different formulations, are extensively employed by the biotechnology industry to predict the molecule’s capacity to undergo deamidation, monitor its loss of efficacy and demonstrate process consistency.Citation48–Citation51 Deamidation is monitored by a variety of techniques since net negative charge as well as hydrophobicity of the molecule is altered and protein mass is changed (addition of 1 Da). Analytical techniques used to detect deamidation include capillary isoelectric focusing (cIEF) and ion exchange, which both measure change in charge, reverse phase chromatography measuring change in hydrophobicity and mass spectrometry measuring change in mass. An enzymatic assay that uses protein isoaspartyl methyltransferase (PIMT) is also frequently used for detecting isoaspartic residues.
Therapeutic antibodies tend to circulate in the blood for many days, or in some instances weeks, thus exposing the molecule to deamidation.Citation44 It is argued that the instability induced by deamidation of endogenous proteins in serum is a normal biological process that serves as a molecular clock.Citation37,Citation52–Citation55 However, deamidation of antibody therapeutics in vivo raise concerns about both loss in activity and the potential immunogenicity of the therapeutic. Thus, PK information for the antibody therapeutic and an estimate of the kinetics of deamidation for the different asparagine residues in serum are important for assessing the exposure of patients to the PTM and establishing criticality of this attribute, which has additional consequences concerning monitoring and controlling levels of the PTM.
For in vivo studies carried out with human therapeutic antibodies, the antibody is recovered from human serum using cross-liked antigen.Citation40 For studies carried out in monkey or other animals, serum recovery of the antibody was either recovered via cross-linked antigen or an anti-human Fcγ antibody.Citation44 The purified antibody is subsequently digested and peptides analyzed by LC/MS/MS. Extracted ion chromatograms for each asparagine-containing peptide are created to monitor the deamidation rates of Asn residues; the half-life for deamidation of different residues in the molecule can then be obtained. In vivo studies on antibody therapeutics have shown that rates of deamidation are independent of the administration route and deamidation in the Fc region of antibodies occurs naturally; both IgG1 and IgG2 are believed to undergo the same rates of deamidation.Citation40,Citation44
Influence of methionine oxidation on serum half-life.
Oxidation of therapeutic proteins can take place on a number of different amino acid residues in the molecule, although methionine residues exposed to solvent tend to be the most susceptible.Citation39,Citation56–Citation58 The end product of methionine oxidation is frequently methionine sulfoxide, which increases mass by 16 Da and makes the molecule more polar and less hydrophobic. Technologies that allow for detection of oxidation include hydrophobic interaction chromatography (HIC), where oxidized species are found to elute earlier that the non-oxidized species; weak cation exchange chromatography (WCX-10), where oxidized species elute later than non-oxidized species; and peptide mapping, where peptides containing oxidized methionine residues elute earlier than non-oxidized methonine residues when separated on a reverse phase (RP) column.Citation42,Citation57,Citation59,Citation60
Oxidation of therapeutic proteins can occur during the cell-culture process, purification, formulation and storage of the molecules, and studies reveal that oxidation of methionine residues not only results in loss of activity, but also reduced stability of the molecule, potential immunogenicity and decreased in vivo half-life.Citation56,Citation59–Citation62 IgG1 and IgG2 molecules share considerable sequence homology (97%) in the Fc potion of the molecule and a number of studies using chemical oxidation, ultraviolet light, or elevated temperatures have shown that two solvent exposed methionine residues in the CH2 domain (Met-252) and CH3 domain (Met-428) are highly susceptible to oxidation compared to other methionine residues in the molecule.Citation39,Citation42,Citation56,Citation58 Further, NMR analysis revealed that oxidation of Met-252 and Met-428 introduced structural changes in regions of the IgG molecule involved in binding to the neonatal receptor (FcRn), e.g., Met-428 interacts with residues on FcRn that are important for their pH dependent binding.Citation58,Citation63,Citation64 In fact, two studies confirmed that oxidation of methionine residues in the Fc region decreased its affinity for the FcRn receptor.Citation39,Citation58 The FcRn receptor has an established role in maintenance of antibody half-life, and decreased binding of an IgG molecule to FcRn translates into a shorter half-life in serum.Citation58,Citation65–Citation67 Oxidation of methionine residues in the Fc region of an IgG molecule in serum can potentially have a significant impact on its half-life.
Influence of Fc glycan forms on clearance in serum.
Glycosylation of recombinant protein drugs is closely monitored by the biotechnology industry because receptors for specific glycan structures are known to bind and rapidly clear these glycans from circulation. For example, studies have shown clearance of glycoproteins via two well-known receptors, the asialoglycoprotein receptor, which binds terminal galactose residues, and the mannose receptor, which binds terminal mannose and N-acetyl-glucosamine.Citation68–Citation73 The Fc glycans present on human antibodies comprise an N-linked oligosaccharide (Asn-297) that shows considerable heterogeneity attributable to 32 oligosaccharides that can give rise to over 400 glycoform species as a result of random pairing of the heavy chains.Citation74 Antibodies manufactured by recombinant DNA technology and expressed in CHO cells show similar oligosaccharide profiles compared with those found in normal human IgG molecules. The major species typically reported e.g., the biantennary fucosylated species with 0, 1 or 2 terminal galactose residues (NA1F, NA2F, NGA2F, NGA2F-GlcNAc, NA1F-GlcNAc) and the high mannose non-fucosylated species (Mannose 5, 6, 7) are shown in .
Fc glycans are partially buried within the CH2 domain and are believed to be not easily accessible to asialoglycoprotein and mannose receptors.Citation75 A number of studies have attempted to study the impact of Fc glycans on clearance. In these studies, Fc glycans are either enriched by a variety of techniques, e.g., affinity purification, use of inhibitors, genetic mutation or enzymatic manipulation, or the entire glycan profile of the therapeutic antibody is studied after administration.Citation76–Citation82 The major advantage of the latter method is that the glycan and antibody are typically very well-characterized prior to administration. Further, these studies are not limited to animals, and in many instances clinical PK studies facilitate analysis of human antibodies in human serum. The therapeutic antibody is recovered from serum via cross-linked antigen or using anti-Fc gamma antibodies. Various analytical techniques can then be used to evaluate the different glycans; the most common are fluorescent or LC/MS methods.Citation75,Citation82
summarizes some of the major studies performed and the conclusions on the impact of Fc glycans on clearance, which are conflicting. An important study by Chen et al. conducted on a recombinant human antibody in human serum, uncover circulating mannosidases in serum that trim high mannose species (M6–M9).Citation75 The major conclusion of this study is that Fc glycan have no impact on clearance. In contrast, in studies where mannosidases in serum were carefully evaluated, oligomannoses in a human IgG1 antibody are preferentially cleared from human serum and the fucosylated biantennary oligosaccharides were unaffected (Correia IR, Alessandri L, Ouellette D, Piparia P, Aikhoje A, Radziejewski C, et al; unpublished results). In summary, the effects of Fc glycan structure on clearance for different therapeutic antibodies should be evaluated on a case by case basis, preferably during clinical PK studies. These studies are relevant not only for clinical justification for setting glycan specifications, but they also establish bioequivalence for the therapeutic, especially if a particular glycan is preferentially cleared from serum.
Influence in Serum on Secondary Structure of mAbs
Disulfide bond formation is an important post-translation event that occurs in the ER with the aid of many chaperones and enzymes such as protein disulfide isomerase (PDI). The primary function of the disulfide bonds is to ensure proper folding and activity of the protein; however, the dissociation energy of a disulfide bond is about 40% less than C-C and C-H bonds and is generally viewed as a “weak link” in a protein. Incorrect disulfide bond assembly and reduction of the bonds are both known to affect the antigen-binding capacity of an antibody, and lead to reduced activity.Citation83,Citation84 The redox environment in serum is due to cysteine/cystine levels, albumin and thiols such as glutathione, and homocysteine.Citation30–Citation36 Dynamic re-arrangement of disulfide bonds in serum, facilitated by the thiols, have been reported for both IgG2 and IgG4 antibodies and the general consensus is that the rearrangements serve as a mechanism for dampening the activity of these molecules; “allosteric” disulfide bonds may be another method by which protein function is regulated.Citation1,Citation85,Citation86
IgG4 in serum (Fab exchange).
Effector functions for the different IgG classes are directly correlated with their affinity for the Fc receptor, with IgG1>IgG3>IgG4>IgG2.Citation1,Citation4,Citation63 In addition, IgG4 molecules have a low affinity for C1q; thus, recombinant IgG4 molecules have emerged as an important therapeutic class of molecules when recruitment of the host effector function is not desirable. Natalizumab (Tysabri) and gemtuzumab ozogamicin (Mylotarg) are both IgG4 molecules that are currently marketed for multiple sclerosis (MS) and acute myeloid leukemia (AML), respectively.Citation1,Citation4 TGN1412 another IgG4 molecule was discontinued after showing side-effects in a Phase 1 study of healthy volunteers.
IgG4 is a tetrameric molecule (H2L2) with two hinge region cysteines (). Although structurally similar to IgG1, significant levels of IgG4 half-antibodies, composed of HL dimers of IgG4, can be detected by a variety of techniques.Citation85,Citation87,Citation88 Evidence now links the wild type sequence (CPSC) in the hinge region of IgG4 molecules to unfavorable inter-chain disulfide bond formation. Mutations, e.g., from CPSC to CPPC (), greatly reduce formation of half-antibody molecules.Citation89–Citation91 The CH3 domain of the IgG4 molecule is believed to also play a significant role in promoting half-antibody formation.Citation92
Fab arm exchanges, where half-antibodies from one endogenous IgG4 molecule exchange with that of another endogenous molecule resulting in formation of bispecific antibodies, is postulated to be a normal biological characteristic of this class of anti-inflammatory molecules that may serve a protective role.Citation1,Citation85,Citation92 However, exchange of Fab arms between a therapeutic antibody and endogenous IgG4 molecules may be highly undesirable (). In an elegant study, Labrijn et al. found that natalizumab, which contains wild type sequence in the hinge region, exchanged its Fab arms with endogenous IgG4 molecules in individuals treated with the drug. In contrast, the stabilizing mutation is incorporated in gemtuzumab ozogamicin and the molecule did not exchange its Fab arms either in vitro or in studies conducted in mice. The authors also show Fab exchange between TGN1412, which contains wild type sequence, and endogenous IgG4 molecules.Citation93 The use of therapeutic IgG4 mAbs in which Fab exchange can take place has profound implications potentially relating not only to adverse events associated with the therapeutics, but also the pharmacokinetic and pharmacodynamic properties of the molecules. Stabilizing mutations in the hinge region or the CH3 domain will facilitate development of the IgG4 isotypes as therapeutics.
IgG2 in serum (disulfide shuffling).
Several IgG2 molecules are either in late stage development (tanezumab, figitumumab, denosumab) or approved for commercial use (panitumumab). Like IgG4, this class of therapeutic antibodies is selected for development when neutralization of soluble antigens with reduced effector function is required. IgG2 molecules are unique in that fully functional covalent dimers can be detected both in recombinant antibodies secreted by myeloma cells, as well in sera of normal donors.Citation94 Intermolecular linkages between the dimers are depicted in ; however, the exact structure has yet to be determined. The natural targets of IgG2 molecules are carbohydrate antigens, and the interactions are generally low affinity. The evolutionary significance of the multi-valence of IgG2 molecules therefore may be to improve avidity of these molecules to their targets.Citation1,Citation94 The clinical safety issues associated with identical covalent dimers of therapeutic IgG2 molecules having high affinity to their intended targets, or cross-linking of therapeutic IgG2 molecules with other endogenous IgG2 molecules, has yet to be determined. Investigation of mutations in the hinge region to prevent dimerization would help mitigate potential safety issue.
Recent studies have revealed another significant feature of this class of IgG molecules, i.e., the activity of the IgG2 molecule is modulated by disulfide shuffling in serum.Citation86,Citation95,Citation96 Three structurally different isoforms were identified: IgG2-A, IgG2-B and IgG2-A/B (). The isoforms were identified both with native human IgG2 antibodies made from myeloma plasma and from normal serum. Inter-conversion in serum from IgG2-A to IgG2-A/B took about 2 days, and the conversion of IgG2-A/B to IgG2-B, the rate limiting step, took several days. More importantly conversion from IgG2-A to IgG2-B resulted in a decrease in activity of the molecule, probably brought about by restricting movement of the Fab arms. It was also discovered that IgG2 molecules with a lambda light chain were less likely to form the IgG2-B isoforms, possibly due to the extra serine residue in the light chain that sterically hindered cross-linking of the cysteine residues. A side-by-side comparison carried out between an IgG1 and an IgG2 molecule designed to block interleukin-1 cell surface receptor type 1 revealed that the IgG2 molecule underwent a statistically significant decrease in activity when compared to the IgG1 molecule (approximately one third the activity of IgG1).Citation96 This decrease in activity was speculated to be due to inter-conversion of the species—a plausible mechanism of dampening activity of endogenous IgG2 molecules over time. However, a significant decrease in activity during circulation of therapeutic IgG2 antibodies may not be desired, and investigations into mutations that prevent disulfide shuffling would also help mitigate potential safety issues.
IgG1 in serum (stabilization of disulfide bonds).
IgG1 is the isotype most frequently chosen for development of an antibody therapeutic.Citation1 The Fc domain of an IgG1 molecule can trigger effector function by binding to its Fc receptor (FcγRI, FcγRII or FcγRIII), the C1q component of the complement pathway or the FcRn receptor. Effector functions such as ADCC (antibody dependent cell mediated cytotoxicity) are mediated via FcγRs, CDC (complement dependent cytotoxicity) occurs via binding to the C1q protein, and long half-life in serum results from interaction of IgG1 and the neonatal Fc receptor (FcRn). Depending on the therapeutic target of the antibody, mutations in the Fc region can be carried out to either enhance or reduce effector functions.Citation3,Citation4,Citation97
A total of 16 disulfide bonds (4 inter-chain and 12 intra-chain) are found in IgG1 molecules (). Correct pairing of the 16 disulfide bonds takes place in the ER, and the process is aided by chaperones and enzymes such as protein disulfide isomerase. However, IgG1 molecules will occasionally have a population of molecules where the intra-chain disulfide bond in the VH domain between Cys22-Cys96 residues is not paired.Citation98,Citation99 Further, the unpaired cysteine residues can be found in molecules extracted from the ER/Golgi, suggesting that, despite the unpaired cysteine residues, they are not targeted for degradation via the ubiquitin pathway (Correia IR and Alessandri L, unpublished results).
In a significant finding, Ouellette et al. recently reported that the unpaired cysteine residues reformed their disulfide bonds when the mAb is exposed to serum.Citation98 Unlike IgG2 and IgG4 molecules, analytical evaluation of the mAb after recovery from serum, from both in vivo studies and after spiking the mAb in serum, showed rapid pairing of the cysteine residues in serum. It is possible that thiol molecules present in serum provide an environment outside the ER for correct cysteine pairing. This is an important study because incomplete disulfide bond formation has been shown to affect antigen recognition and reduce potency of the molecule.Citation100
Influence in Serum on Higher Order Structureof mAbs
Altered antigen binding in serum.
The behavior of protein therapeutics in solution is routinely assessed by analytical ultracentrifugation (AUC), where the velocity of sedimentation (SV) provides first principle hydrodynamic information.Citation101,Citation102 Such studies enable an investigator to obtain information on size, shape and binding properties of the molecule. The principal measurement taken during sedimentation velocity is the radial distribution of concentration over time, where the measurements of concentration are obtained either by UV absorbance or using Rayleigh interference detectors. Recent advance in optics, have incorporated fluorescence detectors that not only improve sensitivity, but also allow analysis of labeled molecules in complex matrices such as serum.Citation29,Citation103 Studies in serum will not only monitor aggregate formation, but also evaluate interaction of the antibody therapeutic with its target antigen.
Studies of Alexa-488 labeled omalizumab, an anti-IgE therapeutic, revealed that incorporation of 4.5 moles of dye per mole of protein did not affect potency of the molecule, nor did it result in increased aggregate or fragment formation.Citation29 The researchers did find that the affinity of omalizumab for its antigen was not only increased in serum, but the complexes observed in serum were different from those characterized in PBS.Citation29 A number of explanations for this discrepancy are possible; however, the most plausible one takes into account the highly crowded environment in serum, where the excluded volume leads antibody therapeutics to adopt a compact structure and show enhanced protein association with antigen in serum.Citation29
Fragmentation and aggregation of mAbs in blood.
Fragmentation and aggregation of antibody therapeutics are commonly monitored during formulation studies at storage temperature, as well as during stressed stability studies.Citation104 While both AUC and SEC enable analysis of labeled proteins in serum, current initiatives in drug development call for “rapid” evaluation and selection of candidate proteins, peptides or mAbs that not only behave well in blood, but also show good PK properties. A high throughput analytical technique with good precision, sensitivity and ease of use is required to identify poorly behaved molecules early in development. Discontinuing studies of candidates that aggregate or fragment in blood or those with poor PK properties can reduce costs, but also facilitate screening of larger numbers of molecules.
The LabChip GXII platform (Caliper Life Sciences) is a high throughput CE based analytical technique that can be used to analyze pre-labeled mAbs directly from whole blood (Correia IR, unpublished results). Samples of blood are simply spun (2,000 rpm for 5 minutes) and the supernatant (3–5 µL) collected for analysis. The GXII will size proteins between 14 to 200 kDa, and the assay has a 4 log linear range (50 pg/µL to 100 ng/µL). Analysis of each sample is performed in about 40 seconds, and can be done directly from a 96 or 384 well plate. Example electropherograms from three separate analysis of a labeled mAb after 4 and 24 hours in whole blood are shown in ; shows electropherograms obtained for a labeled mAb quantifying aggregate and fragment levels after incubation for 0, 4 and 24 hours. The LabChip GXII is a high throughput analytical technique with very good precision and sensitivity and mAbs can be easily analyzed directly from blood, providing real time information on the behavior of candidate mAbs in blood.
Conclusion
The past two decades has seen therapeutic mAbs evolve from murine-based products to chimeric to fully human products. The most spectacular advances have been made in the fields of oncology and immunology, although mAb products for cardiovascular, ophthalmic and infectious diseases are on the market. As of March 2008, 21 mAbs were marketed in the US, and of these, 15 (1 murine, 5 chimeric, 8 humanized and 1 human) were the IgG1 isotype.Citation105 In 2009, four more human IgG1 molecules were approved by the US Food and Drug Administration (ustekinumab, golimumab, canakinumab, ofatumumab).Citation2 However, non-IgG1 isotypes are increasingly being developed as therapeutics, with an IgG2 (panitumumab), and IgG2/4 hybrid (eculizumab) and two IgG4 (natalizumab, gemtuzumab ozogamicin) currently approved in the US, and a number at various stages in development.
It is important to understand the altered physicochemical properties of IgG2 and IgG4 molecules in serum discussed here; however, engineering stabilizing mutations in the hinge region can help mitigate potential issues associated with dimerization or Fab exchange with endogenous molecules. The influence of serum on other physicochemical properties of antibody therapeutics was also discussed; it is apparent that much information can be obtained during PK studies in monkeys or humans. Analysis of PTM can be performed after the antibody therapeutic is recovered from serum using cross-linked antigen or an anti-human Fcγ antibody. These studies are relevant for not only assessing the exposure of patients to a PTM, but also establishing bioequivalence for the therapeutic; this is especially relevant if a particular isoform is preferentially cleared from serum. The influence of glycation of therapeutic IgG molecules in serum has not been fully explored and future studies must include investigations of this PTM, as well as others that were beyond the scope of this review. In summary, the data discussed here clearly indicate that decisions made during the development of an antibody therapeutic must be based not only on an understanding of the basic biology of the isotype chosen, but also on its stability in serum, which is the intended environment of the therapeutic.
Abbreviations
FDA | = | Food and Drug Administration |
IgG | = | immunoglobulin |
MS | = | mass spectrometry |
ER | = | endoplasmic reticulum |
CDR | = | complementarity determining regions |
PTM | = | post translational modification |
cIEF | = | capillary isoelectric focusing |
NA1F | = | asialo, monogalactosylated biantennary, core-substituted with fucose |
NA2F | = | asialo, bigalactosylated biantennary, core-substituted with fucose |
NGA2F | = | asialo, agalacto-, biantennary, core-substituted with fucose |
NGA2F-GlcNAc | = | asialo, agalacto-, biantennary, core-substituted with fucose minus a bisecting N-acetylglucosamine |
NA1F-GlcNAc | = | asialo, monogalactosylated biantennary, core-substituted with fucose minus a bisecting N-acetylglucosamine |
Figures and Tables
Figure 5 Formation of functional covalent dimers between identical as well as different IgG2 molecules.
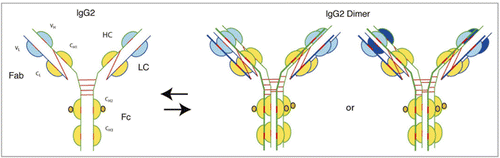
Figure 7 Analysis on LabChip GXII showing recovery of mAbs from blood-precision data (n = 3) after 4 and 24 hours.
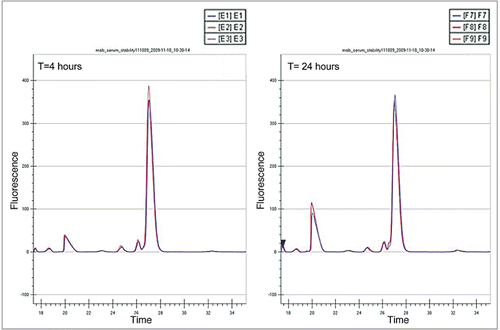
Figure 8 (See opposite page). Monitoring aggregate and fragment formation of a mAb in whole blood using the LabChip GXII (T = 0, 4 and 24 hrs).
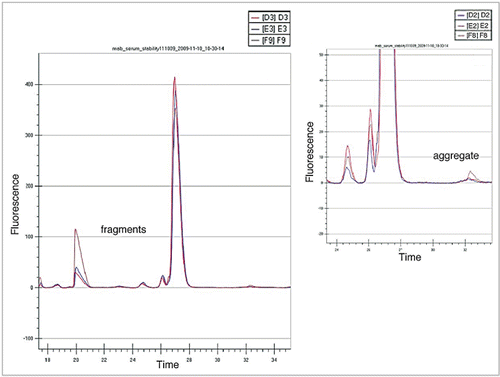
Table 1 Amino acid sequence of hinge region for different IgG isotypes
Table 2 Summary of studies to evaluate influence of the Fc glycan structure on serum clearance
Acknowledgements
The author is grateful to David Lee and Stephanie Moses for the artwork and to Czeslaw Radziejewski, Gary Welch and Peter Moesta for a critical reading of the manuscript and their support. The author acknowledges and thanks members of his group; David Ouellette, Leslie Alessandri, Reema Piparia, Lincoln Muhoro and Aima Aikhoje for their dedication and many helpful discussions.
References
- Salfeld JG. Isotype selection in antibody engineering. Nat Biotechnol 2007; 25:1369 - 1372
- Reichert JM. Antibodies to watch in 2010. MAbs 2010; 2:84 - 100
- Kubota T, Niwa R, Satoh M, Akinaga S, Shitara K, Hanai N. Engineered therapeutic antibodies with improved effector functions. Cancer Sci 2009; 100:1566 - 1572
- Carter PJ. Potent antibody therapeutics by design. Nat Rev Immunol 2006; 6:343 - 357
- Reichert JM, Rosensweig CJ, Faden LB, Dewitz MC. Monoclonal antibody successes in the clinic. Nat Biotechnol 2005; 23:1073 - 1078
- Kola I, Landis J. Can the pharmaceutical industry reduce attrition rates?. Nat Rev Drug Discov 2004; 3:711 - 715
- Pavlou AK, Belsey MJ. The therapeutic antibodies market to 2008. Eur J Pharm Biopharm 2005; 59:389 - 396
- Scott C. The problem with potency. Nature Biotechnol 2005; 23:1037 - 1039
- Porter R. Chemical structure of γ-globulins and antibodies. Br Med Bull 1 1963; 19:197 - 201
- Edelman GM, Poulik MD. Studies on structural units of the gamma-globulins. J Exp Med 1961; 113:861 - 884
- Edelman GM, Benacerraf B, Ovary Z, Poulik MD. Structural differences among antibodies of different specificities. Proc Natl Acad Sci USA 1961; 47:1751 - 1758
- Woof JM, Burton DR. Human antibody-Fc receptor interactions illuminated by crystal structures. Nat Rev Immunol 2004; 4:89 - 99
- Barclay AN. Membrane proteins with immunoglobulin-like domains—a master superfamily of interaction molecules. Semin Immunol 2003; 15:215 - 223
- Jefferis R, Lefranc M. Human immunoglobulin allotypes. MAbs 2009; 1:1 - 7
- Pink JR, Milstein C. Inter heavy-light chain disulphide bridge in immune globulins. Nature 1967; 214:92 - 94
- Milstein C, Pink JR. Structure and evolution of immunoglobulins. Prog Biophys Mol Biol 1970; 21:209 - 263
- Milstein C, Frangione B. Disulphide bridges of the heavy chain of human immunoglobulin G2. Biochem J 1971; 121:217 - 225
- Frangione B, Milstein C, Pink JR. Structural studies of immunoglobulin G. Nature 1969; 221:145 - 148
- Frangione B, Milstein C, Franklin EC. Intrachain disulphide bridges in immunoglobulin G heavy chains. The Fc fragment. Biochem J 1968; 106:15 - 21
- Frangione B, Milstein C, Franklin EC. Chemical typing of immunoglobulins. Nature 1969; 221:149 - 151
- Frangione B, Milstein C. Variations in the S-S bridges of immunoglobins G: interchain disulfide bridges of gamma G3 myeloma proteins. J Mol Biol 1968; 33:893 - 906
- De Preval C, Pink JR, Milstein C. Variability of interchain binding of immunoglobulins. Interchain bridges of mouse IgG2a and IgG2b. Nature 1970; 228:930 - 932
- Issaq HJ, Xiao Z, Veenstra TD. Serum and plasma proteomics. Chem Rev 2007; 107:3601 - 3620
- Anderson NL, Polanski M, Pieper R, Gatlin T, Tirumalai RS, Conrads TP, et al. The human plasma proteome: a nonredundant list developed by combination of four separate sources. Mol Cell Proteomics 2004; 3:311 - 326
- Anderson NL, Anderson NG. The human plasma proteome: history, character and diagnostic prospects. Mol Cell Proteomics 2002; 1:845 - 867
- Pieper R, Gatlin CL, Makusky AJ, Russo PS, Schatz CR, Miller SS, et al. The human serum proteome: display of nearly 3700 chromatographically separated protein spots on two-dimensional electrophoresis gels and identification of 325 distinct proteins. Proteomics 2003; 3:1345 - 1364
- Michel PE, Crettaz D, Morier P, Heller M, Gallot D, Tissot JD, et al. Proteome analysis of human plasma and amniotic fluid by Off-Gel isoelectric focusing followed by nano-LC-MS/MS. Electrophoresis 2006; 27:1169 - 1181
- Coombes KR, Morris JS, Hu J, Edmonson SR, Baggerly KA. Serum proteomics profiling—a young technology begins to mature. Nat Biotechnol 2005; 23:291 - 292
- Demeule B, Shire SJ, Liu J. A therapeutic antibody and its antigen form different complexes in serum than in phosphate-buffered saline: a study by analytical ultracentrifugation. Anal Biochem 2009; 388:279 - 287
- Summa D, Spiga O, Bernini A, Venditti V, Priora R, Frosali S, et al. Protein-thiol substitution or protein dethiolation by thiol/disulfide exchange reactions: the albumin model. Proteins 2007; 69:369 - 378
- Soriani M, Pietraforte D, Minetti M. Antioxidant potential of anaerobic human plasma: role of serum albumin and thiols as scavengers of carbon radicals. Arch Biochem Biophys 1994; 312:180 - 188
- Mills BJ, Weiss MM, Lang CA, Liu MC, Ziegler C. Blood glutathione and cysteine changes in cardiovascular disease. J Lab Clin Med 2000; 135:396 - 401
- Hildebrandt W, Kinscherf R, Hauer K, Holm E, Droge W. Plasma cystine concentration and redox state in aging and physical exercise. Mech Ageing Dev 2002; 123:1269 - 1281
- Fiskerstrand T, Refsum H, Kvalheim G, Ueland PM. Homocysteine and other thiols in plasma and urine: automated determination and sample stability. Clin Chem 1993; 39:263 - 271
- Di Giuseppe D, Di Simplicio P, Capecchi PL, Lazzerini PE, Pasini FL. Alteration in the redox state of plasma in heart-transplant patients with moderate hyperhomocysteinemia. J Lab Clin Med 2003; 142:21 - 28
- Andersson A, Isaksson A, Brattstrom L, Hultberg B. Homocysteine and other thiols determined in plasma by HPLC and thiol-specific postcolumn derivatization. Clin Chem 1993; 39:1590 - 1597
- Weintraub SJ, Manson SR. Asparagine deamidation: a regulatory hourglass. Mech Ageing Dev 2004; 125:255 - 257
- Cournoyer JJ, O’Connor PB. Whitelegge J. Analysis of Deamidation in Proteins. Protein Mass Spectrometry 2008; 52:Amsterdam Elsevier 375 - 410
- Pan H, Chen K, Chu L, Kinderman F, Apostol I, Huang G. Methionine oxidation in human IgG2 Fc decreases binding affinities to protein A and FcRn. Protein Sci 2009; 18:424 - 433
- Liu YD, van Enk JZ, Flynn GC. Human antibody Fc deamidation in vivo. Biologicals 2009; 37:313 - 322
- Wang L, Amphlett G, Lambert JM, Blattler W, Zhang W. Structural characterization of a recombinant monoclonal antibody by electrospray time-of-flight mass spectrometry. Pharm Res 2005; 22:1338 - 1349
- Liu H, Gaza-Bulseco G, Faldu D, Chumsae C, Sun J. Heterogeneity of monoclonal antibodies. J Pharm Sci 2008; 97:2426 - 2447
- Chelius D, Rehder DS, Bondarenko PV. Identification and characterization of deamidation sites in the conserved regions of human immunoglobulin gamma antibodies. Anal Chem 2005; 77:6004 - 6011
- Huang L, Lu J, Wroblewski VJ, Beals JM, Riggin RM. In vivo deamidation characterization of monoclonal antibody by LC/MS/MS. Anal Chem 2005; 77:1432 - 1439
- Harris RJ, Kabakoff B, Macchi FD, Shen FJ, Kwong M, Andya JD, et al. Identification of multiple sources of charge heterogeneity in a recombinant antibody. J Chromatogr B Biomed Sci Appl 2001; 752:233 - 245
- Doyle HA, Zhou J, Wolff MJ, Harvey BP, Roman RM, Gee RJ, et al. Isoaspartyl post-translational modification triggers anti-tumor T and B lymphocyte immunity. J Biol Chem 2006; 281:32676 - 32683
- Doyle HA, Gee RJ, Mamula MJ. Altered immunogenicity of isoaspartate containing proteins. Autoimmunity 2007; 40:131 - 137
- Yan B, Steen S, Hambly D, Valliere-Douglass J, Vanden Bos T, Smallwood S, et al. Succinimide formation at Asn 55 in the complementarity determining region of a recombinant monoclonal antibody IgG1 heavy chain. J Pharm Sci 2009; 98:3509 - 3521
- Liu H, Gaza-Bulseco G, Sun J. Characterization of the stability of a fully human monoclonal IgG after prolonged incubation at elevated temperature. J Chromatogr B Analyt Technol Biomed Life Sci 2006; 837:35 - 43
- Li N, Kessler K, Bass L, Zeng D. Evaluation of the iCE280 Analyzer as a potential high-throughput tool for formulation development. J Pharm Biomed Anal 2007; 43:963 - 972
- Hambly DM, Banks DD, Scavezze JL, Siska CC, Gadgil HS. Detection and quantitation of IgG 1 hinge aspartate isomerization: a rapid degradation in stressed stability studies. Anal Chem 2009; 81:7454 - 7459
- Robinson NE, Robinson AB. Molecular clocks. Proc Natl Acad Sci USA 2001; 98:944 - 949
- Robinson NE, Robinson AB. Deamidation of human proteins. Proc Natl Acad Sci USA 2001; 98:12409 - 12413
- Robinson NE. Protein deamidation. Proc Natl Acad Sci USA 2002; 99:5283 - 5288
- Robinson AB, McKerrow JH, Cary P. Controlled deamidation of peptides and proteins: an experimental hazard and a possible biological timer. Proc Natl Acad Sci USA 1970; 66:753 - 757
- Liu D, Ren D, Huang H, Dankberg J, Rosenfeld R, Cocco MJ, et al. Structure and stability changes of human IgG1 Fc as a consequence of methionine oxidation. Biochemistry 2008; 47:5088 - 5100
- Kroon DJ, Baldwin-Ferro A, Lalan P. Identification of sites of degradation in a therapeutic monoclonal antibody by peptide mapping. Pharm Res 1992; 9:1386 - 1393
- Bertolotti-Ciarlet A, Wang W, Lownes R, Pristatsky P, Fang Y, McKelvey T, et al. Impact of methionine oxidation on the binding of human IgG1 to Fc Rn and Fc gamma receptors. Mol Immunol 2009; 46:1878 - 1882
- Lam XM, Yang JY, Cleland JL. Antioxidants for prevention of methionine oxidation in recombinant monoclonal antibody HER2. J Pharm Sci 1997; 86:1250 - 1255
- Chumsae C, Gaza-Bulseco G, Sun J, Liu H. Comparison of methionine oxidation in thermal stability and chemically stressed samples of a fully human monoclonal antibody. J Chromatogr B Analyt Technol Biomed Life Sci 2007; 850:285 - 294
- Teh LC, Murphy LJ, Huq NL, Surus AS, Friesen HG, Lazarus L, et al. Methionine oxidation in human growth hormone and human chorionic somatomammotropin. Effects on receptor binding and biological activities. J Biol Chem 1987; 262:6472 - 6477
- Hermeling S, Crommelin DJ, Schellekens H, Jiskoot W. Structure-immunogenicity relationships of therapeutic proteins. Pharm Res 2004; 21:897 - 903
- Shields RL, Namenuk AK, Hong K, Meng YG, Rae J, Briggs J, et al. High resolution mapping of the binding site on human IgG1 for FcgammaRI, FcgammaRII, FcgammaRIII and FcRn and design of IgG1 variants with improved binding to the FcgammaR. J Biol Chem 2001; 276:6591 - 6604
- Martin WL, West AP Jr, Gan L, Bjorkman PJ. Crystal structure at 2.8 A of an FcRn/heterodimeric Fc complex: mechanism of pH-dependent binding. Mol Cell 2001; 7:867 - 877
- Medesan C, Matesoi D, Radu C, Ghetie V, Ward ES. Delineation of the amino acid residues involved in transcytosis and catabolism of mouse IgG1. J Immunol 1997; 158:2211 - 2217
- Kim JK, Tsen MF, Ghetie V, Ward ES. Localization of the site of the murine IgG1 molecule that is involved in binding to the murine intestinal Fc receptor. Eur J Immunol 1994; 24:2429 - 2434
- Ghetie V, Ward ES. FcRn: the MHC class I-related receptor that is more than an IgG transporter. Immunol Today 1997; 18:592 - 598
- Stockert RJ. The asialoglycoprotein receptor: relationships between structure, function and expression. Physiol Rev 1995; 75:591 - 609
- Stahl PD. The mannose receptor and other macrophage lectins. Curr Opin Immunol 1992; 4:49 - 52
- Pontow SE, Kery V, Stahl PD. Mannose receptor. Int Rev Cytol 1992; 137:221 - 244
- Lee SJ, Evers S, Roeder D, Parlow AF, Risteli J, Risteli L, et al. Mannose receptor-mediated regulation of serum glycoprotein homeostasis. Science 2002; 295:1898 - 1901
- Bridges K, Harford J, Ashwell G, Klausner RD. Fate of receptor and ligand during endocytosis of asialoglycoproteins by isolated hepatocytes. Proc Natl Acad Sci USA 1982; 79:350 - 354
- Ashwell G, Harford J. Carbohydrate-specific receptors of the liver. Annu Rev Biochem 1982; 51:531 - 554
- Jefferis R. Glycosylation of natural and recombinant antibody molecules. Adv Exp Med Biol 2005; 564:143 - 148
- Chen X, Liu YD, Flynn GC. The effect of Fc glycan forms on human IgG2 antibody clearance in humans. Glycobiology 2009; 19:240 - 249
- Zhou Q, Shankara S, Roy A, Qiu H, Estes S, McVie-Wylie A, et al. Development of a simple and rapid method for producing non-fucosylated oligomannose containing antibodies with increased effector function. Biotechnol Bioeng 2008; 99:652 - 665
- Wright A, Morrison SL. Effect of altered CH2-associated carbohydrate structure on the functional properties and in vivo fate of chimeric mouse-human immunoglobulin G1. J Exp Med 1994; 180:1087 - 1096
- Newkirk MM, Novick J, Stevenson MM, Fournier MJ, Apostolakos P. Differential clearance of glycoforms of IgG in normal and autoimmune-prone mice. Clin Exp Immunol 1996; 106:259 - 264
- Millward TA, Heitzmann M, Bill K, Langle U, Schumacher P, Forrer K. Effect of constant and variable domain glycosylation on pharmacokinetics of therapeutic antibodies in mice. Biologicals 2008; 36:41 - 47
- Keck R, Nayak N, Lerner L, Raju S, Ma S, Schreitmueller T, et al. Characterization of a complex glycoprotein whose variable metabolic clearance in humans is dependent on terminal N-acetylglucosamine content. Biologicals 2008; 36:49 - 60
- Kanda Y, Yamada T, Mori K, Okazaki A, Inoue M, Kitajima-Miyama K, et al. Comparison of biological activity among nonfucosylated therapeutic IgG1 antibodies with three different N-linked Fc oligosaccharides: the high-mannose, hybrid and complex types. Glycobiology 2007; 17:104 - 118
- Huang L, Biolsi S, Bales KR, Kuchibhotla U. Impact of variable domain glycosylation on antibody clearance: an LC/MS characterization. Anal Biochem 2006; 349:197 - 207
- Chaderjian WB, Chin ET, Harris RJ, Etcheverry TM. Effect of copper sulfate on performance of a serum-free CHO cell culture process and the level of free thiol in the recombinant antibody expressed. Biotechnol Prog 2005; 21:550 - 553
- Banks DD, Gadgil HS, Pipes GD, Bondarenko PV, Hobbs V, Scavezze JL, et al. Removal of cysteinylation from an unpaired sulfhydryl in the variable region of a recombinant monoclonal IgG1 antibody improves homogeneity, stability and biological activity. J Pharm Sci 2008; 97:775 - 790
- van der Neut Kolfschoten M, Schuurman J, Losen M, Bleeker WK, Martinez-Martinez P, Vermeulen E, et al. Anti-inflammatory activity of human IgG4 antibodies by dynamic Fab arm exchange. Science 2007; 317:1554 - 1557
- Liu YD, Chen X, Enk JZ, Plant M, Dillon TM, Flynn GC. Human IgG2 antibody disulfide rearrangement in vivo. J Biol Chem 2008; 283:29266 - 29272
- van der Zee JS, van Swieten P, Aalberse RC. Serologic aspects of IgG4 antibodies II. IgG4 antibodies form small, nonprecipitating immune complexes due to functional monovalency. J Immunol 1986; 137:3566 - 3571
- Schuurman J, Van Ree R, Perdok GJ, Van Doorn HR, Tan KY, Aalberse RC. Normal human immunoglobulin G4 is bispecific: it has two different antigen-combining sites. Immunology 1999; 97:693 - 698
- Schuurman J, Perdok GJ, Gorter AD, Aalberse RC. The inter-heavy chain disulfide bonds of IgG4 are in equilibrium with intra-chain disulfide bonds. Mol Immunol 2001; 38:1 - 8
- Bloom JW, Madanat MS, Marriott D, Wong T, Chan SY. Intrachain disulfide bond in the core hinge region of human IgG4. Protein Sci 1997; 6:407 - 415
- Angal S, King DJ, Bodmer MW, Turner A, Lawson AD, Roberts G, et al. A single amino acid substitution abolishes the heterogeneity of chimeric mouse/human (IgG4) antibody. Mol Immunol 1993; 30:105 - 108
- Aalberse RC, Schuurman J. IgG4 breaking the rules. Immunology 2002; 105:9 - 19
- Labrijn AF, Buijsse AO, van den Bremer ET, Verwilligen AY, Bleeker WK, Thorpe SJ, et al. Therapeutic IgG4 antibodies engage in Fab-arm exchange with endogenous human IgG4 in vivo. Nat Biotechnol 2009; 27:767 - 771
- Yoo EM, Wims LA, Chan LA, Morrison SL. Human IgG2 can form covalent dimers. J Immunol 2003; 170:3134 - 3138
- Wypych J, Li M, Guo A, Zhang Z, Martinez T, Allen MJ, et al. Human IgG2 antibodies display disulfide-mediated structural isoforms. J Biol Chem 2008; 283:16194 - 16205
- Dillon TM, Ricci MS, Vezina C, Flynn GC, Liu YD, Rehder DS, et al. Structural and functional characterization of disulfide isoforms of the human IgG2 subclass. J Biol Chem 2008; 283:16206 - 16215
- Duncan AR, Woof JM, Partridge LJ, Burton DR, Winter G. Localization of the binding site for the human high-affinity Fc receptor on IgG. Nature 1988; 332:563 - 564
- Ouellette D, Alessandri L, Chin A, Grinnell C, Tarcsa E, Radziejewski C, et al. Studies in serum support rapid formation of disulfide bond between unpaired cysteine residues in the V(H) domain of an immunoglobulin G1 molecule. Anal Biochem 2010; 397:37 - 47
- Harris RJ. Heterogeneity of recombinant antibodies: linking structure to function. Dev Biol (Basel) 2005; 122:117 - 127
- Jefferis R. Glycosylation of recombinant antibody therapeutics. Biotechnol Prog 2005; 21:11 - 16
- Howlett GJ, Minton AP, Rivas G. Analytical ultracentrifugation for the study of protein association and assembly. Curr Opin Chem Biol 2006; 10:430 - 436
- Cole JL, Lary JW, T PM, Laue TM. Analytical ultracentrifugation: sedimentation velocity and sedimentation equilibrium. Methods Cell Biol 2008; 84:143 - 179
- Kroe RR, Laue TM. NUTS and BOLTS: applications of fluorescence-detected sedimentation. Anal Biochem 2009; 390:1 - 13
- Shire SJ. Formulation and manufacturability of biologics. Curr Opin Biotechnol 2009; 20:708 - 714
- Samaranayake H, Wirth T, Schenkwein D, Raty JK, Yla-Herttuala S. Challenges in monoclonal antibody-based therapies. Ann Med 2009; 41:322 - 331
- Jones AJ, Papac DI, Chin EH, Keck R, Baughman SA, Lin YS, et al. Selective clearance of glycoforms of a complex glycoprotein pharmaceutical caused by terminal N-acetylglucosamine is similar in humans and cynomolgus monkeys. Glycobiology 2007; 17:529 - 540
- Wawrzynczak EJ, Cumber AJ, Parnell GD, Jones PT, Winter G. Blood clearance in the rat of a recombinant mouse monoclonal antibody lacking the N-linked oligosaccharide side chains of the CH2 domains. Mol Immunol 1992; 29:213 - 220