Abstract
Heparin-binding epidermal growth factor-like growth factor (HB-EGF) is a member of the epidermal growth factor family. The accumulated evidence on the tumor-progressing roles of HB-EGF has suggested that HB-EGF-targeted cancer therapy is expected to be promising. However, the generation of neutralizing anti-HB-EGF monoclonal antibodies (mAbs) has proved difficult. To overcome this difficulty, we performed a hybridoma approach using mice from different genetic backgrounds, as well as different types of HB-EGF immunogens. To increase the number of hybridoma clones to screen, we used an electrofusion system to generate hybridomas and a fluorometric microvolume assay technology to screen anti-HB-EGF mAbs. We succeeded in obtaining neutralizing anti-HB-EGF mAbs, primarily from BALB/c and CD1 mice, and these were classified into 7 epitope bins based on their competitive binding to the soluble form of HB-EGF (sHB-EGF). The mAbs showed several epitope bin-dependent characteristics, including neutralizing and binding activity to human sHB-EGF, cross-reactivity to mouse/rat sHB-EGF and binding activity to the precursor form of HB-EGF. The neutralizing activity was also validated in colony formation assays. Interestingly, we found that the populations of mAb bins and the production rates of the neutralizing mAbs were strikingly different by mouse strain and by immunogen type. We succeeded in generating a variety of neutralizing anti-HB-EGF mAbs, including potent sHB-EGF neutralizers that may have potential as therapeutic agents for treating HB-EGF-dependent cancers. Our results also suggest that immunization approaches using different mouse strains and immunogen types affect the biological activity of individual neutralizing antibodies.
Introduction
Heparin-binding epidermal growth factor-like growth factor (HB-EGF) is a member of the epidermal growth factor (EGF) family. It is synthesized as membrane-bound proHB-EGF, which is a precursor of the soluble form of HB-EGF (sHB-EGF).Citation1 Ectodomain shedding of proHB-EGF results in the release of sHB-EGF, which has potent mitogenic activity through the binding and activation of EGF receptor (EGFR) on EGFR-expressing cells.Citation2 Previous studies have shown overexpression of HB-EGF in multiple cancer types,Citation3-Citation7 and the HB-EGF expression level is correlated with poor prognosis in cancer patients.Citation7-Citation9 Therefore, blockage of HB-EGF/EGFR signaling by a potent neutralizing anti-HB-EGF mAb has the potential to be a promising anti-cancer therapy. However, it has been difficult to obtain anti-HB-EGF mAbs by using a hybridoma approach because of the high homology between the amino acid sequences of human and mouse HB-EGF.Citation10 To date, only a few neutralizing anti-HB-EGF monoclonal antibodies (mAbs) have been reported.Citation11,Citation12 DE10 is the first reported anti-HB-EGF antibody with neutralizing activity. This mAb, which is cross-reactive to rat HB-EGF, inhibits sHB-EGF-induced DNA synthesis and inhibits the binding of cells to fibronectin and laminin.Citation11 More recently, KM3566 was established as a neutralizing mAb specific to human HB-EGF.Citation12 Both of these mAbs were obtained by immunizing a single type of immunogen, recombinant sHB-EGF protein, and screening by an enzyme-linked immunosorbent assay (ELISA). The development of neutralizing anti-HB-EGF mAbs with different biochemical or biological profiles is helpful for the advancement of HB-EGF research. For example, more potent neutralizing anti-HB-EGF mAbs might expedite progress in the clinical research of HB-EGF. In addition, if the neutralizing anti-HB-EGF mAbs are cross-reactive to mouse HB-EGF, they could be useful for the evaluation of its anti-tumor activity and adverse events profile in mouse models of cancer.
Previous studies have shown that the same immunogen can elicit different antibody responses in different mouse strains,Citation13-Citation15 and different forms of the antigen can also alter antibody responses.Citation16-Citation18 However, the antibody responses were tested with antisera in these studies, and little information is known about the effects of different mouse strains and immunogen types on the characteristics of individual mAbs.
In this study, we succeeded in generating a variety of neutralizing anti-HB-EGF mAbs by using different mouse strains and different preparations of the immunogen HB-EGF. Here, we discuss the characteristics of the mAbs and their correlation to the epitope bin and the immunization method.
Results
Generation of anti-HB-EGF mAbs
To maximize the chances of obtaining neutralizing anti-HB-EGF mAbs, we tested various immunization methods and screened hybridomas in a high-throughput manner. We used mice with four different genetic backgrounds (BALB/c, C57BL, C3H and CD1) as hosts. Carrier protein-conjugated forms of HB-EGF were used as immunogens through all the immunizations to enhance the antibody response. The four mouse strains were immunized subcutaneously with KLH-conjugated sHB-EGF (KLH-conjugate). In addition, BSA-conjugated sHB-EGF (BSA-conjugate) was tested in CD1 mice (BSA/CD1), and 2 other immunizations were tested in BALB/c mice: KLH-conjugate immunization plus a final boost of sHB-EGF (KLH/sHB-EGF/BALB/c), and co-immunization with KLH-conjugate plus proHB-EGF-expressing 293F cells (KLH/cell/BALB/c). We used an electrofusion system for high-efficiency hybridoma production, and subsequent fluorometric microvolume assay technology (FMAT) assay to screen anti-HB-EGF mAb-secreting hybridoma clones with hybridoma culture supernatant as the antibody source. Using this approach, we identified 3,337 HB-EGF-specific mAb-secreting hybridoma clones. The rates of obtaining HB-EGF-specific mAb-secreting hybridoma clones for each immunization are summarized in .
Table 1. Summary of anti-HB-EGF mAb generation by mouse strain and immunogen
Neutralizing activity of anti-HB-EGF mAbs
Hybridoma clones secreting neutralizing anti-HB-EGF mAbs were then screened by 2 assays. We selected 210 hybridoma clones, whose culture supernatant inhibited sHB-EGF binding to EGFR by more than 70%. The culture supernatant was then applied to EGFR phosphorylation assay using SK-OV-3 ovarian cancer cells, and they all inhibited the sHB-EGF-induced EGFR phosphorylation by more than 50% (). Because antibody concentrations in culture supernatants differed for each hybridoma clone, we then tested their neutralizing activity in the EGFR phosphorylation assay by using protein A-purified mAbs and confirmed that they possessed a neutralizing activity against sHB-EGF-induced EGFR phosphorylation. We found that they neutralized the sHB-EGF-induced EGFR phosphorylation with a broad range of IC50 values, ranging from 5.5 × 10−11 M to 7.6 × 10−7 M (“All” column in ). We further tested the effect of the neutralizing mAbs against sHB-EGF-dependent cancer cell growth by measuring colony formation of RMG-I ovarian cancer cells. Eighty-nine anti-HB-EGF mAbs, which possessed IC50 values of less than 5 × 10−9 M in the EGFR phosphorylation assay, were applied to the colony formation assay (“All” column in ). The IC50 values of neutralizing anti-HB-EGF mAbs in the EGFR phosphorylation and colony formation assays were positively correlated (), suggesting that the inhibition of sHB-EGF-induced EGFR phosphorylation leads to suppression of the sHB-EGF-dependent cancer cell growth.
Figure 1. Neutralizing activity of anti-HB-EGF mAbs against sHB-EGF functions. (A) Neutralizing activity of anti-HB-EGF mAbs against sHB-EGF-induced EGFR phosphorylation in SK-OV-3 cells. The plots represent the IC50 values of 146 mAbs used in the epitope binning study (). The horizontal line indicates the median IC50 value. (B) Neutralizing activity of anti-HB-EGF mAbs against sHB-EGF-induced colony formation in RMG-I cells. We used the 89 mAbs with IC50 values of less than 5 × 10−9 M in the EGFR phosphorylation assay, and this plot represents the IC50 values of the 83 mAbs with IC50 values of less than 1 × 10−7 M. The horizontal line indicates the median IC50 value. (C) Correlation of neutralizing activities of anti-HB-EGF mAbs against sHB-EGF-induced EGFR phosphorylation and colony formation. Each dot represents the IC50 values of 83 mAbs with an IC50 value of less than 1 × 10−7 M in the colony formation assay (B). The data were analyzed by the Spearman’s test (two-tailed). The Spearman correlation was r = 0.6730 (p < 0.0001).
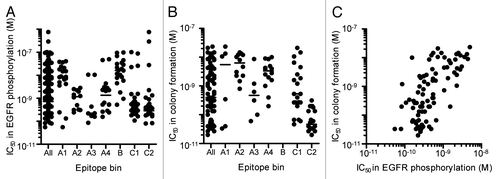
Binding activities of neutralizing anti-HB-EGF mAbs to sHB-EGF and proHB-EGF
As a method to characterize the neutralizing mAbs, we evaluated the binding activities of the mAbs to human, mouse, and rat sHB-EGF by ELISA and to human proHB-EGF by flow cytometry. The EC50 values to human sHB-EGF ranged from 1.3 × 10−11 M to 7.8 × 10−10 M (“All” column in ). We found that the range of EC50 values to mouse and rat sHB-EGF was much broader (1.6 × 10−11 M to 3.0 × 10−6 M and 1.4 × 10−11 M to 1.0 × 10−7 M, respectively) than that to human sHB-EGF (“All” column in ). Likewise, the neutralizing anti-HB-EGF mAbs showed a diverse binding activity to proHB-EGF. Some mAbs showed a strong binding activity to proHB-EGF (“All” column in ).
Figure 2. Binding activities of neutralizing anti-HB-EGF mAbs to HB-EGF. Binding activity of anti-HB-EGF mAbs to (A) human sHB-EGF, (B) mouse sHB-EGF and (C) rat sHB-EGF. The binding activities were determined by ELISA. The plots represent EC50 values of 146 mAbs used in the epitope binning study (). The horizontal line indicates the median EC50 value. (D) Binding activity of anti-HB-EGF mAbs to proHB-EGF was measured by flow cytometry. The plots represent median fluorescence intensity (MFI) ratio of the 146 mAbs used in the epitope binning study (). The MFI ratio of anti-HB-EGF mAb to a control sample was used as the measure of binding activity to proHB-EGF. The horizontal line indicates the median MFI ratio.
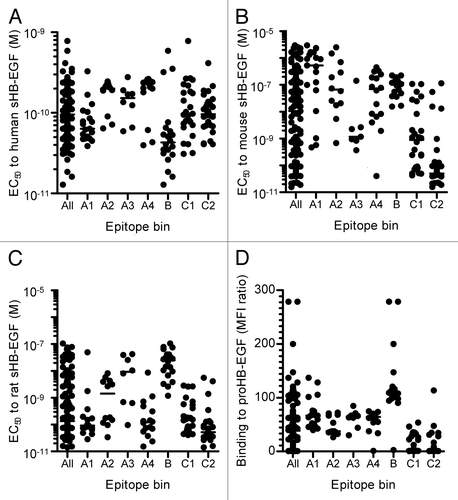
Epitope binning study of neutralizing anti-HB-EGF mAbs
On the basis of the findings described above, we hypothesized that neutralizing anti-HB-EGF mAbs might recognize different epitopes. We performed an epitope binning study by measuring their competitive binding to human sHB-EGF in the FMAT assay. Of the 210 neutralizing mAbs, we used 156 that provided a sufficient amount for this assay from a small-scale hybridoma culture. At first, 129 mAbs were tested, and of those, 119 mAbs were classified into 3 epitope bins, designated A, B and C, which were distinguished by the hierarchical dendrogram and the color-coded map (). The remaining 10 mAbs were not categorized into any of these epitope bins. Because mAbs in epitope bins A and C showed heterogenous properties regarding neutralizing and/or binding activities (data not shown), we decided to subdivide these bins. Therefore, based on the visual inspection of the hierarchical dendrogram in , we further subdivided epitope bin A into A1, A2, A3 and A4, and epitope bin C into C1 and C2. Another 27 mAbs were additionally purified and sorted into the epitope bins by performing the competitive binding assay with several representative mAbs from each epitope bin. Finally, 146 neutralizing anti-HB-EGF mAbs were classified into epitope bins A1 (n = 19), A2 (n = 12), A3 (n = 8), A4 (n = 16), B (n = 28), C1 (n = 29) and C2 (n = 34).
Figure 3. Epitope binning study of anti-HB-EGF mAbs. The competitive binding of all mAb pairs to sHB-EGF was measured in the FMAT assay. Binding of biotinylated anti-HB-EGF mAb to sHB-EGF was detected in the presence of a label-free anti-HB-EGF mAb. Horizontal and vertical axes indicate unlabeled and biotinylated mAbs, respectively. Data were analyzed by Ward’s method with Euclidean distance. Dendrogram on the left axis represents the similarity of each mAb in the competitive binding pattern to sHB-EGF. The color scale from 0 to 100 shows the competitive binding of the 2 mAbs.
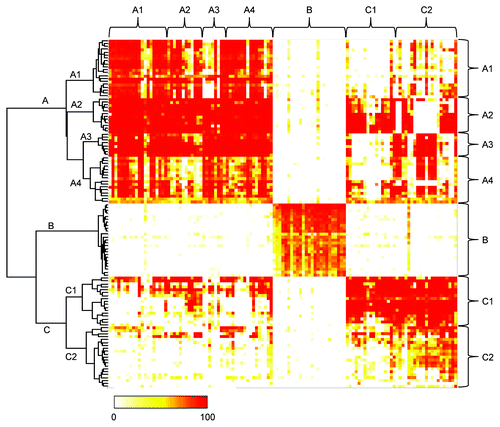
The epitope binning study allowed us to identify characteristics of the antibodies that were unique to each epitope bin. The mAbs in epitope bins A3, C1 and C2 exhibited potent neutralizing activities, whereas those in epitope bins A1 and B had weaker activity (). The mAbs in epitope bins C1 and C2 were highly cross-reactive to mouse and rat sHB-EGF, while the mAbs in epitope bin B were less cross-reactive to mouse and rat sHB-EGF (). The mAbs in epitope bin A3 cross-reacted selectively to mouse HB-EGF, while those in epitope bins A1 and A4 cross-reacted specifically to rat HB-EGF. The mAbs in epitope bin A2 showed a profile similar to those in epitope bin A4 in terms of neutralizing and binding activities to sHB-EGF (; ). However, epitope bin A2 consisted of mAbs with a weaker binding activity to proHB-EGF than those in epitope bin A4 (). The mAbs in epitope bin B demonstrated the strongest binding activity to proHB-EGF, as well as to sHB-EGF (). Meanwhile, the mAbs in epitope bin C1 and C2 showed a weak binding activity to proHB-EGF instead of their binding activities to human sHB-EGF comparable to the mAbs of other epitope bins. These epitope bin-specific characteristics indicated that the epitope bins of the mAbs were properly determined.
Unique epitope bin population by mouse strain and immunogen type
We analyzed each epitope bin population by immunization method to determine whether mouse strain or immunogen type affected the antibody characteristics. Of the 146 antibodies classified in the epitope binning study, we excluded the mAbs from C57BL and C3H mice because both strains produced very few neutralizing mAbs (). As shown in , we found that epitope bin populations were strikingly different among the immunization methods. These results clearly indicated that mouse strain and immunogen type can both affect antibody functionality.
Figure 4. Difference of epitope bin population by mouse strain and immunogen type. Epitope bin populations were analyzed based on the immunization method used to generate the mAbs. a-cBALB/c immunization with aKLH-conjugated sHB-EGF; bKLH-conjugate and a final boost of sHB-EGF; cco-immunization of the KLH-conjugate and proHB-EGF-expressing cells; d,eCD1 immunization with dKLH-conjugate; eBSA-conjugated sHB-EGF.
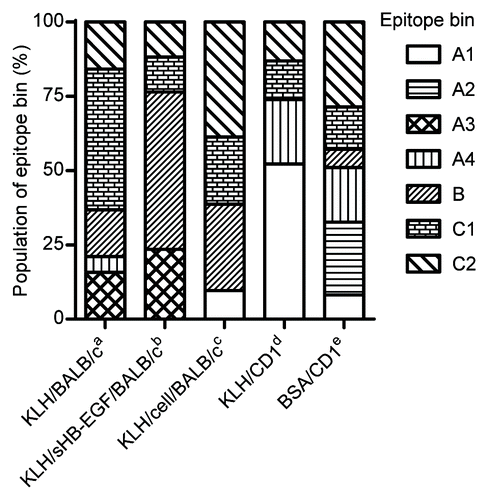
Discussion
Previously reported neutralizing anti-HB-EGF mAbs were generated using the carrier-free recombinant sHB-EGF protein as the immunogen.Citation11,Citation12 In these studies, hybridoma clones were generated by a conventional polyethylene glycol fusion method, and HB-EGF mAb-secreting clones were screened by ELISA. Antigen-null mice are useful tools while obtaining antibodies against an auto-antigen because they do not develop immune tolerance to the antigen.Citation19 Because of the high homology between the amino acid sequences of human and mouse HB-EGF, HB-EGF-null mice were used for the generation of anti-HB-EGF mAbs.Citation11,Citation12 However, the reported number of anti-HB-EGF mAbs from the antigen-null mouse was limited. Herein, we succeeded in identifying 3,337 hybridoma clones secreting HB-EGF-specific antibody, including 210 neutralizing mAb clones from wild-type mice. We leveraged multiple approaches to efficiently obtain neutralizing anti-HB-EGF mAbs. We immunized different mouse strains with different forms of HB-EGF as the immunogen. We used KLH- or BSA-conjugated forms of sHB-EGF for an enhanced immune response. An electrofusion system and a homogeneous high-throughput screening assay enabled us to obtain and evaluate a large number of hybridoma clones. We speculate that the combination of these approaches contributed to the identification of the unprecedented number of the neutralizing mAbs. A subset of the mAbs obtained here showed a previously unreported cross-reactivity to mouse HB-EGF. The mAbs in epitope bins C1 and C2 showed a strong binding activity to mouse and rat sHB-EGF (). Their neutralizing activity was evaluated only against human sHB-EGF (); however, these antibodies are likely to be effective neutralizers against mouse and rat sHB-EGF, making them useful tools for validating in vivo sHB-EGF functions caused by blocking HB-EGF/EGFR signaling in these animals. We also found that the mAbs in epitope bin C2 showed potent neutralizing activity, with IC50 values of less than 1 × 10−10 M in a colony formation assay (). Some mAbs completely inhibited 1 ng/mL of sHB-EGF-induced colony formation at a dose as low as 0.1 nM (data not shown). Although it is difficult to directly compare the neutralizing activities in different cell-based assays, the neutralizing activities of the mAbs obtained here seemed to be more potent than those of previously reported mAbs.Citation11,Citation12 In addition, the neutralizing activity of anti-HB-EGF mAbs in the EGFR phosphorylation assay was well-correlated with that in the colony formation assay (). This finding strongly suggests that blockage of sHB-EGF/EGFR signaling is a promising therapy for treating sHB-EGF-dependent cancers.
As shown in , KLH-conjugate immunizations of different mouse strains produced anti-HB-EGF mAb-positive and neutralizing mAb-positive hybridoma clones at different rates. CD1 mice produced neutralizing anti-HB-EGF mAbs at a higher positive rate than did the other mouse strains (0.72% vs. 0.11%, 0.06% and 0.08%), which probably reflects the highest positive rate of anti-HB-EGF mAbs in CD1 mice. In contrast, the positive rate of neutralizing mAbs in C3H mice was only 0.08%, although its positive rate was as high as 8.0% for HB-EGF-specific mAbs. Thus, the production rates for HB-EGF-specific and neutralizing mAbs were mouse strain-dependent. In the BALB/c immunizations, sHB-EGF injection at the final boost (KLH/sHB-EGF/BALB/c) resulted in a higher positive rate for neutralizing mAbs than KLH-conjugate (KLH/BALB/c) (0.91% vs. 0.11%), although the positive rates for anti-HB-EGF mAbs were similar (7.8% vs. 5.7%). In the CD1 immunizations, BSA-conjugate gave a higher positive rate for neutralizing mAbs than did KLH-conjugate (1.97% vs. 0.72%), although the positive rates for anti-HB-EGF mAbs were similar (11.6% vs. 13.2%). These results suggested that the type of immunogen also affected the production rates for anti-HB-EGF mAbs and neutralizing mAbs.
The population of epitope bins was markedly different between mouse strains and immunogen types. The mAbs in epitope bin A2 were obtained only from BSA/CD1 and those in epitope bin A3 were obtained only from KLH/BALB/c and KLH/sHB-EGF/BALB/c. In addition, the mAbs in epitope bins A4 and B were obtained primarily from CD1 and BALB/c mice, respectively. Although further analysis is required to clarify the mechanism behind this phenomenon, these results demonstrate that immunization methods using different mouse strains and immunogen types produce neutralizing anti-HB-EGF mAbs with various features.
Considering the variation in mAb characteristics, we classified the neutralizing mAbs by using an epitope binning study. To date, multiple methods have been established for epitope binning.Citation20 Although competitive assays using ELISA or Biacore are convenient, these methods are not practical for epitope binning of a large number of mAbs. Therefore, we chose to use the FMAT assay,Citation21 which enabled us to classify antibodies in a high-throughput manner and gave us several insights for HB-EGF studies. Because we used only neutralizing mAbs in the FMAT assay, we expected that all the mAbs could competitively bind to sHB-EGF by recognizing an epitope in sHB-EGF that is critical for EGFR binding. However, the mAbs in epitope bin B did not competitively bind to sHB-EGF with those in epitope bins A and C (), suggesting that the mAbs in epitope bin B and those in epitope bins A and C recognize different epitopes and inhibit sHB-EGF binding to EGFR by different approaches. In fact, previous studies have demonstrated that multiple sites are involved in the interaction between EGF and EGFR. Two domains (I and III) in the extracellular portion of EGFR have been shown to play important roles in ligand binding.Citation22-Citation24 Crystal structure analysis has proposed that the interface between EGF and EGFR consists of 3 sites.Citation25 One of them is located in domain I and the other two in domain III. Binding studies using sHB-EGF and proHB-EGF also provided interesting information. The mAbs in epitope bins C1 and C2 showed weaker binding activity to proHB-EGF, but stronger binding activity to sHB-EGF, compared with the mAbs in epitope bins A2, A3 and A4 (). This result is likely due to the different conformations of sHB-EGF and the ectodomain of proHB-EGF.
In this study, we obtained a number of neutralizing anti-HB-EGF mAbs by using different immunization methods and several high-throughput approaches. We found that the neutralizing activities of these mAbs, as well as their binding features, were defined by the epitope bin. Some of the tested neutralizing mAbs showed potent anti-cell proliferation activity against cancer cells, suggesting that they may be effective anti-cancer agents. Our findings further provide evidence that the immunization method has an effect on the production rates of mAbs and their characteristics.
Materials and Methods
Preparation of recombinant proteins
To prepare recombinant human, mouse, or rat sHB-EGF, the expression plasmids were constructed by cloning human, mouse, or rat sHB-EGF cDNA encoding amino acids 1–148 of proHB-EGF into the pcDNA3.1 vector (Invitrogen). Because the pro-peptide sequences of mouse and rat HB-EGF were not cleaved in human-derived 293F cells (Invitrogen), mouse and rat sHB-EGF cDNA encoding amino acids 1–62 was replaced with a corresponding sequence of human sHB-EGF cDNA. sHB-EGF expression plasmid was transfected into 293F cells by using 293fectin (Invitrogen) according to the supplier’s recommendation. sHB-EGF was purified from the culture supernatant of the 293F cells with HiTrap Heparin HP (GE Healthcare), followed by a purification with a TSKgel CM-5PW column (Tosoh).
We also produced recombinant EGFR ectodomain fused to the human IgG1 Fc region (EGFR-hFc). EGFR ectodomain cDNA (amino acids 1–645 of human EGFR) was inserted into pcDNA3.1 already containing the hFc gene. The expression plasmid was transfected into 293F cells as described above, and the recombinant EGFR-hFc protein was purified from the culture supernatant with rProteinA Sepharose FF (GE Healthcare), and subsequently with a Superdex 200 10/300 column (GE Healthcare).
Generation of anti-HB-EGF mAbs
Five BALB/c mice (8 weeks old, Japan Clea) were immunized subcutaneously with 10 µg of keyhole limpet hemocyanin-conjugated human sHB-EGF (KLH-conjugate) 3 times, and then 5 µg of KLH-conjugate or 5 µg of recombinant human sHB-EGF protein was intravenously injected as a final boost. We also performed co-immunization with the KLH-conjugate and human proHB-EGF-expressing cells. In brief, 1 × 107 proHB-EGF-expressing 293F cells were injected intraperitoneally 4 times on a weekly basis and at a final boost. KLH-conjugate was subcutaneously immunized at the first and fourth injections. Five CD1 mice (8 weeks old, Charles River) were immunized subcutaneously with 10 µg of KLH-conjugate or with 10 µg of BSA-conjugated sHB-EGF (BSA-conjugate) 3 times, followed by an intravenous final boost with 5 µg of the same antigen. Five C57BL and five C3H mice (8 weeks old, Japan Clea) were immunized with the KLH-conjugate alone in the same way. In all cases, other than the injection of the proHB-EGF-expressing cells, immunizations were performed every 2 weeks. Three days after the final boost, the spleen was isolated from each mouse, and the splenocytes were fused with P3X63Ag8U.1 mouse myeloma cells (American Type Culture Collection) at a ratio of 1:1 by using the Hybrimune Electrofusion System (Cyto Pulse Sciences). The fused cells were seeded in a semi-solid selective culture media including hypoxanthine-aminopterin-thymidine, and the grown hybridoma clones were manually transferred to 96-well plates. Hybridomas secreting anti-HB-EGF mAbs were screened using FMAT. First, biotinylated sHB-EGF was prepared with Biotin Labeling Kit-NH2 (Dojindo). Next, the biotinylated sHB-EGF was captured with streptavidin beads (Polysciences), and then incubated with hybridoma culture supernatant and Cy5-labeled anti-mouse IgG Fc antibody (Jackson ImmunoResearch Laboratories) in Can Get Signal solution I (Toyobo) in FMAT 384-well plates (Applied Biosystems) for 7 h at room temperature. The mAb bound to the beads was detected by measuring fluorescence intensity of Cy5 by 8200 Cellular Detection System (Applied Biosystems).
The animal studies were performed in strict accordance with the recommendations in the Guide for the Care and Use of Laboratory Animals of the National Institutes of Health. The protocol was approved by the Committee on the Ethics of Animal Experiments of Takeda Pharmaceutical Company Limited (Permit Number: 2802).
mAb preparation
Hybridoma clones secreting anti-HB-EGF mAbs were expanded in a culture media containing 10% Ultra-low IgG fetal bovine serum (Invitrogen). Hybridoma culture supernatant was mixed with rProteinA Sepharose in MAPSII Binding Buffer (Bio-Rad) and incubated with end-over-end rotation overnight at 4°C. After a washing step with PBS, the bound mAb was eluted with 0.1 M glycine-HCl (pH 3.0) containing 0.15 M NaCl and was immediately neutralized with 1 M TRIS-HCl (pH 8.0). The buffer was then exchanged to PBS by ultrafiltration with Amicon Ultra-4 Centrifugal Filter (Millipore).
sHB-EGF/EGFR-hFc binding assay
The inhibitory activity of the hybridoma culture supernatant against the binding of sHB-EGF to EGFR-Fc was detected as described previously.Citation26 In brief, recombinant EGFR-hFc protein was captured by anti-human IgG Fc antibody (Jackson ImmunoResearch Laboratories) immobilized on 96-well plates. Hybridoma culture supernatant was incubated with 6 ng/mL biotinylated sHB-EGF and with 50 ng/mL sodium heparin on the plates for 1 h at 37°C, followed by incubation with horseradish peroxidase-labeled (HRP-labeled) streptavidin (Jackson ImmunoResearch Laboratories) for 1 h at 37°C. The plates were washed with PBS containing 0.05% Tween-20 after each incubation step. SureBlue TMB Microwell Substrate (KPL) was added to each well and incubated for 2 min. The reaction was stopped by 1 M H2SO4 and the absorbance at 450 nm was measured by a SPECTRA MAX plate reader (Molecular Devices). The inhibitory activity of the hybridoma culture supernatant was determined by measuring the binding of sHB-EGF to EGFR-Fc. We selected 210 hybridoma clones, whose culture supernatant inhibited sHB-EGF binding to EGFR by more than 70% and confirmed their inhibitory activity by an EGFR phosphorylation assay.
EGFR phosphorylation assay
EGFR phosphorylation was detected as described previously.Citation27 Briefly, SK-OV-3 ovarian cancer cells (American Type Tissue Collection) were seeded at 1 × 104 cells/well in McCoy’s 5A medium containing 10% fetal bovine serum in a 96-well plate for 1 d and were then serum-starved for 1 d. Hybridoma culture supernatant or various concentrations of purified mAb were pre-incubated with 10 ng/mL human sHB-EGF for 30 min at room temperature, and the reaction mixture was added to SK-OV-3 cells. After a 30 min incubation period at 37°C, phosphorylated EGFR was detected by Human Phospho-EGFR DuoSet IC ELISA Kit (R&D Systems). Inhibitory activity of hybridoma culture supernatant was determined by measuring the phosphorylation of EGFR. All the culture supernatants tested inhibited the sHB-EGF-induced EGFR phosphorylation by more than 50%. To accurately measure the neutralizing activity of the mAb clones, we confirmed their neutralizing activity with protein A-purified antibodies. In the case of purified mAbs, the phosphorylation of EGFR in the presence of each purified mAb was calculated as the percentage of the maximum phosphorylation induced by sHB-EGF in the absence of mAb. The maximum phosphorylation was considered as 100% and basal phosphorylation in the absence of sHB-EGF and mAb as 0%. IC50 values of purified mAbs were calculated using Prism (GraphPad).
Colony formation assay
Colony formation assay was performed as described previously.Citation28 In brief, RMG-I ovarian cancer cells (Japanese Collection of Research Bioresources) were seeded at 750 cells/well in RPMI1640 containing 0.35% agarose, 10% fetal bovine serum and 1 ng/mL human sHB-EGF in the presence or absence of 10 µg/mL anti-HB-EGF mAb, into Hydrocell 96-well plates (CellSeed). After a 1-week culture period, formed colonies were stained with 0.5 μM Calcein-AM (Invitrogen) and detected with an Acumen eXCitation3 instrument (TTP Labtech). The number of colonies per well in the presence of anti-HB-EGF mAb was calculated as the percentage of the number of colonies induced by sHB-EGF in the absence of anti-HB-EGF mAb. The number of colonies in the absence of sHB-EGF and mAb was considered 0%. IC50 values of purified mAbs were calculated using an SAS preclinical package (SAS).
Species specificity test
ELISA was performed as described previously.Citation29 Human, mouse or rat sHB-EGF was immobilized at a concentration of 1 μg/mL onto 96-well plates overnight at 4°C. Various concentrations of anti-HB-EGF mAb were then added to each well and incubated for 1 h at 37°C, followed by incubation with HRP-labeled anti-mouse IgG antibody (Jackson ImmunoResearch Laboratories). The plates were washed with PBS containing 0.05% Tween-20 after each incubation step. SureBlue TMB Microwell Substrate was then added to the 96-well plates, and the reaction was stopped after 2 min by adding 1 M H2SO4. The mAb binding to sHB-EGF was detected by measuring the absorbance at 450 nm by a Spectra Max plate reader. EC50 values were calculated with Prism.
Flow cytometry
Human proHB-EGF expression plasmid was prepared by cloning the full-length human proHB-EGF cDNA into pEF1 vector (Invitrogen). 293F cells were transfected with the proHB-EGF expression plasmid as described above. Two days after transfection, the cells were reacted with 2 μg/mL anti-HB-EGF mAb for 1 h on ice, followed by incubation with 2 μg/mL Alexa488-labeled anti-mouse IgG (Invitrogen) for 1 h on ice. The cells were washed with PBS containing 2% fetal bovine serum after each antibody incubation step. The binding of anti-HB-EGF mAb was measured by detecting fluorescence intensity with a Cytomics FC 500 instrument (Beckman Coulter). The median fluorescence intensity (MFI) ratio was calculated by dividing the MFI of mAb-treated sample by that of a control mAb-treated sample. MFI ratio was then used as a measure of the binding activity to proHB-EGF. The control sample was prepared by incubating cells with mouse IgG control (BD PharMingen) instead of anti-HB-EGF mAb.
Epitope binning study
The epitope binning study was performed using the FMAT assay as described previously.Citation21 In brief, anti-HB-EGF mAbs were biotinylated with Biotin Labeling Kit-NH2. In brief, polystyrene beads (Spherotech) were coated with human sHB-EGF and incubated with 0.1 μg/mL biotinylated anti-HB-EGF mAb, 5 μg/mL unlabeled anti-HB-EGF mAb and 0.33 μg/mL streptavidin-Alexa Fluor 647 (Invitrogen) in Can Get Signal solution I in FMAT 384-well plates for 7 h at room temperature. Biotinylated mAbs on the beads were detected by measuring Alexa Fluor 647 with 8200 Cellular Detection System. Inhibited binding of the biotinylated mAb to sHB-EGF by unlabeled mAb was calculated using the formula:
Binding inhibition (%) = (1 − A/B) × 100
where A represents total fluorescence in the presence of unlabeled mAb and B represents total fluorescence in the absence of unlabeled mAb. Clustering analysis was performed with the percent inhibition of all combinations of mAb pairs by using Ward’s methods with Euclidean distance. We first classified the mAbs into 3 epitope bins, namely, A, B and C, which were distinguished by the hierarchical dendrogram and the color-coded map (). We then subdivided epitope bin A into A1, A2, A3 and A4, and epitope bin C into C1 and C2, based on visual inspection of the hierarchical dendrogram ().
Abbreviations: | ||
BSA-conjugate | = | BSA-conjugated sHB-EGF |
EGF | = | epidermal growth factor |
EGFR | = | EGF receptor |
ELISA | = | enzyme-linked immunosorbent assay |
FMAT | = | fluorometric microvolume assay technology |
HB-EGF | = | heparin-binding EGF-like growth factor |
HRP | = | horseradish-peroxidase |
KLH | = | keyhole limpet hemocyanin |
KLH-conjugate | = | KLH-conjugated sHB-EGF |
MFI | = | median fluorescence intensity |
proHB-EGF | = | membrane-bound form of HB-EGF |
sHB-EGF | = | soluble form of HB-EGF |
Acknowledgments
The authors thank Hiroyuki Kajiwara for the preparation of sHB-EGF and EGFR-hFc proteins, and Dr. Toshio Kokubo for the helpful discussion.
Disclosure of Potential Conflicts of Interest
No potential conflicts of interest were disclosed.
Author’s contribution
I.T. generated anti-HB-EGF mAbs, executed an epitope binning study, sHB-EGF-binding ELISA, and EGFR phosphorylation assay. S.S. and I.T. wrote the manuscript. K.O. tested the binding activity of anti-HB-EGF mAbs to proHB-EGF. T.W. performed colony formation assay. T.K. supervised antibody generation and critically reviewed the manuscript. All authors contributed to experiment designs and coordinated the studies. All authors have read and approved the final version of the manuscript.
References
- Higashiyama S, Lau K, Besner GE, Abraham JA, Klagsbrun M. Structure of heparin-binding EGF-like growth factor. Multiple forms, primary structure, and glycosylation of the mature protein. J Biol Chem 1992; 267:6205 - 12; PMID: 1556128
- Higashiyama S, Abraham JA, Miller J, Fiddes JC, Klagsbrun M. A heparin-binding growth factor secreted by macrophage-like cells that is related to EGF. Science 1991; 251:936 - 9; http://dx.doi.org/10.1126/science.1840698; PMID: 1840698
- Kobrin MS, Funatomi H, Friess H, Buchler MW, Stathis P, Korc M. Induction and expression of heparin-binding EGF-like growth factor in human pancreatic cancer. Biochem Biophys Res Commun 1994; 202:1705 - 9; http://dx.doi.org/10.1006/bbrc.1994.2131; PMID: 8060360
- Mishima K, Higashiyama S, Asai A, Yamaoka K, Nagashima Y, Taniguchi N, et al. Heparin-binding epidermal growth factor-like growth factor stimulates mitogenic signaling and is highly expressed in human malignant gliomas. Acta Neuropathol 1998; 96:322 - 8; http://dx.doi.org/10.1007/s004010050901; PMID: 9796995
- Miyamoto S, Hirata M, Yamazaki A, Kageyama T, Hasuwa H, Mizushima H, et al. Heparin-binding EGF-like growth factor is a promising target for ovarian cancer therapy. Cancer Res 2004; 64:5720 - 7; http://dx.doi.org/10.1158/0008-5472.CAN-04-0811; PMID: 15313912
- Naef M, Yokoyama M, Friess H, Büchler MW, Korc M. Co-expression of heparin-binding EGF-like growth factor and related peptides in human gastric carcinoma. Int J Cancer 1996; 66:315 - 21; http://dx.doi.org/10.1002/(SICI)1097-0215(19960503)66:3<315::AID-IJC8>3.0.CO;2-1; PMID: 8621250
- Thøgersen VB, Sørensen BS, Poulsen SS, Orntoft TF, Wolf H, Nexo E. A subclass of HER1 ligands are prognostic markers for survival in bladder cancer patients. Cancer Res 2001; 61:6227 - 33; PMID: 11507076
- Hoffmann AC, Danenberg KD, Taubert H, Danenberg PV, Wuerl P. A three-gene signature for outcome in soft tissue sarcoma. Clin Cancer Res 2009; 15:5191 - 8; http://dx.doi.org/10.1158/1078-0432.CCR-08-2534; PMID: 19671876
- Tanaka Y, Miyamoto S, Suzuki SO, Oki E, Yagi H, Sonoda K, et al. Clinical significance of heparin-binding epidermal growth factor-like growth factor and a disintegrin and metalloprotease 17 expression in human ovarian cancer. Clin Cancer Res 2005; 11:4783 - 92; http://dx.doi.org/10.1158/1078-0432.CCR-04-1426; PMID: 16000575
- Hamaoka M, Chinen I, Murata T, Takashima S, Iwamoto R, Mekada E. Anti-human HB-EGF monoclonal antibodies inhibiting ectodomain shedding of HB-EGF and diphtheria toxin binding. J Biochem 2010; 148:55 - 69; http://dx.doi.org/10.1093/jb/mvq033; PMID: 20332144
- Khong TF, Fraser S, Katerelos M, Paizis K, Hill PA, Power DA. Inhibition of heparin-binding epidermal growth factor-like growth factor increases albuminuria in puromycin aminonucleoside nephrosis. Kidney Int 2000; 58:1098 - 107; http://dx.doi.org/10.1046/j.1523-1755.2000.00267.x; PMID: 10972674
- Miyamoto S, Iwamoto R, Furuya A, Takahashi K, Sasaki Y, Ando H, et al. A novel anti-human HB-EGF monoclonal antibody with multiple antitumor mechanisms against ovarian cancer cells. Clin Cancer Res 2011; 17:6733 - 41; http://dx.doi.org/10.1158/1078-0432.CCR-11-1029; PMID: 21918176
- Stollar BD, Fuchs S, Mozes E. Immune response of mice to nucleic acids: strain-dependent differences in magnitude and class of antibody production. J Immunol 1973; 111:121 - 9; PMID: 4576583
- Hoffenbach A, Lagrange PH, Bach MA. Strain variation of lymphokine production and specific antibody secretion in mice infected with Mycobacterium lepraemurium. Cell Immunol 1985; 91:1 - 11; http://dx.doi.org/10.1016/0008-8749(85)90026-7; PMID: 3882240
- Kasai K, Teuscher C, Smith S, Matzner P, Tung KS. Strain variations in anti-sperm antibody responses and anti-fertility effects in inbred mice. Biol Reprod 1987; 36:1085 - 94; http://dx.doi.org/10.1095/biolreprod36.5.1085; PMID: 3620556
- Baraldo K, Mori E, Bartoloni A, Petracca R, Giannozzi A, Norelli F, et al. N19 polyepitope as a carrier for enhanced immunogenicity and protective efficacy of meningococcal conjugate vaccines. Infect Immun 2004; 72:4884 - 7; http://dx.doi.org/10.1128/IAI.72.8.4884-4887.2004; PMID: 15271954
- Defendini ML, Pierres M, Regnier-Vigouroux A, Rochat H, Granier C. Epitope mapping of apamin by means of monoclonal antibodies raised against free or carrier-coupled peptide. Mol Immunol 1990; 27:551 - 8; http://dx.doi.org/10.1016/0161-5890(90)90074-A; PMID: 1696354
- Karle S, Nishiyama Y, Taguchi H, Zhou YX, Luo J, Planque S, et al. Carrier-dependent specificity of antibodies to a conserved peptide determinant of gp120. Vaccine 2003; 21:1213 - 8; http://dx.doi.org/10.1016/S0264-410X(02)00504-2; PMID: 12559800
- Amagai M. Autoimmune and infectious skin diseases that target desmogleins. Proc Jpn Acad Ser B Phys Biol Sci 2010; 86:524 - 37; http://dx.doi.org/10.2183/pjab.86.524; PMID: 20467217
- Ladner RC. Mapping the epitopes of antibodies. Biotechnol Genet Eng Rev 2007; 24:1 - 30; PMID: 18059626
- Swartzman EE, Miraglia SJ, Mellentin-Michelotti J, Evangelista L, Yuan PM. A homogeneous and multiplexed immunoassay for high-throughput screening using fluorometric microvolume assay technology. Anal Biochem 1999; 271:143 - 51; http://dx.doi.org/10.1006/abio.1999.4128; PMID: 10419629
- Defize LH, Boonstra J, Meisenhelder J, Kruijer W, Tertoolen LG, Tilly BC, et al. Signal transduction by epidermal growth factor occurs through the subclass of high affinity receptors. J Cell Biol 1989; 109:2495 - 507; http://dx.doi.org/10.1083/jcb.109.5.2495; PMID: 2553748
- Lax I, Bellot F, Howk R, Ullrich A, Givol D, Schlessinger J. Functional analysis of the ligand binding site of EGF-receptor utilizing chimeric chicken/human receptor molecules. EMBO J 1989; 8:421 - 7; PMID: 2785915
- Lax I, Fischer R, Ng C, Segre J, Ullrich A, Givol D, et al. Noncontiguous regions in the extracellular domain of EGF receptor define ligand-binding specificity. Cell Regul 1991; 2:337 - 45; PMID: 1716463
- Ogiso H, Ishitani R, Nureki O, Fukai S, Yamanaka M, Kim JH, et al. Crystal structure of the complex of human epidermal growth factor and receptor extracellular domains. Cell 2002; 110:775 - 87; http://dx.doi.org/10.1016/S0092-8674(02)00963-7; PMID: 12297050
- Jin P, Zhang J, Beryt M, Turin L, Brdlik C, Feng Y, et al. Rational optimization of a bispecific ligand trap targeting EGF receptor family ligands. Mol Med 2009; 15:11 - 20; http://dx.doi.org/10.2119/molmed.2008.00103; PMID: 19048033
- Giorgio C, Hassan Mohamed I, Flammini L, Barocelli E, Incerti M, Lodola A, et al. Lithocholic acid is an Eph-ephrin ligand interfering with Eph-kinase activation. PLoS One 2011; 6:e18128; http://dx.doi.org/10.1371/journal.pone.0018128; PMID: 21479221
- Beerli RR, Hynes NE. Epidermal growth factor-related peptides activate distinct subsets of ErbB receptors and differ in their biological activities. J Biol Chem 1996; 271:6071 - 6; http://dx.doi.org/10.1074/jbc.271.11.6071; PMID: 8626392
- Engvall E, Perlmann P. Enzyme-linked immunosorbent assay (ELISA). Quantitative assay of immunoglobulin G. Immunochemistry 1971; 8:871 - 4; http://dx.doi.org/10.1016/0019-2791(71)90454-X; PMID: 5135623