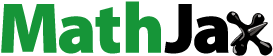
Abstract
Amyloid β (Aβ) immunotherapy is considered a promising approach to Alzheimer disease treatment. In contrast to the use of complete antibodies, administration of single-chain variable fragments (scFv) has not been associated with either meningoencephalitis or cerebral hemorrhage. ScFv-h3D6 is known to preclude cytotoxicity of the Aβ1–42 peptide by removing its oligomers from the amyloid pathway. As is the case for other scFv molecules, the recombinant production of scFv-h3D6 is limited by its folding and stability properties. Here, we show that its urea-induced unfolding pathway is characterized by the presence of an intermediate state composed of the unfolded VL domain and the folded VH domain, which suggests the VL domain as a target for thermodynamic stability redesign. The modeling of the 3D structure revealed that the VL domain, located at the C-terminal of the molecule, was ending before its latest β-strand was completed. Three elongation mutants, beyond VL-K107, showed increased thermodynamic stability and lower aggregation tendency, as determined from urea denaturation experiments and Fourier-transform infrared spectroscopy, respectively. Because the mutants maintained the capability of removing Aβ-oligomers from the amyloid pathway, we expect these traits to increase the half-life of scFv-h3D6 in vivo and, consequently, to decrease the effective doses. Our results led to the improvement of a potential Alzheimer disease treatment and may be extrapolated to other class-I scFv molecules of therapeutic interest.
Introduction
In recent years, amyloid β (Aβ) immunotherapy has been considered a promising approach for Alzheimer disease (AD) treatment.Citation1-Citation3 In August 2012, however, Phase 3 trials of intravenously administered bapineuzumab were halted due to disappointing results.Citation4 In previous Phase 2 trials, adverse effects of bapineuzumab, a humanized monoclonal antibody (mAb) were mostly mild and transient, but vasogenic edema occurred.Citation5 This side effect was dose-related and most cases were reported in APOE4 carriers. Dose restriction and recruitment of only APOE4 non-carriers into another Phase 3 did not improve the outcome.Citation4 This failure does not imply that researchers should abandon the search for a molecule targeting and clearing Aβ oligomers. It is worth mentioning that these trials were performed too late in the disease course, as claimed in the World Alzheimer Report 2011.Citation6 It is also plausible that drawbacks to the use of full-length antibodies could be prevented by using an antibody fragment that does not contain the Fc region, which is responsible for the activation of the microglia.Citation7 Therefore, the use of single-chain variable fragments (scFv) has been proposed as a hopeful therapeutic strategy.Citation8-Citation13
We previously described the recombinant expression and aggregation pathway of scFv-h3D6, a single chain variable fragment derived from mAb-h3D6,Citation14-Citation16 which inhibits amyloid fibril formation and cytotoxicity of the Aβ1–42-peptide.Citation13 Addition of scFv-h3D6 completely precluded the toxic effect of Aβ-oligomers in the SH-SY5Y neuroblastoma cell line. The study of the conformational properties of scFv-h3D6 upon heat treatment showed a conformational reorganization of the native state at ~60°C that led to the formation of an aggregation-prone intermediate state. This aggregation pathway did not correspond to an amyloid fibril pathway, as that followed by the Aβ-peptide, but rather to a worm-like fibril pathway which, noticeably, turned out to be non-toxic. In addition, this pathway was thermodynamically and kinetically favored when the scFv-h3D6 and Aβ1–42-oligomers formed a complex in native conditions, explaining how the scFv-h3D6 withdraws Aβ1–42 oligomers from the amyloid pathway in cell culture.
Because the high-yield production of scFvs is limited by their folding and stability properties, we examined the unfolding pathway of scFv-h3D6. The chemically-induced unfolding is characterized by the presence of an intermediate state, composed of the unfolded VL domain and the folded VH domain, suggesting the VL domain as the target for thermodynamic stability redesign. For this purpose, we modeled the 3D-structure of scFv-h3D6 and observed that the VL domain, located at the C-terminus of the molecule, was ending before its latest β-strand was completed. Three elongation mutants showed increased thermodynamic stability and lower aggregation tendency as determined by urea-denaturation experiments and Fourier-transform infrared spectroscopy (FTIR), respectively. Because the mutants maintained the ability to drive Aβ-oligomers to the non-toxic worm-like pathway, we expect these traits to increase the half-life of scFv-h3D6 and, consequently, to decrease the effective doses in the same mouse model in which the original form has proven to be beneficial.Citation17
Results
Secondary structure, unfolding pathway and stability of scFv-h3D6
In agreement with previous results,Citation13 the FTIR spectrum of scFv-h3D6 decomposes in 64% native β-sheet component, 22% loops/turns component, 11% β-turns components and 3% of a low-frequency component (see later).
The far-UV circular dichroism (CD)-spectrum of the native scFv-h3D6 showed an ellipticity minimum at 218 nm and an ellipticity maximum at 200 nm, as expected from an all β-sheet fold (), but a second ellipticity minimum at 230 nm and a positive shoulder at 237 nm were found.Citation13 These anomalies are contributions from the aromatic or cystinyl side-chains within the far-UV.Citation18 The minimum at 230 nm was also reported for an IgG1-FcCitation19 and described for some VL domains,Citation20,Citation21 the latter attributed to the interaction of the aromatic residues with the conserved Trp35.
Figure 1. Secondary structure of the WT scFV-3D6h. Far-UV CD-spectra in different urea concentrations. (A) 0‒6 M urea. 0 M, black; 2.2 M urea, dark gray; 3.7 M urea, mild gray; 4.8 M, light gray; 6.0 M, faint gray. The spectrum of the native state shows two minima (218 nm and 230 nm), a maximum (200 nm, not shown) and a positive shoulder (237 nm). The initial minimum at 230 nm is maintained until 3.7 M urea. (B) 6–8.6 M urea. 0 M, black; 6.0 M urea, dark gray; 6.9 M urea, mild gray; 8.0 M, light gray; 8.6 M, faint gray. The positive shoulder at 237 nm is lost between 6.9 and 8 M urea and a pure random-coil conformation is not achieved even at 8.6 M urea.
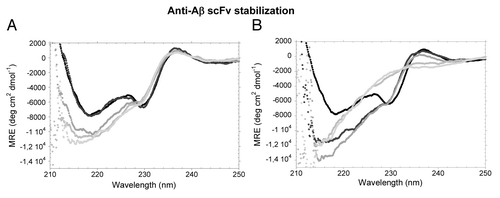
When denaturation of scFv-h3D6 by urea was followed by CD (), the initial ellipticity minimum at 230 nm was progressively lost as the concentration of urea was increased from 2 M to 3.7 M urea, while the ellipticity minimum at 218 nm accentuated and blue-shifted to 215 nm (). The ellipticity maximum at 237 nm was lost between 6.9 M and 8 M urea (), but a pure random-coil conformation was not achieved even at 8.6 M urea. This result indicated the presence of some residual secondary structure in the urea-denatured state. After dialyzing from the different urea concentrations to PBS, the initial spectrum was completely recovered, revealing that the chemical unfolding of scFv-h3D6 was 100% reversible (data not shown).
Chemical denaturation allows for the determination of the thermodynamic parameters in the unfolding process by plotting discrete points in equilibrium (). Trp-fluorescence is the best choice to follow unfolding processes because Trp residues are typically buried in hydrophobic cores of proteins and both the intensity and the location of the maximum of the emission spectrum change upon exposition to the solvent. Because the changes in the intensity of the spectra can increase or decrease upon unfolding when more than one Trp residue is present, as is the case for scFv-h3D6, they may mutually compensate each other and, therefore, this determination would make no sense.Citation22 This effect does not occur when analyzing data for emission maximum because the shift is always in the same direction, and thus the data for the red-shift reliably show the exposition of the Trp-residues to the solvent.Citation22 shows that the red-shift in the fluorescence emission spectra of the wild type (WT) scFv-h3D6 consists of two transitions with plateaus at ~351 and at 355 nm. The plateau at ~351 nm spans the 4–6 M urea region and is indicative of the occurrence of an intermediate state. The final plateau reaches the emission maximum considered for tryptophan-containing fully-unfolded proteins, 355 nm,Citation23 and therefore indicates that the tertiary structure is completely disrupted upon urea denaturation.
Figure 2. Urea denaturation of scFV-3D6h WT and its variants. The maximum of the Trp-fluorescence emission-spectra is plotted as a function of urea concentration. (A-D) Fitting to the three-state model. (A) WT, (B) C1, (C) C2, (D) C3. (E) Comparison of the fitted data. Two transitions are observed during the red-shift. The plateau in the 4–6M urea region is indicative of the occurrence of an intermediate state. (F) Magnification of the first transition shows incremented thermodynamic stability upon mutation. WT, black; C1, dark gray; C2, mild gray; C3, light gray.
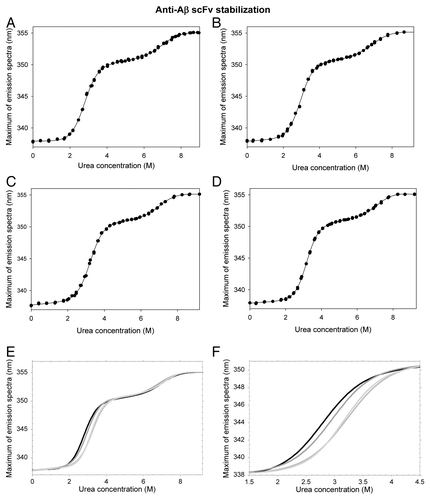
The fitting to the three-state model of the plot for emission maximum is shown in . The first transition corresponds to the unfolding of the less-stable domain (although interface disruption may also contribute to the unfolding process; see Discussion section), whereas the second transition corresponds to the unfolding of the more-stable domain from the intermediate state. The parameters for both transitions are similar when fitted to a two-state model (from 0 to 5.5 M urea and from 5 to 9.2 M). We can summarize that the free energy of unfolding in water of the less-stable domain (ΔGN-I) of the WT form, together with interface disruption, is -19.3 ± 0.7 KJ mol−1. The mI-N value, which reflects the difference in solvent accessibility between the intermediate state and the native state, is 7.0 ± 0.3 KJ mol−1 M−1 (). This value is similar to the m values published for correctly folded domains of similar sizes.Citation24 The thermodynamic stability of the more-stable domain (ΔGI-U) is -36.9 ± 5.7 KJ mol−1 and the mU-I value is 5.2 ± 0.8 KJ mol−1 M−1 (). The resulting [D]50% are 2.8 M and 7.1 M, respectively.
Table 1. Fitting to three-state model of equilibrium denaturation curves of the WT scFV-h3D6 and its variants. Fitting to the two-state model has been done for 0–5.5 M Urea and for 5–9.2 M Urea, to be compared with the three-state model
To characterize the equilibrium intermediate, limited proteolysis with thermolysin was performed at 5 M urea at 37°C (). After 1 h of proteolysis, a 17 kDa main-band accumulated; this band was blotted and N-terminal sequenced, rendering the sequence of the VH domain (GAMEVQ). Thus, the proteolysis-resistant band must consist of the VH domain (13.13 kDa) plus the linker region (0.96 kDa) and a small part of the VL domain (3.01 kDa; FR1 and half of CDR1, plausibly ending at VL-L27B, Kabbat numbering.Citation25,Citation26) The resistance to proteolysis of VL β-stands A, B and C in the intermediate suggests the maintenance of the long and stable β-hairpin that they form in the native state (not shown). In conclusion, the intermediate state in the urea denaturation curves consists of the unfolded VL domain and the folded VH domain, suggesting the VL domain as a target for thermodynamic stability redesign.
Figure 3. Limited proteolysis with thermolysin of the urea-induced intermediate analyzed by SDS-PAGE. (A) Proteolysis of the WT at 5 M Urea (mutants gave the same result, not shown). N-terminal sequencing of the blotted main-band (squared) rendered the sequence of the VH domain (GAMEVQ). (B) Kinetics of proteolysis at 3 M urea is different among variants. WT, black; C1, dark gray; C2, mild gray; C3, light gray.
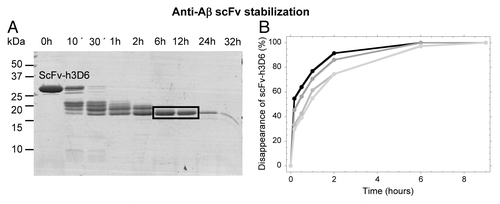
Three-dimensional model and redesign of the WT fold
Ig variable domains contain two antiparallel β-sheets packed tightly against each other in a compressed β-barrel (). The N- and C-terminus of each domain are located apart at the top and bottom of the β-barrel. One of the sheets contains five strands (A, B, C, C ', C,” the additional C’ and C” are about constant region domains) and the other sheet contains four strands (D, E, F, G). The complementary-determining regions (CDRs) are loops located in between B-C, C'-C,” and F-G β-strands and cluster at one end of the β-barrel. The fold is stabilized by hydrogen bonding between β-strands of each sheet, hydrophobic interaction between residues of opposite β-sheets and a disulfide bridge between two β-sheets (strands B and F, C22-C92 in VH and C23-C88 in VL; Kabat numberingCitation25,Citation26).
Figure 4. ScFv-h3D6 3D-model. (A) 3D-model. CDRs, blue; linker, black; Trp residues, red; disulphide bridges, yellow. (B) C-terminus detail showing the main interactions between VL-E105 and VL-K107 and the effect of OXT107-O.
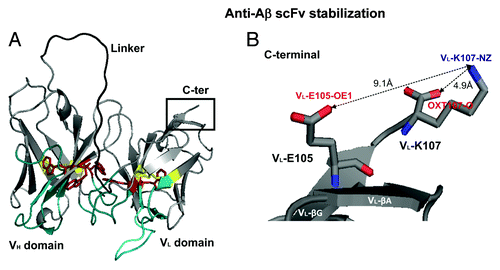
ScFv-h3D6 contains five tryptophan residues (, red), three in the VH domain (the conserved W36, W47 and W103, in strands C, C’ and G, respectively) and two in the VL domain (the conserved W35 in strand C and W89, the first residue of CDR3, in strand F). VH-W36 and VL-W35 constitute the center of the hydrophobic core in each domain, whereas VH-W47, VH-W103 and VL-W89, are located in the domains’ interface. As a point of reference, and an indication of the relevance of hydrophobic interactions in this interface, the distance between VH- W103-CZ2 and VL- W89-CH2 is 3.3 Å and that between VH-W47-CD1 and VL- W89-CZ2 is 5.7 Å (not shown).
Using graphical examination of the model structure, we found that the C-terminal end of the molecule, which corresponds to VL-K107, still belongs to β-strand G (, inset). Therefore, the side-chains of VL-K107 and VL-E105 are faced to the same side of the β-strand and should establish an electrostatic interaction. This electrostatic interaction is not properly performed since the distance between VL-K107-NZ and VL-E105-OE1 is 9.1 Å. This interaction might be weakened by the attraction of the VL-K107-NZ to the OXT107-O. The distance between VL-K107-NZ and the OXT107-O is 4.9 Å, which means that the side-chain of VL-K107 is bent toward the OXT107-O. When we examined the sequences of the 24 scFv molecules with similar sequence (see Material and methods), the structural alignment showed that 16 of them have as the C-terminal VL-K107, 6 VL-R108 and 2 VL-T109. Accordingly, in an attempt to stabilize the end of the β-strand G, we elongated one or two residues to generate the mutant forms VL-el-R108 and VL-el-R108T109, respectively. In addition, and to test our hypothesis on the above mentioned effect of OXT107-O on weakening the electrostatic interaction between VL-K107 and VL-E105, we extended the main-chain by just adding a Gly residue in mutant VL-el-R108G. For the sake of clarity, they will be henceforth referred to as C1 (VL-el-R108G), C2 (VL-el-R108) and C3 (VL-el-R108T109).
Secondary structure, unfolding pathway and stability of the mutants
As expected, the CD spectra of the mutants are slightly different to that of the native protein. In fact, mutation induces a more marked minimum at 230 nm (), plausibly indicating a more populated VL native-state upon stabilization.
Figure 5. Far-UV CD spectra of the scFV-3D6h variants in native conditions. WT, black; C1, dark gray; C2, mild gray; C3, light gray.
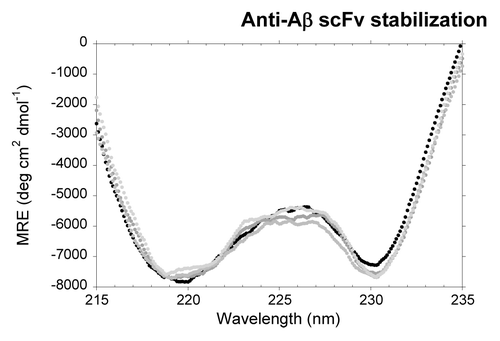
shows the urea denaturation curves of the mutants as a function of the red-shift in the fluorescence emission spectra. Compared with the WT curve (), the unfolding of the VL domain is similar for C1, whereas higher urea concentrations are required for C2 and C3. These forms are thus more stable than the WT scFv-h3D6. The fitting of these data () shows that thermodynamic stability of the variants improves in the order C1 (ΔΔG ≈1.7 KJ mol−1), C2 (ΔΔG ≈3.0 KJ mol−1) and C3 (ΔΔG ≈5.1 KJ mol−1). Cooperativity (mI-N value) is maintained in C1 and C2, and as a consequence [D]50% increases with thermodynamic stability (0.2 M and 0.5 M, respectively). In contrast, the most stable variant, C3, has an increased cooperativity that results in an increase [D]50% of just 0.5 M (similar to that of C2). In the case of the VH domain, determination of the equilibrium parameters is delicate because the red-shift of its transition is small (3–4 nm), i.e., SD is much higher than in the first transition; however, no differences are likely to exist among variants.
We wondered if these increases in stability would be reflected in the kinetics of thermolysin proteolysis in urea. As expected, at urea concentrations where the intermediate state populates all of the variants (i.e., 5 M), the kinetics is exactly that depictured for the WT in (not shown). At the mid-point of the first transition (i.e., 3 M), however, stabilization should decrease the population of the intermediate state. In full agreement, the disappearance of the initial band delays as the thermodynamic stability of the different mutants increases ().
Changes in the heat-induced aggregation pathway upon elongation of the C-terminus
The irreversible aggregation pathway of scFv-h3D6 is already reported and is featured by an intermediate state different from that populating the unfolding pathway.Citation13 Among other differences, thermolysin proteolysis showed that both domains of the heat-induced intermediate are equally susceptible to digestion. This intermediate is more abundant at ~60°C, and thermal denaturation followed by CD or fluorescence shows a conformational reorganization from the native state starting beyond 50°C ().This conformational reorganization is shifted to higher temperatures in all three mutants, indicating a diminished tendency toward aggregation upon extension of the C-terminus of the scFv by one or two residues.
Figure 6. Thermal denaturation of scFV-3D6h WT and its variants. (A) Ellipticity at 218 nm. (B) Trp-Fluorescence emission at 338 nm. WT, black; C1, dark gray; C2, mild gray; C3, light gray.
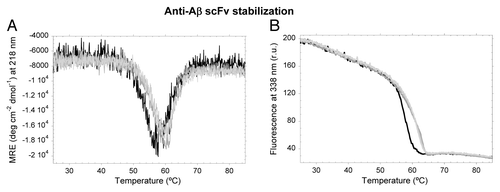
The WT scFv-h3D6 was reported to aggregate in the form of worm-like fibrils (WL), as shown by the visualization of curved fibrils by transmission electron microscopy (TEM) and by the appearance of a FTIR component centered at 1626 nm.Citation13
To study the WL component in the different variants, FTIR spectra were acquired at different temperatures (). At 25°C, there are no observable differences among variants in the native β-sheet component (1637 cm−1), which comprises ~65% of the area of the spectrum. The rest of the spectrum corresponds to loops/turns (1700–1660 cm−1) and is also similar among the mutants. This is not surprising since FTIR is a low-resolution spectroscopy and data treatment can mask small differences in secondary structure. At the temperature where the reorganization of the native state begins (50°C), the secondary structure is similar to the native form for all of the variants. In contrast, once the reorganization has led to the intermediate state (60°C), differences in aggregation tendency are reflected in the distribution of the FTIR components corresponding to β-structures.
Table 2. FTIR analysis of the WT scFV-h3D6 and its variants at different temperatures
For the WT, the main conformational transition from the native state to the intermediate state implies a substantial decrease in the native β-sheet component (1637 cm−1) in favor of the emergence of more compact β-sheet components (centered at lower frequencies; ). These components were previously attributed to WL (1627 cm−1) and amyloid (1616 cm−1) fibrils, and the WL component was shown to govern the spectra at 60°C.Citation13 When cooling is allowed, all three β-sheet bands increase at the expense of the loop/turn component, and the WL component finally contributes 26% to the area of the spectrum (, ).
Figure 7. Deconvolution and comparison of some FTIR spectra. (A) Native WT (25°C). (B) Renatured WT. (C) Renatured C1. (D) Renatured C2. (E) Renatured C3. (A-E) Band corresponding to native β-sheet is dotted and bands corresponding to fibril β-strand components are in black. (F) Comparison of spectra. Native WT, dotted; renatured WT black; renatured C1, dark gray; renatured C2, mild gray; renatured C3, light gray.
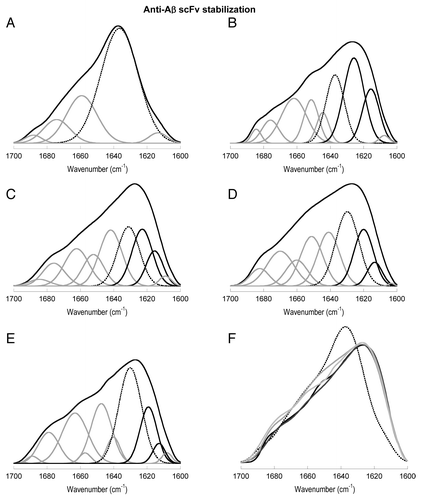
In the case of C1 (, ), more native β-sheet is kept in the intermediate state, the WL component does not predominate, and the amyloid component is diminished with respect to the WT. The maintenance of the native β-sheet component is also observed in the renatured states of C2 (, ) and, especially of C3 (, ). It is noticeable that the wavenumbers for all three β-sheet components are shifted to lower frequencies (~5 cm−1) in the mutants. The intermediate state of C3 shows these shifts in a more marked manner and, more importantly, has a different conformation. Concretely, the band for the amyloid component is negligible, the WL component is diminished, and the native β-sheet component is bigger than in the WT and mutants C1 and C2 (, ).
FTIR analysis allows the conclusion that the stabilization of the native state by extending the C-terminus drives to a destabilization of the heat-induced intermediate state and a subsequent decrease in the aggregation tendency of the molecule.
Although aggregation tendency has clearly diminished upon mutation, the WL fibril component is more or less present in all of the C-terminal extension mutants. We determined using TEM that, as described for the WT scFv-h3D6-Aβ complex, WL fibrils are formed from all of the variants (data not shown). The formation of WL fibrils by the scFv-h3D6:Aβ complex is the basis for the protective effect of scFv-h3D6,Citation13 and thus this result is critical to the future development of the variants. In conclusion, we observed that the extension of the C-terminus decreased the aggregation tendency of the isolated molecule, but not that of the Aβ1–42:scFv-h3D6 complex. We thus improved the fold without impairing its protective effect.
Discussion
ScFv-h3D6 unfolding classifies into Class I
In contrast to thermal denaturation,Citation13 urea denaturation led to the unfolding of the molecule and not to aggregation. The unfolding followed a three-state transition, as is reported for other scFv molecules.Citation27-Citation29
ScFv-h3D6 contains five buried Trp residues, one within the core of each domain (VH-W36, VL-W35) and three in the interface between both domains (VH-W47, VH-W103, VL-W89; Kabat numberingCitation30) (). These residues in the interface became completely exposed when the less-stable domain unfolded, thereby generating an intermediate state that was populated at ~5 M urea. Plückthun and coworkers observed that, because three processes occur in the unfolding of a scFv molecule (interface disruption, denaturation of one domain, denaturation of the other domain), the changes in the intensity of the spectra can increase or decrease and may mutually compensate each other. Therefore, the proper way to quantify Trp-fluorescence changes in the unfolding of any scFv molecule is following the emission maximum of the spectra, which displays a red-shift upon exposition to the solvent.Citation22
The same authors have proposed a model where, depending on the stability of the domains’ interface and on the intrinsic stability of each domain, unfolding of scFv molecules may be sequential.Citation27,Citation31 This model classifies the scFv fragments into four classes and, among them, class I is characterized by the intrinsic stability of one domain being significantly higher than the total stability (intrinsic plus interface contribution) of the other domain. In consequence, an unfolding intermediate state would accumulate in the equilibrium and a clear step in the unfolding curve would be detected. This step in the fluorescence emission maximum plot will be more evident when the first domain to unfold is VL because it will completely disrupt the interface, exposing the VH Trp residues. This is the case for scFv-h3D6, which shows 37 KJ mol−1 for the intrinsic stability of the VH domain and 19 KJ mol−1 for the sum of the intrinsic stability of the VL domain plus interface contribution.
The assignation of each domain to the transitions in the unfolding has been corroborated by limited proteolysis of the intermediate state and subsequent N-terminal sequencing of the proteolysis-resistant domain (VH). Also in consonance, the initial ellipticity minimum at 230 nm in the CD spectra, characteristic of some VL domains,Citation20,Citation21 progressively disappears during the first transition. These observations pointed out the VL domain of scFv-h3D6 as being the preferential target for protein stability redesign.
Elongation of the C-terminal domain increases stability and diminishes aggregation tendency of the scFv
The 3D-model of scFv-h3D6 allowed for the observation that the C-terminal end of the molecule, which corresponds to VL-K107, still belongs to β-strand G. Therefore, the side-chains of VL-K107 and VL-E105 are faced to the same side of the β-strand and establish an electrostatic interaction, which may be weakened by the proximity of the OXT107-O. Hence, elongation of the C-terminus should separate the OXT-O from the side-chain of VL-K107 and propitiate secondary structure stabilization. To test the possibility of the intrinsic stability of the VL domain being increased by elongation of its C-terminus, we examined the C-terminal residue of the 24 scFv molecules used in the structural alignment and determined that 16 of them finished at VL-K107, six at VL-R108 and two at VL-T109. Accordingly, mutants VL-el-R108 (C2) and VL-el-R108T109 (C3) were generated. In addition, mutant VL-el-R108G (C1) was constructed to test our hypothesis of the effect of OXT107-O on β-strand G packing.
The thermodynamic stability for the mutant elongated just with a Gly (C1) is slightly increased (). Although the difference is small (≈1.7 KJ mol−1), stabilization concurs with the fact that the aggregation tendency has been diminished as a consequence of the stabilization of the native state. Mutant C2, elongated with the natural Arg, is stabilized ≈3 KJ ml−1 and C3, ending with ArgThr, ≈5 KJ mol−1. Cooperativity is maintained in mutants extended by one residue, but increased in the case of C3, which is the most stable mutant. This increase in cooperativity indicates a more compact native state upon mutation.
Thermal denaturation followed by CD and Trp-fluorescence show the reorganization of the WT native state beginning beyond 50°C and finishing at ~60°C; however, this transition occurs at higher temperatures for the mutants.
Analysis of the IR amide I’ band at different temperatures allows deepening in the aggregation process because it has the capability of distinguishing among different β-sheet structures. The amide I’ band arises from the C = O stretching vibration of each peptidic bond and the strength of coupling depends greatly on the conformation of the polypeptide backbone. As a general rule, the position of β-sheet components shifts to lower wavenumbers as a result of increased hydrogen bonding, a more planar sheet or a larger number of strands.Citation32 There are no differences among WT and mutants spectra before thermal transition (25°C and 50°C), being dominated by the native β-sheet component (1637 cm−1). Once the reorganization has led to the intermediate state (60°C), more compact β-sheet components (centered at lower frequencies) appear. For the WT, these components were previously attributed to worm-like (WL) (1627 cm−1) and amyloid (1616 cm−1) fibrils, and the WL component was shown to govern the spectra at 60°C.Citation13 Upon extending the C-terminus of scFv-h3D6 progressively (from C1 to C3), more native β-sheet is kept in the intermediate state and the WL and amyloid components are diminished. Additionally, the center of the bands corresponding to β-sheet structure is shifted to lower wavenumbers in the mutants, which indicates a higher-density interaction-network among the side-chains. This is especially clear for the most stable mutant C3 (R108T109), where it is difficult to differentiate among bands because they are at the boundary between the values previously defined in the literature for different β-structures.Citation13,Citation32 Although it is tempting to correlate the different FTIR spectra of the heat-induced intermediate of mutant C3 to the higher cooperativity of its VL domain (mI-N value), we cannot conclude this putative correlation because mutants C1 and C2, which maintain the mI-N value, also showed a different FTIR spectra (despite to a lesser extent than that of C3).
In summary, stabilization of the native state has resulted in destabilization of the heat-induced intermediate state and subsequent decrease in the aggregation tendency of the fold. Furthermore, we have observed that the extension of the C-terminus has decreased the aggregation tendency of the isolated molecule, but not that of the Aβ1–42:scFv-h3D6 complex. In this way, we improved the fold without impairing its protective effect. This suggests that, in an in vivo model, these variants with increased thermodynamic stability and diminished aggregation tendency should show increased half-life and, consequently, be effective at lower doses than the WT form. The WT scFv-h3D6 has recently been shown to be beneficial in a mouse model of ADCitation17 and our next goal is to test the elongation mutants described here in the same model. Apart from the importance of improving a molecule with therapeutic potential for AD treatment, we believe these results can be extrapolated to other class-I scFv molecules of therapeutic interest.
Materials and Methods
Protein expression and mutagenesis
Protein expression in the form of a Trx-fusion precursor was performed as previously reportedCitation13 and a final cationic exchange chromatography was implemented to completely fractionate the native state from scrambled forms of the scFv-h3D6. Mutagenesis was performed by QuikChangeTM.
Secondary structure determination by CD
Protein secondary structure was monitored at different urea concentrations in PBS by far-UV CD spectroscopy from 260–200 nm in a Jasco J-715 spectrophotopolarimeter. The protein concentration was 20 μM, and 20 scans were recorded at 50 nm min−1 (response 2 sec) in 0.2-cm path-length cuvettes.
Secondary structure determination by FTIR
Samples at 100 μM in PBS were analyzed on a Variant Resolutions Pro spectrometer using a peltier mount and excavated cells with a 50-μm path (Reflex Analytical co.). Spectra were acquired at 25°C, 50°C, 60°C and after returning to 25°C. The samples were equilibrated for 5 min at the indicated temperature before recording. Recording and data treatment were as previously reported.Citation13
Thermal denaturation
Thermal denaturation was performed at protein concentration 20 μM and a rate of 1°C min−1, and followed by CD at 218 nm or Trp-fluorescence emission at 338 nm.
Chemical denaturation
Chemical denaturation was performed by creating four series of progressive urea concentrations, where protein was added at 2 μM. Samples were equilibrated overnight at room temperature before being tested for Trp-fluorescence at 25°C. Excitation was performed at 290 nm and emission spectrum recorded from 310 nm to 400 nm. Both slits were set to 5, and 10 scans were averaged in a Varian Cary Elipse fluorimeter. The maximum of each emission spectrum was obtained by fitting to a three-parametric polynomial.Citation33 Urea concentrations were calculated with a hand refractometer.Citation34 The equilibrium parameters for denaturation were calculated for each denaturant concentration ([D]) using Eq. 1:
where, FN = a + (b*x); FI = c + (d*x); FU = e + (h*x); k1 = exp(-ΔG1 / (R*T)); k2 = exp(-ΔG2 / (R*T)); ΔG1 = G1 - m1*x; ΔG2 = G2- m2*x;
and in which the dependence of the intrinsic fluorescence in the native (FN), intermediate (FI) and unfolded (FU) states upon increasing denaturant concentration (x) is taken into account by the terms b*x, d*x and h*x, respectively (linear approximation). With this kind of analysis, a three-state model for the chemical denaturation is being assumed, with one intermediate species significantly accumulating apart from the native and unfolded forms of the protein.
To compare, two separated two-state fittings, ranging 0‒5.5 M and 5‒9.2 M urea, were performed using Eq. 2:Citation34, Citation35
where, FN = a + (b*x); FU = c + (d*x); k = exp(-ΔG / (R*T); ΔG = G-m*x
Limited protease digestion studies
To characterize the equilibrium intermediate state present in the chemical denaturation curves, limited thermolysin digestion was performed in urea.Citation27 Thermolysin hydrolyzes peptide bonds on the N-terminal side of Val, Leu, Ile and Phe residues.Citation36 A ratio of thermolysin to scFv of 1:200 (w:w) was used in samples at 1 mg/ml of scFv-h3D6 in either 3 M or 5 M Urea, 20 mM TRIS-HCl, 10 mM CaCl2 (pH 7.8), the time-course of the digestion at 37°C was followed for 32 h, and the samples were stopped with SDS-loading buffer. Subsequent SDS-PAGE (18%), blotting and N-terminal sequencing of the main-accumulated band were performed.
Three-dimensional model of the WT scFv-h3D6
ScFv-h3D6 consists of the VH domain linked to the VL domain by a (Gly4Ser)3 linker. We searched for homologs with known structure among the sequences in the PDB for each domain using BLAST (Basic Local Alignment Search Tool).Citation37 Low-complexity regions of each non-redundant aligned sequence were filtered to discard false results. The PDB code of the sequence with the highest score coincident in the search of both domains was selected among a total of 24 candidates. The crystal structure of a scFv antibody against the SARS-spike protein-receptor binding-domain (with PDB code: 2GHW-B), matching the alignment with a 70% identity (94% similarity) and E-value 2e-84, was selected to construct a 3D model for the scFv-h3D6 using MODELER 9v2.Citation38 Five possible conformations were initially constructed for the VH and VL domains. Although the linker region is the same in both scFv molecules, (Gly4Ser)3, a defined diffraction pattern in the template structure (2GHW-B) could not be obtained because of the linker’s high degree of flexibility, and the coordinates for the model were calculated using the loop-refinement and energy minimization approach (MODELER 9v2Citation38). Ten different structures matched the five initial models for the domains and the best conformation with minimum energy was selected using the criterion of knowledge-based potentials of ProSa2003.Citation39
Abbreviations: | ||
AD | = | Alzheimer disease |
CD | = | circular dichroism |
FITR | = | Fourier-transformed infrared spectroscopy |
scFV | = | single chain variable Fragment |
WL | = | worm-like |
Acknowledgments
The authors acknowledge Dr Jose C. Martínez for critical comments on the manuscript. Instituto de Salud Carlos III (FIS-PI10–00975), Generalitat de Catalunya (SGR 2009–00761), FEDER (BIO2011–22568) and MICIN (BES-2009–024653). G.R-H is supported by a MAEC-AECI fellowship and M.M-A by a PIF (UAB) fellowship.
Submitted
03/17/2013
Revised
05/14/2013
Accepted
06/12/2013
Disclosure of Potential Conflicts of Interest
No potential conflict of interest was disclosed.
References
- Gouras GK. Immunotherapy for Alzheimer disease. MAbs 2009; 1:112 - 4; http://dx.doi.org/10.4161/mabs.1.2.7829; PMID: 20061820
- Panza F, Frisardi V, Solfrizzi V, Imbimbo BP, Logroscino G, Santamato A, et al. Immunotherapy for Alzheimer’s disease: from anti-β-amyloid to tau-based immunization strategies. Immunotherapy 2012; 4:213 - 38; http://dx.doi.org/10.2217/imt.11.170; PMID: 22339463
- Delrieu J, Ousset PJ, Caillaud C, Vellas B. ‘Clinical trials in Alzheimer’s disease’: immunotherapy approaches. J Neurochem 2012; 120:Suppl 1 186 - 93; http://dx.doi.org/10.1111/j.1471-4159.2011.07458.x; PMID: 21883222
- Thomas K. Trials for Alzheimer’s drug halted after poor results. The New York Times 2012.
- Rinne JO, Brooks DJ, Rossor MN, Fox NC, Bullock R, Klunk WE, et al. 11C-PiB PET assessment of change in fibrillar amyloid-beta load in patients with Alzheimer’s disease treated with bapineuzumab: a phase 2, double-blind, placebo-controlled, ascending-dose study. Lancet Neurol 2010; 9:363 - 72; http://dx.doi.org/10.1016/S1474-4422(10)70043-0; PMID: 20189881
- Prince M, Bryce R, Ferri C. World Alzheimer Report. The benefits of early diagnosis and intervention. Alzheimer’s Disease International 2011. http://www.alz.co.uk/research/WorldAlzheimerReport2011.pdf.
- Schenk D, Barbour R, Dunn W, Gordon G, Grajeda H, Guido T, et al. Immunization with amyloid-beta attenuates Alzheimer-disease-like pathology in the PDAPP mouse. Nature 1999; 400:173 - 7; http://dx.doi.org/10.1038/22124; PMID: 10408445
- Frenkel D, Solomon B, Benhar I. Modulation of Alzheimer’s beta-amyloid neurotoxicity by site-directed single-chain antibody. J Neuroimmunol 2000; 106:23 - 31; http://dx.doi.org/10.1016/S0165-5728(99)00232-5; PMID: 10814779
- Fukuchi K, Accavitti-Loper MA, Kim HD, Tahara K, Cao Y, Lewis TL, et al. Amelioration of amyloid load by anti-Abeta single-chain antibody in Alzheimer mouse model. Biochem Biophys Res Commun 2006; 344:79 - 86; http://dx.doi.org/10.1016/j.bbrc.2006.03.145; PMID: 16630540
- Zameer A, Kasturirangan S, Emadi S, Nimmagadda SV, Sierks MR. Anti-oligomeric Abeta single-chain variable domain antibody blocks Abeta-induced toxicity against human neuroblastoma cells. J Mol Biol 2008; 384:917 - 28; http://dx.doi.org/10.1016/j.jmb.2008.09.068; PMID: 18929576
- Robert R, Dolezal O, Waddington L, Hattarki MK, Cappai R, Masters CL, et al. Engineered antibody intervention strategies for Alzheimer’s disease and related dementias by targeting amyloid and toxic oligomers. Protein Eng Des Sel 2009; 22:199 - 208; http://dx.doi.org/10.1093/protein/gzn052; PMID: 18927231
- Pul R, Dodel R, Stangel M. Antibody-based therapy in Alzheimer’s disease. Expert Opin Biol Ther 2011; 11:343 - 57; http://dx.doi.org/10.1517/14712598.2011.552884; PMID: 21261567
- Marín-Argany M, Rivera-Hernández G, Martí J, Villegas S. An anti-Aβ (amyloid β) single-chain variable fragment prevents amyloid fibril formation and cytotoxicity by withdrawing Aβ oligomers from the amyloid pathway. Biochem J 2011; 437:25 - 34; http://dx.doi.org/10.1042/BJ20101712; PMID: 21501114
- Jacobson JS. Antibodies specific for epitopes within amyloid β (Aβ), for use in improving cognition. PCT Int Appl (2006), WO 2006066171 A1 20060622.
- Wisniewski T, Konietzko U. Amyloid-beta immunisation for Alzheimer’s disease. Lancet Neurol 2008; 7:805 - 11; http://dx.doi.org/10.1016/S1474-4422(08)70170-4; PMID: 18667360
- Panza F, Frisardi V, Imbimbo BP, Seripa D, Paris F, Santamato A, et al. Anti-β-amyloid immunotherapy for Alzheimer’s disease: focus on bapineuzumab. Curr Alzheimer Res 2011; 8:808 - 17; http://dx.doi.org/10.2174/156720511798192718; PMID: 21592055
- Villegas S, Rivera-Hernández G, Cervera L, Marín-Argany M, Blasco-Moreno B, Giménez-Llort L. Effects of scFv-h3D6 on 3xTg-AD mouse model of Alzheimer's disease: Cognitive improvement and modification of the Aβ-peptide aggregation pathway. 8th FENS 2012.
- Sreerama N, Manning MC, Powers ME, Zhang JX, Goldenberg DP, Woody RW. Tyrosine, phenylalanine, and disulfide contributions to the circular dichroism of proteins: circular dichroism spectra of wild-type and mutant bovine pancreatic trypsin inhibitor. Biochemistry 1999; 38:10814 - 22; http://dx.doi.org/10.1021/bi990516z; PMID: 10451378
- Traxlmayr MW, Faissner M, Stadlmayr G, Hasenhindl C, Antes B, Rüker F, et al. Directed evolution of stabilized IgG1-Fc scaffolds by application of strong heat shock to libraries displayed on yeast. Biochim Biophys Acta 2012; 1824:542 - 9; http://dx.doi.org/10.1016/j.bbapap.2012.01.006; PMID: 22285845
- Tsybovsky Y, Shubenok DV, Kravchuk ZI, Martsev SP. Folding of an antibody variable domain in two functional conformations in vitro: calorimetric and spectroscopic study of the anti-ferritin antibody VL domain. Protein Eng Des Sel 2007; 20:481 - 90; http://dx.doi.org/10.1093/protein/gzm034; PMID: 17962224
- Baden EM, Owen BA, Peterson FC, Volkman BF, Ramirez-Alvarado M, Thompson JR. Altered dimer interface decreases stability in an amyloidogenic protein. J Biol Chem 2008; 283:15853 - 60; http://dx.doi.org/10.1074/jbc.M705347200; PMID: 18400753
- Wörn A, Plückthun A. Different equilibrium stability behavior of ScFv fragments: identification, classification, and improvement by protein engineering. Biochemistry 1999; 38:8739 - 50; http://dx.doi.org/10.1021/bi9902079; PMID: 10393549
- Schmid FX. Optical spectroscopy to characterize protein conformation and conformational changes. In: Creighton TE, ed. Protein Structure: A Practical Approach. 2ond ed.: IRL Press, Oxford University Press, 1997:261-267.
- Myers JK, Pace CN, Scholtz JM. Denaturant m values and heat capacity changes: relation to changes in accessible surface areas of protein unfolding. Protein Sci 1995; 4:2138 - 48; http://dx.doi.org/10.1002/pro.5560041020; PMID: 8535251
- Wu TT, Kabat EA. An analysis of the sequences of the variable regions of Bence Jones proteins and myeloma light chains and their implications for antibody complementarity. J Exp Med 1970; 132:211 - 50; http://dx.doi.org/10.1084/jem.132.2.211; PMID: 5508247
- Johnson G, Wu TT. Kabat Database and its applications: future directions. Nucleic Acids Res 2001; 29:205 - 6; http://dx.doi.org/10.1093/nar/29.1.205; PMID: 11125092
- Wörn A, Plückthun A. Mutual stabilization of VL and VH in single-chain antibody fragments, investigated with mutants engineered for stability. Biochemistry 1998; 37:13120 - 7; http://dx.doi.org/10.1021/bi980712q; PMID: 9748318
- Ramm K, Gehrig P, Plückthun A. Removal of the conserved disulfide bridges from the scFv fragment of an antibody: effects on folding kinetics and aggregation. J Mol Biol 1999; 290:535 - 46; http://dx.doi.org/10.1006/jmbi.1999.2854; PMID: 10390351
- Jäger M, Plückthun A. Domain interactions in antibody Fv and scFv fragments: effects on unfolding kinetics and equilibria. FEBS Lett 1999; 462:307 - 12; http://dx.doi.org/10.1016/S0014-5793(99)01532-X; PMID: 10622716
- Kabat EA, Wu TT, Reid-Miller M, Perry H, Gottesman K, eds. Sequences of Proteins of Immunological Interest. Fourth Edition ed. US Govt. Printing Off. No. 165-492; 1987.
- Wörn A, Plückthun A. Stability engineering of antibody single-chain Fv fragments. J Mol Biol 2001; 305:989 - 1010; http://dx.doi.org/10.1006/jmbi.2000.4265; PMID: 11162109
- Zandomeneghi G, Krebs MR, McCammon MG, Fändrich M. FTIR reveals structural differences between native beta-sheet proteins and amyloid fibrils. Protein Sci 2004; 13:3314 - 21; http://dx.doi.org/10.1110/ps.041024904; PMID: 15537750
- Knappik A, Plückthun A. Engineered turns of a recombinant antibody improve its in vivo folding. Protein Eng 1995; 8:81 - 9; http://dx.doi.org/10.1093/protein/8.1.81; PMID: 7770457
- Pace CN, Scholtz M. In Protein structure, a practical approach (Creighton TE ed.), 1st edition. 1997:383.
- Pace CN. Determination and analysis of urea and guanidine hydrochloride denaturation curves. Methods Enzymol 1986; 131:266 - 80; http://dx.doi.org/10.1016/0076-6879(86)31045-0; PMID: 3773761
- Heinrikson RL. Applications of thermolysin in protein structural analysis. Methods Enzymol 1977; 47:175 - 89; http://dx.doi.org/10.1016/0076-6879(77)47022-8; PMID: 927174
- Altschul SF, Gish W, Miller W, Myers EW, Lipman DJ. Basic local alignment search tool. J Mol Biol 1990; 215:403 - 10; PMID: 2231712
- Sali A, Blundell TL. Comparative protein modelling by satisfaction of spatial restraints. J Mol Biol 1993; 234:779 - 815; http://dx.doi.org/10.1006/jmbi.1993.1626; PMID: 8254673
- Wiederstein M, Sippl MJ. ProSA-web: interactive web service for the recognition of errors in three-dimensional structures of proteins. Nucleic Acids Res 2007; 35:Web Server issue W407-10; http://dx.doi.org/10.1093/nar/gkm290; PMID: 17517781