Abstract
Antibodies are well-established as therapeutics, and the preclinical and clinical pipeline of these important biologics is growing rapidly. Consequently, there is considerable interest in technologies to engineer and manufacture them. Mammalian cell culture is commonly used for production because eukaryotic expression systems have evolved complex and efficient chaperone systems for the folding of antibodies. However, given the ease and manipulability of bacteria, antibody discovery efforts often employ bacterial expression systems despite their limitations in generating high titers of functional antibody. Open-Cell Free Synthesis (OCFS) is a coupled transcription-translation system that has the advantages of prokaryotic systems while achieving high titers of antibody expression. Due to the open nature of OCFS, it is easily modified by chemical or protein additives to improve the folding of select proteins. As such, we undertook a protein additive screen to identify chaperone proteins that improve the folding and assembly of trastuzumab in OCFS. From the screen, we identified the disulfide isomerase DsbC and the prolyl isomerase FkpA as important positive effectors of IgG folding. These periplasmic chaperones function synergistically for the folding and assembly of IgG, and, when present in sufficient quantities, gram per liter IgG titers can be produced. This technological advancement allows the rapid development and manufacturing of immunoglobulin proteins and pushes OCFS to the forefront of production technologies for biologics.
Introduction
Antibodies are remarkable proteins that function to specifically recognize and neutralize antigens associated with invading organisms and other diseases. Antibodies, or immunoglobulins, are higher order protein complexes that form a quaternary structure stabilized by interchain disulfide bridges. Immunoglobulin gamma (IgG) is a class of antibodies composed of two heavy chain (HC) and two light chain (LC) polypeptides. They are further modified by glycosylation in the conserved Fc region of the HC, which can help mediate interactions with other components of the immune system. Both HCs and LCs are composed of protein domains characterized by the β-stranded immunoglobulin fold. B cells or plasma cells are optimized for the folding and assembly of these complex molecules in the endoplasmic reticulum (ER).Citation1 The lumen of the ER is an oxidative environment, which is required to form and stabilize native intra and interchain disulfide bonds. In the ER, special chaperone proteins such as protein disulfide isomerases (PDI) catalyze disulfide bond formation and shuffling until the correct cysteine linkages are formed.Citation2,Citation3 Other chaperone families participate in the folding of IgG molecules, in particular of the HC polypeptide, which is difficult to fold.Citation1,Citation2,Citation4 BiP, an immunoglobulin HC binding protein, is a member of the hsp70 superfamily that associates to HC molecules during folding.Citation4-Citation6 In the monomeric state, HC proteins are prone to misfolding and require BiP for stabilization against aggregation. Once HC is able to bind to LC, a stable protein complex is formed. Prolyl isomerization has also been proposed to be a rate-limiting step in HC folding and peptidyl prolyl isomerases (PPIases) have been implicated in catalyzing this folding step.Citation4
Mammalian cells have evolved highly complex and efficient chaperone systems for the folding of immunoglobulins. However, because of their ease of use and manipulability, bacterial expression systems are often employed for antibody discovery techniques such as phage display or ribosome display. This has generated a need for bacterial systems that are specifically optimized for the folding of eukaryotic disulfide-bonded proteins. As such a technology, Open-Cell Free Synthesis (OCFS) utilizes a coupled transcription-translation system derived from cell extracts of rapidly dividing, engineered E. coli strains, for the expeditious generation of a single protein-of-interest.Citation7,Citation8 Due to the open nature of this system, it is easily modified by chemical or protein additives to improve the folding of select proteins. We thus undertook a protein additive screen to identify chaperone proteins that may improve the folding and assembly of the human epidermal growth factor receptor (HER)2-targeted trastuzumab in OCFS. We chose a limited subset of chaperones to screen based on their potential ability to functionally replace chaperones critical for the folding of IgGs in the eukaryotic ER. From the screen, we identified the disulfide isomerase DsbC and the prolyl isomerase FkpA as important positive effectors of IgG folding. These periplasmic chaperones function synergistically for the folding and assembly of IgG, and, when present in sufficient quantities, gram per liter IgG titers can be obtained in OCFS. This is in contrast to other bacterial systems that may produce 10–100 times less protein. This technological advancement may facilitate the rapid development and manufacturing of a variety of immunological proteins and contribute to the burgeoning therapeutic antibody business, which is projected to generate annual revenues of $58 billion by 2016.Citation9
Results
Chaperone sequential expression screen
In vivo, eukaryotic chaperones are known to play an important role in the folding and assembly of IgGs. Therefore, expression of IgG molecules in bacterial systems that lack these physiological foldases has been challenging.Citation10,Citation11 Given that bacterial expression systems still have several advantages over eukaryotic systems, we undertook a screening approach to identify positive effectors of IgG folding or assembly in a prokaryotic expression system (). Candidate chaperones were expressed in a coupled prokaryotic in vitro transcription/translation system (hereto referred as Open Cell-Free Synthesis, or OCFS) and expressed chaperones were subsequently titrated into new cell-free reactions for the expression of trastuzumab, a HER2-binding IgG1 molecule. IgG was translated in the presence of 14C-Leucine to allow quantification of expressed proteins and assembled IgG. Any improvements in IgG folding were expressed as a fold-improvement in titer over the addition of a GFP-expressing control extract (, ). To improve the throughput of the screen, chaperones were not purified from the extract, but were added to IgG reactions in the presence of spent extract. To ensure that chaperone DNA was not being transcribed and expressed in subsequent IgG reactions, chaperone proteins were expressed from PCR template that is significantly more labile than plasmid DNA. The addition of GamS protein helped preserve the PCR template so that sufficient levels of chaperone protein could be synthesized from the PCR amplified DNA templates.Citation12 This PCR DNA was still degraded by the end of the chaperone synthesis reaction as demonstrated by the lack of chaperone synthesis in the subsequent cell-free reactions (Fig. S1).
Figure 1. Chaperone Screen. (A) Schematic of screen for positive effectors of IgG folding or assembly. Positive effectors were expressed from a PCR template by open cell-free synthesis (OCFS). Crude preparations containing the positive effectors were diluted (at 5, 10, or 20% v/v) into a second 100 μl OCFS reaction for trastuzumab in the presence of 14C-Leucine as a tracer for heavy and light chain folding and assembly. (B and C) Positive effectors of IgG synthesis were identified by their ability to improve the folding or assembly of IgG as compared with a GFP-expressed negative control. DsbC, SlyD, and Skp are representative examples of positive effectors from functional groups controlling redox, prolyl isomerization, and deaggregation. (C) Improvements in IgG folding were expressed as a fold-change over the GFP control (20% v/v shown).
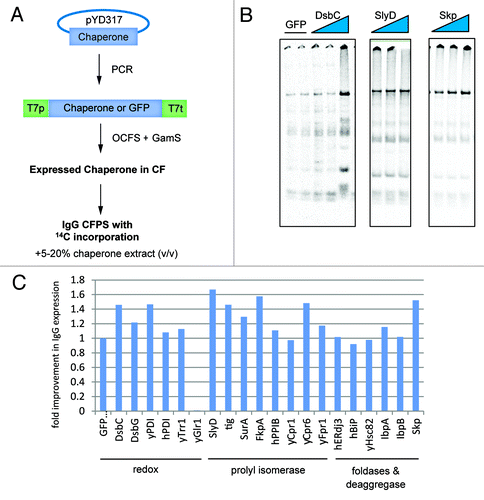
Several families of chaperones were of particular interest given their role in folding IgG in vivo. Redox proteins, PPIases, foldases and deaggregases from bacterial, yeast, and human species were tested (). Among the redox chaperones, we found that yeast PDI (yPDI) and DsbC significantly aided IgG formation, consistent with previous findings (, and ref. Citation7). Notably, human PDI (hPDI) did not significantly affect IgG folding, probably due to its poor expression in cell extract, which did not allow sufficient quantities to be added to IgG expression reactions (, ). By contrast, the bacterial protein DsbC expressed very well in the extract, allowing the addition of ~5 μM DsbC to the IgG reaction (DsbC was expressed at ~25 μM and it was added at 5%, 10%, and 20% v/v to an IgG reaction in ; 20% chaperone addition reactions were tabulated in ). Among the PPIases tested, several proved to be beneficial to IgG expression as well ().
Table 1. Expression titers of positive effectors in the screen
Interchangeability of PDI and DsbC
From the screen, as well as from previous work,Citation7,Citation8,Citation13 we had observed that both yeast PDI and bacterial DsbC had the ability to promote disulfide bond formation and IgG assembly in cell-free reactions. While both proteins are disulfide bond isomerases, it is possible that eukaryotic and prokaryotic isomerases may have evolved to specifically fold proteins found in their respective proteomes. This would predict that PDI would be superior at folding a eukaryotic substrate protein such as IgG. To better understand the dependence of IgG folding and assembly on eukaryotic and prokaryotic disulfide bond isomerases, IgG cell-free protein synthesis reactions were run at varying concentrations of PDI and DsbC. IgG was expressed in cell-free reactions in the presence of 0–5 μM PDI in combination with 0–13 μM DsbC. Expressed IgG-His6 was purified by Ni2+ resin and quantified by capillary electrophoresis (). Ni2+-based purification was utilized to avoid acidic elution conditions employed in Protein A purifications that might dissociate IgG complexes. In the absence of DsbC, IgG was highly dependent on PDI for folding (, closed circles). As the concentration of DsbC in the reaction increased, however, the dependence on PDI decreased such that, at 6.4 μM DsbC, there was no additional benefit attributable to PDI in the reaction (, open triangles). Furthermore, by increasing the concentration of DsbC in the reaction, we saw marked improvements in IgG titers beyond what we had previously observed (, open circles and ref. Citation7). In effect, we observed the efficient substitution of a eukaryotic disulfide bond isomerase with a bacterial chaperone of a similar function in the folding of a eukaryotic protein.
Figure 2. PDI and DsbC are interchangeable. The functional interchangeability of human and bacterial disulfide isomerases was tested by evaluating IgG expression titers at various different concentrations of PDI or DsbC in 100 μl OCFS reactions. IgG folding is dependent on PDI for folding and assembling (closed circles), but this dependence is diminished as the concentration of DsbC is increased.
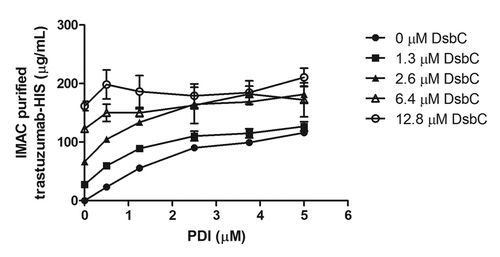
Purified Skp, SlyD, and FkpA can improve IgG titers
Among the positive effectors identified in the chaperone screen, we decided to further evaluate the bacterial proteins Skp, SlyD, and FkpA. To confirm that they benefited IgG folding, we expressed and purified Skp, SlyD, and FkpA and added them back into IgG cell-free protein synthesis reactions in the presence of 13 μM DsbC ( and S2). The chaperone Skp aided the solubility of HC and LC, but did not increase the amount of assembled IgG significantly. However, for the prolyl isomerases, SlyD and FkpA, we observed that the solubility of expressed proteins, as well as the proportion of assembled IgG, increased dramatically with added chaperone protein. We reasoned that prolyl isomerization was a function that may have been limiting for IgG formation in OCFS, and the addition of these exogenous proteins substantially improved IgG folding and assembly, consistent with previous observations.Citation14 Because of the improvements observed with DsbC and FkpA, we decided to further characterize their roles in IgG folding.
Figure 3. Validation of chaperone positive effectors. Purified Skp, SlyD, or FkpA were added into 100 μl IgG OCFS reactions containing 13 μM DsbC. (B) The soluble protein produced was measured by TCA-precipitating 14C-counts in the soluble fraction of the sample. (A and B) The assembled IgG was measured as the proportion of soluble proteins that migrated at 150 kDa in a non-reducing SDS-PAGE gel.
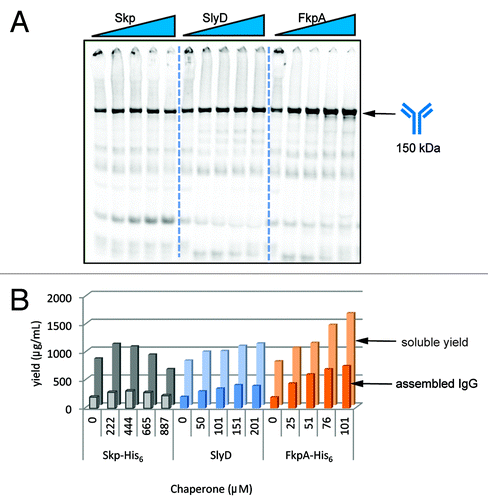
FkpA and DsbC have different roles in the folding and assembly of IgG
To better understand the roles that FkpA and DsbC play in IgG formation, we independently evaluated their contributions to IgG folding (). Purified FkpA and DsbC protein were titrated into cell extract derived from strain SBJY001,Citation7 and trastuzumab HC and LC were expressed in the presence of 14C-Leucine followed by visualization with SDS-PAGE and autoradiography. Notably, the addition of FkpA significantly reduced the degree of higher molecular weight aggregates formed during HC and LC synthesis. With increasing amounts of FkpA, we also observed the formation of IgG, as well as a number of partially assembled products. These proteins migrated as indistinct bands, suggesting that they may represent mixed populations of cross-disulfide bonded proteins. The addition of DsbC, on the other hand, generated IgG that migrated in clear, defined bands by SDS-PAGE. Without FkpA, a significant proportion of the expressed proteins formed higher order aggregates that could not completely enter the gel. Together, these results suggest that FkpA and DsbC have distinct roles in the folding of IgGs.
Figure 4. FkpA and DsbC-mediated folding. The independent effects of FkpA and DsbC on IgG folding were assessed. (A) The addition of FkpA to 100 μl IgG OCFS reactions significantly enhanced HC and LC solubility but had a limited impact on IgG assembly. (B) The addition of DsbC dramatically enhanced IgG assembly, but could not eliminate protein aggregation.
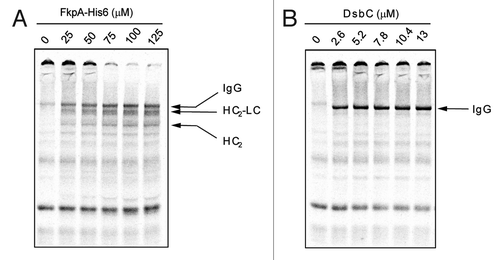
Developing extract with high levels of DsbC and FkpA
Adding high concentrations of purified chaperone proteins is expensive and labor-intensive, and we wanted to move toward a strain-engineered solution. As such, we undertook to develop bacterial strains that overexpressed DsbC and FkpA, which would then be present at high concentrations in the subsequent cell-free extract. Plasmids carrying single (1xDsbC) or double (2xDsbC) copies of the DsbC gene behind the constitutive promoter Mt-cons-10 were constructed and transformed into bacteria. ELISA quantitation of these bacterial extracts suggested that we had 1–2 g/L and 3–4 g/L of DsbC (or ~65 and ~150 μM, respectively) expressed in the 1xDsbC and 2xDsbC extracts, respectively (). IgG expression in OCFS with DsbC-containing extracts (2xDsbC) was very efficient, with a high proportion of soluble proteins forming assembled IgG (, lane 1).
Figure 5. Synergistic effects of FkpA and DsbC on IgG folding. (A) Plasmids driving constitutive expression of one (1x) or two (2x) copies of DsbC or FkpA genes were transformed into E. coli strain SBJY001. Cells were grown to log phase and cell extracts were prepared from the cell pellets. Expressed DsbC or FkpA were detected in the respective extracts by ELISA using a rabbit polyclonal sera. ‘2xD + 2xF’ contains 2 copies of chromosomally integrated DsbC and the 2xFkpA expression plasmid. (B) Purified FkpA was added to a 100 μl OCFS reaction expressing trastuzumab IgG using 2xDsbC extract. (C) Strain engineering of high chaperone concentrations in the extract allowed for increased expression of IgG.
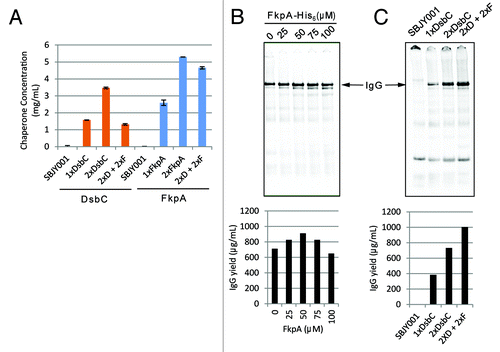
We then evaluated the synergy of combined DsbC and FkpA action on the folding of IgG (). Trastuzumab HC and LC were expressed in a DsbC-containing extract with exogenous FkpA protein added into the reactions. Addition of 50 μM FkpA significantly improved IgG folding and assembly (). Accordingly, we developed bacterial strains and the corresponding cell extracts that overexpress FkpA (). 2–3 g/L and 5 g/L FkpA (or ~90 and ~180 μM, respectively) could be achieved in the 1xFkpA and 2xFkpA cell extracts, respectively, which corresponds to intracellular concentrations as high as 15 g/L FkpA. However, given that FkpA alone is inadequate for IgG assembly (), a strain engineering solution was desired for both chaperones. Two tandem copies of the DsbC gene were chromosomally integrated, and the resultant strain was transformed with the 2xFkpA plasmid (2xD + 2xF). Although extract made from this strain had lower overall titers of DsbC compared with strains overexpressing only one chaperone (), the extract was nonetheless able to support gram per liter titers of trastuzumab in an overnight cell-free synthesis reaction ().
DsbC and FkpA-overexpressing extract can improve the expression and assembly of multiple IgGs
Having identified chaperones that dramatically improve the folding and assembly of trastuzumab in OCFS, we wanted to understand if our engineered extracts could also benefit the folding of other IgGs. Accordingly, we expressed a panel of IgGs in a control extract (SBJY001), DsbC extract (2xDsbC extract), and DsbC + FkpA extract (2xD + 2xF) (). Included in the panel were the therapeutic antibodies trastuzumab (an anti-HER2 IgG1) and brentuximab (an anti-CD30 IgG1), in addition to two germline HCs VH3–7 and VH3–23 in combination with the LC Vk3–20. Notably, DsbC was essential for the expression of all the IgGs tested. Further improvements were observed in the DsbC + FkpA extract, bringing expression levels to 1 g/L for both trastuzumab and brentuximab, and nearly 1.5 g/L for the germline IgGs. Together, these results suggest that the engineered extracts are likely to benefit the expression of a wide-range of immunoglobulin proteins in OCFS.
Figure 6. Chaperone-expressing extract improves the folding of multiple IgGs in OCFS. (A) trastuzumab, the CD30-targeted brentuximab, and the germline HC’s VH3–7 and VH3–23 in combination with the germline LC Vk3–20 were expressed in SBJY001, 2xDsbC, and 2xD + 2xF extracts in the presence of 14C-leucine and visualized by SDS-PAGE and autoradiography. (B) Assembled IgG expressed in the different extracts was quantified as described in the Materials and Methods.
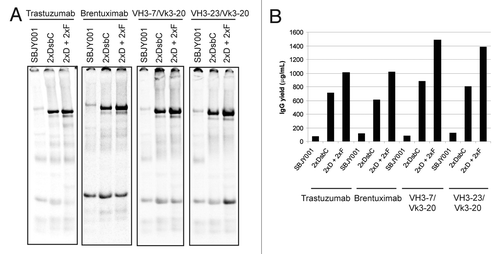
Discussion
The mammalian ER is a powerful system that can properly fold and assemble complex disulfide-bonded molecules such as IgGs. In an attempt to mimic the suite of different chaperone activities in the mammalian ER, chaperones from the same functional classes implicated in IgG folding were screened. In agreement with other reports, addition of disulfide isomerase dramatically improved IgG assembly.7,1513 In fact, addition of > 10 μM DsbC to our bacterial transcription/translation system improved IgG expression by more than 20-fold (). We identified two disulfide isomerases, DsbC from E. coli and PDI from S. cerevisiae that were capable of enhancing the production of IgG in OCFS. Surprisingly, DsbC was able to boost IgG assembly to a similar extent as PDI, despite having evolved as a redox catalyst for proteins in the bacterial periplasm. This suggests a striking functional overlap for these proteins despite limited sequence or substrate similarity.
Similar to the mammalian ER, high level IgG production also required the presence of a PPIase. From a panel of eight PPIases, the E. coli proteins FkpA and SlyD were identified for their ability to augment IgG folding and assembly during OCFS (Fig. One and 3). The hsp70 BiP is another chaperone reported to be highly upregulated during plasma cell differentiation and contributes to HC stabilization during IgG folding.Citation1,Citation2,Citation16 We did not, however, observe any benefit with the addition of human BiP or its cofactor human Erdj3, possibly due to the relatively low levels that were added into the IgG synthesis reactions (Fig. One and ). Given that these human chaperones expressed more poorly than bacterial proteins whose sequences have evolved to translate and fold efficiently in E. coli systems (), we were not able to test the effect of these chaperones on IgG folding over a wide range of concentrations. Another possibility for why a BiP-associated improvement was not observed is that the bacterial hsp70 DnaK and cofactor DnaJ are at sufficiently high concentrations in the extract that addition of another hsp70 would have little effect. Indeed, hsp70 proteins are highly conserved and abundant. The hsp70 DnaK has been estimated to be 1–2% of total protein (or 2–5 g/L DnaK) in E. coli.Citation17,Citation18 One deaggregase from our screen that did have a positive effect on IgG solubility was Skp, which modestly increased IgG titers. Because FkpA markedly increased HC solubility while also improving IgG assembly, we further investigated the role of FkpA.
We evaluated the different roles of FkpA and DsbC and found that the chaperones act synergistically to fold IgG. DsbC is critical for the formation of properly assembled and disulfide-bonded IgG (). The addition of FkpA further enhances the degree of IgG folding, likely through improved HC solubility and perhaps prolyl isomerization ( and ). Indeed, we have observed FkpA addition to significantly improve solubility in both small-scale and large-scale reactions (data not shown). FkpA has been reported to improve protein solubility as well as catalyze prolyl isomerization for several model proteins.Citation14,Citation19-Citation21 In addition, FkpA localized to the cytoplasm has also been shown to enhance secretion of both Fabs and scFv into the E. coli periplasm, possibly by helping each stay soluble long enough for export from the cytoplasm.Citation22 However, FkpA alone is insufficient to support IgG assembly (), highlighting the critical role of disulfide isomerases in IgG formation.
Addition of exogenous chaperones such as DsbC and FkpA is a powerful metric for understanding the functional requirements of IgG maturation. Keeping with our goal of modeling the eukaryotic ER, we were curious about whether DsbC and FkpA could be produced in vivo at titers sufficient to support high level IgG production in a cell free reaction. While the concentration of PDI is estimated to be as high as 2 mM in the mammalian ER,Citation23 the above experiments indicate that disulfide isomerase concentrations 2 orders of magnitude lower are sufficient for proper disulfide formation in OCFS (Fig. Two and 5B). To determine if the required chaperone levels could be achieved, we constructed a low copy plasmid carrying two copies of DsbC or FkpA behind a constitutive promoter. Bacteria transformed with these plasmids had intracellular concentrations of 450 μM DsbC (10 mg/mL) or 550 μM FkpA (15 mg/mL), approximately three times the concentration of our assessed extracts. This falls in the range of chaperone proteins required for efficient IgG folding (). Therefore, strain 2xD + 2xF was engineered to produce high concentrations of both chaperones, thus allowing for the rapid production and folding of IgG without the addition of large amounts of exogenous protein. Traditional methods of intracellular IgG expression in bacterial cultures often result in cellular toxicity, especially if the cells are also generating high levels of chaperone to support IgG folding. However, in OCFS, the cell’s resources are first allocated for the production of high concentrations of chaperone protein during bacterial fermentation. Once the cells are harvested and used to generate cell extract, the cellular machinery, which now includes high chaperone concentrations, can be reallocated and fully dedicated to the production of one protein of interest – in our case, IgG. In this manner, we are able to achieve rapid production of extremely high antibody titers.
In this study, the versatility of open cell free protein synthesis was utilized to quickly screen a large variety of chaperones to determine which might boost production of native IgGs in the context of prokaryotic protein synthesis machinery. By expressing these factors in the extract-production strain, it was possible to achieve gram per liter titers in an overnight reaction. These titers are on par with high expressing stable cell lines, without the risk, time, and cost of developing the cell lines. This advancement pushes OCFS to the forefront of production technologies for IgGs.
Materials and Methods
Small-scale cell-free expression
100 μl cell-free reactions were run at 30 °C for 12 h in a 96-well microtiter plate at 650 rpm in a VWR Thermomixer in the presence of 10 μg/mL DNA (2.5 µg/mL trastuzumab LC DNA, 7.5 µg/mL trastuzumab HC DNA in the expression vector pYD317Citation7,Citation8). To facilitate disulfide bond formation, cell-free extracts were treated with 50 µM iodoacetamide for 30 min at RT (20 °C) to quench reactive thiol groups and added to a premix of components. The final concentration in the protein synthesis reaction was 30% cell extract (v/v), 2 mM GSSG, 8 mM magnesium glutamate, 10 mM ammonium glutamate, 130 mM potassium glutamate, 35 mM sodium pyruvate, 1.2 mM AMP, 0.86 mM each of GMP, UMP, and CMP, 2 mM amino acids (except 1 mM for Tyrosine and Phenylalanine), 4 mM sodium oxalate, 1 mM putrescine, 1.5 mM spermidine, 15 mM potassium phosphate, and 20 ug/mL T7 RNAP, unless otherwise indicated. Extracts were generated from bacteria growing in log phase as previously described.Citation8
Chaperone sequential expression screen
Candidate chaperones were cloned into the cell-free expression plasmid pYD317. From these plasmids, PCR fragments were generated that contained the chaperone gene sandwiched between T7 promoter and terminator sequences. Chaperones were subsequently expressed from these PCR fragments by cell-free protein synthesis under standard microtiter plate conditions for 16 h at 30 °C. To stabilize the PCR fragments against DNA degradation, 40 ug/mL GamS protein was added to the reactions. Chaperone-expressing extract was subsequently centrifuged at 5000xg for 10 min and chaperone-containing supernatants were added into new cell-free reactions at 20% (v/v) for the expression of IgG (8 μg/mL trastuzumab HC DNA and 2 μg/mL trastuzumab LC DNA) in the presence of 14C-Leucine. Because DNA sequences can translate at different rates due to differential codon usage and mRNA structure, the HC:LC DNA ratio was empirically optimized to 8:2 μg/mL for the highest IgG titers. IgG titers were calculated based on the incorporation of 14C-Leucine into the IgG molecule, as previously described.Citation7 Briefly, synthesized proteins were quantified by 14C counts that could be precipitated with trichloroacetic acid. Assembled IgG was further calculated as the proportion of assembled IgG in a non-reducing gel. Chaperone-related improvements in IgG titer were expressed as a fold improvement over the addition of a GFP-expressing extract. To estimate the amount of chaperone being added to the IgG expression reactions, chaperone cell-free reactions were run in a parallel reaction in the presence of 14C-Leucine and the expressed protein was quantified.
Interchangeability of PDI and DsbC
Cell-free protein synthesis reactions were run at varying concentrations of PDI and DsbC to understand the requirements for disulfide bond isomerases on IgG folding and assembly. 0–5 μM recombinant PDI was added to cell-free reactions in combination with 0–13 μM recombinant DsbC. 100 μl cell-free reactions were run with 30% control extract for 12 h at 30 °C in a 96-well microtiter plate at 650 rpm in a VWR Thermomixer in the presence of 8 μg/mL HC-His6 DNA and 2 μg/mL LC DNA. The reactions were subsequently centrifuged at 5000xg for 10 min and supernatants were diluted 2-fold with PBS prior to purification on IMAC Phytips (200 μl tips, 5 μl resin bed) using a Biomek robotic system. Samples were eluted in imidazole elution buffer (20 mM Tris pH8, 300 mM NaCl, 500 mM imidazole) and the eluted IgG was quantified using capillary electrophoresis on a Caliper LapChip GXII according to the manufacturer’s instructions. PDI protein was purchased from MC Labs (MC Lab, South San Francisco, CA; recombinant yeast Protein Disulfide Isomerase) and bacterial DsbC was purified as described.Citation8
Chaperone expression and purification
Skp-His6, SlyD, and FkpA-His6 were expressed in SBJY001 cell extracts under standard expression conditions and purified via small-scale Ni2+ NTA purification (note: SlyD construct was not His6-tagged but the SlyD sequence contains an internal His-rich sequence). Purified chaperones were eluted in imidazole elution buffer and dialyzed into 10 mM TRIS-acetate pH 8.2, 60 mM potassium acetate.
2xDsbC and 2xFkpA extracts
The E. coli strain SBJY001Citation7 was transformed with pACYC-based chaperone overexpression plasmids and harvested in log phase to make cellular extracts. Plasmids carrying one copy (1xDsbC) or two tandem copies (2xDsbC) of dsbC behind the E. coli promoter Mt-cons-10 24 were generated and transformed into bacteria, as were one copy (1xFkpA) or two copies (2xFkpA) of fkpA. These strains were grown to log phase and lysed for the production of cell-free extract, as described.Citation8 The IgG-producing activities of each of these extracts were tested, either alone or in combination with exogenously added purified protein. A bacterial strain SBHS016 (derived from bacterial strain SBJY001) optimized for OCFS extracts was further modified to enhance the production of DsbC protein. This strain has dual tandem copies of dsbC integrated into the bacterial galK locus, constitutively expressed using a modified MT-cons-10 promoter.Citation24 This is in addition to the wild type gene at the normal dsbC locus. The dual tandem gene cassette contains one copy of the parental dsbC gene, and one copy of a synthetic version of the dsbC gene designed to encode the wild type protein, but with altered codons to suppress unwanted sequence recombination with other versions of dsbC gene elsewhere in the genome. This DsbC overexpressing strain was transformed with the 2xFkpA plasmid to produce strain ‘2xD + 2xF’.
Abbreviations: | ||
Open Cell Free Synthesis | = | OCFS |
heavy chain | = | HC |
light chain | = | LC |
immunoglobulin | = | IgG |
peptidyl prolyl isomerase | = | PPIase |
protein disulfide isomerase | = | PDI |
endoplasmic reticulum | = | ER |
binding immunoglobulin protein | = | BiP |
heat shock protein 70 | = | hsp70 |
single chain Fragment variable | = | scFv |
fragment antigen-binding | = | Fab |
Fragment constant | = | Fc |
Additional material
Download Zip (718.7 KB)Disclosure of Potential Conflicts of Interest
No potential conflicts of interest were disclosed.
Acknowledgments
The authors would like to acknowledge Steve Zolnay for helpful discussions and Melissa Frenchmeyer for technical assistance.
References
- van Anken E, Romijn EP, Maggioni C, Mezghrani A, Sitia R, Braakman I, Heck AJR. Sequential waves of functionally related proteins are expressed when B cells prepare for antibody secretion. Immunity 2003; 18:243 - 53; http://dx.doi.org/10.1016/S1074-7613(03)00024-4; PMID: 12594951
- Feige MJ, Hendershot LM, Buchner J. How antibodies fold. Trends Biochem Sci 2010; 35:189 - 98; http://dx.doi.org/10.1016/j.tibs.2009.11.005; PMID: 20022755
- Sevier CS, Kaiser CA. Formation and transfer of disulphide bonds in living cells. Nat Rev Mol Cell Biol 2002; 3:836 - 47; http://dx.doi.org/10.1038/nrm954; PMID: 12415301
- Feige MJ, Groscurth S, Marcinowski M, Shimizu Y, Kessler H, Hendershot LM, Buchner J. An unfolded CH1 domain controls the assembly and secretion of IgG antibodies. Mol Cell 2009; 34:569 - 79; http://dx.doi.org/10.1016/j.molcel.2009.04.028; PMID: 19524537
- Mayer M, Kies U, Kammermeier R, Buchner J. BiP and PDI cooperate in the oxidative folding of antibodies in vitro. J Biol Chem 2000; 275:29421 - 5; http://dx.doi.org/10.1074/jbc.M002655200; PMID: 10893409
- Wiest DL, Burkhardt JK, Hester S, Hortsch M, Meyer DI, Argon Y. Membrane biogenesis during B cell differentiation: most endoplasmic reticulum proteins are expressed coordinately. J Cell Biol 1990; 110:1501 - 11; http://dx.doi.org/10.1083/jcb.110.5.1501; PMID: 2335560
- Yin G, Garces ED, Yang J, Zhang J, Tran C, Steiner AR, Roos C, Bajad S, Hudak S, Penta K, et al. Aglycosylated antibodies and antibody fragments produced in a scalable in vitro transcription-translation system. MAbs 2012; 4:4; http://dx.doi.org/10.4161/mabs.4.2.19202; PMID: 22377750
- Zawada JF, Yin G, Steiner AR, Yang J, Naresh A, Roy SM, Gold DS, Heinsohn HG, Murray CJ. Microscale to manufacturing scale-up of cell-free cytokine production--a new approach for shortening protein production development timelines. Biotechnol Bioeng 2011; 108:1570 - 8; http://dx.doi.org/10.1002/bit.23103; PMID: 21337337
- Research) C (bcc. BCC Research: Global market for therapeutic monoclonal antibodies to grow to $58 billion in 2016 [Internet]. BCC Res.2012 [cited 2013 Nov 1]; Available from: http://bccresearch.blogspot.com/2012/03/global-market-for-therapeutic.html
- Frey S, Haslbeck M, Hainzl O, Buchner J. Synthesis and characterization of a functional intact IgG in a prokaryotic cell-free expression system. Biol Chem 2008; 389:37 - 45; http://dx.doi.org/10.1515/BC.2008.007; PMID: 18095868
- Makino T, Skretas G, Kang T-H, Georgiou G. Comprehensive engineering of Escherichia coli for enhanced expression of IgG antibodies. Metab Eng 2011; 13:241 - 51; http://dx.doi.org/10.1016/j.ymben.2010.11.002; PMID: 21130896
- Sitaraman K, Esposito D, Klarmann G, Le Grice SF, Hartley JL, Chatterjee DK. A novel cell-free protein synthesis system. J Biotechnol 2004; 110:257 - 63; http://dx.doi.org/10.1016/j.jbiotec.2004.02.014; PMID: 15163516
- Levy R, Weiss R, Chen G, Iverson BL, Georgiou G. Production of correctly folded Fab antibody fragment in the cytoplasm of Escherichia coli trxB gor mutants via the coexpression of molecular chaperones. Protein Expr Purif 2001; 23:338 - 47; http://dx.doi.org/10.1006/prep.2001.1520; PMID: 11676610
- Bothmann H, Pluckthun A. The periplasmic Escherichia coli peptidylprolyl cis,trans-isomerase FkpA. I. Increased functional expression of antibody fragments with and without cis-prolines. J Biol Chem 2000; 275:17100 - 5; http://dx.doi.org/10.1074/jbc.M910233199; PMID: 10748200
- Yin G, Swartz JR. Enhancing multiple disulfide bonded protein folding in a cell-free system. Biotechnol Bioeng 2004; 86:188 - 95; http://dx.doi.org/10.1002/bit.10827; PMID: 15052638
- Shimizu Y, Hendershot LM. Organization of the functions and components of the endoplasmic reticulum. Adv Exp Med Biol 2007; 594:37 - 46; http://dx.doi.org/10.1007/978-0-387-39975-1_4; PMID: 17205673
- Blum P, Ory J, Bauernfeind J, Krska J. Physiological consequences of DnaK and DnaJ overproduction in Escherichia coli. J Bacteriol 1992; 174:7436 - 44; PMID: 1429465
- Zimmerman SB, Trach SO. Estimation of macromolecule concentrations and excluded volume effects for the cytoplasm of Escherichia coli. J Mol Biol 1991; 222:599 - 620; http://dx.doi.org/10.1016/0022-2836(91)90499-V; PMID: 1748995
- Jakob RP, Zoldák G, Aumüller T, Schmid FX. Chaperone domains convert prolyl isomerases into generic catalysts of protein folding. Proc Natl Acad Sci U S A 2009; 106:20282 - 7; http://dx.doi.org/10.1073/pnas.0909544106; PMID: 19920179
- Ow DS-W, Lim DY-X, Nissom PM, Camattari A, Wong VV-T. Co-expression of Skp and FkpA chaperones improves cell viability and alters the global expression of stress response genes during scFvD1.3 production. Microb Cell Fact 2010; 9:22; http://dx.doi.org/10.1186/1475-2859-9-22; PMID: 20388215
- Ramm K, Plückthun A. High enzymatic activity and chaperone function are mechanistically related features of the dimeric E. coli peptidyl-prolyl-isomerase FkpA. J Mol Biol 2001; 310:485 - 98; http://dx.doi.org/10.1006/jmbi.2001.4747; PMID: 11428902
- Levy R, Ahluwalia K, Bohmann DJ, Giang HM, Schwimmer LJ, Issafras H, Reddy NB, Chan C, Horwitz AH, Takeuchi T. Enhancement of antibody fragment secretion into the Escherichia coli periplasm by co-expression with the peptidyl prolyl isomerase, FkpA, in the cytoplasm. J Immunol Methods 2013; 394:10 - 21; http://dx.doi.org/10.1016/j.jim.2013.04.010; PMID: 23624043
- Nagradova NK. Foldases: Enzymes Catalyzing Protein Folding. Nova Publishers; 2008.
- Thouvenot B, Charpentier B, Branlant C. The strong efficiency of the Escherichia coli gapA P1 promoter depends on a complex combination of functional determinants. Biochem J 2004; 383:371 - 82; http://dx.doi.org/10.1042/BJ20040792; PMID: 15250823