Abstract
Currently available rapid diagnostic tests (RDTs) for malaria show large variation in sensitivity and specificity, and there are concerns about their stability under field conditions. To improve current RDTs, monoclonal antibodies (mAbs) for novel malaria antigens have been developed and screened for their possible use in new diagnostic tests. Three antigens, glutamate rich protein (GLURP), dihydrofolate reductase-thymidylate synthase (DHFR-TS) and heme detoxification protein (HDP), were selected based on literature searches. Recombinant antigens were produced and used to immunize mice. Antibody-producing cell lines were subsequently selected and the resulting antibodies were screened for specificity against Plasmodium falciparum and Plasmodium vivax. The most optimal antibody couples were selected based on antibody affinity (expressed as dissociation constants, KD) and detection limit of crude antigen extract from P. falciparum 3D7 culture. The highest affinity antibodies have KD values of 0.10 nM ± 0.014 (D5) and 0.068 ± 0.015 nM (D6) for DHFR-TS mAbs, 0.10 ± 0.022 nM (H16) and 0.21 ± 0.022 nM (H18) for HDP mAbs and 0.11 ± 0.028 nM (G23) and 0.33 ± 0.093 nM (G22) for GLURP mAbs. The newly developed antibodies performed at least as well as commercially available histidine rich protein antibodies (KD of 0.16 ± 0.13 nM for PTL3 and 1.0 ± 0.049 nM for C1–13), making them promising reagents for further test development.
Introduction
Accurate early diagnosis and subsequent treatment are essential to reduce morbidity and mortality due to malaria. Diagnosis of malaria based on clinical signs and symptoms may lead to over-diagnosis even in highly endemic areas, as the clinical presentations of the disease resemble those of a number of other common illnesses, such as pneumonia, equally associated with morbidity and mortality.Citation1–Citation3 Introduction of effective but expensive, artemisinin-based combination therapies, due to increasing parasite resistance to older drugs such as chloroquine and sulfadoxine-pyrimethamine, increase the urgency for cheap and accurate diagnosis.Citation4
Microscopic examination of blood slides is, in principle, sensitive and specific for the parasitological detection of malaria, if used correctly, and has been used for over a hundred years. Unfortunately, it is not always widely available in many highly endemic areas or it is poorly executed, and for that reason an easy-to-use alternative is needed in situations where good quality microscopy is unavailable.Citation5
Over a decade ago, rapid diagnostic tests (RDTs) for malaria were introduced that have the advantages of being quick and can be easily performed in remote settings.Citation6,Citation7 These lateral flow immuno-chromatographic tests are based on the detection of malaria parasite antigens in patient blood by specific monoclonal antibodies (mAbs) that bind the antigen and immobilize and mark it with a label on nitrocellulose. Commercially available RDTs for malaria all detect one or more of the following antigens: histidine rich protein 2 (HRP2), lactate dehydrogenase (pLDH) or aldolase. Tests detecting HRP2 are most commonly used because of their lower cost, better stability and lower detection threshold compared with pLDH-based tests.Citation8–Citation11 Moreover, HRP2-based RDTs have better sensitivity than pLDH-based tests, although specificity is better for pLDH-based tests.Citation12 HRP2-based tests, however, only detect Plasmodium falciparum and the genetic diversity within the HRP2 antigen affects RDT sensitivity.Citation13,Citation14 Sensitivities for aldolase-detecting RDTs have often been reported to be poor, especially for non-falciparum parasites.Citation15–Citation19 It is therefore not commonly used as a stand-alone test. Concerns about test stability, performance (in terms of sensitivity and specificity) and antigen genetic diversity prompt the need for improvement of test performance characteristics, and therefore new antigens should be targeted by RDTs.Citation5,Citation9,Citation10,Citation13,Citation20
In this study, novel antigens for detection by RDTs were indentified through literature search. These antigen targets were chosen as suitable antigen targets according to the following three criteria. The first criterion is that the antigen should be unique for Plasmodia in some way, such as sequence or structure. Further, expression throughout the different life stages of the parasite is important, as well as having a conserved gene or regions within the gene, since antigenic variation can cause false negatives.
Dihydrofolate reductase (DHFR) is an enzyme that functions in the folate pathway in Plasmodium species, where it catalyzes the reduction of dihydrofolate to tetrahydrofolate. It was chosen because, unlike bacteria and higher eukaryotes, DHFR in protozoa exists as a bi-functional protein with thymidylate synthase (TS). Moreover, the plasmodial DHFR domain contains two specific insert regions.Citation21,Citation22 All Plasmodia contain these inserts, but the sequences vary slightly, making it potentially possible for the antibodies to differentiate between the species.Citation22
Heme detoxification protein (HDP) has been described as highly efficient in mediating the conversion of heme into hemozoin (malaria pigment).Citation23 HDP is encoded by a single copy gene containing 3 exons and is conserved within all (fully sequenced) Plasmodium species with a sequence identity of 60% and conserved within different P. falciparum isolates.Citation23,Citation24 HDP was chosen because of its unique function and although homologs have been found in other species, they only have a sequence identity of less than 15% with plasmodium HDP.Citation23 HDP is present in several life stages of Plasmodia and in the early ring stage it can be found in the cytosol of the infected red blood cell and in later stages it is trafficked to the food vacuole in transport vesicles together with heme.Citation23
Glutamate Rich Protein (GLURP) was chosen because it is a highly antigenic exoantigen of P. falciparum that is expressed in all parasite life stages in the host, and its gene is conserved in geographically different isolates.Citation25 The protein includes two repeat regions, R1 and R2, of which the R2 repeat sequence is well conserved between different isolates.Citation25 Additionally, several major B-cell epitopes in the R0 (non-repetitive) region of GLURP have been identified.Citation26 This target is only suitable for P. falciparum diagnosis, because the gene is not present in the other species, but it might be used in combination with other antibodies to make a diagnostic test against multiple species.
mAbs were raised against the P. falciparum specific antigen glutamate rich protein (GLURP) and the PAN-plasmodial antigens dihydrofolate reductase-thymidylate synthase (DHFR-TS) and heme detoxification protein (HDP). These antibodies were screened for specificity against P. falciparum and Plasmodium vivax. Subsequently, affinity and detection limit were determined and compared with commercially available HRP2 antibodies.
Results
Generation and screening of mAbs.
Antibody response to the antigens in mouse serum was measured during the immunization process. High titers were observed for all antigens and 20 × 106 to 390 × 106 cells/ml could be harvested from the spleens (). Half of the cells from one mouse for each antigen with the highest titer or cell count were fused with myeloma cells, except for GLURP R2, where the cells might have been contaminated during harvest of the mouse with the highest titer. After selection of hybridoma cells on selective medium with aminopterin, on average 45% of the wells contained growing clones. These cells were screened for specific antibody production against P. falciparum NF54 and recombinant antigen in ELISA. Depending on the type of antigen, 10–25% of the growing hybridomas (5–11% of the wells) were highly reactive with both NF54 and the corresponding recombinant antigen (). For each antigen the most reactive antibodies were selected and these cell lines were further sub-cloned to derive monoclonal hybridoma cell lines for further characterization. Isotyping of selected clones was performed and, for the DHFR-TS antibodies, mostly IgM antibodies were selected, but for GLURP and HDP a larger proportion of mAbs were IgG ().
Dissociation constant of antibodies.
Dissociation constants were estimated by ELISA and are listed in for selected DHFR-TS, HDP and GLURP antibodies. The DHFR-TS antibodies with the highest affinities are D5 and D6, for HDP H16 and H18 and for GLURP are G22 and G23 (). The dissociation constants of the commercially obtained HRP2 antibodies were not found in literature, but were measured in the present study as 0.16 ± 0.13 nM for PTL3 and 1.0 ± 0.049 nM for C1–13 in the ELISA. This is in the same order of magnitude as the antisera against synthetic peptides of PfHRP2 described by Tomar et al. Many of the developed antibodies for DHFR-TS, HDP and GLURP have a similar or higher affinity for their antigen than the HRP2 antibodies.
Crude parasite antigen detection.
The ELISA with crude parasite antigen allowed the detection of native DHFR, HDP and GLURP antigens from culture at different concentrations by selected mAbs. The lower detection limit of several developed antibodies was comparable to the detection limit of two HRP2 antibodies (). The detection of antibody, expressed as absorbance at 450 nm, was linearly correlated with the antigen concentration for all described mAbs (r ≥ 0.93 and p < 0.05) (). The strongest antibodies, D6, D15, H12 and H16 are very comparable in absorbance pattern to the HRP2 antibodies ().
Detection of P. vivax.
Although the antibodies were raised against P. falciparum recombinant antigens, five HDP (H2, H7, H10, H13 and H16) and four DHFR-TS (D7, D15, D16 and D28) of the selected antibodies were able to detect pooled P. vivax patient samples in ELISA, besides P. falciparum. Positive absorbance at 450 nm in the ELISA ranged from 0.31–1.06 compared with 0.24 ± 0.03 of background absorption (no-antibody negative controls) and 0.22 ± 0.02 of GLURP (Pf specific) negative controls.
Discussion
Concerns regarding the diagnostic accuracy and test stability of malaria RDTs have prompted development of novel antibodies that could be incorporated in new diagnostic tests. In the present study, novel mAbs were developed and characterized against the newly selected Plasmodium antigen targets dihydrofolate reductase-thymidylate synthase, heme detoxification protein and glutamate rich protein.
The present work generated several mAb-producing hybridoma cell lines, and specific clones were selected on the basis of their reactivity with recombinant antigen and malaria cell culture material. Affinities of the developed antibodies for their recombinant antigens are higher than, or comparable to, the two tested commercially available HRP2 antibodies. High affinity is important for sensitivity and the detection limit of diagnostic assays, suggesting that the newly developed antibodies are good candidates for diagnostic test development. The antibodies can detect the native antigens from NF54 and 3D7 P. falciparum isolates in a concentration-dependent manner. Some of the antibodies were able to give similar detection limit and absorbance as the HRP2 antibodies, which is important for the sensitivity of a diagnostic test. Many antibodies had a lower absorbance than the HRP2 antibodies, but nevertheless many of them did have a similar detection limit. Higher absorbance does not necessarily mean a better antibody, but might also reflect the abundance of the antigen in the crude parasite extract. HRP2 is a very stable antigen and known to persist, and may therefore be more abundant in the extract, which seems like a plausible explanation, because the antibody affinities are very similar. The abundance of the antigen in clinical samples is important for the detection limit of a diagnostic assay, and should be kept into consideration for future test development. Some of the DHFR-TS and HDP antibodies were able to detect the homologous antigens in P. vivax, an important feature for malaria diagnostic tests, since the available RDTs poorly detect this species (pLDH and aldolase), or not at all (HRP2).Citation9,Citation10
Mutations in the DHFR domain of Plasmodia are involved in anti-malarial resistance, and the presence of these mutations might influence antibody binding. One of these mutations, Ser108, is a residue that is present in one of the recombinant antigens used in the present study, which is the penultimate amino acid at the C-terminal side of DHFR-TS insert 1 and 2. Whether the developed antibodies against DHFR-TS are affected by this mutation should be further determined by screening several genotypically different P. falciparum strains.
In further test development, the antibodies should be combined to select good couples of capture- and detection-antibodies, and the detection limit of different P. falciparum culture isolates and patient samples should be evaluated next. The sandwich assay then needs to be transformed to a rapid test format for field application. Since stability of the reagents is a critical issue for the RDTs, the stability of the antibodies at ambient temperatures needs to be investigated to choose the most stable antibodies. Further research is needed if the DHFR-TS and HDP antibodies can also detect Plasmodium malariae and Plasmodium ovale parasites. The mAbs presented in this study show much potential for further development in diagnostic assays.
Material and Methods
In vitro culture of P. falciparum.
Plasmodium falciparum strains NF54 (UMC St. Radboud, Nijmegen, The Netherlands) and 3D7 (MRA-102, MR4, ATCC® Manassas Virginia) parasites were cultured in a candle jar on RPMI 1640 medium with L-Glutamine and 25 mM HEPES (Gibco, Invitrogen) with human A+ serum and O+ red blood cells according to the candle-jar technique of Trager-Jensen.Citation28,Citation29 The parasitemia was determined with Fields-stained microscopy slides and counted as percentage of infected red blood cells. The parasite strain used in the experiments depends on the isolate that was in culture because the department changed the parasite strain to be used as a standard from NF54 to 3D7. NF54 is the parental isolate of clone 3D7; therefore no difference is expected between the clones concerning these antigens. The recombinant antigens were amplified from NF54 parasites (see section below) and the vectors were sequenced and compared with the published 3D7 genes in PlasmoDB; the sequences were identical (data not shown).Citation30
Preparation of P. falciparum lysate.
Parasite culture (∼2–5%) was diluted 10 times in PBS and sonicated vigorously for 30 sec. This suspension was used in the ELISA procedures described below.
P. vivax samples.
Frozen samples from patients infected with P. vivax parasites were provided by the Instituto de Medicina Tropical “Alexander von Humboldt“—Universidad Peruana Cayetano de Heredia in Peru. Samples were characterized with microscopy. A pool of samples was used with a resulting parasitemia of ∼0.1% and used in the screening ELISA described below.
Production of recombinant antigens.
The recombinant HDP protein was provided for the purpose of this study from the Virginia Bioinformatics Institute, Virginia Tech.Citation23 Primers were developed to amplify gene fragments of the DHFR-TS and GLURP antigens and sequences are listed in . Genetic sequences were derived from PlasmoDB, with the following accession numbers: DHFR-TS: PFD0830w and GLURP: PF10_0344.Citation30 Amplification was performed with Accuprime Taq High Fidelity (Invitrogen) and the supplied PCR buffer II with the addition of 0.05 mM dNTPs and 0.2 µM each of forward and reverse primer. Amplification was performed on a Peltier Thermal cycler 100 with initial hot start of 1 min at 94°C and subsequently 40 cycles of 30 sec at 94°C, 30 sec at 58°C (DHFR1 and 2, TS, GLURP R0) or 60°C (GLURP R2) and 1.10 min at 68°C, and a final elongation step of 10 min at 68°C. The PCR amplicons were precipitated with ethanol and mixed with pTXB1 vector (IMPACT KIT, New England Biolabs) and digested with BmtI (DHFR1&2 and TS) or NdeI (GLURP R0 and GLURP R2) and SapI. All restriction enzymes were from New England Biolabs and used as described by the manufacturer. The digested vector and amplicons were ligated with T4 DNA ligase (Roche) and transformed to T7 Express Competent E. coli High Efficiency (New England Biolabs) with heat shock at 42°C for 10 sec. Recombinant antigens were expressed and purified with Chitine beads as described in the IMPACT KIT (New England Biolabs) and confirmed on SDS-PAGE gel. This work was performed in a controlled environment under GMO license nr 02–080 of the Dutch ministry of Infrastructure and Environment.
Immunization protocol and hybridoma culture.
Ten to twelve week old specific pathogen free Balb/c mice (two for each antigen) were immunized twice (with a 28-d interval) with 100 µg recombinant antigen in PBS with 50% Stimune adjuvant (Prionics) intra peritoneal (i.p.) and subcutaneous (s.c.) and with a final booster after 11 d with 100 µg recombinant antigen in PBS intravenous (i.v.). All animal experiments were approved by the local animal ethics committee. Antibody response in mice serum was determined with ELISA as described below. Splenocytes were harvested and frozen according to the protocol from Marusich et al. The cells of half a spleen were fused to myeloma cells according to a standardized protocol.Citation32 Cell lines were selected based on ELISA results described below, and subsequently sub-cloned to obtain single clones. Hybridoma cells were grown in Optimem I with Glutamax (Invitrogen) with 10% FCS (Fetalclone I, Hyclone) or Hybridoma Serum Free medium (Invitrogen).
Screening ELISA.
Mice serum and supernatant of hybridoma cell lines were screened with ELISA for specific reactivity with recombinant antigen and NF54 and/or 3D7 culture lysate. ELISA plates (medium binding, Greiner) were coated over night at 4°C with recombinant antigen (20 µg/ml) in PBS or P. falciparum lysate or a P. vivax patient sample (5,630 parasites/µl diluted 1:5 in PBS) and subsequently washed with PBS + 0.1% Tween 20 (PBST) and blocked with 5% BSA (Sigma) in PBS for 1 h at 37°C. Plates were washed and incubated 1 h at 37°C with 50 µl of supernatant of the hybridoma clones or mouse serum diluted in 1% BSA in PBST (1:3 × 103–1:10 × 106). After washing, plates were incubated 1 h at 37°C with peroxidase conjugated goat-anti-mouse (Jackson Immunoresearch) 1:5,000 diluted in 1% BSA in PBST. The presence of antibody was measured by adding 0.04% 3,3′,5,5′-Tetramethylbenzidine (Sigma), 0.04% urea peroxide (Sigma) in 0.1 M Sodium acetate citrate pH 4 and the reaction was stopped with 0.5 M Sulfuric acid. Color development was measured at 450 nm in a SUNRISE ELISA reader (Tecan).
Purification of mAbs.
Antibodies were isotyped (Pierce Rapid ELISA Mouse Antibody Isotyping Kit, Thermo Scientific) and HDP and DHFR-TS antibodies were purified directly from hybridoma supernatant (filtered through 0.2 µm) with 1 ml HiTrap protein GHP columns (GE Healthcare) in case of IgG and with 1 ml HiTrap IgM purification HP columns (GE Healthcare) in case of IgM, according to manufacturer's instructions. Purifications were performed on an ÄKTA FPLC system (Amersham Biosciences). Antibody containing fractions were pooled and concentrated with 50 kD (IgG) or 100 kD (IgM) Amicon® Ultra-4 Centrifugal Filter Units (Millipore) until a concentration of approximately 1 mg/ml was reached. Antibody concentration was determined by adsorption at 280 nm with a Nanodrop 1000 (Thermo Scientific).
The GLURP antibodies used in the experiments were precipitated with ammonium sulfate. Hybridoma supernatant was clarified by a 0.2 µm syringe filter (Millipore) and saturated ammonium sulfate pH 7.4 was slowly added till the final concentration of ammonium sulfate was 45% and left on a rotator for 45 min. Subsequently, the mixture was incubated O/N at 4°C and then centrifuged at 3,893 g for 40 min. The pellet was resuspended in 8 ml cold PBS and concentrated with 50 kD Amicon® Ultra-4 Centrifugal Filter Units (Millipore) until a concentration of approximately 1 mg/ml was reached.
Measurement of true affinity (KD) by ELISA.
The binding affinities of the antibodies were determined by measuring the dissociation constant (KD).Citation33 Briefly, antigen at various concentrations ranging from 1 × 10−7 M to 5 × 10−11 M was mixed with a constant amount of antibody in 0.1 M potassium phosphate, 2 mM EDTA, 10 mg/ml BSA, pH 7.8 and incubated for 15 h at room temperature. The antigen-antibody mix was then transferred to micro-titer plates previously coated with capture antigens at 150 ng/well in 50 mM sodium carbonate pH 9.6 (DHFR1&2, DHFR-TS, GLURP R0, GLURP R2 or HDP) and incubated for 60 min at room temperature. Washing of plates and detection of the presence of antibody was performed as described for the screening ELISA. Dissociation constants were calculated using regression analysis and the Scatchard-Klotz equation.Citation33 In comparison, two HRPII antibodies (C1–13 and PTL3, National Bioproducts Institute) were tested as well with recombinant HRP2 (ReaMatrix).
Crude parasite antigen ELISA.
A crude parasite antigen extract was prepared from a late stage 3D7 culture (∼3–4% schizonts) with Percoll as described by Troye-Blomberg et al.Citation29,Citation34 This antigen extract was coated overnight on ELISA plates in a dilution series from 20–0.03 µg/ml in 1% BSA in PBST. 1% BSA in PBST was used as negative control and the wells were subsequently blocked with 5% BSA in PBS for 30 min to 1 h at 37°C. After washing 3 times with PBST, purified antibodies (5 µg/ml) were added to each dilution series. In comparison two HRP2 antibodies (C1–13 and PTL3, National Bioproducts Institute) were taken along on each ELISA plate in the same concentration.
Statistical analysis.
The dissociation constant (KD) was determined by calculating the slope of the lines by regression analysis.Citation33 The correlation between absorbance in the ELISA and the antigen concentration was determined by Pearson product moment correlation coefficient (PMCC).
Abbreviations
RDTs | = | rapid diagnostic tests |
GLURP | = | glutamate rich protein |
DHFR-TS | = | dihydrofolate reductase-thymidylate synthase |
HDP | = | heme detoxification protein |
KD | = | dissociation constant |
HRP2 | = | histidine rich protein |
pLDH | = | parasite lactate dehydrogenase |
Figures and Tables
Figure 1 Detection of native antigens in a crude parasite extract dilution from P. falciparum culture with selected monoclonal antibodies. (A) HRP2 antibodies (C1–13 and PTL3, triangles) compared with strongest HDP (marked with H, solid lines) and DHFR-TS (marked with D, open squares) antibodies, (B) DHFR-TS antibodies, (C) HDP antibodies and (D) GLURP antibodies.
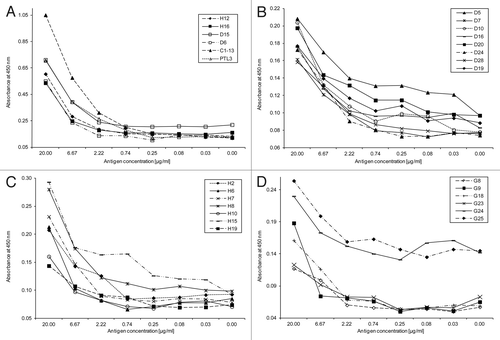
Table 1 Antibody response of mouse sera to the recombinant antigen in ELISA at different days before each (booster) immunization during the immunization procedure
Table 2 Overview and characteristics of hybridoma clones during immunization and selection process
Table 3 Dissociation constants (KD) of monoclonal antibodies as determined by ELISA
Table 4 Detection limit of crude parasite antigen in ELISA by selected antibodies compared with HRP2 antibodies
Table 5 Primer sequences for recombinant antigens and encoding nucleotide range
Acknowledgments
This project is part of a collaborative effort for the improvement of malaria diagnosis with the Foundation for Innovative New Diagnostics (FIND). Support for this project was provided through funding from FIND. HRP2 recombinant protein was provided by ReaMetrix India Pvt., Ltd.; anti-HRP2 antibodies by National Bioproducts Institute in South Africa; and the purified recombinant heme-detoxification protein (HDP) by Virginia Polytechnic Institute and State University (Virginia Tech.,) in the USA. We thank Dionicia Gamboa from the Instituto de Medicina Tropical “Alexander von Humboldt”—Universidad Peruana Cayetano de Heredia in Peru for sending samples from patients infected with P. vivax parasites. Those samples were collected in the frame of collaborative projects with FIND with the required approval of a local ethical committee. We thank MR4 for providing us with 3D7 malaria parasites contributed by Daniel Carucci and Alister Craig. We thank the Centre for clinical malaria studies of the University Medical Centre St. Radboud in Nijmegen for providing us with the NF54 malaria parasites.
References
- Amexo M, Tolhurst R, Barnish G, Bates I. Malaria misdiagnosis: effects on the poor and vulnerable. Lancet 2004; 364:1896 - 1898; PMID: 15555670; http://dx.doi.org/10.1016/S0140-6736(04)17446-1
- O'Dempsey TJ, McArdle TF, Laurence BE, Lamont AC, Todd JE, Greenwood BM. Overlap in the clinical features of pneumonia and malaria in African children. Trans R Soc Trop Med Hyg 1993; 87:662 - 665; PMID: 8296367; http://dx.doi.org/10.1016/0035-9203(93)90279-Y
- Källander K, Nsungwa-Sabiiti J, Peterson S. Symptom overlap for malaria and pneumonia—policy implications for home management strategies. Acta Trop 2004; 90:211 - 214; PMID: 15177148; http://dx.doi.org/10.1016/j.actatropica.2003.11.013
- Zikusooka CM, McIntyre D, Barnes KI. Should countries implementing an artemisinin-based combination malaria treatment policy also introduce rapid diagnostic tests?. Malar J 2008; 7:176; PMID: 18793410; http://dx.doi.org/10.1186/1475-2875-7-176
- Bell D, Wongsrichanalai C, Barnwell JW. Ensuring quality and access for malaria diagnosis: how can it be achieved?. Nat Rev Microbiol 2006; 4:682 - 695; PMID: 16912713; http://dx.doi.org/10.1038/nrmicro1474
- Shiff CJ, Premji Z, Minjas JN. The rapid manual ParaSight-F test. A new diagnostic tool for Plasmodium falciparum infection. Trans R Soc Trop Med Hyg 1993; 87:646 - 648; PMID: 8296363; http://dx.doi.org/10.1016/0035-9203(93)90273-S
- Piper R, Lebras J, Wentworth L, Hunt-Cooke A, Houzé S, Chiodini P, et al. Immunocapture diagnostic assays for malaria using Plasmodium lactate dehydrogenase (pLDH). Am J Trop Med Hyg 1999; 60:109 - 118; PMID: 9988333
- Chiodini PL, Bowers K, Jorgensen P, Barnwell JW, Grady KK, Luchavez J, et al. The heat stability of Plasmodium lactate dehydrogenase-based and histidine-rich protein 2-based malaria rapid diagnostic tests. Trans R Soc Trop Med Hyg 2007; 101:331 - 337; PMID: 17212967; http://dx.doi.org/10.1016/j.trstmh.2006.09.007
- WHO. Malaria Rapid Diagnostic Test Performance—Results of WHO product testing of malaria RDTs: Round 1 2008; http://www.wpro.who.int/NR/rdonlyres/ED81BDE9-B812-4B80-8408-3A129A6365C4/0/OMSFINDRapportMalaria200900514v25.pdf
- WHO. Malaria Rapid Diagnostic Test Performance—Results of WHO product testing of malaria RDTs: Round 2 2009; http://www.wpro.who.int/internet/files/rdt/RDTMalariaRd2_FINAL.pdf
- Bell DR, Wilson DW, Martin LB. False-positive results of a Plasmodium falciparum histidine-rich protein 2-detecting malaria rapid diagnostic test due to high sensitivity in a community with fluctuating low parasite density. Am J Trop Med Hyg 2005; 73:199 - 203; PMID: 16014858
- Abba K, Deeks JJ, Olliaro P, Naing CM, Jackson SM, Takwoingi Y, et al. Rapid diagnostic tests for diagnosing uncomplicated P. falciparum malaria in endemic countries. Cochrane Database Syst Rev 2011; 7:8122; PMID: 21735422
- Baker J, McCarthy J, Gatton M, Kyle DE, Belizario V, Luchavez J, et al. Genetic diversity of Plasmodium falciparum histidine-rich protein 2 (PfHRP2) and its effect on the performance of PfHRP2-based rapid diagnostic tests. J Infect Dis 2005; 192:870 - 877; PMID: 16088837; http://dx.doi.org/10.1086/432010
- Lee N, Baker J, Andrews KT, Gatton ML, Bell D, Cheng Q, et al. Effect of sequence variation in Plasmodium falciparum histidine-rich protein 2 on binding of specific monoclonal antibodies: Implications for rapid diagnostic tests for malaria. J Clin Microbiol 2006; 44:2773 - 2778; PMID: 16891491; http://dx.doi.org/10.1128/JCM.02557-05
- Cho D, Kim KH, Park SC, Kim YK, Lee KN, Lim CS. Evaluation of rapid immunocapture assays for diagnosis of Plasmodium vivax in Korea. Parasitol Res 2001; 87:445 - 448; PMID: 11411942; http://dx.doi.org/10.1007/s004360000360
- Gatti S, Bernuzzi AM, Bisoffi Z, Raglio A, Gulletta M, Scaglia M. Lombardy Region's Study Group on International Health (SIRL). Multicentre study, in patients with imported malaria, on the sensitivity and specificity of a dipstick test (ICT Malaria P.f./P.v.) compared with expert microscopy. Ann Trop Med Parasitol 2002; 96:15 - 18; PMID: 11989528; http://dx.doi.org/10.1179/000349802125000457
- Huong NM, Davis TM, Hewitt S, Huong NV, Uyen TT, Nhan DH, et al. Comparison of three antigen detection methods for diagnosis and therapeutic monitoring of malaria: a field study from Southern Vietnam. Trop Med Int Health 2002; 7:304 - 308; PMID: 11952945; http://dx.doi.org/10.1046/j.1365-3156.2002.00869.x
- Mason DP, Kawamoto F, Lin K, Laoboonchai A, Wongsrichanalai C. A comparison of two rapid field immunochromatographic tests to expert microscopy in the diagnosis of malaria. Acta Trop 2002; 82:51 - 59; PMID: 11904103; http://dx.doi.org/10.1016/S0001-706X(02)00031-1
- Richter J, Göbels K, Müller-Stöver I, Hoppenheit B, Häussinger D. Co-reactivity of plasmodial histidine-rich protein 2 and aldolase on a combined immuno-chromographic-malaria dipstick (ICT) as a potential semi-quantitative marker of high Plasmodium falciparum parasitaemia. Parasitol Res 2004; 94:384 - 385; PMID: 15549388; http://dx.doi.org/10.1007/s00436-004-1213-6
- Perkins MD, Bell DR. Working without a blindfold: the critical role of diagnostics in malaria control. Malar J 2008; 7:5; PMID: 19091039; http://dx.doi.org/10.1186/1475-2875-7-S1-S5
- Ivanetich KM, Santi DV. Bifunctional thymidylate synthase-dihydrofolate reductase in protozoa. FASEB J 1990; 4:1591 - 1597; PMID: 2180768
- Yuvaniyama J, Chitnumsub P, Kamchonwongpaisan S, Vanichtanankul J, Sirawaraporn W, Taylor P, et al. Insights into antifolate resistance from malarial DHFR-TS structures. Nat Struct Biol 2003; 10:357 - 365; PMID: 12704428; http://dx.doi.org/10.1038/nsb921
- Jani D, Nagarkatti R, Beatty W, Angel R, Slebodnick C, Andersen J, et al. HDP-a novel heme detoxification protein from the malaria parasite. PLoS Pathog 2008; 4:1000053; PMID: 18437218; http://dx.doi.org/10.1371/journal.ppat.1000053
- Vinayak S, Rathore D, Kariuki S, Slutsker L, Shi YP, Villegas L, et al. Limited genetic variation in the Plasmodium falciparum heme detoxification protein (HDP). Infect Genet Evol 2009; 9:286 - 289; PMID: 19135554; http://dx.doi.org/10.1016/j.meegid.2008.12.004
- Borre MB, Dziegiel M, Høgh B, Petersen E, Rieneck K, Riley E. Primary structure and localization of a conserved immunogenic Plasmodium falciparum glutamate rich protein (GLURP) expressed in both the preerythrocytic and erythrocytic stages of the vertebrate life cycle. Mol Biochem Parasitol 1991; 49:119 - 131; PMID: 1775153; http://dx.doi.org/10.1016/0166-6851(91)90135-S
- Theisen M, Soe S, Jessing SG, Okkels LM, Danielsen S, Oeuvray C, et al. Identification of a major B-cell epitope of the Plasmodium falciparum glutamate-rich protein (GLURP), targeted by human antibodies mediating parasite killing. Vaccine 2000; 19:204 - 212; PMID: 10930674; http://dx.doi.org/10.1016/S0264-410X(00)00181-X
- Tomar D, Biswas S, Tripathi V, Rao DN. Development of diagnostic reagents: raising antibodies against synthetic peptides of PfHRP-2 and LDH using microsphere delivery. Immunobiology 2006; 211:797 - 805; PMID: 17113917; http://dx.doi.org/10.1016/j.imbio.2006.05.003
- Trager W, Jensen JB. Human malaria parasites in continuous culture. Science 1976; 193:673 - 675; PMID: 781840; http://dx.doi.org/10.1126/science.781840
- Moll K, Ljungström I, Perlmann H, Scherf A, Wahlgren A. Methods in malaria research 2008; 5
- Aurrecoechea C, Brestelli J, Brunk BP, Dommer J, Fischer S, Gajria B, et al. PlasmoDB: a functional genomic database for malaria parasites. Nucleic Acids Res 2009; 37:539 - 543; PMID: 18957442; http://dx.doi.org/10.1093/nar/gkn814
- Marusich MF. Efficient hybridoma production using previously frozen splenocytes. J Immunol Methods 1988; 114:155 - 159; PMID: 3183388; http://dx.doi.org/10.1016/0022-1759(88)90167-6
- Köhler G, Milstein C. Continuous cultures of fused cells secreting antibody of predefined specificity. Nature 1975; 256:495 - 497; PMID: 1172191; http://dx.doi.org/10.1038/256495a0
- Friguet B, Chaffotte AF, Djavadi-Ohaniance L, Goldberg ME. Measurements of the true affinity constant in solution of antigen-antibody complexes by enzyme-linked immunosorbent assay. J Immunol Methods 1985; 77:305 - 319; PMID: 3981007; http://dx.doi.org/10.1016/0022-1759(85)90044-4
- Troye-Blomberg M, Perlmann H, Patarroyo ME, Perlmann P. Regulation of the immune response in Plasmodium falciparum malaria. II. Antigen specific proliferative responses in vitro. Clin Exp Immunol 1983; 53:345 - 353; PMID: 6192953