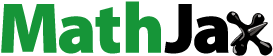
Abstract
The neonatal Fc receptor (FcRn) plays an important and well-known role in immunoglobulin G (IgG) catabolism; however, its role in the disposition of IgG after subcutaneous (SC) administration, including bioavailability, is relatively unknown. To examine the potential effect of FcRn on IgG SC bioavailability, we engineered three anti-amyloid β monoclonal antibody (mAb) reverse chimeric mouse IgG2a (mIgG2a) Fc variants (I253A.H435A, N434H and N434Y) with different binding affinities to mouse FcRn (mFcRn) and compared their SC bioavailability to that of the wild-type (WT) mAb in mice. Our results indicated that the SC bioavailability of mIgG2a was affected by mFcRn-binding affinity. Variant I253A.H435A, which did not bind to mFcRn at either pH 6.0 or pH 7.4, had the lowest bioavailability (41.8%). Variant N434Y, which had the greatest increase in binding affinity at both pH 6.0 and pH 7.4, had comparable bioavailability to the WT antibody (86.1% vs. 76.3%), whereas Variant N434H, which had modestly increased binding affinity at pH 6.0 to mFcRn and affinity comparable to the WT antibody at pH 7.4, had the highest bioavailability (94.7%). A semi-mechanism-based pharmacokinetic model, which described well the observed data with the WT antibody and variant I253A.H435A, is consistent with the hypothesis that the decreased bioavailability of variant I253A.H435A was due to loss of the FcRn-mediated protection from catabolism at the absorption site. Together, these data demonstrate that FcRn plays an important role in SC bioavailability of therapeutic IgG antibodies.
Introduction
The neonatal Fc receptor (FcRn) plays an important and well-known role in immunoglobulin G (IgG) catabolism.Citation1 It is expressed widely in endothelial, epithelial and hematopoietic cells including organs such as skin, muscle, kidney, liver and placenta.Citation1,Citation2 A unique feature of the interaction of IgG with FcRn is a strict pH dependency. FcRn binds to the Fc domain (CH2-CH3) of IgG in the acidic environment of the endosome (pH 6.0) and is recycled back into circulation where dissociation occurs at a neutral pH. IgG that is not bound to FcRn in the endosome undergoes transport to and proteolysis in the lysosomes.Citation1 The fact that half-life of an IgG in vivo can be influenced by altering its binding affinity to FcRn at different pH is well-established.Citation1 It has also been reported that several IgG Fc variants that bind stronger to FcRn at neutral pH have shorter in vivo half-lives compared with the corresponding wild-type (WT) IgG because they cannot dissociate from FcRn.Citation3,Citation4
There is now growing evidence that FcRn may also play an important role in IgG bioavailability after subcutaneous (SC) administration.Citation5,Citation6 For example, we reported that a human IgG1 Fc variant with stronger affinity to mouse FcRn (mFcRn) than WT antibody at pH 7.4 had slightly lower bioavailability in mice after SC administration (61.0 vs. 73.0%, respectively).Citation6 Overall, these results have raised questions regarding the role played by FcRn on IgG SC bioavailability.
The SC bioavailability of IgG is thought to be determined not only by the relative rate and extent of systemic absorption, but also the rate and extent of presystemic catabolism. Systemic absorption of antibodies is believed to be mediated mainly via lymphatic drainage due to its large molecular weight. Presystemic IgG catabolism, however, is influenced by many processes including extracellular degradation (e.g., via proteolysis), antibody endocytosis (e.g., receptor-mediated, fluid phase), as well as potential recycling through interaction with FcRn. A physiologically-based pharmacokinetic (PBPK) model, which incorporated the protective role of FcRn, predicted that the skin is one of the major organs responsible for IgG catabolism in mice, accounting for approximately 33% of the total IgG elimination.Citation7 This finding is also supported by evidence of FcRn expression on the endothelium of the large vascular beds of skin in miceCitation8 and on hematopoietic cells such as monocytes, macrophages and dendritic cells,Citation2,Citation9 which are also presented in the subcutaneous tissue. Furthermore, in FcRn deficient mice, the SC bioavailability of a mouse monoclonal antibody (mAb) IgG1 was reduced significantly (∼3-fold lower) compared with the WT mice.Citation5
To examine the effect of FcRn on IgG SC bioavailability in more detail, we engineered three anti-amyloid β (Aβ) reverse chimeric mouse IgG2a (mIgG2a) Fc variants (I253A.H435A (EU numbering), N434H and N434Y) with different binding affinities to mFcRn and compared their SC bioavailability to that of the WT antibody in mice. Fc residues Ile253, Asn434 and His435 are critical for IgG interaction with FcRn in mice.Citation10,Citation11 Given the importance of pH-dependent interactions to the efficient recycling of an IgG, an ideal IgG Fc variant should have increased FcRn affinity at acidic pH compared with a WT antibody, thus retaining binding while in the endosome, and having weaker or equivalent affinity at neutral pH to facilitate release back into systemic circulation.Citation3,Citation4,Citation6,Citation12 Variant I253A.H435A did not bind to mFcRn at either pH 6.0 or pH 7.4. Variant N434Y had the highest increased binding affinity to mFcRn at both pH 6.0 (∼76.7-fold) and pH 7.4. Variant N434H had modestly increased binding affinity at pH 6.0 (∼13.1-fold) to mFcRn with affinity comparable to the WT antibody at pH 7.4. Following a single intravenous (IV) or SC administration to mice, we observed that variant I253A.H435A had a lower SC bioavailability in mice compared with the WT antibody. In contrast, variant N434H, which had higher binding affinity to mFcRn at pH 6.0 and comparable binding affinity at pH 7.4, appeared to have comparatively higher SC bioavailability in mice. Of note, due to the lower endogenous level of Aβ in mice, the potential interaction of antigen-IgG interaction should not affect the pharmacokinetics of these mIgG2a Fc variants including bioavailability in our study. These results, coupled with results obtained using a semi-mechanism-based pharmacokinetic (PK) model, which described well the observed data with the WT antibody and variant I253A.H435A, support the hypothesis that the effects of FcRn on IgG SC bioavailability are primarily related to FcRn-mediated protection from catabolism at the absorption site (i.e., skin and its surrounding environments).
Results
Binding of anti-Aβ mIgG2a antibodies to mouse FcRn.
Binding of anti-Aβ mIgG2a WT antibody and its Fc variants to mFcRn at pH 6.0 were measured by enzyme-linked immunosorbent assay (ELISA). ELISA plates were coated with NeutrAvidin followed by biotinylated mFcRn. Serial dilutions of standard and variants were added. Bound mIgG2a was detected using horseradish peroxidase (HRP)-conjugated anti-(Fab').Citation2 Titration curves of the WT antibody and variants I253A.H435A, N434H and N434Y are shown in . Variant I253A.H435A did not show detectable mFcRn binding. In contrast, variants N434H and N434Y showed estimated 13.1 and 76.7-fold increased mFcRn binding compared with the WT antibody (). Dissociation of mIgG2a from mFcRn at neutral pH is important for effective recycling of IgG by FcRn. To assess dissociation, the mIgG2a-mFcRn complex at pH 6.0 on the ELISA plate was exposed to a pH 6.0 (B) or a 7.4 (C) buffer before the bound mIgG2a was detected. No titration curve was detected for the WT antibody at pH 7.4, which indicates that the WT antibody was released by mFcRn. Only a slight titration curve was detected for N434H (OD readings at 50 and 0 ng/mL were 0.064 and 0.021, respectively). Comparatively, a titration curve was clearly detected for N434Y. The OD at 50 ng/mL at pH 7.4 was 0.571, which was equivalent to 3.70 ng/mL of N434Y at pH 6.0. This suggested that approximately 7.40% of the complex remained at pH 7.4.
In vitro serum stability study.
Anti-Aβ mIgG2a WT antibody and its three Fc variants (N434H, N434Y and I253A.H435A) were stable in severe combined immunodeficiency (SCID) mouse serum during 7 d in vitro incubation at 37°C. The recovery for all the variants in SCID mouse serum was similar to that in PBS + 0.5% BSA, with mean values ranging from 85% to 105% (Fig. S1).
Pharmacokinetic study in SCID mice.
The WT antibody and variants N434H and N434Y exhibited biphasic disposition after a single IV dose of 5 mg/kg in SCID mice, showing a rapid distribution phase followed by a prolonged elimination phase (). Variant I253A.H435A had a rapid monoexponential disposition after a single IV administration (). The WT antibody, variants N434H and N434Y exhibited comparable clearance (CL) (range from 24.2–27.8 mL/day/kg) and terminal half-life (range from 3.80–3.86 d), and variant I253A.H435A cleared approximately 3-fold faster than the other antibodies with CL of 88.1 mL/day/kg and terminal half-life of 0.590 d (). All antibodies had a rapid absorption phase after a single SC administration (). Variant I253A.H435A had the lowest bioavailability after SC administration compared with others (∼41.8% compared with ∼76.3%, 94.7% and 86.1% for the WT antibody, variants N434H and N434Y, respectively). Of note, although the detection limit of variant N434Y is lower that of the WT and other variants (1.56 ng/mL vs. 3.12 ng/mL), the measured concentration for variant N434Y at all-time points were greater than 3.12 ng/mL, therefore, the different minimal quantifiable concentrations would not influence the pharmacokinetics.
Semi-mechanism-based pharmacokinetic model.
A semi-mechanism-based PK model was developed based on a previously reported modelCitation13 to describe the WT antibody and variant I253A.H435A bioavailability data following IV and SC administration in SCID mice. is the schematic representation of the PK model, which includes the protective role of FcRn in the central as well as the SC compartment. The proposed PK model was able to describe the serum concentration-time profiles for the WT antibody and variant I253A.H435A following IV and SC administration in SCID mice (). The performance of this PK model was also evaluated based on several goodness-of-fit criteria (data not shown). lists the parameter values obtained from the fitting with reasonable coefficient variation. The estimated parameters, such as the capacity of FcRn (Rt), the first order uptake rate constant for IgG from central compartment to endosomal compartment (kup), and the first order degradation rate constant for unbound IgG (kdeg), are consistent with previous reports in reference Citation13 and Citation14.
Discussion
In humans, the pharmacokinetics of IgG after intravenous administration is relatively well-understood; in contrast, little is known about the disposition of IgG following SC administration. Relative to mAbs given IV, the bioavailability following SC administration has been reported to be approximately 50–60% in humans.Citation5 If SC bioavailability can be significantly improved through antibody engineering, administration of mAbs by this route may be a better option.
It has been suggested recently that FcRn, a receptor that protects IgG from catabolism, may play an important role in IgG SC bioavailability.Citation5,Citation6 Understanding IgG disposition, including bioavailability, in the context of the biological interaction between IgG and FcRn in the skin, may have a substantial effect on the development of mAbs for SC administration. The study presented here was designed to test the hypothesis that FcRn may play an important role in IgG SC bioavailability. One anti-Aβ reverse chimeric mIgG2a Fc variant (I253A.H435A) with undetectable mFcRn binding and two Fc variants (N434H and N434Y) with improved mFcRn binding affinity at pH 6.0 were engineered. These variants maintained comparable binding affinity to their target Aβ (data not shown). They likely maintained Fcγ receptor (FcγR) binding as well since previous study showed that human IgG1 Fc variant N434A had normal binding to human FcγRs.Citation15 The pharmacokinetics, including SC bioavailability, of the anti-Aβ mIgG2a WT antibody and its three Fc variants were evaluated in SCID mice. In addition, a semi-mechanism-based PK model was adapted from a previous modelCitation13 to understand how FcRn was involved in the disposition of IgG after SC administration.
SCID mice were used in this PK study to minimize the effect of any anti-therapeutic antibody (ATA) response on the PK profiles. As expected, after IV administration, variant I253A.H435A with undetectable mFcRn binding at pH 6.0 and pH 7.4 had the fastest CL (88.1 mL/day/kg) and shortest terminal half-life (0.590 d) among the four antibodies. This is consistent with one previous report, where variant I253A with negligible binding affinity to human FcRn had a faster CL and shorter half-life in human FcRn transgenic mice compared with the WT antibody.Citation16 It was also observed that variant I253A.H435A had the lowest SC bioavailability compared with the WT antibody and the two Fc variants with improved FcRn binding affinity (∼41.8% compared with ∼76.3%, 94.7% and 86.1% for the WT antibody, variants N434H and N434Y, respectively). The decrease in the systemic availability for variant I253A.H435A with undetectable mFcRn binding affinity in mice may be due to the loss of the protection role provided by mFcRn and the resulting increased pre-systemic catabolism in the skin, a tissue where FcRn expression has been reported in reference Citation8.
The CL and terminal half-life for the WT antibody and two Fc variants (N434Y, N434H) with increased binding affinity to mFcRn at pH 6.0 in SCID mice are comparable (CL is 24.2, 23.9 and 27.8 mL/day/kg and terminal half-life is 3.81, 3.80 and 3.86 d for the WT antibody, variants N434H and N434Y, respectively, after IV administration). Failure to distinguish CL and half-life differences among the variants may be due to the low endogenous IgG concentration in SCID mice, resulting in enhanced FcRn protection. However, a previous study showed that pharmacokinetics of human IgG of different isotypes were statistically indistinguishable in normal and SCID mice,Citation17 and also that PK differences of various human IgG Fc variants could be detected in SCID mice.Citation18 The pharmacokinetics of our WT reverse chimeric mouse IgG2a in SCID mice was also comparable with that in normal mice (C57bl/6J, Fig. S2). Additionally, the reduced half-life of one human IgG1 Fc variant with increased binding affinity to mFcRn at pH 7.4 in SCID miceCitation6 signified that fine PK differences due to different FcRn binding affinity could indeed be identified in our model. The CL of the anti-Aβ mIgG2a WT antibody (23.9 mL/day/kg) is faster than that of a typical mIgG2a antibody (∼10 mL/day/kg) with low endogenous target levels in SCID mice (data not shown). Given the low expression levels of Aβ in animals, target-mediated clearance was not expected to contribute significantly to the overall mAb clearance at a dose of 5 mg/kg for any of the anti-Aβ mAbs. Hence, it is possible that the anti-Aβ WT antibody may have additional clearance mechanisms compared with other mIgG2as in SCID mice (e.g., proteolysis, non-specific binding etc.). In mice lacking expression of FcRn, IgG clearance is increased by approximately 7- to 10-fold;Citation19 however, the anti-Aβ I253A.H435A variant with undetectable mFcRn binding affinity only had ∼3.6-fold faster CL than WT antibody. Therefore, it is possible that the FcRn-mediated clearance pathway may not be predominant and modest modification of the binding affinity to FcRn may not yield a substantial change in the in vivo fate of this mIgG2a antibody, which is consistent with other reports in reference Citation20 and Citation21 as well as our observation that the WT antibody and two Fc variants (N434Y, N434H) have comparable systemic CL and half-life after IV administration. It is plausible that FcRn-mediated protection may be more dominant for the pre-systemic catabolism in the skin. Therefore, changing FcRn binding affinity may have a greater effect on the anti-Aβ mIgG2a SC bioavailability than on the systemic CL in SCID mice.
A unique feature of the interaction of FcRn with IgG is the strict pH dependency. Several studies have now demonstrated that maintaining low binding affinity of IgG to FcRn at pH 7.4 is as essential as the increased binding affinity at pH 6.0 for the increase of IgG half-lifeCitation3,Citation4,Citation12 and SC bioavailability.Citation6 In the current study, SC bioavailability also appears to be influenced by the pH dependency of FcRn-IgG binding. Variant N434H, which has 13.1-fold increased binding affinity at pH 6.0 and comparable binding affinity at pH 7.4 relative to the WT antibody by ELISA (), had a slightly higher SC bioavailability compared with the WT antibody (94.7% vs. 76.3%). This was likely due to greater protection against IgG pre-systemic catabolism by FcRn at pH 6.0. However, variant N434Y, which had the highest increased binding affinity at pH 6.0 (76.7-fold) and a modest increased binding affinity at pH 7.4 relative to the WT antibody by ELISA (), had SC bioavailability comparable to that of the WT antibody (86.1% vs. 76.3%). The greater increase in FcRn binding affinity at pH 7.4 may delay the release of the antibody into systemic circulation and accelerate the degradation rate of IgG locally. Thus, it has a negative effect on IgG bioavailability.
FcRn protects IgG from intracellular catabolism. FcRn has also been shown to be capable of transporting IgG across cell monolayers in both the apical-to-basolateral and basolateral-to-apical direction.Citation1,Citation22 It is not yet known whether the effects of FcRn on SC bioavailability are primarily related to FcRn-mediated protection from catabolism or from FcRn-mediated transport across the vascular endothelium (from interstitial fluid to the blood). In support of either model, FcRn has been shown to both recycle and transcytose IgG in cultured human endothelial cells;Citation22 however, the former mechanism is considered to be more plausible.Citation5 A semi-mechanism-based PK (), which described the observed data well for the WT antibody and variant I253A.H435A, is consistent with the hypothesis that the decreased bioavailability for variant I253A.H435A was due to a loss of the FcRn-mediated protection from catabolism at the absorption site. Because variants N434H and N434Y also had different binding properties at pH 7.4 compared with the WT antibody, the pH dependence of the FcRn-IgG interaction needs to be included in the current model structure to explain all the data. We are collecting more in vivo and in vitro data to establish a model to integrate the current model structure with FcRn pH-binding dependency.
In the current study, mouse was used to investigate the role of FcRn on mAb SC bioavailability. mAbs, in general, have a higher SC bioavailability in mice compared with humans (data not shown). Therefore, the range for SC bioavailability improvement in mice is limited. Of note, the WT antibody in the current study already had a high SC bioavailability (76.3%) in mice. To test the hypothesis that increasing FcRn binding affinity at pH 6.0 would result in greater bioavailability, we need to have a robust in vivo model, as well as a model antibody with a lower SC bioavailability in that model. Nevertheless, the current study demonstrated the effect of FcRn binding on the SC bioavailability of mAbs in mice. The minipig has been a widely used model to determine the SC absorption of small molecules and other proteins due to its physiologic, anatomic, nutritional and metabolic similarities to humans, especially the similarities in the epidermis, such as thickness of epidermis, presence of SC fat layer and epidermal morphology.Citation23 We are currently evaluating the minipig as an animal model for studying the SC bioavailability of mAbs.
The bioavailability of mAbs after SC administration is dependent on many other factors, including proteolytic degradation at the injection site, the conformational and aggregation states of the mAb, and location of the injection site.Citation24 In the current study, all mAbs were administered over the shoulder of the mouse. The anti-Aβ WT and its three Fc variants, which have similar aggregation level in vitro, demonstrated comparable in vitro proteolytic degradation profiles (Fig. S2). Additionally, the mutation on the Fc portion to change the FcRn binding affinity did not impact the FcγR binding affinity or the binding affinity to the Aβ target (data not shown). Therefore, the changes on the bioavailability of the WT and its three Fc variant mAbs after SC administration is most likely due to the differences in the binding affinity to FcRn.
In summary, to our knowledge this is the first study to demonstrate that an engineered mIgG2a mAb with undetectable binding affinity to mFcRn has a lower SC bioavailability in mice compared with the WT antibody. In addition, we also observed that an engineered mIgG2a mAb with higher binding affinity to mFcRn at pH 6.0 and comparable binding affinity at pH 7.4 to the WT anybody had a slightly higher SC bioavailability in mice. A semi-mechanism-based PK model suggests that effects of FcRn on IgG SC bioavailability may be primarily related to FcRn-mediated protection from catabolism at the absorption site. Overall, FcRn plays an important role in IgG SC bioavailability. Engineered mAbs with optimum FcRn binding affinity may improve the bioavailability for mAbs following SC administration. Additional studies, including a dose-dependent study in a higher species for a WT antibody with poor SC bioavailability and further understanding the role of lymphatic drainage on antibody SC absorption, are needed to better understand the role of FcRn on the IgG disposition after SC administration, including SC bioavailability.
Methods
Antibodies.
Full-length reverse chimeric anti-Aβ human/mouse IgG2a WT antibody, consisting of a human anti-Aβ variable region and mouse IgG2a constant regions, and its three Fc variants (I253A.H435A, N434H and N434Y) were generated at Genentech, Inc., (South San Francisco, CA) and formulated in phosphate buffered saline (PBS, pH 6.0). I253A.H435A indicates mutation of Ile253 to Ala253 and His435 to Ala435. N434H and N434Y indicate mutations of Asn434 to His434 and Tyr434, respectively. No significant aggregation was found for all mAbs (WT and its three Fc variants), with aggregation level ranging from 0.43–1.65%.
Measurements of mAb binding affinity to mouse FcRn.
ELISA was used to measure the binding affinity of anti-Aβ WT mIgG2a antibody and its three Fc variants to mFcRn. Previously, we compared binding of human IgG1 wild type, Fc variants N434H and N434Y to human FcRn by ELISA or surface plasmon resonance (SPR) and obtained similar relative binding values of the variants vs. the WT antibody (data not shown). Maxisorp 96-well plates were coated with 2 µg/mL NeutrAvidin (Pierce, Rockford, IL) in 50 mM carbonate buffer, pH 9.6, at 4°C overnight. Plates were washed with phosphate buffered saline (PBS) containing 0.05% polysorbate, pH 7.4 (wash buffer) and blocked with PBS containing 0.5% BSA, pH 7.4. After 1 h incubation at room temperature, plates were washed. Biotinylated soluble mouse FcRn (mFcRn), a heterodimer consisting of the extracellular domain of mFcRn with a His6 tag at the carboxy-terminus and β2-microglobulin (Genentech), was diluted in PBS containing 0.5% BSA, 0.05% polysorbate 20 and 15 ppm Proclin 300 (Supelco, Bellefonte, PA), pH 7.4 and added to the plates. After a 1 h incubation, plates were washed and anti-Aβ antibodies (3.1–200 ng/mL for the WT antibody and variant I253A.H435A or 0.78–50 ng/mL for variants N434H and N434Y) in 2-fold serial dilution in assay buffer at pH 6.0 were added in duplicate and incubated at room temperature for 2 h. Bound antibody was detected with horseradish peroxidase (HRP)-conjugated goat F(ab')2 anti-mouse IgG F(ab')2 antibody (Jackson ImmunoResearch, West Grove, PA) followed by 3,3′,5,5′-tetramethyl benzidine (TMB, Kirkegaard and Perry Laboratories, Gaithersburg, MD) as the substrate. Absorbance was read at 450 nm. For data analysis, the average optical density (OD) at 3.1 and 200 ng/mL anti-A2 WT antibody (mid-OD) was calculated. Antibody concentrations corresponding to this mid-OD for the WT antibody and its three Fc variants (I253A.H435A, N434H and N434Y) were determined from the titration curves using a four-parameter regression curve-fitting program (XLfit, Guildford Surrey, UK). Relative FcRn binding was calculated by dividing the mid-OD concentration of the WT antibody by that of the variant.Citation25 To assess dissociation of bound antibodies from mFcRn at pH 7.4, the FcRn ELISAs were performed as described above, except that a dissociation step was added. After the sample incubation step, the plates were washed and a pH 6.0 or pH 7.4 buffer was added. The plates were incubated for 45 min to allow the mIgG2a-mFcRn complex on the plate to dissociate. The OD at 50 ng/mL at pH 7.4 was assessed and then the concentration corresponding to that OD was calculated based on the IgG titration curve at pH 6.0 (four-parameter curve fitting, KaleidaGraph, Synergy Software, Reading, PA). The percentage of complex remained was calculated by dividing this concentration with 50 and multiplying by 100.
Bioanalysis of serum samples from in vitro serum stability study and in vivo pharmacokinetic study.
Samples from in vitro serum stability study and in vivo PK study in severe combined immunodeficiency (SCID) mice were analyzed by a quantitative ELISA to measure concentrations of anti-Aβ WT antibody and its three Fc variants (I235A.H435A, N434H and N434Y). Briefly, MaxiSorp 384-well plates (Nalge Nunc, Rochester, NY) were coated with human Aβ1–42 peptide (rPeptide, Bogart, GA) at 1 µg/mL in 50 mM carbonate buffer, pH 9.6, at 4°C overnight. Plates were washed with wash buffer and blocked with PBS containing 0.5% BSA. Standards and samples were diluted in sample buffer (PBS containing 0.5% BSA, 0.35 M NaCl, 5 mM EDTA, 0.25% CHAPS, 0.05% polysorbate 20 and 10 ppm Proclin 300) and added to plates. After a 2 h incubation, plates were washed and bound antibody was detected with HRP-labeled goat anti-mouse IgG2a (Bethyl Laboratories, Inc., Montgomery, TX) at 1:5,000 dilution in sample buffer. After a final 1 h incubation, plates were washed and developed using TMB (Kirkegaard and Perry Laboratories). Absorbance at 450 nm was measured on a Multiskan Ascent reader (Thermo Scientific, Hudson, NH). Concentrations were determined from the standard curve using a four-parameter nonlinear regression program. The assay performance was similar for the WT antibody and its three Fc variants. The standard curves ranged from 0.078 to 10 ng/mL for variant N434Y and from 0.156 to 20 ng/mL for the WT antibody, variants I253A.H435A and N434H. This assay tolerated a 1/20 minimum dilution of SCID mouse serum for the WT antibody and Fc variants. Therefore, the minimum quantifiable concentrations in neat SCID mouse serum were 1.56 ng/mL for variant N434Y and 3.12 ng/mL for the WT antibody, variants I253A.H435A and N434H.
In vitro serum stability study.
Anti-Aβ WT antibody and its three Fc variants (I235A.H435A, N434H and N434Y) were spiked into SCID mouse serum and PBS + 0.5% bovine serum albumin (BSA) and were incubated at 37°C for up to 168 h. Briefly, the antibodies were vortexed before being spiked into mouse serum to a final concentration of 200 µg/mL. Each sample was mixed and kept on ice. Aliquots of 100 µL from each mixture were transferred to 0.5-mL microcentrifuge tubes and incubated at 37°C with gentle rotation. To stop the incubation, samples were transferred to dry ice at predetermined timepoints (0, 1, 6, 24, 72 and 168 h) and then stored frozen at −70°C until analysis. The time zero samples (0 h) were transferred immediately from wet ice to dry ice before storage. Antibody concentrations were then analyzed by ELISA at predetermined timepoints (0, 1, 6, 24, 72 and 168 h).
Pharmacokinetic study in SCID mice.
The PK study in SCID mice (Charles River Laboratories, Wilmington, MA) was performed following animal protocols approved by the Institutional Animal Care and Use Committee (IACUC) at Genentech, Inc. SCID female mice received a single IV or SC dose of 5 mg/kg anti-Aβ WT antibody, variants I253A.H435A, N434H and N434Y (n = 12/group). Blood samples were collected from the periorbital sinus or cardiac puncture in the case of terminal time points for PK at the following time points: 15, 30 min; 1, 8, 24 h; 3, 7, 10, 14, 17, 21 and 28 d, and transferred into serum separator tubes. Three mice/group underwent periorbital or terminal bleeding for each time point, with 125 µL blood collected/mouse; these collections were staggered such that an individual mouse was only drawn for three times.
Pharmacokinetic data analysis.
Non-compartmental analysis. Individual anti-Aβ WT antibody and its Fc variants serum concentration vs. time data in mice were naive pooled together and analyzed by non-compartmental approaches (sparse sampling, WinNonlin, version 5.1.2, Pharsight Corp., Mountain View, CA) to provide an estimation of PK parameters in mice. The parameters calculated included the area under the serum concentration-time curve from time = 0 to infinity (AUC0-inf), clearance (CL), volume of distribution at steady-state (Vss), terminal half-life (t1/2,γz) and bioavailability (F). Of note, no significance testing was done for these parameters due to the limitation of the data (sparse design of this study and uncertainties around the assumptions for the statistical test which could be applied to these data).
Semi-mechanism-based pharmacokinetic model.
In this semi-mechanism-based model (), IgG distribution was described by a two-compartmental model following IV administration. The model assumes that IgG distributes within a central compartment, which includes the plasma, and into a peripheral compartment (skin and non-skin) that includes endosomal vesicles. IgG is assumed to distribute to the peripheral compartment by linear processes (e.g., fluid phase endocytosis). Following SC administration, the absorption of IgG into systemic distribution was described by lymphatic uptake and uptake into endosomal space at the absorption site (i.e., skin and its surrounding environments), where IgG can bind to FcRn. Within the endosomes, IgG binds to FcRn at pH 6.0, as dictated by a standard Langmuir-type binding isotherm. Unbound antibody is eliminated (e.g., by intracellular proteolysis), and FcRn-bound IgG is recycled to the central compartment. This model, which is consistent with the accepted mechanism of FcRn-mediated protection of IgG from degradation, is described by equations Equation1(1)
(1) –Equation7
(7)
(7) :
(1)
(1)
(2)
(2)
(3)
(3)
(4)
(4)
(5)
(5)
(6)
(6)
(7)
(7)
In these equations, Xa, X1, X2 and X3 are the mass of IgG (nmole/kg) in the skin interstitial, central compartments, endosomal compartment not including skin, and skin endosomal compartment respectively; V1 is the volume of distribution in the central compartment (L/kg); C1 is the IgG concentrations (nM) in the central and endosomal compartments (assumes IgG reaches equilibrium between the central and endosomal compartment instantly) and R(1) and R(2) are the dose (nmole/kg) for anti-Aβ mAb after SC and IV administration, respectively. Model parameters include a hybrid parameter for first order absorption rate constant and bioavailability through lymphatic uptake (F × ka), a first order uptake rate constant from skin interstitial space to endosomal compartment (ke), a first order uptake rate constant for IgG from central compartment to endosomal compartment (kup), a first order transport rate constant for FcRn-bound IgG (kret), a first order degradation rate constant for unbound IgG (kdeg), the capacity of FcRn in the endosomal compartment [Rt (nM)], which is divided into Rt,1 (non-skin) and Rt,2 (skin), the dissociation constant between IgG and mouse FcRn at pH 6.0 (kd), and the unbound fraction of IgG (fu) in the endosomal compartment (fu,1 and fu,2 for non-skin and skin, respectively). EquationEquations 5(5)
(5) and Equation6
(6)
(6) were derived based on a “Langmuir-type” binding isotherm to characterize the relationship between fu and binding capacity (Rt), binding affinity (kd), and IgG concentrations (C1).
The value of kd was fixed at 750 nM, as reported for the interaction of mouse IgG with mouse FcRnCitation10 and has been used by others for similar model structures.Citation13,Citation14 kret was set to be equal to kup to simplify the model since it is not possible to obtain reliable, independent estimates for kup and kret with the present data.Citation13,Citation14 The competition between endogenous IgG and anti-Aβ mIgG2a for FcRn was not considered here due to the low endogenous IgG level (less than 0.1 µg/mL) in SCID mice.Citation26,Citation27 Based on the work of Garg and Balthasar,Citation7 the amount of FcRn in each tissue was assumed to be proportional to tissue weight, therefore, the capacity of FcRn (nM) in the no-skin endosomal compartment (Rt,1) was set to be equal to that in the skin endosomal compartment (Rt,2). For variant I253A.H435A, fu (fu,1 and fu,2) was set to 1; therefore, no recycling of FcRn-bound IgG would occur and the endocytosed IgG would degrade. All other PK parameters (kdeg, kup, Fxka, V1, Rt) were estimated by simultaneously fitting the model to the mean WT antibody and variant I253A.H435A serum concentrations following IV and SC administration.
The PK parameters were estimated using nonlinear regression analysis with the ADAPT V computer program by the maximum likelihood method.Citation28 The variance model was defined as follows:
where VARi is the variance of the ith data point, σ and γ are the variance parameters, and M (θ,ti) is ith predicted value from the models. Goodness-of-fit was evaluated by visual inspection, model convergence, Akaike Information Criterion (AIC), Schwarz Criterion (SC), estimation criterion value for the maximum likelihood method in ADAPT V, correlation coefficients (R2) and examination of residuals.
Disclosure of Potential Conflicts of Interest
R.D., G.M., K.H., J.L., Y.L., S.I., L.E.D., F.P.T., P.J.F. and S.P. are employees of Genentech, Inc., which supported the study financially.
Abbreviations
mAb | = | monoclonal antibody |
IgG | = | immunoglobulin G |
FcRn | = | neonatal Fc receptor |
mFcRn | = | mouse FcRn |
WT | = | wild type |
PK | = | pharmacokinetic |
SC | = | subcutaneous |
Figures and Tables
Figure 1 Binding and dissociation of anti-Aβ WT mIgG2a (●) and its Fc variants I253A.H435A (○), N434H (▴) and N434Y (△) to mFcRn measured by ELISA. Serially diluted anti-Aβ antibodies were added to biotinylated mFcRn captured on NeutrAvidin-coated plate at pH 6.0. Bound mIgG2a was detected with anti-mouse IgG F(ab′)2-HRP (A). The mid-OD (the average OD reading of the highest and the lowest concentrations) of the titration curve of the WT was 0.213. The concentrations corresponding to this OD were 72.9, 5.57 and 0.950 ng/mL for the WT antibody, variants N434H and N434Y, respectively. The relative mFcRn binding of the variant to the WT was estimated by dividing the WT concentration with the variant concentration. Variants N434H and N434Y had 13.1 and 76.7-fold increased binding compared with the WT, respectively. To assess dissociation of bound antibodies from mFcRn, the mIgG2a-mFcRn complex on the ELISA plates was exposed to a pH 6.0 (B) or a 7.4 (C) buffer for 45 min before the bound mIgG2a was measured.
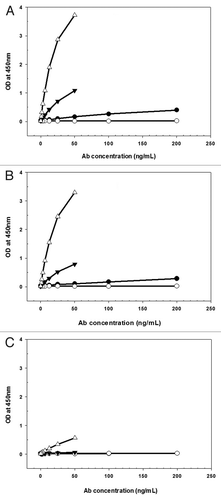
Figure 2 Mean (±SD) anti-Aβ mIgG2a mAbs serum concentration vs. time following single IV (A) or SC (B) administration of 5 mg/kg WT (●), I253A.H435A (○), N434H (▴) or N434Y (△) to SCID mice, n = 3 mice/timepoint.
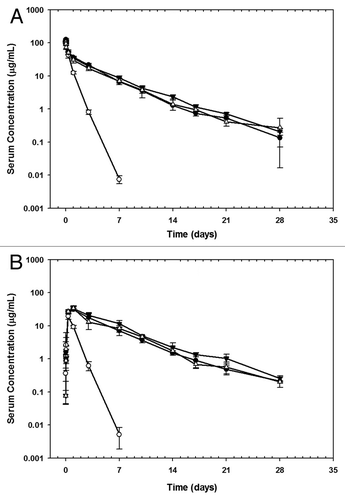
Figure 3 Schematic representation of pharmacokinetic model for anti-Aβ WT and Fc variant I253A.H435A disposition after IV and SC administration in mice. Differential equations describing the change in mIgG2a mAbs concentrations with time for each compartment are described in the text. The symbols are also described in the text.
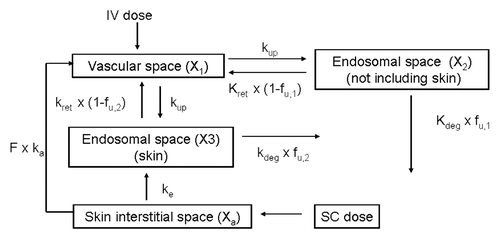
Figure 4 Observed [IV (●) and SC (○)] and model predicted [IV (-), SC (…)] concentration-time profile following single administration of 5 mg/kg anti-Aβ mIgG2a WT (A) and Fc variant I253A.H435A (B) in SCID mice. The concentrations of the WT and variant I253A.H435A were simultaneously fit to the model shown in . Parameter values obtained from the fit are listed in .
![Figure 4 Observed [IV (●) and SC (○)] and model predicted [IV (-), SC (…)] concentration-time profile following single administration of 5 mg/kg anti-Aβ mIgG2a WT (A) and Fc variant I253A.H435A (B) in SCID mice. The concentrations of the WT and variant I253A.H435A were simultaneously fit to the model shown in Figure 3. Parameter values obtained from the fit are listed in Table 2.](/cms/asset/e1eada5d-89b4-497f-92fd-0996e459cab8/kmab_a_10918543_f0004.gif)
Table 1 Non-compartmental pharmacokinetic parameters for anti-Aβ WT and its Fc variants I253A, H435A, N434H and N434Y in SCID mice
Table 2 Pharmacokinetic parameters for anti-Aβ WT and variant I253A.H435A in SCID mice by fitting data to the model shown in
Additional material
Download Zip (185.7 KB)Acknowledgments
Our sincere thanks to Camellia Adams for engineering the antibodies, Ernest Oh for measuring the mIgG2a Abs binding to mouse FcRn and Jose Imperio for conducting the mouse PK study.
References
- Roopenian DC, Akilesh S. FcRn: the neonatal Fc receptor comes of age. Nat Rev Immunol 2007; 7:715 - 725
- Montoyo HP, Vaccaro C, Hafner M, Ober RJ, Mueller W, Ward ES. Conditional deletion of the MHC class I-related receptor FcRn reveals the sites of IgG homeostasis in mice. Proc Natl Acad Sci USA 2009; 106:2788 - 2793; PMID: 19188594; http://dx.doi.org/10.1073/pnas.0810796106
- Dall'Acqua WF, Woods RM, Ward ES, Palaszynski SR, Patel NK, Brewah YA, et al. Increasing the affinity of a human IgG1 for the neonatal Fc receptor: biological consequences. J Immunol 2002; 169:5171 - 5180; PMID: 12391234
- Gan Z, Ram S, Vaccaro C, Ober RJ, Ward ES. Analyses of the recycling receptor, FcRn, in live cells reveal novel pathways for lysosomal delivery. Traffic 2009; 10:600 - 614; PMID: 19192244; http://dx.doi.org/10.1111/j.1600-0854.2009.00887.x
- Wang W, Wang EQ, Balthasar JP. Monoclonal antibody pharmacokinetics and pharmacodynamics. Clin Pharmacol Ther 2008; 84:548 - 558; PMID: 18784655; http://dx.doi.org/10.1038/clpt.2008.170
- Deng R, Loyet KM, Lien S, Iyer S, DeForge LE, Theil FP, et al. Pharmacokinetics of humanized monoclonal anti-tumor necrosis factor-alpha antibody and its neonatal Fc receptor variants in mice and cynomolgus monkeys. Drug Metab Dispos 2010; 38:600 - 605; PMID: 20071453; http://dx.doi.org/10.1124/dmd.109.031310
- Garg A, Balthasar JP. Physiologically-based pharmacokinetic (PBPK) model to predict IgG tissue kinetics in wild-type and FcRn-knockout mice. J Pharmacokinet Pharmacodyn 2007; 34:687 - 709; PMID: 17636457; http://dx.doi.org/10.1007/s10928-007-9065-1
- Borvak J, Richardson J, Medesan C, Antohe F, Radu C, Simionescu M, et al. Functional expression of the MHC class I-related receptor, FcRn, in endothelial cells of mice. Int Immunol 1998; 10:1289 - 1298; PMID: 9786428; http://dx.doi.org/10.1093/intimm/10.9.1289
- Zhu X, Meng G, Dickinson BL, Li X, Mizoguchi E, Miao L, et al. MHC class I-related neonatal Fc receptor for IgG is functionally expressed in monocytes, intestinal macrophages and dendritic cells. J Immunol 2001; 166:3266 - 3276; PMID: 11207281
- Zhou J, Johnson JE, Ghetie V, Ober RJ, Ward ES. Generation of mutated variants of the human form of the MHC class I-related receptor, FcRn, with increased affinity for mouse immunoglobulin G. J Mol Biol 2003; 332:901 - 913; PMID: 12972260; http://dx.doi.org/10.1016/S0022-2836(03)00952-5
- Kim JK, Tsen MF, Ghetie V, Ward ES. Identifying amino acid residues that influence plasma clearance of murine IgG1 fragments by site-directed mutagenesis. Eur J Immunol 1994; 24:542 - 548; PMID: 8125126; http://dx.doi.org/10.1002/eji.1830240308
- Yeung YA, Leabman MK, Marvin JS, Qiu J, Adams CW, Lien S, et al. Engineering human IgG1 affinity to human neonatal Fc receptor: impact of affinity improvement on pharmacokinetics in primates. J Immunol 2009; 182:7663 - 7671; PMID: 19494290; http://dx.doi.org/10.4049/jimmunol.0804182
- Hansen RJ, Balthasar JP. Pharmacokinetic/pharmacodynamic modeling of the effects of intravenous immunoglobulin on the disposition of antiplatelet antibodies in a rat model of immune thrombocytopenia. J Pharm Sci 2003; 92:1206 - 1215; PMID: 12761810; http://dx.doi.org/10.1002/jps.10364
- Deng R, Balthasar JP. Pharmacokinetic/pharmacodynamic modeling of IVIG effects in a murine model of immune thrombocytopenia. J Pharm Sci 2007; 96:1625 - 1637; PMID: 17238194; http://dx.doi.org/10.1002/jps.20828
- Shields RL, Namenuk AK, Hong K, Meng YG, Rae J, Briggs J, et al. High resolution mapping of the binding site on human IgG1 for Fcgamma RI, Fcgamma RII, Fcgamma RIII and FcRn and design of IgG1 variants with improved binding to the Fcgamma R. J Biol Chem 2001; 276:6591 - 6604; PMID: 11096108; http://dx.doi.org/10.1074/jbc.M009483200
- Petkova SB, Akilesh S, Sproule TJ, Christianson GJ, Al Khabbaz H, Brown AC, et al. Enhanced half-life of genetically engineered human IgG1 antibodies in a humanized FcRn mouse model: potential application in humorally mediated autoimmune disease. Int Immunol 2006; 18:1759 - 1769; PMID: 17077181; http://dx.doi.org/10.1093/intimm/dxl110
- Zuckier LS, Georgescu L, Chang CJ, Scharff MD, Morrison SL. The use of severe combined immunodeficiency mice to study the metabolism of human immunoglobulin G. Cancer 1994; 73:794 - 799; PMID: 8306262; http://dx.doi.org/10.1002/1097-0142(19940201)73:3+<794::AID-CNCR2820731308>3.0.CO;2-A
- Zuckier LS, Chang CJ, Scharff MD, Morrison SL. Chimeric human-mouse IgG antibodies with shuffled constant region exons demonstrate that multiple domains contribute to in vivo half-life. Cancer Res 1998; 58:3905 - 3908; PMID: 9731501
- Roopenian DC, Christianson GJ, Sproule TJ, Brown AC, Akilesh S, Jung N, et al. The MHC class I-like IgG receptor controls perinatal IgG transport, IgG homeostasis and fate of IgG-Fc-coupled drugs. J Immunol 2003; 170:3528 - 3533; PMID: 12646614
- Datta-Mannan A, Witcher DR, Tang Y, Watkins J, Wroblewski VJ. Monoclonal antibody clearance. Impact of modulating the interaction of IgG with the neonatal Fc receptor. J Biol Chem 2007; 282:1709 - 1717; PMID: 17135257; http://dx.doi.org/10.1074/jbc.M607161200
- Datta-Mannan A, Witcher DR, Tang Y, Watkins J, Jiang W, Wroblewski VJ. Humanized IgG1 variants with differential binding properties to the neonatal Fc receptor: relationship to pharmacokinetics in mice and primates. Drug Metab Dispos 2007; 35:86 - 94; PMID: 17050651; http://dx.doi.org/10.1124/dmd.106.011734
- Antohe F, Radulescu L, Gafencu A, Ghetie V, Simionescu M. Expression of functionally active FcRn and the differentiated bidirectional transport of IgG in human placental endothelial cells. Hum Immunol 2001; 62:93 - 105; PMID: 11182218; http://dx.doi.org/10.1016/S0198-8859(00)00244-5
- Mahl JA, Vogel BE, Court M, Kolopp M, Roman D, Nogués V. The minipig in dermatotoxicology: methods and challenges. Exp Toxicol Pathol 2006; 57:341 - 345; PMID: 16713702; http://dx.doi.org/10.1016/j.etp.2006.03.004
- Kagan L, Turner MR, Balu-Iyer SV, Mager DE. Subcutaneous Absorption of Monoclonal Antibodies: Role of Dose, Site of Injection and Injection Volume on Rituximab Pharmacokinetics in Rats. Pharm Res 2011; In press PMID: 21887597; http://dx.doi.org/10.1007/s11095-011-0578-3
- Lu Y, Vernes JM, Chiang N, Ou Q, Ding J, Adams C, et al. Identification of IgG(1) variants with increased affinity to FcγRIIIa and unaltered affinity to FcγRI and FcRn: comparison of soluble receptor-based and cell-based binding assays. J Immunol Methods 2011; 365:132 - 141; PMID: 21185301; http://dx.doi.org/10.1016/j.jim.2010.12.014
- Bosma MJ, Carroll AM. The SCID mouse mutant: definition, characterization and potential uses. Annu Rev Immunol 1991; 9:323 - 350; PMID: 1910681; http://dx.doi.org/10.1146/annurev.iy.09.040191.001543
- Michel RB, Ochakovskaya R, Mattes MJ. Rapid blood clearance of injected mouse IgG2a in SCID mice. Cancer Immunol Immunother 2002; 51:547 - 556; PMID: 12384806; http://dx.doi.org/10.1007/s00262-002-0319-x
- D'Argenio D, Schumitzky A, Wang X. ADAPT 5 User's Guide: Pharmacokinetic/Pharmacodynamic Systems Analysis Software. Biomedical Simulations Resource, Los Angeles 2009;