Abstract
We have recently reported numerous cases of horizontal transfers of transposable elements between species of drosophilids. These studies revealed a substantial number of horizontal transfers between species of the subgroup melanogaster of the genus Drosophila and between these species and species of the genus Zaprionus. In this review, these transfers and similar, previously reported events are discussed and reanalysed to portray the interrelationships between the species that allowed the occurrence of so many horizontal transfers. The paper also addresses problems that may arise in drawing inferences about the time period during which the horizontal transfers occurred and the factors that may be associated with these transfers are discussed.
Transposable elements (TEs) belong to a particular category of DNA sequences. They have been labeled at various times as parasiticCitation1 or junk DNA,Citation2 seeds of evolutionCitation3 and genomic treasures.Citation4 TEs are governed by regular evolutionary processes, such as nucleotide substitutions, deletions and insertions and structural rearrangements.Citation5,Citation6 Nevertheless, owing to their capacity for mobilization, TEs combine these processes to form an evolutionary cycle that includes vertical and horizontal inheritance, a burst of transposition, domestication, and sequence degeneration (for a recent review see ref. Citation7). The cycle can start when an active TE arises in a naive genome through horizontal transfer (HT) of a pre-existing copy in a donor species. Alternatively, TEs can be reborn in a genome through the recombination of pre-existing eroded sequences (de novo origin).Citation8 After introduction or reintroduction, the TEs duplicate themselves and spread throughout the host genome. They often cause a burst of transposition that culminates in a large increase in the copy number. Following this phase, because the TEs generally serve no immediate function in the host genome, a degenerative process occurs over time, and they may progressively decay and eventually disappear.Citation8,Citation9 Throughout these phases, vertical transmission is the main form of inheritance, and the ultimate fate of a TE in a genome is vertical inactivation and stochastic loss. Paradoxically, the TEs are the most abundant sequences in the eukaryote genomes. This paradox might be explained in part by the ability of TEs to move between genomes and to increase in copy number after invasion. In such a situation, an element has to be immediately active and transpositionally efficient; otherwise, it may be lost due to genetic drift and natural selection.Citation10,Citation11
In recent years, we have reported numerous cases of HTs of TEs between species of drosophilids.Citation12–Citation16 These studies and reports by other authors (for references see Table S1) revealed an outstanding number of HTs between Drosophila species of the subgroup melanogaster and between these species and species of the genus Zaprionus. This prevalence might result from the fact that the subgroup includes D. melanogaster and D. simulans, the most studied species of the genus Drosophila. The high number of HTs between these species allows comparisons to portray the interrelationships between both closely related and distantly related species that allowed the occurrence of so many HTs.
Before focusing on this objective, we should note that the taxonomic position of the genus Zaprionus within the Drosophilidae is still an open question. Zaprionus was first classified as a subgenus of the genus Drosophila.Citation17 Nevertheless, this classification was criticized, primarily because ThrockmortonCitation17 did not follow the concept of monophyly and did not make explicit the phylogenetic methods on which the analysis was based.Citation18 Further analyses using morphological charactersCitation19 and mtDNA markersCitation20 indicated that Zaprionus and other subgenera previously included by ThrockmortonCitation17 in the genus Drosophila should be elevated to generic status. This classification has subsequently been followed. However, studies using different molecular markers have continued to corroborate the original positioning of Zaprionus within the genus Drosophila.Citation21–Citation25 Despite these results, the scientific community still considers Zaprionus as a separate genus. Regardless of the phylogenetic position adopted for the genus Zaprionus, their species and those of the melanogaster subgroup (subgenus Sophophora, genus Drosophila), the focus of this review, have evolved separately for at least 40 Mya and may be as old as 60 Mya.Citation26,Citation27
Two Intertwined Evolutionary Histories
The melanogaster subgroup apparently originated in tropical Africa, where it evolved from a proto-melanogaster founder population that arrived on the continent ∼17–20 Mya from the Oriental biogeographic region. Lachaise et al. offered a historical reconstruction of the distribution pattern of the nine species of the melanogaster subgroup.Citation28 These authors proposed the existence of three speciation centers in Africa (western, central and eastern) that produced the three species complexes in the subgroup in the following manner. The erecta (D. erecta, D. orena) and the yakuba (D. yakuba, D. teissieri and D. santomea) complexes evolved in the western region from the melanogaster common ancestor lineage at approximately 13–15 Mya and 8–15 Mya, respectively. In the melanogaster complex (D. melanogaster, D. simulans, D. sechellia and D. mauritiana), the D. melanogaster ancestor first evolved 2–3 Mya in Central Africa. In Madagascar or in the Indian Ocean islands, the ancestors of the three species of the simulans subcomplex (D. simulans, D. sechellia and D. mauritiana) apparently arose from the same splitting event at approximately 0.40 Mya ().Citation29 The species of the melanogaster complex currently show distinct geographical distributions and ecological patterns. D. melanogaster and D. simulans are opportunist and cosmopolitan. D. sechellia is specialized and geographically confined to the Seychelles, whereas D. mauritiana is semi-domestic and is confined to the island of Mauritius. The divergence times reported above are generally assumed in the literature.Citation29,Citation30 However, more recent estimates suggest that the divergence between the complexes and subcomplexes of the melanogaster group may be older.Citation27
Figure 1 Graphic reconstruction of the historical biogeography of the three complexes of species (erecta, yakuba and melanogaster) subgroups of the melanogaster group of the genus Drosophila (reddish colors) and of the two species groups (armatus and inermis) of the subgenus Zaprionus of the Zaprionus genus (blue colors). The ages of the African continent colonization of the melanogaster subgroupCitation28,Citation29 and Zaprionus subgenusCitation32 and lineages diversification in Tropical Africa are indicated (Mya). The area demarcated by green dotted lines represents the returning to sympatry of the species D. melanogaster and D. simulans after speciation of the first in Central Africa and second in Madagascar and the Indian Ocean islands. (This figure was modified from refs. Citation29 and Citation30.)
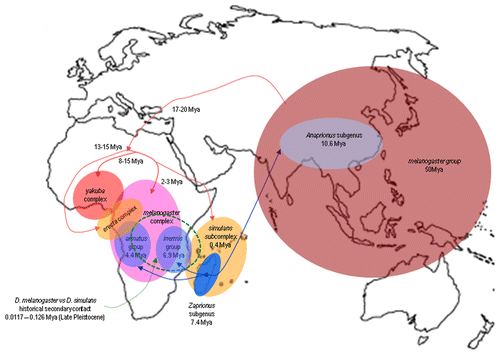
D. melanogaster and D. simulans are currently cosmopolitan, but they were not always sympatric. The current biogeographic distribution of D. melanogaster and D. simulans in the Afrotropical region shows a marked west-to-east separation that indicates the distinct origins of the two taxa. D. melanogaster is widespread in domestic and wild habitats in the western Afrotropical region, whereas D. simulans is rare. In contrast, D. simulans is widespread and abundant in East Africa, Madagascar and the Indian Ocean islands and is sometimes confined to wild habitats, whereas D. melanogaster is rare and occasionally occurs in domestic habitats.Citation31 It has been proposed that after speciation in allopatry in separate western and eastern regions, an ancient secondary contact occurred in the late Pleistocene, when D. simulans dispersed to East Africa and contacted the large autochtonous populations of D. melanogaster.Citation28 Subsequently, both species probably continued to evolve in sympatry over a large area of equatorial West Africa.
The genus Zaprionus Coquillett, 1901 (Drosophilidae) also originated in the Oriental region, apparently during the Late Miocene (∼10 Mya).Citation32 It is classified into two subgenera that are presently geographically disjoint: Zaprionus, in the Afrotropical biogeographic region, and Anaprionus, a subgenus that remained in the Oriental region.Citation33 The exceptions to this pattern are two Zaprionus species that have recently invaded other continents, Z. indianus and Z. tuberculatus.Citation34,Citation35 The subgenus Zaprionus contains 44 described species, which are very common and abundant in drosophilid communities and seem to have approximately the same age and geographic origin as the species of the melanogaster subgroup. Analyses of mitochondrial and nuclear markers and a reconstruction of the historical biogeography of the group with a maximum likelihood model suggest that an ancestor lineage of the Zaprionus subgenus colonised Africa during the Quaternary (∼7 Mya).Citation32 Subsequently, two groups of species originated in tropical Africa, the inermis group on the islands of the Indian Ocean (6.9 ± 0.8 Mya), with many independent dispersals between mainland Africa and these islands, and the armatus group in Central Africa (4.37 ± 0.99 Mya).Citation32 illustrates the proposed regions and periods of the radiation of the subgenus Zaprionus.
The scenario proposed above shows that the species of the melanogaster subgroup and of the subgenus Zaprionus overlapped geographically and temporally in tropical Africa. One of the requirements for HT to occur is therefore satisfied. However, geographical overlap is not sufficient for HTs to occur. In addition, ecological overlap must be present, at least partially, and might have occurred between both species groups. Drosophilid communities consist primarily of saprophagous species that develop in decaying plants, including sweet fruits, leaves, vegetables, flowers, and fungi. The species of the melanogaster group tend to use rotting fruits, flowers, other plant parts, and cactus as substrates for feeding and breeding sites.Citation18 Species of the Zaprionus genus also breed on flowers and fruitsCitation36 and have been found sharing breeding sites with Drosophila species. For example, 222 individuals of Z. indianus, 118 of D. nebulosa, and 18 individuals classified into three other species emerged from fallen fruits of Hancornia speciosa, a common plant species of the Brazilian savanna.Citation37 The flies feed upon the microbial community (bacteria, molds, and yeasts) responsible for the decomposition of this material as well as on the decomposed material itself. Most important for the focus of this addendum is that flies deposit microbes at breeding sites and on the surfaces of oviposited eggs through their faecal deposits.Citation38,Citation39 This environment is highly conducive to the occurrence of HT mediated by vectors such as viruses, bacteria and microparasitoids. In addition, the ecological overlap in this environment allows physical contact that may favor hybridization between closely related species. Thus, the species of these two groups have had the opportunity to exchange TEs during the period of their diversification in Africa as well as at later times.
Exchange of TEs between Species of the Melanogaster Subgroup and Species of the Subgenus Zaprionus
A total of 113 putative HT events between species of the melanogaster subgroup and between them an those of the subgenus Zaprionus have been reported to date (Table S1). The occurrence of HTs involving LTR retrotransposons (99) is significantly higher than that of non-LTR retrotransposons (5) and DNA transposons (9), as previously reported for the genus Drosophila.Citation40 A total of 52 different families or sub-families have been found to be involved in these events, being 43 of LTR and 4 of both non-LTR retrotransposons and of DNA transposons.
Traditionally, the occurrence of HTs between species is inferred according to three criteria: (1) a high sequence similarity between the TEs of distantly related species; (2) a discontinuous distribution of the TE among a group of species; and (3) a lack of congruence between the host and the TE phylogenies.Citation40 All the HT inferences listed in Table S1 were supported by analyses of similarity between nucleotide or amino acid sequences, of shared specific signatures, and of phylogenetic reconstructions that aimed to demonstrate a lack of congruence between the TE and the host phylogenies or a discontinuous distribution of the TEs within the phylogenies. Various approaches have been incorporated in these analyses to help strengthen the HT inferences. One noteworthy approach is to analyze the TE rate of the synonymous substitution (Ks or Ds) and that of nuclear genes.Citation14–Citation16,Citation41–Citation47 This approach allows the HT event to be dated using the rationale that if the TEs are evolving vertically and the lack of congruence in the phylogeny is a consequence of differential subfamily distribution in the species analyzed, the Ks values of the TE sequences and those of the nuclear gene(s) should be equivalent. However, if the Ks of the TE are significantly lower than that of the nuclear gene, the lower Ks values can be explained by a small divergence time of the TE copies between the species as a consequence of HT. This estimate can be obtained with the molecular clock equation (r = k/2T, where r is the evolutionary rate for synonymous sites in genes with low codon bias in Drosophila, k is the Ks pairwise divergence, and T is the divergence time between species). Two different rates of substitution at neutral sites have been estimated for Drosophila genes, 0.016 subs/site/MyCitation48 and 0.011subs/site/ My.Citation27 Table S1 include the estimated times for the occurrence of HTs.
The data of the LTR retrotransposons will be used below to estimate the average ages of the HTs within groups of species. Before we present these results, we need to offer one caveat. The age estimates in the literature must be interpreted with caution and should only be considered as approximations to the times at which the HTs may have occurred. The use of partial or complete sequences to calculate Ks may produce differing results. Complete coding sequences should be used to calculate Ks. This task is straightforward if we are studying species with sequenced genomes. Nevertheless, such analyses are not always possible, and many such estimates in Drosophila and other organisms are based on partial TE sequences owing to the limited number of sequenced genomes. In such cases, although this approach is not ideal, the analysis of partial sequences is the only way to infer the evolutionary history of TEs. To illustrate this issue, 12 sequences of the retrotransposon Copia were used to estimate Ks and the age of the most recent common ancestor of these sequences (11 sequences extracted from chromosome 2L of D. melanogaster and the Copia coding sequence X02599 from GenBank). The complete CDSs (gag + pol) were aligned and slightly edited (owing to the presence of superimposed codons) to produce a 4,227-bp multiple alignment. The gag (nt 1–810) and integrase (nt 1,285–2,001) domains were also aligned separately. Table 1 shows the differences that result from using the entire Copia CDS or using different domains. The average times of origin of the ancestor sequence differ approximately 3-fold, from 82,700 y (only gag) to 145,630 y (gag + pol) and 236,000 y (only integrase). Hence, the results can be biased if one portion has evolved very fast or very slowly. Such estimates illustrate that if the entire coding sequences cannot be used, the results should be interpreted as a rough estimate to avoid compromising the inferences to be made. All these sequences include copies that involve very recent descent from a common ancestral sequence (0 y) as well as copies with maximum ages varying from 313,640 y (complete CDS) to 595,000 y (only integrase). These values are consistent with estimates showing that most of the euchromatic LTR retrotransposons in the D. melanogaster genome are less than 200,000 y old and are derived from a recent amplification event that occurred less than 500,000 y ago.Citation42
Since active TEs might suffer selective pressure to maintain their coding sequences, to test whether the estimated TE conservation is due to selective constraints or to HT, the ratio of non-synonymous divergence per synonymous divergence have to be estimated and compared with those of the nuclear genes. Nuclear genes would be expected to be even more restricted if they play essential role in the cell metabolism. Following Silva and Kidwell (2000), a horizontal transfer event can be inferred when the estimated TE divergence is significantly lower than that of host genes under similar or higher levels of selective constraints than those operating on the TEs themselves. A better alternative possibly is to compare the Ks of TEs with other genomic regions presumably evolving neutrally in order to choose the best regions to be used as molecular clock.
We can now examine the information provided by the estimated times of the HTs about the history of these TEs in the melanogaster subgroup and subgenus Zaprionus. Because the LTR retrotransposons are the most frequent TEs involved in the HTs (88%), they will represent the focus of the subsequent discussion. As indicated above, all of the species involved in the HT events listed in Table S1 show overlapping geographic and temporal distributions and could have had some degree of niche overlap. These prerequisites are essential for the exchange of genetic material. As stressed above, the breeding sites of the species can be rich in organisms that can act as vehicles of TEs, such as viruses,Citation49 symbiotic bacteria,Citation50 or parasites and parasitoids, such as bugs,Citation51 mitesCitation52 and wasps.Citation53 However, vectors are not strictly required for some types of TEs. LTR retrotransposons such as Gypsy, which produce virus-like particles, can potentially be transmitted without the presence of a vector.Citation54 This property by itself can explain the high frequency of HT involving the Gypsy subfamilies in Table S2. Some of the transfers are relatively recent, e.g., those occurring between D. melanogaster and D. erecta (Gypsy S1: 0–3.1 Mya) and between D. erecta and D. yakuba (Gypsy6: 0.562 Mya).Citation44 However, the HTs can also have occurred in more distant times, e.g., those occurring between D. erecta and D. melanogaster (Gypsy S2: 2.5–3.9 Mya) and between D. erecta and Z. tuberculatus (Gypsy S2: 5.2–11.2 Mya).Citation15 However, the fact that Gypsy produces viral particles does not exclude the possibility of other modes of transmission, such as transmission by vectors or even sexual transmission via introgressive hybridization. The last-named possibility is a very likely way for exchanging TEs between species of the melanogaster complex because intercrosses and introgression can eventually occur among D. melanogaster, D. simulans, D. mauritiana and D. sechellia.Citation55–Citation57
illustrates the distribution of 99 average ages of HTs estimated for LTR retrotransposons shared by species of the melanogaster subgroup and shared with species of the subgenus Zaprionus listed in Table S1. The values used are only those for which the authors stated that HT was the most likely explanatory hypothesis for the ages obtained. If the ages of the HTs were not calculated by the authors, they were estimated from the published Ks values.Citation45,Citation58,Citation59 Importantly, among these data there is a general estimate of the HTs involving 37 TE families of D. melanogaster, D. simulans and D. yakuba as having “occurred over a broad period of time centered between 30,000 and 40,000 years ago” based on the Ks range 0.03–0.05.Citation45 It is necessary to make two comments about this result. First, the authors did not aim to calculate specific ages for each transfer; however, the values do not correspond to the reported Ks. For the range 0.03–0.05, the ages should be 1.36 to 2.72 Mya (using the rate of 0.011 substitutions/site/My and the equation r = kS/2T). So, to get an estimate of the age of the HTs between these pairs of species, we used the Ks values identified by the authors as significantly lower than those of the host genes (see their and Table S1) and calculated the age for each transfer. The average values obtained were 1.31 My for the pair D. melanogaster / D. yakuba (Ks = 0.029), 1.41 My for D. simulans / D. yakuba (Ks = 0.031), and 409,000 y for D. melanogaster / D. simulans (Ks = 0.009), much higher than those above-cited; nevertheless, still suggestive of HT between these species. Second, with the knowledge of the authors, we utilized in the ages of the HT estimated for these pairs of species.
Figure 2 Distribution of ages (ordinate) of 60 HT events (abscissa) of LTR retrotransposons between species of the melanogaster subgroup and with those of the subgenus Zaprionus. The blue bars correspond to HTs between species of the melanogaster subgroup (light blue: within the melanogaster complex, indigo: between the supercomplex melanogaster and dark blue: between the complexes melanogaster, yakuba and erecta). The green bars represent HTs between species of the melanogaster subgroup and those of the genus Zaprionus (light green), and between the Zaprionus species (dark green). The unfilled bars represent the HT between an unknown donor and species of the melanogaster subgroup (black border) or the Zaprionus subgenus (green border). Columns: HT ages according to refs. Citation15, Citation16, Citation43, Citation44, Citation45, Citation46, Citation58, Citation59 and Citation67.
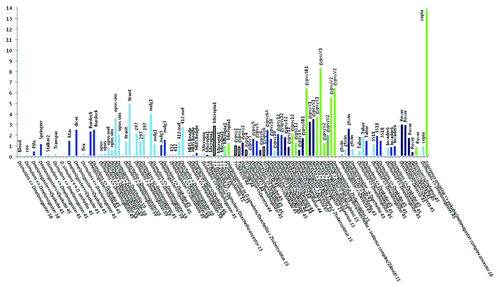
Three groups of ages were formed for this analysis. These groups reflected the phylogenetic relationships between species or between species complexes and were ordered from the closest to the most distant degree of phylogenetic relationship. The blue bars correspond to estimates of the ages of HT between species of the melanogaster subgroup. Group 1 corresponded to transfers inside the melanogaster complex (between D. melanogaster / D. simulans / D. sechellia). Group 2 corresponded to transfers among the complexes of the melanogaster subgroup (the melanogaster complex, yakuba complex and erecta complex). The green bars represent estimates of the ages of HT between species of the melanogaster subgroup and the subgenus Zaprionus (group 3, light green), and among the Zaprionus species (dark green). The unfilled bars represent the ages of HT between an unknown donor and species of the melanogaster subgroup (black border) or the Zaprionus genus (green border). As previously indicated, the estimates should be regarded as approximations because the complete TE CDSs were not used in all the studies. Subject to this caution, the data reveal that the periods for HTs are consistent with the periods during which the species shared space and time. As shown in , the average HT occurrence of 37 LTR retrotransposons was dated at 0.957 Mya within the melanogaster complex, after the split that produced two evolutionary lineages, D. melanogaster and the D. simulans sub-complex, approximately 2 Mya.Citation29,Citation30 The maximum value for the age of an HT occurrence was 4.8 Mya (tirant, between D. melanogaster and D. sechelliaCitation59). Two observations apply to this value and other values that exceed the estimated time of divergence between species or that represent times at which the species supposedly did not share the same geographical region. It should first be noted that the estimated divergence times in the melanogaster group may vary depending on the source, as mentioned above.Citation27,Citation29,Citation30 It is also possible that the event may have involved the ancestors of the species studied rather than their current representatives. The ages for the pair D. melanogaster / D. simulans (N: 27) is 0.687 Mya. These averages suggest that several LTR retrotransposon transfers have occurred between D. melanogaster and the common ancestor of D. simulans and D. sechellia, as previously indicated.Citation59 However, given the variation in these ages and that some of them can be overestimated because the sequences used were mostly partial, it can be possible that HTs may have occurred more recently, during the period when both species returned to sympatry in mainland Africa, possibly during the Late Pleistocene, in agreement with the proposition that most of the retrotransposons in the D. melanogaster are less than 200,000 y old and can be derived from elements recently introduced via horizontal transfer.Citation42
The average ages of HTs between species of the complexes melanogaster, yakuba and erecta (1.58 My) is higher the average of the melanogaster complex. Twenty-three of these 33 comparisons involve exchanges of TEs between D. melanogaster and D. yakuba or D. erecta. The ranges of D. melanogaster, D. yakuba and D. erecta show the geographical overlap necessary for such transfers (). The estimated age suggests that the transfers occurred between species of these complexes when those of the melanogaster complex were beginning to diversify. Finally, we can observe that the estimated average age of the HTs between species of the melanogaster subgroup and those of the genus Zaprionus is significantly higher (4.32 My). This value matches the time period during which the species of the groups armatus (4.4 Mya) and inermis (6.9 Mya) and the ancestral lineages of the complexes melanogaster, yakuba and erecta diversified in tropical Africa ().
The factors associated with these HTs also deserve to be investigated. Many of these species are closely related (e.g., the melanogaster complex), but others evolved more than 50 My apart (e.g., the subgenus Zaprionus and the melanogaster subgroup). Overlap in space and time seems to have persisted since both groups of species began to diverge. We cannot give positive proof of ecological overlap, but this possibility cannot be ignored. Given the presence of the prerequisites for the HTs, what kinds of factors facilitated these exchanges? Unfortunately, we can only speculate about these factors by reasoning from the population or genomic characteristics of the species. Because vectors are essential for many of the HTs, we can consider the existence of a past or present common putative vector (e.g., viruses, bacteria) for these species. This hypothesis is very plausible, but data relevant to the hypothesis are still not available. These vectors may well exist, but the reason that the transfers seem to occur preferentially in one direction remains unclear. For example, the transfers seem to occur preferentially from the D. simulants / D.sechellia ancestor to D. melanogaster or to the species of the subgenus Zaprionus. Could some species be more permissive to HTs than others? A possible answer to this question is that the effective population size (Ne) may influence the outcome of the HT. A small Ne intensifies genetic drift and thereby allows TEs to proliferate, whereas a large Ne would produce a more efficient elimination of the laterally introduced TEs by selection.Citation60 Because D. melanogaster currently seems to have a smaller Ne than D. simulans,Citation30,Citation61.Citation62 this factor could explain the apparent higher frequency of transfers in the direction of D. melanogaster. Although both species have the same population size, as suggested by recent data,Citation63 a small value of Ne during the beginning of speciation or a decrease in Ne during colonization by invasive species, such as D. melanogaster, D. simulans,Citation64 and the Zaprionus species,Citation34,Citation35,Citation65 could enhance HTs owing to proliferation of the recently introduced TE. An alternative hypothesis is that the D. simulans genome is not resilient to invasion but can resist the proliferation of TEs, as experimentally demonstrated.Citation66
Another possibility is that introgression may have been frequent during the process that produced reproductive isolation. Frequent introgression would explain the massive exchange of TEs that occurred between the ancestral lineages of D. simulans and D. melanogaster.Citation59 The average occurrence times for the LTR retrotransposons exchanging between the species of the melanogaster complex (0.957 Mya) seem also to support this hypothesis. However, introgression between species that are more distantly related is implausible.
Neither the genome sizes of the Zaprionus species nor the TE contents of these species are known. The subgenus Zaprionus is rich in species, and these species share an evolutionary history in Africa since 7 Mya with species of the subgroup melanogaster of the genus Drosophila. They also share transposable elements, genetic sequences essential for genome evolution. Genomic and population data on this group of species are still very scarce. There is great need for such data to estimate the extent to which mobile elements are exchanged by distantly related species and to find clues to the determinants and effects of these transfers.
Figures and Tables
Table 1 Comparative analysis of Ks and estimation of the average divergence time (Mya) from the common ancestor sequence of 12 sequences of the retrotransposon Copia in D. melanogaster.
Table 2 Mean values of the HT ages (Mya) of LTR retrotransposons between groups of species differing by degrees of evolutionary relatedness calculated according to the equation r = kS/2T (r = 0.011Citation25)
Additional material
Download Zip (126.1 KB)Acknowledgments
The author is grateful N. Setta for comments during the preparation of this article and for making available 11 sequences of the retrotransposon Copia extracted from the D. melanogaster genome. The data produced in the C.M.A.C laboratory cited in this work were supported by grants from the Brazilian Agencies CNPq (Conselho Nacional de Desenvovimento Científico e Tecnológico) and FAPESP (Fundação de Amparo à Pesquisa do Estado de São Paulo) and the CAPES-COFECUB International Cooperation Program.
References
- Orgel LE, Crick FHC, Sapienza C. Selfish DNA. Nature 1980; 288:645 - 646; PMID: 7453798; http://dx.doi.org/10.1038/288645a0
- Ohno S, Yomo T. The grammatical rule for all DNA: junk and coding sequences. Electrophoresis 1991; 12:103 - 108; PMID: 2040257; http://dx.doi.org/10.1002/elps.1150120203
- Brosius J. Retroposons-Seeds of Evolution. Science 1991; 251:753; PMID: 1990437; http://dx.doi.org/10.1126/science.1990437
- Nowak R. Mining treasures from junk DNA. Science 1994; 263:608 - 610; PMID: 7508142; http://dx.doi.org/10.1126/science.7508142
- Jordan IK, McDonald J. Interelement Selection in the regulatory region of the copia Retrotransposon. J Mol Evol 1998; 47:670 - 676; PMID: 9847408; http://dx.doi.org/10.1007/PL00006425
- Petrov DA, Hartl DL. High rate of DNA loss in the Drosophila melanogaster and Drosophila virilis species groups. Mol Biol Evol 1998; 15:293 - 302; PMID: 9501496
- Biémont C. A Brief History of the Status of Transposable Elements: From Junk DNA to Major Players in Evolution. Genetics 2010; 186:1085 - 1093; PMID: 21156958; http://dx.doi.org/10.1534/genetics.110.124180
- Pinsker W, Haring E, Hagemann S, Miller WJ. The evolutionary life history of P transposons: from horizontal invaders to domesticated neogenes. Chromosoma 2001; 110:148 - 158; PMID: 11513290; http://dx.doi.org/10.1007/s004120100144
- Brookfield JFY. The ecology of the genome -Mobile DNA elements and their hosts. Nat Rev Genet 2005; 6:128 - 136; PMID: 15640810; http://dx.doi.org/10.1038/nrg1524
- Le Rouzic A, Capy P. The first steps of transposable elements invasion: Parasitic strategy vs. genetic drift. Genetics 2005; 169:1033 - 1043; PMID: 15731520; http://dx.doi.org/10.1534/genetics.104.031211
- Le Rouzic A, Boutin TS, Capy P. Long-term evolution of transposable elements. Proc Natl Acad Sci USA 2007; 104:19375 - 19380; PMID: 18040048; http://dx.doi.org/10.1073/pnas.0705238104
- de Almeida LM, Carareto CMA. Multiple events of horizontal transfer of the Minos transposable element between Drosophila species. Mol Phylogenet Evol 2005; 35:583 - 594; PMID: 15878127; http://dx.doi.org/10.1016/j.ympev.2004.11.026
- De Almeida LM, Carareto CMA. Sequence heterogeneity and phylogenetic relationships between the copia retrotransposon in Drosophila species of the repleta and melanogaster groups. Genet Sel Evol 2006; 38:535 - 550; PMID: 16954045; http://dx.doi.org/10.1186/1297-9686-38-5-535
- de Setta N, Loreto ELS, Carareto CMA. Is the evolutionary history of the O-type P element in the saltans and willistoni groups of Drosophila similar to that of the canonical P element?. J Mol Evol 2007; 65:715 - 724; PMID: 18034216; http://dx.doi.org/10.1007/s00239-007-9051-7
- de Setta N, Van Sluys MA, Capy P, Carareto CMA. Multiple invasions of Gypsy and Micropia retroelements in genus Zaprionus and melanogaster subgroup of the genus Drosophila.. BMC Evol Biol 2009; 9:279; PMID: 19954522; http://dx.doi.org/10.1186/14712148-9-279
- de Setta N, Van Sluys MA, Capy P, Carareto CMA. Copia Retrotransposon in the Zaprionus Genus: Another Case of Transposable Element Sharing with the Drosophila melanogaster Subgroup. J Mol Evol 2011; 72:326 - 338; PMID: 21347850; http://dx.doi.org/10.1007/s00239-011-9435-6
- Throckmorton LH. King RCE. The phylogeny, ecology, and geography of Drosophila 1975; New York Plenum Press 421 - 469
- Markow TA, O'Grady PM. Evolutionary genetics of reproductive behavior in Drosophila: Connecting the dots. Annu Rev Genet 2005; 39:263 - 291; PMID: 16285861; http://dx.doi.org/10.1146/annurev.genet.39.073003.112454
- Grimaldi DA. A phylogenetic, revised classification of genera in the Drosophilidae (Diptera). Bull Am Mus Nat Hist 1990; 197:1 - 139
- DeSalle R. The Phylogenetic Relationships of Flies in the Family Drosophilidae Deduced from mtDNA Sequences. Mol Phylogenet Evol 1992; 1:31 - 40; PMID: 1342922; http://dx.doi.org/10.1016/10557903(92)90033-D
- Thomas RH. Phylogenetic relationships in Drosophila a conflict between molecular and morphological data. Mol Biol Evol 1993; 10:362 - 374; PMID: 8487635
- Tatarenkov A, Kwiatowski J, Skarecky D, Barrio E, Ayala FJ. On the evolution of Dopa decarboxylase (Ddc) and Drosophila systematics. J Mol Evol 1999; 48:445 - 462; PMID: 10079283; http://dx.doi.org/10.1007/PL00006489
- Kwiatowski J, Ayala FJ. Phylogeny of Drosophila and related genera: Conflict between molecular and anatomical analyses. Mol Phylogenet Evol 1999; 13:319 - 328; PMID: 10603260; http://dx.doi.org/10.1006/mpev.1999.0657
- Remsen J, O'Grady PM. Phylogeny of Drosophilinae (Diptera: Drosophilidae), with comments on combined analysis and character support. Mol Phylogenet Evol 2002; 24:249 - 264; PMID: 12144760; http://dx.doi.org/10.1016/S1055-7903(02)00226-9
- Da Lage JL, Kergoat GJ, Maczkowiak F, Silvain JF, Cariou ML, Lachaise D. A phylogeny of Drosophilidae using the Amyrel gene: questioning the Drosophila melanogaster species group boundaries. J Zoological Syst Evol Res 2007; 45:47 - 63; http://dx.doi.org/10.1111/j.1439-0469.2006.00389.x
- Russo CAM, Takezaki N, Nei M. Molecular phylogeny and divergence times of Drosophilid species. Mol Biol Evol 1995; 12:391 - 404; PMID: 7739381
- Tamura K, Subramanian S, Kumar S. Temporal patterns of fruit fly (Drosophila) evolution revealed by mutation clocks. Mol Biol Evol 2004; 21:36 - 44; PMID: 12949132; http://dx.doi.org/10.1093/molbev/msg236
- Lachaise D, Cariou ML, David JR, Lemeunier F, Tsacas L, Ashburner M. Historical biogeography of the Drosophila melanogaster species subgroup. Evol Biol 1988; 22:159 - 225
- Lachaise D, Silvain J-F. How two Afrotropical endemics made two cosmopolitan human commensals: the Drosophila melanogaster-D.simulans palaeogeographic riddle. Genetica 2004; 120:17 - 39; PMID: 15088644; http://dx.doi.org/10.1023/B:GENE.0000017627.27537.ef
- Hey J, Kliman RM. Population genetics and phylogenetics of DNA sequence variation at multiple loci within the Drosophila melanogaster species complex. Mol Biol Evol 1993; 10:804 - 822; PMID: 8355601
- Tsacas L, Lachaise D, David JR. Composition and biogeography of the Afrotropical drosophilid fauna 1981; New York Academic Press 197 - 259
- Yassin A, Araripe LO, Capy P, Da Lage JL, Klaczko LB, Maisonhaute C, et al. Grafting the molecular phylogenetic tree with morphological branches to reconstruct the evolutionary history of the genus Zaprionus (Diptera: Drosophilidae). Mol Phylogenet Evol 2008; 47:903 - 915; PMID: 18462955; http://dx.doi.org/10.1016/j.ympev.2008.01.036
- Okada T, Carson HL. The genera Phorticella Duda and Zaprionus Coquillett (Diptera, Drosophilidae) of the Oriental region and New Guinea. Kontyu 1983; 539 - 553
- Chassagnard MT, Kraaijeveld AR. The occurrence of Zaprionus sensu stricto in the palearctic region (Diptera, Drosophilidae). Ann Soc Entomol Fr 1991; 27:495 - 496
- Vilela CR. Is Zaprionus indianus Gupta, 1970 (Diptera, Drosophilidae) currently colonizing the Neotropical region?. Drosoph Inf Serv 1999; 37 - 39
- R'Kha S, Capy P, David JR. Host plant specialization in the Drosophila melanogaster species complex - a physiological, behavioral, and genetic analysis. Proc Natl Acad Sci USA 1991; 88:1835 - 1839; PMID: 1900368; http://dx.doi.org/10.1073/pnas.88.5.1835
- Roque F, Hay JD, Tidon R. Breeding sites of drosophilids (Diptera) in the Brazilian Savanna. I. Fallen fruits of Emmotum nitens (Icacinaceae), Hancornia speciosa (Apocynaceae) and Anacardium humile (Anacardiaceae). Rev Bras Entomol 2009; 53:308 - 313; http://dx.doi.org/10.1590/S0085-56262009000200016
- Bakula M. Persistence of a microbial flora during postembryogenesis of Drosophila melanogaster. J Invertebr Pathol 1969; 14:365; PMID: 4904970; http://dx.doi.org/10.1016/0022-2011(69)90163-3
- Gilbert DG. Dispersal of yeasts and bacteria by Drosophila in a temperate forest. Oecologia 1980; 46:135 - 137; http://dx.doi.org/10.1007/BF00346979
- Loreto ELS, Carareto CMA, Capy P. Revisiting horizontal transfer of transposable elements in Drosophila. Heredity 2008; 100:545 - 554; PMID: 18431403; http://dx.doi.org/10.1038/sj.hdy.6801094
- Silva JC, Kidwell MG. Horizontal transfer and selection in the evolution of P elements. Mol Biol Evol 2000; 17:1542 - 1557; PMID: 11018160
- Bowen NJ, McDonald JF. Drosophila euchromatic LTR retrotransposons are much younger than the host species in which they reside. Genome Res 2001; 11:1527 - 1540; PMID: 11544196; http://dx.doi.org/10.1101/gr.164201
- Ludwig A, Loreto ELS. Evolutionary pattern of the gtwin retrotransposon in the Drosophila melanogaster subgroup. Genetica 2007; 130:161 - 168; PMID: 16897442; http://dx.doi.org/10.1007/s10709-006-9003-y
- Ludwig A, Valente V, Loreto ELS. Multiple invasions of Errantivirus in the genus Drosophila.. Insect Mol Biol 2008; 17:113 - 124; PMID: 18353101; http://dx.doi.org/10.1111/j.1365-2583.2007.00787.x
- Bartolomé C, Bello X, Maside X. Widespread evidence for horizontal transfer of transposable elements across Drosophila genomes. Genome Biol 2009; 10:R22; PMID: 19226459; http://dx.doi.org/10.1186/gb-200910-2-r22
- Vidal NM, Ludwig A, Loreto ELS. Evolution of Tom, 297, 17.6 and rover retrotransposons in Drosophilidae species. Mol Genet Genomics 2009; 282:351 - 362; PMID: 19585148; http://dx.doi.org/10.1007/s00438009-0468-0
- Diao X, Freeling M, Lisch D. Horizontal transfer of a plant transposon. PLoS Biol 2006; 4:e5; http://dx.doi.org/10.1371/journal.pbio.0040005
- Sharp PM, Li WH. On the rate of DNA sequence evolution in Drosophila. J Mol Evol 1989; 28:398 - 402; PMID: 2501501; http://dx.doi.org/10.1007/BF02603075
- Fraser MJ, Coszczon T, Elick T, Bauser C. Precise excision of TTAA-specific lepidopteran transposons piggyBac(IFP2) and tagalong (TFP3) from the baculovirus genome in cell lines from two species of Lepidoptera. Insect Mol Biol 1996; 5:141 - 151; PMID: 8673264; http://dx.doi.org/10.1111/j.1365-2583.1996.tb00048.x
- Hotopp JCD, Clark ME, Oliveira D, Foster JM, Fischer P, Torres MC, et al. Widespread lateral gene transfer from intracellular bacteria to multicellular eukaryotes. Science 2007; 317:1753 - 1756; PMID: 17761848; http://dx.doi.org/10.1126/science.1142490
- Gilbert C, Schaack S, Pace JK, Brindley PJ, Feschotte C. A role for host-parasite interactions in the horizontal transfer of transposons across phyla. Nature 2010; 464:1347 - 1350; PMID: 20428170; http://dx.doi.org/10.1038/nature08939
- Houck MA, Clark JB, Peterson KR, Kidwell MG. Possible horizontal transfer of Drosophila genes by the mite Proctolaelaps regalis.. Science 1991; 253:1125 - 1128; PMID: 1653453; http://dx.doi.org/10.1126/science.1653453
- Yoshiyama M, Tu Z, Kainoh Y, Honda H, Shono T, Kimura K. Possible horizontal transfer of a transposable element from host to parasitoid. Mol Biol Evol 2001; 18:1952 - 1958; PMID: 11557800
- Syomin BV, Kandror KV, Semakin AB, Tsuprun VL, Stepanov AS. Presence of the gypsy (mdg4) retrotransposon in extracellular virus-like particles. FEBS Lett 1993; 323:285 - 288; PMID: 7684710; http://dx.doi.org/10.1016/0014-5793(93)81358-7
- Lachaise D, David JR, Lemeunier F, Tsacas L, Ashburner M. The reproductive relationships of Drosophila sechellia with Drosophila mauritiana, Drosophila simulans, and Drosophila melanogaster from the afrotropical region. Evolution 1986; 40:262 - 271; http://dx.doi.org/10.2307/2408806
- Solignac M, Monnerot M. Race formation, speciation, and introgression within Drosophila simulans, Drosophila mauritiana, and Drosophila sechellia inferred from mitochondrial DNA analysis. Evolution 1986; 40:531 - 539; http://dx.doi.org/10.2307/2408575
- Michalak P, Noor MAF. Association of misexpression with sterility in hybrids of Drosophila simulans and D. mauritiana. J Mol Evol 2004; 59:277 - 282; PMID: 15486701; http://dx.doi.org/10.1007/s00239-0042622-y
- Sánchez-Gracia A, Maside X, Charlesworth B. High rate of horizontal transfer of transposable elements in Drosophila. Trends Genet 2005; 21:200 - 203; PMID: 15797612; http://dx.doi.org/10.1016/j.tig.2005.02.001
- Lerat E, Burlet N, Biemont C, Vieira C. Comparative analysis of transposable elements in the melanogaster subgroup sequenced genomes. Gene 2011; 473:100 - 109; PMID: 21156200; http://dx.doi.org/10.1016/j.gene.2010.11.009
- Lynch M, Conery JS. The origins of genome complexity. Science 2003; 302:1401 - 1404; PMID: 14631042; http://dx.doi.org/10.1126/science.1089370
- Moriyama EN, Powell JR. Intraspecific nuclear DNA variation in Drosophila. Mol Biol Evol 1996; 13:261 - 277; PMID: 8583899
- Aquadro CF, Lado KM, Noon WA. The rosy region of Drosophila melanogaster and Drosophila simulans. I. Contrasting levels of naturally occurring DNA restriction map variation and divergence. Genetics 1988; 119:875 - 888; PMID: 2900794
- Nolte V, Schlotterer C. African Drosophila melanogaster and D. simulans populations have similar levels of sequence variability, suggesting comparable effective population sizes. Genetics 2008; 178:405 - 412; PMID: 18202383; http://dx.doi.org/10.1534/genetics.107.080200
- Capy P. Evolutionary biology - A plastic genome. Nature 1998;; 396:522 - 523; PMID: 9859983; http://dx.doi.org/10.1038/25007
- da Conceiçã Galego LGD, Carareto CMA. Scenario of the spread of the invasive species Zaprionus indianus Gupta, 1970 (Diptera, Drosophilidae) in Brazil. Genet Mol Biol 2010; 33:767 - 773; PMID: 21637589; http://dx.doi.org/10.1590/S1415-47572010005000080
- Kimura K, Kidwell MG. Differences in P-element population dynamics between the sibling species Drosophila melanogaster and Drosophila simulans. Genet Res 1994; 63:27 - 38; PMID: 8206365; http://dx.doi.org/10.1017/S0016672300032055
- Herédia F, Loreto ELS, Valente VLS. Complex evolution of gypsy in drosophilid species. Mol Biol Evol 2004; 21:1831 - 1842; PMID: 15175416; http://dx.doi.org/10.1093/molbev/msh183