Abstract
Autocrine and paracrine cell communication can be conveyed by multiple mediators, including membrane-associate proteins, secreted proteins and exosomes. Exosomes are 30–100 nm endosome-derived vesicles consisting in cytosolic material surrounded by a lipid bilayer containing transmembrane proteins. We have previously shown that dendritic cells (DCs) express on their surface multiple TNF superfamily ligands (TNFSFLs), by which they can induce the apoptotic demise of tumor cells as well as the activation of natural killer (NK) cells. In the present study, we demonstrate that, similar to DCs, DC-derived exosomes (DCex) express on their surface TNF, FasL and TRAIL, by which they can trigger caspase activation and apoptosis in tumor cells. We also show that DCex activate NK cells and stimulate them to secrete interferonγ (IFNγ) upon the interaction of DCex TNF with NK-cell TNF receptors. These data demonstrate that DCex can mediate essential innate immune functions that were previously ascribed to DCs.
Introduction
Coordinated communications between cells are essential for the life of multicellular organisms. Intercellular communications are mediated by transmembrane and secreted mediators/molecules that stimulate receptors of neighboring and/or distant cells, respectively. Recent studies have revealed a third pathway of communications between cells, which is based on the secretion of endosome-derived vesicles named exosomes.Citation1,Citation2 Exosomes have originally been described as cell-secreted vesicles that eliminate obsolete molecules, such as the transferrin receptors in reticulocytes.Citation3 Exosomes are nano-vesicles of 30 to 100 nm in diameter that are secreted by a variety of cells. It is believed that exosomes are produced from early endosomes by the process of inward budding and formation of multivesicular endosomes (MVE), fusion of the endosome membrane with plasma membrane, and release of the vesicles (exosomes) into extracellular microenvironment.Citation1,Citation2 Exosomes are composed of a limiting membrane, which consists in a plasma membrane-derived lipid bilayer with transmembrane molecules, and cytosolic materials enclosed by the limiting membrane. Thus, exosomes integrate transmembrane and soluble mediators of intercellular communication.
Dendritic cells (DCs) have been characterized as professional antigen-presenting cells (APCs), whose main function is to cross-prime effector T cells against specific protein or glycolipid antigens.Citation4 DCs have also been identified as essential effector cells of the innate immune system, which rapidly recognizes and eliminates microbial pathogens and abnormal cells, induce adaptive immune functions and regulate them.Citation5,Citation6 We have previously demonstrated in both humans and mice that DCs express on their plasma membrane multiple TNF superfamily ligands (TNFSFLs), including TNF, FasL and TRAIL, and employ them to efficiently induce apoptosis in virtually all types of cultured and freshly isolated tumor cells.Citation7-Citation9 DCs are also known to crosstalk with natural killer (NK) cells, prototypical effector cells of innate immunity. These cells reciprocally stimulate and regulate each other, resulting on one side in NK cell activation and on the other side DC maturation.Citation10-Citation24 In both mice and humans, this crosstalk occurs mainly through cell-to-cell contacts and largely involved DC and NK-cell transmembrane TNF.Citation25,Citation26
DCs constitutively produce exosomes.Citation27 DC-derived exosomes (DCex) contain numerous plasma membrane and cytoplasmic DC components. Several plasma membrane molecules that are relevant for the immune functions of DCs are also expressed on the DCex bounding membrane. These include class I and II major histocompatibility complex (MHC) molecules, intercellular adhesion molecule-1 (ICAM-1), integrins, and T cell co-stimulatory molecules such as CD40, CD80 and CD86.Citation27,Citation28 These molecules are correctly oriented on the outer surface of the DCex membrane and are functional. Accordingly, DCex MHC-tumor peptide complexes induce antigen-specific CD8+ and CD4+ T cells and exert antitumor activity in vivo.Citation29,Citation30 The immune effects of DCex are indirect, proceeding via host DCs.Citation30 Currently, there are no reports on the ability of DCex to directly mediate innate immune functions. In the present study, we demonstrated for the first time that DCex express on their surface transmembrane TNF, FasL and TRAIL, by which they directly mediate the apoptotic killing of tumor cells. We also showed that DCex can directly activate NK cells via TNF.
Results
Phenotype of DCex
To verify the purity and quality of DCex obtained by standard isolation protocols used in the present study, we performed morphologically and phenotypically characterized then by transmission electron microscopy (TEM) and flow cytometry, respectively. TEM showed that isolated DCex were a relatively homogenous population of typical 30 to 100 nm oval-biconcave vesicles (, left and upper right panels), composed of an electron-dense double layer limiting membrane surouding an electron-clear material (, bottom right panel). Thus, DCex were undamaged during purification. In addition, purified DCex were not contaminated with microparticles of cellular organelles or membranes. Flow cytometry analysis of DCex attached to Dynabeads demonstrated that the classical DC surface markers Class I and Class II MHC, CD80, CD86 and CD40 were expressed on DCex from both immature (iDCex) and mature (mDCex) DCs. With the exception of Class I MHC molecules, mDCex expressed these markers at higher levels than iDCex (). In line with the fact that CD14 is a marker of monocytes, macrophages and iDCs that is normally lost during the differentiation of iDCs to mDCs, mDCex but not iDCex expressed CD14.
Figure 1. Purified DCex have oval-biconcave shape, 30 to 100 nm in diameter and DC phenotype. iDC and mDC were generated by culturing linage marker-negative bone marrow cells in the presence of GM-CSF/IL4 and GM-CSF/IL4/LPS, respectively. DCex were isolated from conditioned media of these DC cultures using fractionated centrifugation and filtrations. Morphology (A) and surface markers (B) of purified DCex were examined by TEM and flow cytometry, respectively. (A) Purified iDCex either unbound or bound to anti-Class II MHC antibody-coated beads were processed for and examined using TEM. The left and the upper right panels of TEM micrographs of purified DCex show several 30–100 nm diameter oval-biconcave structures. The lower right panel is a TEM micrograph of a DCex bound to anti-Class II MHC antibody-coated beads showing a section of an oval structure having an electron-dense double layer membrane structure that surrounds an electron-clear material (indicated with the arrow), attached to amorphous bead structure. (B) Purified iDCex and mDCex were captured onto anti-Class II MHC antibody-coated beads, stained with fluorochrome-conjugated antibodies specific for the DC markers Class I MHC (αMHC I), Class II MHC (αMHC II), CD80 (αCD80), CD86 (αCD86), CD40 (αCD40) and CD14 (αCD14), as well as isotype control antibodies (Isotype), and analyzed by flow cytometry. Data are mean fluorescence intensity (MFI) of a representative experiment of 4 independent experiments performed.
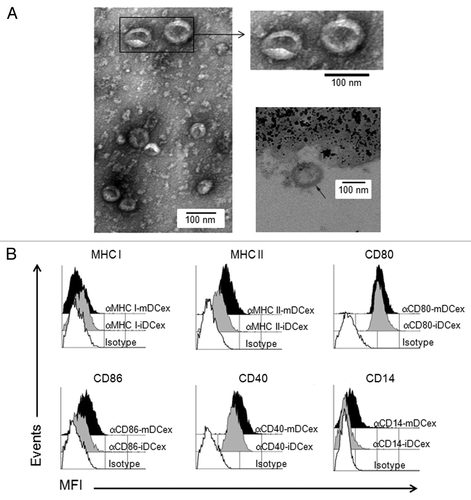
Expression of TNF superfamily ligands on DCex
We have previously demonstrated that DCs express on their plasma membrane TNF, FasL and TRAIL.Citation8 As exosomes are cell-derived nanovesicles likely constituted of plasma membrane that surrounds cytoplasmic material, we examined whether DCex also expressed TNFSFLs and how these ligands were oriented. Using ELISA, we found that both intact and lysed iDCex and mDCex contained TNF and FasL. The levels of both these TNFSFLs were significantly higher in intact mDCex than in intact iDCex, supporting the notion that that mDCex express on their surface higher levels of transmembrane TNFSFL than iDCex. We also found that DCex contained a few hundred folds higher quantities TNF than FasL. In addition, while TNF was present at similar quantities in intact and lysed DCex, FasL was present at higher quantities in lysed DCex (). These data suggest that DCex contain only transmembrane TNF, but both transmembrane and soluble FasL. To confirm the surface expression and determine the orientation of transmembrane TNFSFLs on DCex, we performed flow cytometry analysis of DCex bound onto Dynabeads fluorochrome-conjugated antibodies specific for the receptor-reactive extracellular domains of TNF, FasL and TRAIL. We found that antibodies against all these three TNFSFLs stained the surface of both iDCex and mDCex, and that staining of mDCex was more intense than that of iDCex (). Thus, transmembrane TNFSFLs appear to be expressed on DCex surface, correctly orientated and at higher quantities on mDCex than iDCex.
Figure 2. DCex express TNF superfamily ligands. (A,B) Quantification of surface-associated and total TNFSFLs of DCex. Untreated (Intact) or lysed (Lysed) iDCex and mDCex were examined by ELISA for the presence and quantity of surface-associated and total TNFSFL, respectively. TNF (A) and FasL (B) were measured using ELISA. Data are means + SD of triplicates pg/100 μg DCex. Asterisks indicate statistical significance (***, p < 0.001) of data differences between Intact iDCex vs. Intact mDCex, Lysed iDCex vs. Lysed mDCex in A; Intact iDCex vs. Intact mDCex, Intact vs. Lysed iDCex, and Intact vs Lysed mDCex in B. C. Analyses of DCex membrane-bound TNFSFLs. Purified intact iDCex and mDCex were bound to 2.8 μm Dynabeads coated with anti-class II MHC antibody, stained with fluorochrome-conjugated anti-TNF (αTNF), anti-FasL (αFasL), anti-TRAIL (αTRAIL) or isotype control (Isotype) antibodies. Data are MFI from a representative experiment of 4 experiments performed.
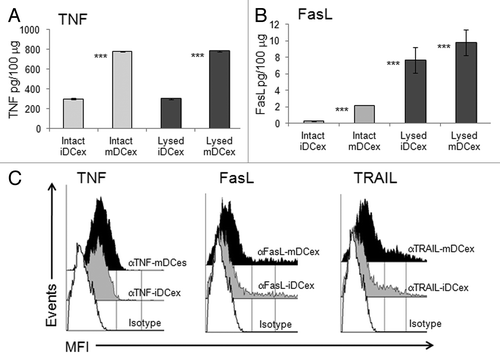
DCex kill tumor cells via TNF superfamily ligands
As DCs mediate the apoptotic death of cancer cells via TNFSFLs that are exposed on the DC surface,Citation7-Citation9 and DCex express the same ligands, we investigated whether DCex could likewise kill tumor cells via their TNFSFLs. We found that the direct exposure of B16 melanoma cells to iDCex or mDCex induced significant cell death in a dose-dependent () and time-dependent () manner. The killing of tumor cells was more pronounced with mDCex, becoming significant at 24h and increasing at 48h and 72h. mDCex could kill not only B16 melanoma cells, but also other cancer cell types, including squamous lung carcinoma KLN205 cells and colon carcinoma MC38 cells ().
Figure 3. DCex kill tumor cells. (A) iDCex and more potently mDCex kill tumor cells. Graded amounts of purified wild type (wt) mouse iDCex and mDCex were mixed with 2,500 B16 melanoma cells. The mixtures of DCex and tumor cells were incubated for 48 h, and cytotoxicity was determined using the MTT assay. (B) Time course of DCex killing of tumor cells. Fifty micrograms of wt mDCex were co-incubated with 2,500 B16 melanoma cells, and their cytotoxic activity was determined by MTT assay after 24 h, 48 h and 72 h of the incubation. (C) DCex kill not only melanoma cells but also cancer cells. 25, 50 and 100 μg of wt mDCex were co-incubated with 2,500 KLN205 squamous cell carcinoma or MC38 colon carcinoma cells for 48 h. After this incubation, cytotoxic activity of mDCex was determined by MTT assay. Data are representative of 2 experiments performed. They are % cytotoxicity means ± SD of triplicates. Statistical significance of cytotoxicity differences of mDCex vs. iDCex is indicated by asterisks (***, p < 0.001).
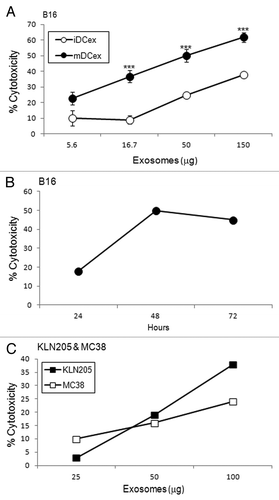
Z-VAD-fmk, a broad-spectrum caspase inhibitor, suppressed mDCex-induced cytotoxicity, measured by the apoptosis-specific DNA hypo-staining assay () as well as by a colorimetric test indirectly measuring mitochondrial activity (). TNF- and FasL-deficient mouse mDCex were significantly less cytotoxic than their wild type counterparts (), and an anti-TRAIL antibody suppressed the tumoricidal activity of wild type mouse mDCex (). These findings demonstrate that - similar to DCs - DCex mediate potent tumoricidal activity via TNFSFLs.
Figure 4. DCex induce apoptosis in tumor cells via TNF superfamily ligands. A-B. DCex induce apoptosis in tumor cells. Fifty micrograms of wt mDCex were coincubated with 2,500 B16 melanoma cells in the presence of 2% DMSO or 200 μM Z-VAD-fmk for 5 h or 48 h and tested using the apoptosis specific Hoechst DNA hypo-staining (A) and MTT (B) assays, respectively. p value represents statistically significant differences between DCex cytotoxic activities in the presence of DMSO and Z-VAD-fmk C-D. DCex of TNF- (Tnf−/−) and FasL- (Fasl−/−) deficient mice have impaired antitumor cytotoxic activity. Mature Tnf−/− DCex (C) and Fasl−/− DCex (D) were compared with mature wtDCex for their abilities to kill B16 melanoma cells as measured using 48 h MTT assay. p values represent statistically significant differences between tumoricidal activities of wtDCex vs. Tnf−/− DCex or Fasl−/− DCex. E-F. Neutralization of TRAIL inhibits DCex-mediated tumoricidal activity. Fifty micrograms of wt mDCex were coincubated with 2,500 B16 melanoma cells in the presence of 20 μg of IgG control or anti-TRAIL antibodies for 5 h or 48 h and tested using the apoptosis specific DNA hypo-staining (E) and MTT (F) assays, respectively. p values represent statisticallly significant differences between DCex cytotoxic activities in the presence of IgG control and anti-TRAIL antibodies. Data are from representative experiments of 2 experiments performed and represent % cytotoxicity means ± SD of triplicates.
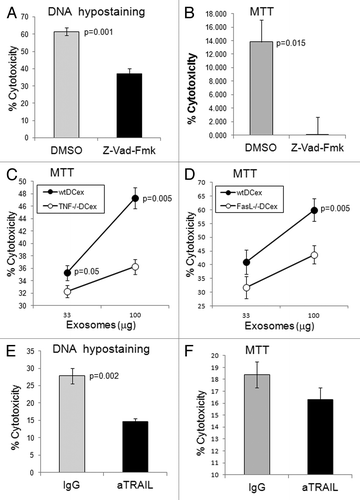
DCex activate NK cells via TNF
We have previously shown that both mouse and human DCs crosstalk with and activate NK cells via cell-to-cell contacts and transmembrane TNF.Citation9,Citation25,Citation26 As DCex express transmembrane TNF, we examined whether DCex could also activate NK cells and what would be the role of transmembrane TNF in this activation. We found that the direct exposure of NK cells to either iDCex or mDCex induced cell activation and stimulated the secretion of interferon γ (IFNγ). mDCex induced significantly higher NK cell activation than iDCex () and this manifested in a dose-dependent manner (). Of note, TNF-deficient mouse mDCex had impaired ability to activate wild type mouse NK cells (), and TNFR1- or TNFR2-deficient mouse NK cells were unable to respond to wild type mouse mDCex ().
Figure 5. DCex activate NK cells. (A) iDCex and more potently mDCex induce NK cell IFNγ secretion. One hundred μg of purified iDCex and mDCex were mixed with aNK cells, and incubated for 24 h. (B) DCex induce NK cell IFNγ secretion in a dose-dependent manner. Graded amounts of purified mDCex were mixed with aNK cells, and incubated for 24 h. After incubation, cell-free supernatants were separated and assessed for the presence and quantity of IFNγ using ELISA. Data are from a representative experiment of 2 experiments performed. They are means ± SD of triplicates IFNγ ng/0.5 × 106NK cells/mL. Asterisks indicate statistical significance of data differences: in (A), NK vs. NK+iDCex (*, p < 0.05), NK vs. NK+mDCex (***, p < 0.001), and NK+iDCex vs. NK+mDCex (***, p < 0.001); and in (B), NK (0) vs. NK+mDCex (*, p < 0.05; ***, p < 0.001).
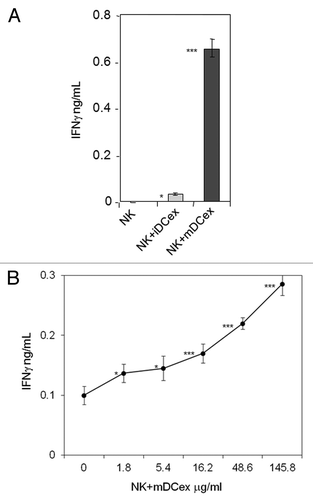
Figure 6. DCex mediate activation of NK cells via engagement of DCex TNF with NK cell TNF receptors. A. DCex of TNF-deficient mice (T−/− DCex) are unable to activate NK cells of wild type mice (wtNK). B. NK cells of TNFR1-deficient mice (R1−/− NK) have decreased responsiveness to DCex of wild type mice (wtDCex). C. NK cells of TNFR2-deficient mice (R2−/− NK) do not respond to wtDCex. wtNK cells alone, and their mixtures with 25 μg wtDCex or T−/− DCex (A); wtNK cells or R1−/− NK cells alone, and their mixtures with 25 μg of wtDCex (B); and wtNK cells or R2−/− NK cells alone, and their mixtures with 25 μg wtDCex (C) were incubated for 24 h. After incubation, cell-free supernatants were collected and tested for the presence and quantity of IFNγ using ELISA. Data are from a representative experiment of 2 experiments performed. They are means ± SD of IFN-γ ng/0.5 × 106 NK cells/mL. Asterisks indicate statistically significant differences in data from wtNK, R1−/− NK or R2−/− NK vs. wtNK+wtDCex, wtNK+wtDCex vs. wtNK+T−/−DCex; wtNK+wtDCex vs. R1−/−NK+wtDCex and R2−/−NK+wtDCex (***, p < 0.001).
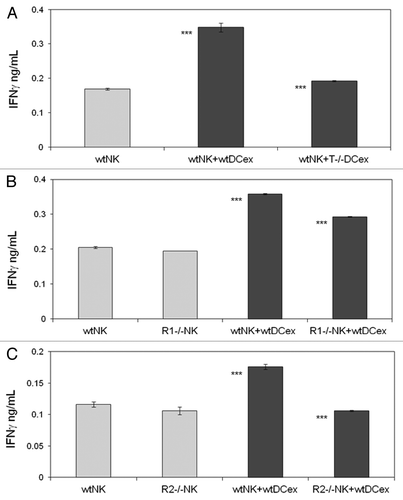
Dominant negative TNF (DNTNF), which selectively sequestrates soluble TNF, did not affect NK-cell activation and IFNγ secretion as triggered by DCex. In sharp contrast, an anti-TNF antibody, which neutralizes both the soluble and transmembrane form of TNF, significantly inhibited DCex-induced NK-cell activation. Along similar lines, anti-TNFR1 and anti-TNFR2 antibodies, which block the interaction of these receptors with TNF, significantly inhibited the activation of NK cells as induced by DCex (). These findings demonstrate that DCex, like DCs, induce the activation of NK cells, and this activity results from the engagment of DCex transmembrane TNF with NK-cell TNF receptors.
Figure 7. Transmembrane TNF, but not soluble TNF, mediates DCex-induced activation of NK cells. NK cells alone, and NK cells mixed with 20 μg DCex in the presence of isotype control antibodies (Isotype), dominant negative TNF (DNTNF), which selectively sequestrates soluble TNF, anti-TNF XT22 antibody (αTNF), which neutralizes both soluble and transmembrane TNF, anti-TNFR1 (αTNFR1) and anti-TNFR2 (αTNFR2) antibodies, which both block the TNF-binding domain of the corresponding receptors, were incubated for 24 h. Following incubation, cell-free supernatants were assessed for IFNγ using ELISA. Data are from a representative experiment of 3 experiments performed. They are means ± SD of triplicates IFNγ ng/0.5 × 106 NK cells/mL. Asterisks indicate statistically significant differences in data from of NK vs. NK+mDCex+Isotype; NK vs. NK+mDCex+DNTNF; NK+mDCex+Isotype or NK+mDCex+DNTNF vs. NK+mDCex+αTNF, NK+mDCex+αTNFR1 or NK+mDCex+αTNFR2 (**, p = 0.005; ***, p < 0.001).
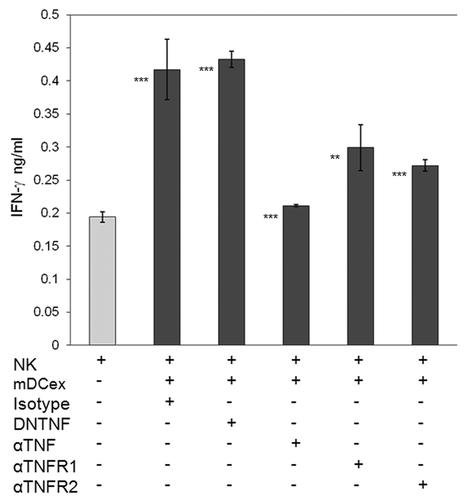
Discussion
DCs express biologically active transmembrane molecules on the plasma membrane and secrete immunoregulatory cytokines. These molecules stimulate and regulate essential innate and adaptive immune functions via juxtacrine (cell-to-cell contact) and/or paracrine/endocrine pathways, respectively. DCs also constitutively produce exosomes, which potentially carry DC transmembrane molecules and cytokines, and hence have the ability to convey DC functions via paracrine/endocrine pathway. It has been shown that DCex express on their limiting membrane functional MHC and co-stimulatory molecules. The expression of these molecules enable DCex to efficiently present antigenic peptides in the context of MHC molecules to T cells and induce adaptive immune responses, similar to parental DCs.Citation29,Citation30 This immune mechanism appears to be mediated by host DCs that acquire functional MHC-peptide complexes from DCex.Citation30 The demonstration that DCex express other biologically active DC molecules, and that DCex can directly mediate immune functions is largely missing or incomplete. Here, we demonstrate for the first time that DCex express functional transmembrane TNFSFLs and mediate via these ligands two essential DC innate immune functions, direct killing of tumor cells and activation of NK cells.
It has been previously shown that mouse iDCex express TNF, and that human cancer cell-derived exosomes express FasL.Citation28,Citation31 However, there is no published report on DCex TNF function, as well as on the DCex expression and function of FasL, TRAIL and other TNFSFLs. We show that mouse DCex, especially mDCex, express correctly oriented transmembrane TNF, FasL and TRAIL on their surface. These DCex ligands are functional, as they effectively mediate caspase-activation and apoptosis in tumor cells. The activity of mDCex is more pronounced than that of iDCex,correlating with higher expression levels of TNFSFLs on exosomes. The expression of transmembrane TNFSFLs on the DCex limiting membrane and TNFSFL-mediated tumoricidal activity of DCex mirror the previously described expression of these ligands on DC plasma membrane and their tumoricidal potential.Citation8,Citation9
In a Phase I clinical trial performed with non-small cell lung carcinoma patients who were vaccinated with autologous iDCex-tumor peptide complexes, Chaput et al. found that few of the patients who received this vaccine had increased numbers of circulating CD3-CD56+ NK cells, which showed an enhanced ability to respond to interleukin 2 (IL-2) or DC stimulation by IFNγ secretion and killing of K562 target cells.Citation27 The authors suggested that DCex may be capable of stimulating NK cells in vivo. However, no direct experimental evidence supporting this possibility and proving whether the suspected DCex-induced NK cell activation occurs directly or indirectly was provided. Using a well defined in vitro experimental model system, we show that iDCex, and more potently mDCex, directly induce the activation of NK cells, leading to increased secretion of the central Th1 cytokine IFNγ. This process is mediated by the engagement of DCex-bound TNF with NK-cell TNFR1 and TNFR2, and mimics the activity of DCs.Citation25,Citation26 However, the expression levels of transmembrane TNF and the stimulation of NK cells by DCex are several folds lower than those of parental DCs.Citation25 In addition, while mouse DCs crosstalk with NK cells by selectively engaging NK-cell TNFR2 receptors with plasma membrane-bound TNF,Citation25 DCex-attached TNF interact with NK cells by engaging both NK-cell TNFR1 and TNFR2. This may be due to differences in expression level, topography and motility of transmembrane TNF.
In summary, DCex not only express functional transmembrane MHC and co-stimulatory molecules that enable them to indirectly stimulate adaptive T cell response,Citation29,Citation30 but also express functional transmembrane TNFSFLs that enable them to directly mediate innate immune functions such as the apoptotic killing of tumor cells and the activation of NK cells. Therefore, DCs are not only capable of mediating essential innate immune functions in spatially close targets by a juxtacrine/cell-to-cell contact pathway via plasma membrane transmembrane TNFSFLs, but also in distant targets by a paracrine/endocrine pathway that is mediated by secreted DCex and their TNFSFLs. While the juxtacrine pathway might mediate innate immune functions locally, to a small number of target cells, the paracrine/endocrine pathway might extend these functions in an orchestrated way to a large number of distant target cells and thus induce systemic and proportionally amplified innate immune functions.
Materials and methods
Mice
Eight-week old wild type (WT) female C57BL/6 (H-2kCitation2/I-Ab) mice were obtained from Taconic (Germantown, NY). TNF and FasL deficient (Tnf−/− and Fasl−/−, respectively) female mice with C57BL/6 background were purchased from The Jackson Laboratory (Bar Harbor, ME). Mice were housed at the University of Pittsburgh Cancer Institute’s Association for Assessment and Accreditation of Laboratory Animal Care (AAALAC) internationally accredited animal facility. The animal studies were performed in accordance with the protocols approved by the University of Pittsburgh Institutional Animal Care and Use Committee.
Reagents
The following reagents, antibodies and kits were used in the present study: low toxic rabbit complement (Accurate Chemical and Scientific Corp., Westbury, NY); mouse recombinant GM-CSF and IL-4 (R&D Systems, Minneapolis, MN); recombinant human interleukin 2 (IL-2) (Chiron Corp., Emeryville, CA); Escherichia coli lipopolysaccharide (LPS) (Sigma Chemical, St. Louis, MO); Z-Val-Ala-Asp(OMe)-fmk [Z-VAD(OMe)-fmk, MP Biomedicals, Solon, OH]; rat anti-mouse CD4, CD8, CD45/B220 monoclonal antibodies (mAb) produced by the corresponding hybridomas obtained from American Type Culture Collection (ATCC, Rockville, MD); fluorochrome-conjugated mouse anti-mouse class I MHC molecule, mouse anti-mouse Class II MHC molecule, rat anti-mouse TNF, hamster anti-mouse FasL, rat anti-mouse TRAIL, hamster anti-mouse CD80, rat anti-mouse CD86, rat anti-mouse CD14, hamster anti-mouse CD40 and corresponding isotype control monoclonal antibodies (BD PharMingen, CA, USA); biotin-conjugated mouse anti-mouse I-Ab (BD PharMingen, CA, USA); unconjugated rat anti-mouse TNF (XT22, Pierce-Endogen, Rockford, IL), hamster anti-mouse TNFR1 and TNFR2 (BD-PharMingen); LEAFTM unconjugated rat anti-mouse TRAIL (CD253), rat anti-mouse CD16/32 and non-reactive isotype control monoclonal antibodies (BioLegend, San Diego, CA); DNTNF (XPro1595, Xencor,, Monrovia, CA); streptavidin-conjugated 2.8 μm diameter polystyrene beads (Dynal Biotech, Oslo, Norway); MACS NK-Cell Isolation Kit (Miltenyi Biotec, Auburn, CA); mouse TNF and FasL DuoSet enzyme-linked immunosorbent assay (ELISA) kits (R&D Systems), and mouse IFNγ Quantikine ELISA kit (R&D Systems).
Tumor cell lines
C57BL/6 mouse B16 melanoma and MC38 colon adenocarcinoma, and DBA/2 mouse KLN205 squamous cell carcinoma cell lines were purchased from ATCC. The cell lines were cultured in Dulbecco’s modified Eagle’s medium (DMEM), RPMI-1640 medium and Eagle’s minimum essential medium (EMEM), respectively, supplemented with 10% fetal bovine serum (FBS: Life Technologies, Long Island, NY). These tumor cells were grown adherent and utilized in experiments when their cultures were 70% confluent. The cell lines were regularly tested and were mycoplasma free.
Generation of DCs
Immature myeloid DCs (iDCs) were produced by 5-d culturing of mature cell-depleted bone marrow cells (0.1 × 106/mL) in complete cell culture medium (CM) consisted of RPMI 1640 medium supplemented with 0.1 mM nonessential amino acids, 2 mM sodium pyruvate, 1 mM L-glutamine, 100 μg/ml streptomycin, 100 U/mL penicillin, 20 mM HEPES buffer,10% FBS (Life Technologies), and 50 μM 2-mercaptoethanol (Bio-Rad, Hercules, CA); and supplemented with 15 ng/mL of recombinant mouse GM-CSF and IL-4. iDCs were 95% CD11c+CD205+/− lineage marker-, and expressed CD80, CD86, CD40, Class I and II MHC molecules at low to intermediate levels. Mature DCs (mDCs) were produced by overnight stimulation of iDCs with 1 μg/ml LPS. Tnf−/− and Fasl−/− DCs showed normal growth and expression of the costimulatory molecules.
Purification of fresh NK cells
NK1.1+CD3- cells were purified from splenocytes by negative immunoselection using MACS mouse NK cell isolation kit as recommended by the manufacturer. These cells were more than 75% NK1.1+CD3-CD69-.
Activation and expansion of NK cells
Purified fresh NK cells (0.1 × 106/mL) were suspended in CM supplemented with 6,000 IU/mL IL-2, and cultured for 6 d. In this culture, NK cells expanded 10–20 fold and were > 97% NK1.1+CD3-CD69+. They are referred as cultured IL-2-activated NK (aNK) cells.
Generation and purification of DCex
Briefly, iDCex were generated by replacing day 5 iDC culture-conditioned medium with fresh CM supplemented with GM-CSF/IL-4, and additional culturing of iDCs for 24 h. mDCex were generated by replacing day 5 iDC culture conditioned media with fresh CM supplemented with GM-CSF/IL-4 and 1 μg/mL LPS, and additional culturing of DCs for 24 h. The newly generated cell culture-conditioned media were collected from the iDC and mDC cultures. The cell culture-conditioned media were centrifuged for 10 min at 300 g and 1000 g, and filtered through 0.45 μm pore filters, to remove cells and their debris. Filtered cell culture-conditioned media were concentrated in Centricon Plus-70 Millipore filter units (Billerica, MA) by centrifugation at 1000 g for 45 min. DCex were separated from the cell culture-conditioned media by ultracentrifugation at 100,000 g (Optima LE-80K ultracentrifuge, 90-Ti rotor, Beckman Coulter) for 1 h. DCex were washed twice in PBS using Amicon Ultra-15 filter units (Millipore) and centrifugations at 1000 g for 25 min. DCex were finally resuspended in 200 μL PBS. Quantifications of the purified DCex were performed using BCA Protein Assay Kit (Thermo Scientific, Rockford, IL).
Coupling DCex to streptavidin-conjugeted beads
One mg of streptavidin conjugated 2.8 μm diameter Dynabeads was washed 3 times in ice-cold PBS containing 0.1% sodium azide (Sigma) and 1% FBS (FACS buffer). The beads were resuspended in 300 μL FACS buffer containing 7 μg of biotinylated anti-MHC class II molecule antibody. Beads and antibody were incubated with gentle mixing at room temperature for 30 min. Following incubation, the formed conjugates of antibody-streptavidin-beads were washed twice with PBS supplemented with 1% FBS. The antibody-streptavidin-bead conjugates were incubated with purified exosomes (equivalent to 10 μg of exosome protein per 280,000 beads) in a total volume of 300 μL of PBS with gentle mixing overnight at room temperature. The next day, the bead-antibody-exosome complexes were washed twice either in FACS buffer for flow cytometry analyses or with PBS for functional studies.
Analysis of DCex by TEM
The main marker of exosomes is their morphology, including oval-biconcave shape and size of 30 to 100 nm. To define their purity and quality, we examined DCex morphology using TEM. Ten μL of purified DCex were loaded on a Formvar-coated copper grid (200 mesh), and incubated for 0.5 min. Excess DCex suspension was blotted with filter paper. The grid was placed on a drop of 1% uranyl acetate solution in milli-Q H2O for 5 sec, and excess stain was removed. Exosomes were examined using a JEOL JEM 1011 (JEOL, Peabody, MA) transmission electron microscope at 80 kV.
Exosomes attached to 2.8 μm Dynabeads were pelleted at 500 g and fixed in 2.5% glutaraldehyde solution in PBS for 1 h. Pellets were washed three times in PBS then post-fixed in 1% OsO4 and 1% K3Fe(CN)6 solution for 1 h. Pellets were washed three times in PBS, and dehydrated through a graded series of 30% to 100% ethanol then infiltrated in Polybed 812 epoxy resin (Polysciences, Warrington, PA) for 1 h. Pellets were embedded in resin, cured at 37°C overnight, and hardened at 65°C for two days. Ultrathin (70 nm) sections were prepared and placed on 200 mesh copper grids, stained with 2% uranyl acetate in 50% methanol for 10 min, and 1% lead citrate for 7 min. Sections were examined using a JEOL JEM 1011 transmission electron microscope at 80 kV.
Quantification of DCex TNF superfamily ligands
TNF and FasL were quantified using intact DCex, to measure the levels of ligands on exosome outer surface, and lysed DCex, to determine the total amount of ligands both on the surface and inside of exosomes. DCex were lysed using radioimmunoprecipitation assay (RIPA) cell-lysis buffer (Upstate, Charlottesville, VA) containing EDTA-supplemented protease inhibitor cocktail (Roche Applied Science, Indianapolis, IN). The ELISAs of intact and lysed DCex were performed using ELISA kits according to the manufacture recommendations. The amounts of TNF family ligands per 100 μg of exosomal proteins were determined. Data were presented as means of triplicates ± SD of TNF and FasL pg/100 μg of exosome proteins.
Flow cytometry of DCex
DCex-bead conjugates were incubated with fluorescently tagged anti-class I MHC molecules, anti-class II MHC molecules, anti-CD80, anti-CD86, anti-CD40, anti-CD14, anti-TNF, anti-FasL, anti-TRAIL, and isotype-matched nonreactive control antibodies (20 μg/mL) at 4°C, for 1 h. After this incubation, the bead-exosome-antibody complexes were washed three times with FACS buffer and fixed with 1% paraformaldehyde solution in PBS. The bead-exosome-antibody complexes were examined using XL Epics Flow Cytometer (Beckman Coulter Ramsey, MN) and the obtained data analyzed using Beckman Coulter Expo32 Software.
MTT cytotoxicity assay
Tumoricidal activity of DCex was tested using a modified MTT cytotoxicity assay.Citation7 Tumor target cells were suspended in complete cell culture media and plated (2,500 per well) in a flat bottom 96 well plate (Corning Inc., Corning, NY). Exosomes were suspended in the same cell culture medium and plated on top of tumor cells. Control wells contained tumor cells alone. The plates were gently shaken for 1 h at room temperature, to ensure exosome-tumor cell interaction, and then incubated at 37°C, for 24 to 72 h. After this incubation, the cell culture conditioned media were removed by pipetting. One hundred μL of MTT [3-(4,5-Dimethylthiazol-2-yl)-2,5-diphenyltetrazolium bromide, Sigma] solution in RPMI (0.5 mg/mL) were added to the wells. After 4 h incubation, MTT solution was removed and 150 μL of dimethyl sulfoxide (DMSO, Sigma) was added to the wells. The plate was gently shaken for 20 min and examined on a microplate reader (Bio-Rad, Hercules, CA) using excitation and emission wavelengths of 570 nm and 490 nm, respectively. Percentages of cytotoxicity were calculated using the following formula [(control–experimental)/control] × 100, where “control” is absorbance (OD) value obtained in the wells containing tumor cells alone, and “experimental” is OD value obtained in the wells containing both tumor cells and DCex. Data were presented as means of triplicates ± SD.
DNA hypo-staining assay
B16 melanoma cells were suspended in complete cell culture media, plated (2,500 per well) in flat bottom 96 well plates and cultured for 24 h. After this culture, tumor cells were either left untreated (negative control, spontaneous apoptosis) or were exposed to UV (UV) C radiation using a germicidal lamp (UVP, Upland, CA), for 2 min (positive control, total apoptosis) or to DCex (experimental). The plates with untreated and treated tumor cells were incubated at 37°C, for 5 h. After this incubation, tumor cells were fixed with 3% paraformaldehyde for 20 min, rinsed once with PBS, and stained with Hoechst 33342 (Enzo Life Sciences, Inc., Farmingdale, NY) solution (10 μg/mL), for 1 h. The tumor cells were rinsed once with PBS and imaged using a Cellomics Arrayscan Vti (Thermo Fisher Scientific, Pittsburgh, PA) and 20x magnification. Hoechst 33342-stained nuclei were segmented and individually analyzed with Compartmental Analysis v3.5 package (Thermo Fisher Scientific). Data were further analyzed using Spotfire DecisionSite (Tibco, Palo Alto, Ca), to set a threshold and demarcate high/low Hoechst intensity in cell nuclei. The percentage of DCex-induced cytotoxicity was calculated using the following formula: % Cytotoxicity = [% Experimental (DCex-treated)-% Control (spontaneous)/% Total (UV-treated)-Control (spontaneous)] × 100. Data were presented as means of triplicates ± SD.
DCex cytotoxicity blocking experiments
In antibody-blocking experiments, DCex were preincubated with 10 μg/mL of anti-CD16/32 antibody, for 10 min, and then with 20 μg/mL of either rat non-reactive (isotype control) or rat anti-mouse TRAIL monoclonal antibody at room temperature, for 60 min. Thus pretreated exosomes were co-incubated with tumor cells at 37°C, and tested for their tumoricidal activity using cytotoxicity assays described above. In caspase-blocking experiments, 2% DMSO (control) or 200 μM Z-VAD(OMe)-fmk were cocultured with the mixture of DCex and tumor cells at 37°C, and DCex tumoricidal activity was assessed using cytotoxicity assays.
Test of NK cell activation by DCex
aNK cells were suspended in CM and plated (100,000 per well) in a round bottom 96-well plate (Corning). Purified DCex were suspended in CM and added to the wells containing aNK cells. Control wells contained aNK cells alone. To assess the role of TNF and their receptors in DCex-mediated activation of NK cells, DNTNF (Xencor) that selectively sequestrates soluble TNF, anti-TNF neutralizing antibody XT22 (Pierce-Endogen) and anti-TNFR1 and anti-TNFR2 blocking antibodies (BD-PharMingen) (20 μg/mL) were applied to the mixture of aNK cells and DCex. Control sample was a mixture of aNK cells and DCex without and with isotype control antibodies. The plates were gently shaken for 1 h at room temperature, to ensure exosome-NK cell interaction. The plates were then incubated overnight at 37°C. After incubation, IFNγ was measured in cell culture-conditioned media using IFNγ R&D ELISA kit. Data were presented as means of triplicates ± SD of IFNγ pg/0.5 × 106 NK cells/mL.
Statistics
Data were statistically evaluated using the SPSS commercial program package (version 10.0 SPSS Inc., Chicago, IL). The data are reported as means of triplicates ± SD. Statistical significance of data was assessed using two-tailed unpaired Student’s t-tests. The results were considered significantly different when p ≤ 0.05.
Disclosure of Potential Conflicts of Interest
The authors declare that they have no conflict of interest.
Acknowledgments
This study was supported by research funding from the National Institute of Health grants I-PO DE13059, RO1 DE14775, RO1 DE17150 and the University of Pittsburgh Cancer Institute and the Henry L. Hillman Foundation to N.L.V. The study used the UPCI Animal and Flow Cytometry Facilities supported by P30CA047904 award. The authors thank to Jennifer Liberatore-Tan and Michael Magee for their technical assistance. We are also obliged to David Szymkowski (Xencor, Monrovia, CA) who has generously provided us with dominant negative TNF (DNTNF, XPro1595) reagent.
References
- Février B, Raposo G. Exosomes: endosomal-derived vesicles shipping extracellular messages. Curr Opin Cell Biol 2004; 16:415 - 21; http://dx.doi.org/10.1016/j.ceb.2004.06.003; PMID: 15261674
- Stoorvogel W, Kleijmeer MJ, Geuze HJ, Raposo G. The biogenesis and functions of exosomes. Traffic 2002; 3:321 - 30; http://dx.doi.org/10.1034/j.1600-0854.2002.30502.x; PMID: 11967126
- Johnstone RM, Mathew A, Mason AB, Teng K. Exosome formation during maturation of mammalian and avian reticulocytes: evidence that exosome release is a major route for externalization of obsolete membrane proteins. J Cell Physiol 1991; 147:27 - 36; http://dx.doi.org/10.1002/jcp.1041470105; PMID: 2037622
- Steinman RM. The dendritic cell system and its role in immunogenicity. Annu Rev Immunol 1991; 9:271 - 96; http://dx.doi.org/10.1146/annurev.iy.09.040191.001415; PMID: 1910679
- King PD, Katz DR. Mechanisms of dendritic cell function. Immunol Today 1990; 11:206 - 11; http://dx.doi.org/10.1016/0167-5699(90)90084-M; PMID: 2191684
- Banchereau J, Steinman RM. Dendritic cells and the control of immunity. Nature 1998; 392:245 - 52; http://dx.doi.org/10.1038/32588; PMID: 9521319
- Janjic BM, Lu G, Pimenov A, Whiteside TL, Storkus WJ, Vujanovic NL. Innate direct anticancer effector function of human immature dendritic cells. I. Involvement of an apoptosis-inducing pathway. J Immunol 2002; 168:1823 - 30; PMID: 11823515
- Lu G, Janjic BM, Janjic J, Whiteside TL, Storkus WJ, Vujanovic NL. Innate direct anticancer effector function of human immature dendritic cells. II. Role of TNF, LT-α1β2, Fas ligand and TRAIL. J Immunol 2002; 168:1831 - 9; PMID: 11823516
- Vujanovic NL. Role of TNF superfamily ligands in innate immunity. Immunol Res 2011; 50:159 - 74; http://dx.doi.org/10.1007/s12026-011-8228-8; PMID: 21717067
- Fernandez NC, Lozier A, Flament C, Ricciardi-Castagnoli P, Bellet D, Suter M, et al. Dendritic cells directly trigger NK cell functions: cross-talk relevant in innate anti-tumor immune responses in vivo. Nat Med 1999; 5:405 - 11; http://dx.doi.org/10.1038/7403; PMID: 10202929
- Goodier MR, Londei M. Lipopolysaccharide stimulates the proliferation of human CD56+CD3- NK cells: a regulatory role of monocytes and IL-10. J Immunol 2000; 165:139 - 47; PMID: 10861046
- Osada T, Nagawa H, Kitayama J, Tsuno NH, Ishihara S, Takamizawa M, et al. Peripheral blood dendritic cells, but not monocyte-derived dendritic cells, can augment human NK cell function. Cell Immunol 2001; 213:14 - 23; http://dx.doi.org/10.1006/cimm.2001.1858; PMID: 11747352
- Yu Y, Hagihara M, Ando K, Gansuvd B, Matsuzawa H, Tsuchiya T, et al. Enhancement of human cord blood CD34+ cell-derived NK cell cytotoxicity by dendritic cells. J Immunol 2001; 166:1590 - 600; PMID: 11160200
- Gerosa F, Baldani-Guerra B, Nisii C, Marchesini V, Carra G, Trinchieri G. Reciprocal activating interaction between natural killer cells and dendritic cells. J Exp Med 2002; 195:327 - 33; http://dx.doi.org/10.1084/jem.20010938; PMID: 11828007
- Piccioli D, Sbrana S, Melandri E, Valiante NM. Contact-dependent stimulation and inhibition of dendritic cells by natural killer cells. J Exp Med 2002; 195:335 - 41; http://dx.doi.org/10.1084/jem.20010934; PMID: 11828008
- Ferlazzo G, Tsang ML, Moretta L, Melioli G, Steinman RM, Münz C. Human dendritic cells activate resting natural killer (NK) cells and are recognized via the NKp30 receptor by activated NK cells. J Exp Med 2002; 195:343 - 51; http://dx.doi.org/10.1084/jem.20011149; PMID: 11828009
- Poggi A, Carosio R, Spaggiari GM, Fortis C, Tambussi G, Dell’Antonio G, et al. NK cell activation by dendritic cells is dependent on LFA-1-mediated induction of calcium-calmodulin kinase II: inhibition by HIV-1 Tat C-terminal domain. J Immunol 2002; 168:95 - 101; PMID: 11751951
- Jinushi M, Takehara T, Kanto T, Tatsumi T, Groh V, Spies T, et al. Critical role of MHC class I-related chain A and B expression on IFN-alpha-stimulated dendritic cells in NK cell activation: impairment in chronic hepatitis C virus infection. J Immunol 2003; 170:1249 - 56; PMID: 12538683
- Mailliard RB, Son YI, Redlinger R, Coates PT, Giermasz A, Morel PA, et al. Dendritic cells mediate NK cell help for Th1 and CTL responses: two-signal requirement for the induction of NK cell helper function. J Immunol 2003; 171:2366 - 73; PMID: 12928383
- Andrews DM, Scalzo AA, Yokoyama WM, Smyth MJ, Degli-Esposti MA. Functional interactions between dendritic cells and NK cells during viral infection. Nat Immunol 2003; 4:175 - 81; http://dx.doi.org/10.1038/ni880; PMID: 12496964
- Moretta A, Marcenaro E, Sivori S, Della Chiesa M, Vitale M, Moretta L. Early liaisons between cells of the innate immune system in inflamed peripheral tissues. Trends Immunol 2005; 26:668 - 75; http://dx.doi.org/10.1016/j.it.2005.09.008; PMID: 16198147
- Ferlazzo G, Münz C. NK cell compartments and their activation by dendritic cells. J Immunol 2004; 172:1333 - 9; PMID: 14734707
- Moretta A. Natural killer cells and dendritic cells: rendezvous in abused tissues. Nat Rev Immunol 2002; 2:957 - 64; http://dx.doi.org/10.1038/nri956; PMID: 12461568
- Kalinski P, Mailliard RB, Giermasz A, Zeh HJ, Basse P, Bartlett DL, et al. Natural killer-dendritic cell cross-talk in cancer immunotherapy. Expert Opin Biol Ther 2005; 5:1303 - 15; http://dx.doi.org/10.1517/14712598.5.10.1303; PMID: 16197336
- Xu J, Chakrabarti AK, Tan JL, Ge L, Gambotto A, Vujanovic NL. Essential role of the TNF-TNFR2 cognate interaction in mouse dendritic cell-natural killer cell crosstalk. Blood 2007; 109:3333 - 41; http://dx.doi.org/10.1182/blood-2006-06-026385; PMID: 17164346
- Vujanovic L, Szymkowski DE, Alber S, Watkins SC, Vujanovic NL, Butterfield LH. Virally infected and matured human dendritic cells activate natural killer cells via cooperative activity of plasma membrane-bound TNF and IL-15. Blood 2010; 116:575 - 83; http://dx.doi.org/10.1182/blood-2009-08-240325; PMID: 20430958
- Chaput N, Flament C, Viaud S, Taieb J, Roux S, Spatz A, et al. Dendritic cell derived-exosomes: biology and clinical implementations. J Leukoc Biol 2006; 80:471 - 8; http://dx.doi.org/10.1189/jlb.0206094; PMID: 16809645
- Morelli AE, Larregina AT, Shufesky WJ, Sullivan MLG, Stolz DB, Papworth GD, et al. Endocytosis, intracellular sorting, and processing of exosomes by dendritic cells. Blood 2004; 104:3257 - 66; http://dx.doi.org/10.1182/blood-2004-03-0824; PMID: 15284116
- Zitvogel L, Regnault A, Lozier A, Wolfers J, Flament C, Tenza D, et al. Eradication of established murine tumors using a novel cell-free vaccine: dendritic cell-derived exosomes. Nat Med 1998; 4:594 - 600; http://dx.doi.org/10.1038/nm0598-594; PMID: 9585234
- Therry C, Duban L, Segura E, Veron P, Lantz O, Amigorena S. Indirect activation of naïve CD4+ T cells by dendritic cell-derived exosomes. Naure Immunol 2002; 3:1156 - 62; http://dx.doi.org/10.1038/ni854
- Wieckowski E, Whiteside TL. Human tumor-derived vs dendritic cell-derived exosomes have distinct biologic roles and molecular profiles. Immunol Res 2006; 36:247 - 54; http://dx.doi.org/10.1385/IR:36:1:247; PMID: 17337785