Abstract
Despite its efficacy and widespread use, radiation therapy is often associated with local or distal tumor relapse. We have recently found that CD8+ T cells and their cytokines are essential for maintaining irradiated neoplasms under control. In line with this notion, enhancing T-cell functions by means of immune checkpoint inhibitors can tilt the balance toward tumor rejection.
Keywords: :
Approximately 50% of cancer patients worldwide receive radiation therapy. In many of these patients, the malignancy relapses after a long, yet variable, disease-free or progression-free period. In breast and prostate cancer patients, over 50% of local recurrences occur after 5 y following radiotherapy.Citation1,Citation2 The mechanisms whereby radiotherapy induce persistent objective responses as well as those that underpin subsequent therapeutic failures remain poorly understood. Classically, delayed relapses have been explained as the combined function of initial tumor cell death, the number of malignant cell clones surviving therapy, and the rate of repopulation. However, disease stabilization might equally well reflect an intrinsic cellular dormancy, a failure in angiogenesis, or an equilibrium between cell proliferation and death.
To study the mechanisms underpinning radiotherapy-induced disease stabilization, we established a murine model that could recapitulate the diversity of tumor responses observed in clinical settings. Importantly, in this model a fraction of tumors responded to radiation with a period of dormancy/stabilization.Citation3 Wild-type BALB/c mice were challenged with TUBO neoplastic cells, which are derived from a breast carcinoma spontaneously developed by an ERBB2-overexpressing (BALB-neuT) transgenic mouse. Established tumors were treated then with single-dose (15 Gy) local irradiation. Irradiated tumors exhibited a spectrum of responses encompassing complete unresponsiveness, partial responsiveness (with delayed malignant growth), and prolonged disease stabilization. Of note, later on, stable tumors either relapsed or were completely rejected. In this setting, we investigated whether host immune responses contribute to radiotherapy-induced disease stabilization. TUBO tumors growing in severe combined immunodeficient (SCID) hosts could not be controlled by single-dose local irradiation, raising the possibility that host adaptive immunity provides a critical contribution to the stabilization of tumor growth. When we repeated the same experiments in tolerized hosts (i.e., Balb-neuT transgenic mice), we could evoke disease stabilization, but a high radiation dose (30 Gy) was required, lending further support to the role for tumor-specific immune responses in this setting.
We next investigated the mechanisms potentially underlying the differential responses of TUBO tumors in wild-type BALB/c mice by examining the intrinsic radiosensitivity of these lesions. To this aim, irradiated tumors were classified into responsive or irresponsive based on their behavior in the first week after treatment. Single-cell suspensions were then generated from tumors collected 7–8 d post-irradiation and plated. Malignant cells were further irradiated and subjected to clonogenic assays to determine whether variations in their radiosensitivity could explain the responses of tumors subjected to radiotherapy in vivo. We discovered that the differential response of TUBO tumors to radiotherapy in vivo is not due to variations in the radiosensitivity of malignant cells. Rather, animals exhibiting objective responses or disease stabilization upon radiation therapy mounted more robust tumor-specific T-cell responses compared to animals bearing irresponsive tumors, demonstrating a correlation between the antineoplastic effects of radiotherapy and the intensity of host antitumor immune responses.
We also determined that tumor-specific immune responses vary in intensity thought the period of disease stabilization. Cancer-specific T-cell responses in tumor-draining lymph nodes were most intense 8 d post-irradiation while they were significantly reduced in stabilized tumors 3–5 wk post-irradiation. Conversely, the expression levels of programmed cell death ligand 1 (PD-L1) and tumor necrosis factor α (TNFα) were higher in tumors that had gone through 3–5 wk of stabilization than in tumors collected at earlier time points. We hypothesized that radiotherapy-induced disease stabilization relies on the development of a T-cell response in the tumor microenvironment that is sufficient to maintain a temporary equilibrium between the proliferation and (T cell-mediated) death of malignant cells. This equilibrium is eventually broken along with the upregulation of immunosuppressive molecules like PD-L1 in the tumor microenvironment, which is paralleled by the waning of T-cell responses. The neutralization of PD-L1 in tumors stabilized by radiotherapy was sufficient to rescue T-cell function and to drive complete tumor rejection. Our study not only reveals the essential role of T cells in the antineoplastic effects of radiation therapy, but also elucidates key molecular pathways underpinning radiotherapy-induced disease stabilization.
We have previously demonstrated that components of the host immune systems, in particular T cells, play an essential role in the tumor response to local radiotherapy.Citation4,Citation5 Thereafter, we have begun to elucidate some of the signaling pathways and soluble mediators that regulate radiation-induced innate and adaptive immune responses, such as Type I interferon (IFN). We now suggest that disease stabilization as triggered by radiotherapy represents an equilibrium between an enhanced killing of malignant cells by effector T lymphocytes and tumor cell proliferation (). In addition, we demonstrate that the immunosuppressive tumor microenvironment critically contributes to such an equilibrium by partially attenuating T-cell responses. Thus, shortly after ablative radiotherapy, a strong cytotoxic T-cell response rapidly develops. However, regulatory cells are recruited to the tumor microenvironment and secrete high levels of immunosuppressive mediators. Understanding the evolution of this process will inform the design of novel therapeutic interventions to complete eradicate irradiated neoplastic lesions.
Figure 1. Shifting the equilibrium between the proliferation and T lymphocyte-mediated killing of malignant cells in radiation-stabilized tumors. Within irradiated tumors, an equilibrium is established between proliferating cancer cells and the cytotoxic activity of tumor-specific T lymphocytes. When the equilibrium is compromised by alterations that affect the cytotoxic potential of T cells, neoplastic lesions relapse. Conversely, the neutralization of immunosuppressive molecules such as programmed cell death ligand 1 (PD-L1) tilts the balance toward tumor rejection.
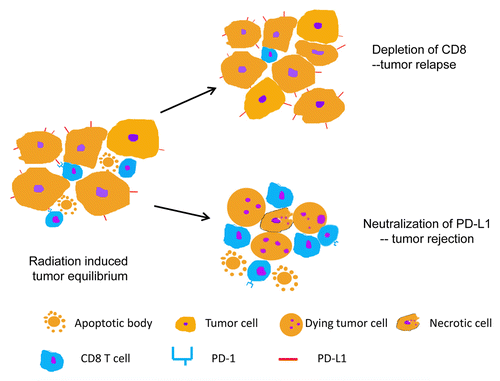
Recent studies point to an interesting role for T cells and IFN in the stabilization of carcinogen-induced murine tumors.Citation5-Citation7 These works suggest that emerging tumors are immunologically selected, i.e., they reflect the expansion of malignant cell clones that have lost major antigenic determinants (antigen-loss variants) or are resistant to cytotoxic immune effectors. Antigen-specific T-cell responses can be detected in patients bearing neoplasms that continue to express the same antigen, demonstrating that established lesions can escape immune attacks through mechanisms other than antigen loss. Among these mechanisms, a prominent role is played by the establishment of an immunosuppressive tumor microenvironment, based on the recruitment of regulatory cells or/and the overexpression of T cell-inhibitory molecules like PD-L1. Understanding the precise timing and function of these immunosuppressive factors will open a therapeutic window through which immunomodulatory agents can be applied to efficiently prevent disease relapse.
Disclosure of Potential Conflicts of Interest
Drs Fu and Weichselbaum currently receive grant funding from Bristol Myers Squibb, and Dr Weichselbaum received a one-time consulting fee from Bristol Myers Squibb.
References
- Darby S, McGale P, Correa C, Taylor C, Arriagada R, Clarke M, et al, Early Breast Cancer Trialists’ Collaborative Group (EBCTCG). Effect of radiotherapy after breast-conserving surgery on 10-year recurrence and 15-year breast cancer death: meta-analysis of individual patient data for 10,801 women in 17 randomised trials. Lancet 2011; 378:1707 - 16; http://dx.doi.org/10.1016/S0140-6736(11)61629-2; PMID: 22019144
- Pilepich MV, Winter K, Lawton CA, Krisch RE, Wolkov HB, Movsas B, et al. Androgen suppression adjuvant to definitive radiotherapy in prostate carcinoma--long-term results of phase III RTOG 85-31. Int J Radiat Oncol Biol Phys 2005; 61:1285 - 90; http://dx.doi.org/10.1016/j.ijrobp.2004.08.047; PMID: 15817329
- Liang H, Deng L, Chmura S, Burnette B, Liadis N, Darga T, et al. Radiation-induced equilibrium is a balance between tumor cell proliferation and T cell-mediated killing.. J Immunol 2013; 190:5874 - 81; http://dx.doi.org/10.4049/jimmunol.1202612; PMID: 23630355
- Lee Y, Auh SL, Wang Y, Burnette B, Wang Y, Meng Y, et al. Therapeutic effects of ablative radiation on local tumor require CD8+ T cells: changing strategies for cancer treatment. Blood 2009; 114:589 - 95; http://dx.doi.org/10.1182/blood-2009-02-206870; PMID: 19349616
- Burnette BC, Liang H, Lee Y, Chlewicki L, Khodarev NN, Weichselbaum RR, et al. The efficacy of radiotherapy relies upon induction of type i interferon-dependent innate and adaptive immunity. Cancer Res 2011; 71:2488 - 96; http://dx.doi.org/10.1158/0008-5472.CAN-10-2820; PMID: 21300764
- Dunn GP, Koebel CM, Schreiber RD. Interferons, immunity and cancer immunoediting. Nat Rev Immunol 2006; 6:836 - 48; http://dx.doi.org/10.1038/nri1961; PMID: 17063185
- Koebel CM, Vermi W, Swann JB, Zerafa N, Rodig SJ, Old LJ, et al. Adaptive immunity maintains occult cancer in an equilibrium state. Nature 2007; 450:903 - 7; http://dx.doi.org/10.1038/nature06309; PMID: 18026089
- Schreiber RD, Old LJ, Smyth MJ. Cancer immunoediting: integrating immunity’s roles in cancer suppression and promotion. Science 2011; 331:1565 - 70; http://dx.doi.org/10.1126/science.1203486; PMID: 21436444