Abstract
Macrophages constitute a dominant fraction of the population of immune cells that infiltrate developing tumors. Recruited by tumor-derived signals, tumor-infiltrating macrophages are key orchestrators of a microenvironment that supports tumor progression. However, the phenotype of macrophages is pliable and, if instructed properly, macrophages can mediate robust antitumor functions through their ability to eliminate malignant cells, inhibit angiogenesis, and deplete fibrosis. While much effort has focused on strategies to block the tumor-supporting activity of macrophages, emerging approaches designed to instruct macrophages with antitumor properties are demonstrating promise and may offer a novel strategy for cancer immunotherapy.
Introduction
Macrophages are key determinants of the biology of developing tumors. The impact of macrophages on tumor biology is largely instructed by a dynamic network of signals present within their surrounding microenvironment. These signals produce a heterogeneous population of macrophages which are commonly described based on their similarity to an M1 (classically activated) or M2 (alternatively activated) phenotype. M1 macrophages are characterized by an interleukin (IL)-12highIL-10low cytokine production profile and are activated by interferon (IFN)γ, toll-like receptor (TLR) ligands, nucleotide-binding oligomerization domain (NOD)-specific agonists, and pro-inflammatory cytokines such as tumor necrosis factor α (TNFα). Conversely, M2 macrophages are associated with a IL-12lowIL-10high cytokine production profile and are induced by stimulation with IL-4 and IL-13.Citation1 M1 and M2 macrophages represent the extremes of a spectrum of macrophage phenotypes that can be observed within tumors.
Within most solid malignancies, macrophages are polarized by the tumor microenvironment toward an M2 phenotype.Citation2 These macrophages, in turn, foster a microenvironment that supports many of the hallmarks of cancer that have been enumerated by Hanahan and Weinberg. Namely, macrophages can promote tumor angiogenesis, provide growth factors that sustain the proliferation and survival of malignant cells, facilitate invasion and metastasis, and protect developing tumors from the pressures imposed by adaptive immunosurveillance.Citation3 In most solid malignancies, an increased density of tumor-infiltrating macrophages portends a poor prognosis.Citation4 Further, macrophages are fundamental to chronic inflammatory processes that are often associated with malignancy.Citation5 Thus, macrophages play a critical role in defining tumor biology.
The identification of macrophages as significant supporters of tumor progression has generated interest in the identification of strategies to block macrophage infiltration into tumor tissue, to suppress the tumor-promoting functions of macrophages, or to selectively deplete macrophages.Citation6 However, macrophage biology is pliable such that under the appropriate conditions, macrophages can acquire potent antitumor properties. As such, shifting the phenotype of tumor-infiltrating macrophages toward an M1 phenotype has been shown to inhibit tumor progression in preclinical and clinical studies. To this end, macrophages can be polarized to eliminate tumor cells,Citation7-Citation10 to inhibit tumor-induced angiogenesis,Citation11,Citation12 and to deplete tumor-associated stromal fibrosis.Citation7 These observations provide the basis for studies investigating strategies that exploit the antitumor potential of macrophages. Here, we review recent advances on the preclinical and clinical evaluation of therapeutic approaches designed to “educate” macrophages with tumor-suppressing properties and to exploit their tropism for malignant lesions.
Tumor Biology
It has long been recognized that microbial products (e.g., TLR ligands and NOD specific agonists) as well as pro-inflammatory cytokines (e.g., IFNγ and TNFα) can induce macrophages with antitumor properties. Liposomal encapsulation has been explored as a means to specifically deliver these activating signals to macrophages in vivo. For example, systemic administration of liposomes containing NOD2 agonists (e.g., muramyldipeptide) was found to provide alveolar macrophages with tumoricidal properties capable of eradicating established spontaneous pulmonary and lymph node metastases.Citation8 In a Phase I clinical trial, the intravenous delivery of liposomes encapsulated with muramyl tripeptide phosphatidylethanolamine induced circulating monocytes with tumoricidal properties.Citation13 In a randomized phase III study, this approach in combination with chemotherapy was found to improve the overall survival of patients with osteosarcoma.Citation14 These clinical data demonstrate the potential benefit that can be achieved by selectively activating macrophages with antitumor properties in vivo.
Macrophages are actively recruited to neoplastic lesions by chemokines that are produced by both malignant and non-malignant cells within the tumor microenvironment. The phenotype of tumor-infiltrating macrophages depends on signals received within the blood stream (prior to infiltration), as well as on cues provided by the tumor microenvironment. To polarize tumor-infiltrating macrophages with antitumor properties, activation signals can be delivered either locally or systemically. For example, the intravesical administration of bacillus Calmette-Guérin (an attenuated strain of Mycobacterium bovis) for the treatment of bladder carcinoma can activate macrophages via TLR2 with pro-inflammatory and tumoricidal properties.Citation15,Citation16 In addition, the systemic delivery of an agonist monoclonal antibody (mAb) specific for CD40, a member of the TNF receptor superfamily that is expressed on macrophages as well as on many other hematopoietic and non-hematopoietic cells, has demonstrated potent macrophage-dependent antitumor activity in a clinically relevant mouse model of pancreatic ductal adenocarcinoma (PDA) and in patients with advanced PDA. In these studies, the administration of CD40-targeting mAbs was observed to induce a rapid systemic inflammatory response marked by high levels of IFNγ and TNFα, which may have sculpted tumor-infiltrating macrophages with tumoricidal properties.Citation7 Similarly, the systemic administration of Listeria monocytogenes, a gram-positive facultative intracellular bacterium, has been shown to exert antitumor activity in preclinical and clinical studies.Citation17-Citation19 This antitumor activity produced by the administration of Listeria may be due, at least in part, to its ability to activate macrophages with tumoricidal properties.Citation20 Thus, tumor-infiltrating macrophages can be polarized with an antitumor phenotype.
Macrophages have been shown to eliminate malignant cells through the production of soluble factors (e.g., nitric oxide and TNFα) that can induce tumor cell apoptosis.Citation21-Citation25 Macrophages can also eliminate cancer tumor cells through phagocytosis, based on their recognition of “eat-me” molecules present on tumor cells (). For example, phosphatidylserine, which is commonly expressed on the surface of exosomes and apoptotic cells,Citation26 delivers a robust “eat-me” signal to macrophages. Phosphatidylserine can also be expressed on the surface of viable cancer cells, although this does not appear to be sufficient to induce phagocytosis.Citation27 Nonetheless, macrophages can recognize and engulf viable tumor cells. This can occur through the interaction between the low density lipoprotein (LDL) receptor-related protein (LRP) on macrophages and calreticulin exposed on the surface of tumor cells.Citation28,Citation29 Calreticulin is a chaperone that is involved in the homeostatic control of cytosolic and reticular Ca2+ levels, and is commonly overexpressed on the surface of tumor cells.Citation28 Macrophages can also clear viable antibody-coatedtumor cells based on their expression of activating Fc receptors.Citation10,Citation30 Thus, macrophages are armed with multiple strategies for recognizing and eliminating tumor cells.
Figure 1. Macrophage immunosurveillance in cancer is regulated by a balance of pro- (“eat-me”) and anti- (“don't eat-me”) phagocytic signals presented by cancer cells. CD47 is a “don't eat-me” molecule expressed on cancer cells which interacts with signal regulatory protein α (SIRPα) on macrophages to inhibit pro-phagocytic signals received by (1) the engagement of Fc receptors with antibodies (Ab) bound to antigens (Ag) expressed on the surface of cancer cells; and (2) the interaction between LDL-receptor related protein (LRP) and calreticulin expressed on the surface of cancer cells.
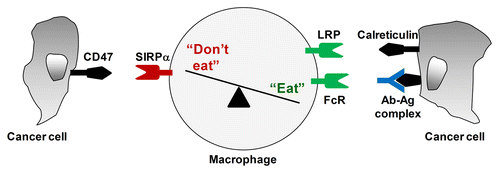
Pro-phagocytic signals encountered by macrophages are balanced by “don't eat-me” signals, which allow cancer cells to evade engulfment by macrophages (). CD47 is an integrin-associated protein that is expressed on the surface of normal cells and interacts with signal regulatory protein α (SIRPα) on macrophages to inhibit phagocytosis.Citation31 In essence, CD47 is a marker of “self” that is recognized by the innate immune system. For example, CD47-SIRPα interactions prevent macrophages from clearing healthy red blood cells.Citation32 Similarly, CD47 is upregulated on circulating hematopoietic stem cells in response to mobilizing cytokines and inflammatory stimuli in order to allow these cells to avoid elimination by macrophages.Citation33
To evade recognition by macrophages, tumor cells also express increased amounts of CD47 on their surface. This was first observed in myeloid leukemia, a setting in which CD47 is commonly overexpressed and correlates with increased pathogenicity.Citation33,Citation34 Similar findings have now been reported for many solid tumors.Citation9 This suggest that the interaction between CD47 on malignant cells and SIRPα on macrophages is a critical determinant of the outcome of innate cancer immunosurveillance. Blocking this interaction has been shown to restore macrophage-dependent Fc gamma receptor-mediated phagocytosis.Citation10 In addition, CD47-SIRPα can control calreticulin-LRP mediated phagocytosis.Citation28,Citation29 However, because CD47 allows for distinguishing self from non-self, strategies that interfere with CD47-SIRPα interactions may be complicated by anemia as a result of the macrophage-dependent clearance of red blood cells, as demonstrated in preclinical studies. However, the limitations observed with CD47-blocking antibodies can be circumvented using SIRPα variants engineered to exhibit high affinity for CD47. In xenograft tumor models, the systemic administration of such SIRPα variants enhances the recognition and phagocytosis of cancer cells by macrophages, hence prolonging the overall survival of tumor-bearing mice when used in combination with monoclonal antibodies.Citation10 These observations suggest that the innate immunosurveillance mediated by macrophages is regulated by a balance between “eat-me” and “don't eat-me” signals present on the surface of tumor cells.
Angiogenesis
Macrophages are important orchestrators of angiogenesis within the tumor microenvironment. Their presence within human tumors correlates with microvessel density,Citation35,Citation36 and in preclinical models, macrophages are observed to infiltrate premalignant lesions just prior to an “angiogenic switch,” in which the necessary pro-angiogenic signals are established to drive the transition to malignancy.Citation37 Moreover, the elimination of macrophages is associated with a reduction in vascular density.Citation37 Thus, macrophages can be instructed by developing tumors to promote angiogenesis.
Hypoxia is a common feature of solid malignancies. Within the tumor microenvironment, tumor-associated macrophages respond to hypoxia by producing cytokines including IL-1β, which can drive the infiltration of macrophages into neoplastic lesions in an IL-1R dependent manner. This is important for the subsequent recruitment of endothelial cells from neighboring tissues, which in turn can provide macrophages with pro-angiogenic properties.Citation38 Endothelial cells generate an instructive niche that serves to differentiate and polarize macrophages. For example, TIE2+ monocytes, which express the angiopoietin (ANG) tyrosine kinase receptor TEK (best known as TIE2), are a subpopulation of peripheral blood monocytes that are recruited by tumors to promote angiogenesis.Citation39 In response to ANG2 produced by activated endothelial cells, TIE2+ monocytes acquire a pro-angiogenic phenotype marked by a decreased capacity to produce IL-12 and TNFα.Citation40,Citation41 However, in the presence of anti-angiogenic therapeutics, which normalize the tumor vasculature, tumor-infiltrating macrophages can be reprogrammed toward an M1 phenotype.Citation42 These findings suggest the importance of the cross-talk between endothelial cells and macrophages in defining both macrophage phenotype and angiogenesis within the tumor microenvironment.
Macrophages are important contributors to a balance of pro- and anti-angiogenic signals that define the extent of angiogenesis observed within tumors (). Tumor-associated macrophages responding to hypoxia can release matrix metalloproteinase (MMP)9, which mobilizes vascular endothelial growth factor (VEGF), produced by both malignant and non-malignant cells, to stimulate angiogenesis by inducing the proliferation and survival of endothelial cells.Citation43 In contrast, in the presence of hypoxia, tumor-derived factors such as granulocyte macrophage colony-stimulating factor (GM-CSF) have been shown to stimulate macrophages to secrete high levels of a soluble form of the VEGF receptor 1 (VEGFR1) in a hypoxia-inducible factor (HIF)2α-dependent manner. Soluble VEGFR1 neutralizes VEGF and hence, suppresses angiogenesis. In this regard, GM-CSF can inhibit breast cancer growth and metastasis by invoking an anti-angiogenic program in tumor-associated macrophages. This effect was observed to depend on soluble VEGFR1 produced by macrophages in response to GM-CSF.Citation44 Stabilization of HIF-2α has also been found to induce the secretion of soluble VEGFR1 by tumor-associated macrophages, leading to decreased tumor growth in a murine melanoma model.Citation12 Thus, macrophages can provide both pro- and anti-angiogenic signals.
Figure 2. Macrophages can shape the balance of pro- and anti-angiogenic signals within the tumor microenvironment. Tumor-infiltrating macrophages responding to pro-angiogenic signals such as interleukin (IL)-1β, IL-4, angiopoietin 2 (ANG2), and hypoxia acquire a pro-angiogenic phenotype and secrete vascular endothelial growth factor (VEGF), as well as matrix metalloproteinase 9 (MMP9), which mobilizes VEGF for the activation of endothelial cells. In contrast, macrophages responding to anti-angiogenic signals such as interferon γ (IFNγ) and granulocyte macrophage colony-stimulating factor (GM-CSF) acquire an anti-angiogenic phenotype and produce IL-12 as well as a soluble variant of the VEGF receptor 1 (sVEGFR1), which blocks VEGF activity.
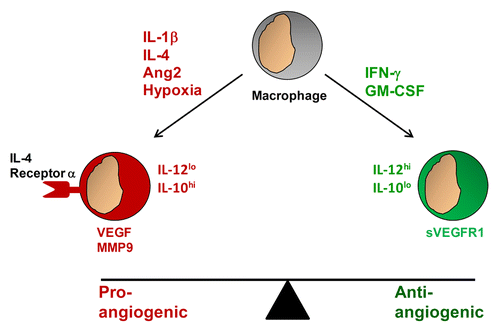
Disruption of pro-angiogenic signals can reprogram macrophages with anti-angiogenic properties. For example, IL-4 is a cytokine that polarizes macrophages toward an M2 phenotype. Blocking IL-4 signaling with antibodies specific for IL-4 receptor α has been shown to reprogram macrophages toward an M1 phenotype, leading to vascular normalization.Citation11 Similarly, it has been demonstrated that TLR4 signaling in the absence of IFNγ activates the mammalian target of rapamycin (mTOR) signaling pathway in macrophages, leading to increased secretion of IL-10 and limited production of IL-12. When mTOR signaling is inhibited with rapamycin, the response of macrophages to TLR4 signaling is shifted toward an M1 phenotype with an increase in macrophage production of IL-12 and a decrease in the release of IL-10. In a xenograft model of hepatocarcinoma, rapamycin was found to inhibit tumor growth and angiogenesis in a macrophage-dependent manner.Citation45 These findings suggest that disrupting key signaling pathways that define macrophage phenotype can shift the behavior of macrophages from pro- to anti-angiogenic.
The production of IL-12 by macrophages is an important regulator of tumor angiogenesis.Citation46 The systemic or local administration of IL-12 to tumor-bearing mice can modulate the function of tumor capillaries leading to ischemic-hemorrhagic necrosis. However, IL-12 does not directly alter endothelial cell function but rather appears to activate lymphocytes to produce soluble factors that can arrest the growth of endothelial cells and inhibit angiogenesis.Citation47,Citation48 Guiducci et al. showed that combining CpG oligodeoxynucleotides (a TLR9 ligand) with an antibody specific for the IL-10 receptor shifted tumor-infiltrating macrophages from an M2 to M1 phenotype, resulting in increased production of IL-12 and a rapid debulking of large tumors that was associated with a diffuse hemorrhagic necrosis.Citation49 These findings demonstrate that macrophages, when properly instructed, can mediate potent antitumor activity by targeting the tumor-associated vasculature.
Tumor-Associated Fibrosis
Macrophages are important mediators of wound healing and repair. In wound healing studies, macrophages are observed to regulate angiogenesis as well as the deposition of collagen by myofibroblasts.Citation50 During the early phases of wound healing, tissue-resident macrophages and infiltrating monocytes are polarized toward an M1 phenotype in response to injury or infection. However, after this initial inflammatory response, these macrophages shift toward an M2 phenotype and produce anti-inflammatory cytokines as part of the normal wound healing process. This transition in macrophage behavior alters the phenotype of neighboring fibroblasts and induces their differentiation into myofibroblasts, which are capable of producing extracellular matrix (ECM) proteins, such as collagen.Citation50 The intercellular communications and the consequent matrix remodeling that are normally observed during wound healing are also seen in the tumor microenvironment. For this reason, tumors have often been referred to as “wounds that never heal.”Citation51
In fibrotic disorders, macrophages are recognized for their capacity to both promote and inhibit ECM accumulation. For example, in a carbon tetrachloride (CCl4)-induced liver injury model, macrophages are necessary for both the development as well as the spontaneous resolution of hepatic fibrosis. In these studies, the depletion of macrophages was found to prevent the generation of mature, cross-linked collagen in the liver.Citation52 In contrast, the ability of macrophages to secrete MMP13 (best known as collagenase 3), a member of the MMP family of neural endopeptidases, was determined to be necessary for the resolution of hepatic fibrosis induced by CCl4.Citation53 In an effort to understand the mechanism driving the distinct functional outcomes of macrophage activation, Ramachandran et al. found that macrophages involved in resolving CCl4-induced hepatic fibrosis are recruited from the peripheral blood and display a gene signature profile that is distinct from that of macrophages promoting fibrosis. In this study, the macrophages that were responsible for fibrosis resolution showed a marked increase in the expression of several MMPs, including MMP13 as well as MMP2, 9 and 12. These restorative macrophages were also found to express elevated levels of genes involved in phagocytosis, which were necessary for accelerating fibrosis resolution.Citation54 Consistent with the existence of pro- and anti-fibrotic macrophages, in vitro studies have revealed that the expression pattern of MMPs can differ markedly between M1 and M2 macrophages.Citation55 Thus, macrophages can be programmed with either pro- or anti-fibrotic properties.
In cancer, macrophages can promote fibrosis by recruiting fibroblasts through the production of chemokines, and by producing growth factors such as transforming growth factor β1 (TGFβ1), which can stimulate the differentiation of fibroblasts into myofibroblasts and hence promote collagen deposition.Citation50,Citation56 Through the production of tissue inhibitors of metalloproteinases, macrophages can also block ECM degradation.Citation55 These pro-fibrotic activities of tumor-associated macrophages support the production and persistence of the ECM that surrounds neoplastic lesions.Citation50 In this regard, developing tumors often establish a microenvironment that is characterized as a dense desmoplastic stromal reaction marked by a robust infiltration of macrophages. This collagen-rich microenvironment can inhibit the diffusion of therapeutics.Citation57-Citation59 In preclinical models of PDA, disrupting this fibrotic reaction has been shown to improve the delivery of chemotherapy, resulting in enhanced efficacy.Citation59-Citation61 These findings illustrate the potential benefit from derailing the signaling network that supports tumor-associated fibrosis.
The ability of macrophages to inhibit fibrosis and deplete fibrosis seen in other diseases suggests the possibility that macrophages might be stimulated to resolve tumor-associated fibrosis. However, little is known about the role of macrophages in regulating cancer-associated fibrosis. In PDA, both macrophages and fibrosis are common features of the tumor microenvironment. This microenvironment, though, is not well reproduced in transplantable mouse models of PDA and therefore, could not be properly studied until recently, when a genetically engineered mouse model of PDA was developed. These mice spontaneously develop pancreatic neoplasms accompanied by a desmoplastic stromal reaction that is indistinguishable from that observed in PDA patients.Citation62 In this preclinical model, macrophages responding to a single administration of an agonist CD40-specific mAb were observed to rapidly infiltrate the tumor microenvironment and facilitate the depletion of collagen, leading to tumor regression.Citation7 While collagen depletion in this model was found to be focal and transient, the repeated administration of a fully human agonist CD40-targeting antibody in combination with gemcitabine-based chemotherapy to patients with advanced PDA induced clinical responses with each cycle of treatment.Citation63 Consistent with a role for macrophages in mediating the antitumor activity of CD40-targeting antibodies, a biopsy of a regressing tumor lesion demonstrated an impressive macrophage infiltrate with an absence of viable tumor cells.Citation7 As the tumor microenvironment in PDA is often described as fibrotic, these findings suggest the potential of macrophages induced with anti-stromal properties to impact tumor-associated fibrosis (). However, further studies are needed to understand the full capacity of macrophages to regulate fibrosis associated with cancer.
Figure 3. The phenotype of tumor-infiltrating macrophages is a critical determinant of tumor-associated stromal fibrosis. Systemic inflammation induced with an agonist CD40-specific monoclonal antibody (mAb) shifts the phenotype of tumor-infiltrating macrophages from pro- to anti-fibrotic. ECM, extracellular matrix.
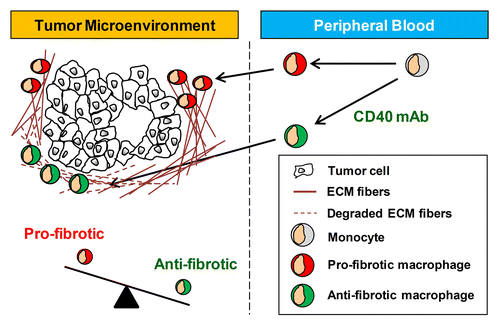
Cancer Immunosurveillance
Oncogenesis and tumor progression are contingent on the capacity of malignant cells to evade elimination by the immune system. In this regard, macrophages can be fundamental in establishing a microenvironment that is unfavorable to adaptive immunosurveillance. For example, macrophages responding to neoplastic lesions can express amino acid-metabolizing enzymes such as indoleamine 2,3-dioxygenase 1 (IDO1), nitric oxide synthase 2 (NOS2) and arginase, which generate metabolites that inhibit T-cell responses.Citation64 Tumor-associated macrophages can also produce reactive oxygen species and reactive nitrogen species, which disrupt the proper binding of the T-cell receptor (TCR) to MHC-peptide complexes present on antigen-presenting and cancer cells. In addition, through the production of immunosuppressive soluble factors such as prostaglandin E2 (PGE2), TGFβ1 and IL-10, as well as through the expression of immune checkpoint molecules such as CD174 (best known as PD-L1) and galectin 9, macrophages can suppress T-cell activation and even induce T-cell exhaustion.Citation2,Citation65 Thus, macrophages represent a major obstacle to T cell-based immunotherapy.
Strategies to reverse the immunosuppressive behavior of macrophages may offer a novel approach for restoring productive antitumor T-cell immunity in cancer. For example, signaling through the colony stimulating factor 1 receptor (CSF1R) was recently shown to be critical for defining the phenotype of tumor-infiltrating macrophages as either pro- or antitumor. In a mouse model of glioma, CSF1R inhibition was found to induce a shift in the genetic signature and phenotype of tumor-infiltrating macrophages from pro- to antitumor, prolonging the survival of tumor-bearing mice.Citation66 Blocking the CSF1R has also been shown to promote immunosurveillance by CD8+ T cells when combined with chemotherapy in a mouse model of breast carcinoma.Citation67 These findings suggest that reprogramming macrophages in vivo may support the development of a productive antitumor T cell-mediated immune response.
With an improved understanding of the genetic signatures that define pro- vs. antitumor macrophages, novel strategies to reprogram macrophages with properties supportive of antitumor T-cell immunity are now being explored. One such approach involves the use of microRNAs, short non-coding RNAs that can inhibit the expression of a panel of target genes. miR-125b is a microRNA that is enriched in macrophages. The overexpression of miR-125b in macrophages was found to increase the expression of the IFNγ receptor, allowing macrophages to respond to IFNγ with increased levels of co-stimulatory molecules and hence with an improved ability to induce T-cell activation.Citation68 miR-155 is another microRNA that is upregulated in macrophages responding to inflammatory stimuli.Citation69 Using a bioinformatics approach, Martinez-Nunez et al. found that miR-155 downregulates the IL-13 receptor α1 (IL-13Rα1), a component of the IL-4 receptor, hence inhibiting IL-4/IL-13 signaling.Citation70 In a mouse model of breast cancer, the knockdown of miR-155 accelerated tumor growth, a process that was associated with the skewing of tumor-infiltrating macrophages toward a pro-tumor phenotype.Citation71 Taken together, these findings suggest that augmenting the expression of distinct microRNAs to re-direct the biology of tumor-infiltrating macrophages toward an antitumor phenotype may support the development of antitumor T-cell immunity ().
Figure 4. Macrophage phenotype regulates T-cell activation. Growth factors such as macrophage colony-stimulating factor (M-CSF) and granulocyte macrophage colony-stimulating factor (GM-CSF), cytokines including interferon γ (IFNγ), interleukin (IL)-4, and IL-13, as well as microRNAs such as let-7c, miR-125b, miR-142–3p, and miR-155 can instruct macrophages with phenotypes associated with T-cell activation or suppression. Macrophages capable of T-cell suppression produce IL-10 and express T cell-inhibitory molecules including CD274 (best known as PD-L1) and galectin 9 (Gal9), whereas macrophages that sustain T-cell activation produce IL-12 and express co-stimulatory molecules including CD86.
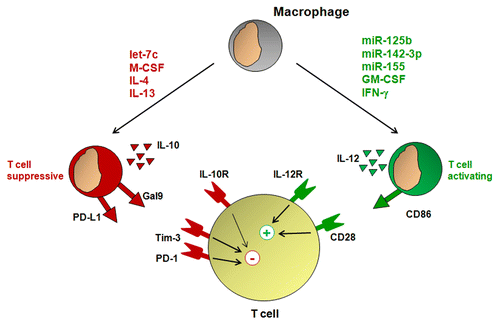
While microRNAs can be critical in directing macrophages toward an antitumor phenotype, they can also be involved in polarizing macrophages with a pro-tumor phenotype. For example, macrophages responding to macrophage colony-stimulating factor express high levels of the miRNA let-7c, which inhibits the production of molecules associated with antitumor T-cell immunity, including IL-12, and enhances the production of arginase which can promoting tumor growth by suppressing T cell immunosurveillance.Citation64,Citation72 An understanding of the complexity by which microRNAs regulate the biology of macrophages is beginning to emerge, and is revealing that multiple microRNAs can act to polarize macrophages with either pro- or antitumor properties.Citation73,Citation74 It is likely that a balance in the expression of various microRNAs that are regulated by microenvironmental factors is critical for defining the polarization status of macrophages.
MicroRNAs can also be involved in the differentiation of macrophages during tumor-induced myelopoiesis. In particular, miR-142–3p, which is generally downregulated in tumor-recruited myeloid cells, has recently been demonstrated to be an important regulator of T-cell immunity. Reduced levels of miR-142–3p have been found to support the differentiation of macrophages with immunosuppressive properties, whereas the constitutive expression of miR-142–3p in myeloid cells inhibits macrophage differentiation by skewing tumor-induced myelopoiesis toward the granulocyte lineage, resulting in improved T-cell activation.Citation75 Intriguingly, Sonda et al. used bone marrow chimera studies in a mouse model of fibrosarcoma to show that constitutive expression of miR-142–3p in bone marrow cells can enhance the efficacy of adoptive therapy with tumor-specific T cells. These findings point to the potential of strategies designed to reprogram macrophages for enhancing T-cell immunotherapy.
Concluding Remarks
Macrophages are attractive targets for cancer immunotherapy because of their unique ability to regulate key elements of oncogenesis and tumor progression, including cancer cell viability and invasiveness, angiogenesis, and fibrosis. Because the activity of macrophages is dependent on microenvironmental signals, it is likely that many anticancer therapies that are designed to target malignant cells also impact the biology of macrophages. For example, chemotherapy- and radiotherapy-induced death of tumor cells is known to induce the recruitment of macrophages to the tumor microenvironment.Citation67,Citation76 Similarly, targeted anticancer therapeutics that are currently being explored in the clinic, such as specific inhibitors of JAK/STAT, NOTCH, and PI3K/AKT1 signaling, may also exert on-target effects in normal cells such as macrophages. Thus, targeting these signaling pathways may have an important impact on macrophage activity, which may either enhance or hinder therapeutic responses. As a result, it will be critical to understand the role of conventional and experimental therapies in shaping macrophage behavior.
Macrophages are commonly recognized as obstacles to many forms of anticancer therapy. However, if instructed properly, macrophages may mediate robust antitumor effects. For example, macrophages can reduce tumor-associated fibrosis, which is a key barrier against the delivery of chemotherapy.Citation7 Thus, providing macrophages with anti-fibrotic properties may hold promise for facilitating the delivery of chemotherapy to neoplastic lesions. Because macrophages can rapidly debulk tumors, they may also be useful in downsizing tumors that were initially considered borderline for surgical resection. In addition, blocking CD47-SIRPα signaling may prime macrophages for enhancing antibody-based immunotherapies, as it facilitates the Fc receptor-mediated phagocytosis of antibody-coated cancer cells. Finally, shifting the phenotype of tumor-promoting macrophages may reverse many of the immunosuppressive mechanisms established within the tumor microenvironment and thus enhance the efficacy of T cell-based therapeutic approaches.
In summary, strategies to tame macrophages and instruct them with antitumor properties hold promise. Clinical studies testing approaches that directly activate macrophages or induce a systemic inflammatory reaction that confers antitumor activity to macrophages have been shown to provide clinical benefit to some patients. Further studies are needed to improve our understanding of the intricate link between macrophage activity and tumor viability, angiogenesis and fibrosis. This knowledge is expected to facilitate the development of novel approaches to harness macrophages for debulking tumors as well as for enhancing the efficacy of conventional and experimental forms of cancer therapy.
Abbreviations: | ||
ANG2 | = | angiopoietin 2 |
CCl4 | = | carbon tetrachloride |
CSF1R | = | colony stimulating factor 1 receptor |
ECM | = | extracellular matrix |
GM-CSF | = | granulocyte macrophage colony-stimulating factor |
HIF | = | hypoxia-inducible factor |
IFN | = | interferon |
IL | = | interleukin |
LDL | = | low density lipoprotein |
LRP | = | LDL-receptor related protein |
mAb | = | monoclonal antibody |
MMP | = | matrix metalloproteinase |
mTOR | = | mammalian target of rapamycin |
NOD | = | nucleotide-binding oligomerization domain |
PDA | = | pancreatic ductal adenocarcinoma |
SIRPα | = | signal regulatory protein α |
TLR | = | toll-like receptor |
TNFα | = | tumor necrosis factor α |
VEGF | = | vascular endothelial growth factor |
Disclosure of Potential Conflicts of Interest
No potential conflicts of interest were disclosed.
Acknowledgments
This work was supported by grants from the National Institutes of Health (K08 CA138907), W.W. Smith Charitable Trust Foundation, Department of Defense (W81XWH-12–1-0411), and the Damon Runyon Cancer Research Foundation for which Gregory L. Beatty is the Nadia's Gift Foundation Innovator of the Damon Runyon-Rachleff Innovation Award (DRR-15–12). We are grateful for members of the Beatty lab for helpful discussions.
Citation: Long KB, Beatty GL. Harnessing the antitumor potential of macrophages for cancer immunotherapy. OncoImmunology 2013; 2:e26860; 10.4161/onci.26860
References
- Gordon S. Alternative activation of macrophages. Nat Rev Immunol 2003; 3:23 - 35; http://dx.doi.org/10.1038/nri978; PMID: 12511873
- Mantovani A, Sozzani S, Locati M, Allavena P, Sica A. Macrophage polarization: tumor-associated macrophages as a paradigm for polarized M2 mononuclear phagocytes. Trends Immunol 2002; 23:549 - 55; http://dx.doi.org/10.1016/S1471-4906(02)02302-5; PMID: 12401408
- Hanahan D, Weinberg RA. Hallmarks of cancer: the next generation. Cell 2011; 144:646 - 74; http://dx.doi.org/10.1016/j.cell.2011.02.013; PMID: 21376230
- Qian BZ, Pollard JW. Macrophage diversity enhances tumor progression and metastasis. Cell 2010; 141:39 - 51; http://dx.doi.org/10.1016/j.cell.2010.03.014; PMID: 20371344
- Ferrone C, Dranoff G. Dual roles for immunity in gastrointestinal cancers. J Clin Oncol 2010; 28:4045 - 51; http://dx.doi.org/10.1200/JCO.2010.27.9992; PMID: 20644090
- De Palma M, Lewis CE. Macrophage regulation of tumor responses to anticancer therapies. Cancer Cell 2013; 23:277 - 86; http://dx.doi.org/10.1016/j.ccr.2013.02.013; PMID: 23518347
- Beatty GL, Chiorean EG, Fishman MP, Saboury B, Teitelbaum UR, Sun W, Huhn RD, Song W, Li D, Sharp LL, et al. CD40 agonists alter tumor stroma and show efficacy against pancreatic carcinoma in mice and humans. Science 2011; 331:1612 - 6; http://dx.doi.org/10.1126/science.1198443; PMID: 21436454
- Fidler IJ, Sone S, Fogler WE, Barnes ZL. Eradication of spontaneous metastases and activation of alveolar macrophages by intravenous injection of liposomes containing muramyl dipeptide. Proc Natl Acad Sci U S A 1981; 78:1680 - 4; http://dx.doi.org/10.1073/pnas.78.3.1680; PMID: 6940181
- Willingham SB, Volkmer JP, Gentles AJ, Sahoo D, Dalerba P, Mitra SS, Wang J, Contreras-Trujillo H, Martin R, Cohen JD, et al. The CD47-signal regulatory protein alpha (SIRPa) interaction is a therapeutic target for human solid tumors. Proc Natl Acad Sci U S A 2012; 109:6662 - 7; http://dx.doi.org/10.1073/pnas.1121623109; PMID: 22451913
- Weiskopf K, Ring AM, Ho CC, Volkmer JP, Levin AM, Volkmer AK, Ozkan E, Fernhoff NB, van de Rijn M, Weissman IL, et al. Engineered SIRPα variants as immunotherapeutic adjuvants to anticancer antibodies. Science 2013; 341:88 - 91; http://dx.doi.org/10.1126/science.1238856; PMID: 23722425
- Linde N, Lederle W, Depner S, van Rooijen N, Gutschalk CM, Mueller MM. Vascular endothelial growth factor-induced skin carcinogenesis depends on recruitment and alternative activation of macrophages. J Pathol 2012; 227:17 - 28; http://dx.doi.org/10.1002/path.3989; PMID: 22262122
- Roda JM, Wang Y, Sumner LA, Phillips GS, Marsh CB, Eubank TD. Stabilization of HIF-2α induces sVEGFR-1 production from tumor-associated macrophages and decreases tumor growth in a murine melanoma model. J Immunol 2012; 189:3168 - 77; http://dx.doi.org/10.4049/jimmunol.1103817; PMID: 22869907
- Kleinerman ES, Murray JL, Snyder JS, Cunningham JE, Fidler IJ. Activation of tumoricidal properties in monocytes from cancer patients following intravenous administration of liposomes containing muramyl tripeptide phosphatidylethanolamine. Cancer Res 1989; 49:4665 - 70; PMID: 2787207
- Meyers PA, Schwartz CL, Krailo MD, Healey JH, Bernstein ML, Betcher D, Ferguson WS, Gebhardt MC, Goorin AM, Harris M, et al, Children’s Oncology Group. Osteosarcoma: the addition of muramyl tripeptide to chemotherapy improves overall survival--a report from the Children’s Oncology Group. J Clin Oncol 2008; 26:633 - 8; http://dx.doi.org/10.1200/JCO.2008.14.0095; PMID: 18235123
- Luo Y, Knudson MJ. Mycobacterium bovis bacillus Calmette-Guérin-induced macrophage cytotoxicity against bladder cancer cells. Clin Dev Immunol 2010; 2010:357591; http://dx.doi.org/10.1155/2010/357591; PMID: 20862387
- Heldwein KA, Liang MD, Andresen TK, Thomas KE, Marty AM, Cuesta N, Vogel SN, Fenton MJ. TLR2 and TLR4 serve distinct roles in the host immune response against Mycobacterium bovis BCG. J Leukoc Biol 2003; 74:277 - 86; http://dx.doi.org/10.1189/jlb.0103026; PMID: 12885945
- Le DT, Brockstedt DG, Nir-Paz R, Hampl J, Mathur S, Nemunaitis J, Sterman DH, Hassan R, Lutz E, Moyer B, et al. A live-attenuated Listeria vaccine (ANZ-100) and a live-attenuated Listeria vaccine expressing mesothelin (CRS-207) for advanced cancers: phase I studies of safety and immune induction. Clin Cancer Res 2012; 18:858 - 68; http://dx.doi.org/10.1158/1078-0432.CCR-11-2121; PMID: 22147941
- Maciag PC, Radulovic S, Rothman J. The first clinical use of a live-attenuated Listeria monocytogenes vaccine: a Phase I safety study of Lm-LLO-E7 in patients with advanced carcinoma of the cervix. Vaccine 2009; 27:3975 - 83; http://dx.doi.org/10.1016/j.vaccine.2009.04.041; PMID: 19389451
- Rothman J, Paterson Y. Live-attenuated Listeria-based immunotherapy. Expert Rev Vaccines 2013; 12:493 - 504; http://dx.doi.org/10.1586/erv.13.34; PMID: 23659298
- Rai RK, Vishvakarma NK, Mohapatra TM, Singh SM. Augmented macrophage differentiation and polarization of tumor-associated macrophages towards M1 subtype in listeria-administered tumor-bearing host. J Immunother 2012; 35:544 - 54; http://dx.doi.org/10.1097/CJI.0b013e3182661afa; PMID: 22892451
- Nathan CF. Secretory products of macrophages. J Clin Invest 1987; 79:319 - 26; http://dx.doi.org/10.1172/JCI112815; PMID: 3543052
- MacMicking J, Xie QW, Nathan C. Nitric oxide and macrophage function. Annu Rev Immunol 1997; 15:323 - 50; http://dx.doi.org/10.1146/annurev.immunol.15.1.323; PMID: 9143691
- Blankenstein T, Qin ZH, Uberla K, Müller W, Rosen H, Volk HD, Diamantstein T. Tumor suppression after tumor cell-targeted tumor necrosis factor alpha gene transfer. J Exp Med 1991; 173:1047 - 52; http://dx.doi.org/10.1084/jem.173.5.1047; PMID: 2022919
- McBride WH. Phenotype and functions of intratumoral macrophages. Biochim Biophys Acta 1986; 865:27 - 41; PMID: 3524684
- Urban JL, Shepard HM, Rothstein JL, Sugarman BJ, Schreiber H. Tumor necrosis factor: a potent effector molecule for tumor cell killing by activated macrophages. Proc Natl Acad Sci U S A 1986; 83:5233 - 7; http://dx.doi.org/10.1073/pnas.83.14.5233; PMID: 3487788
- Miyanishi M, Tada K, Koike M, Uchiyama Y, Kitamura T, Nagata S. Identification of Tim4 as a phosphatidylserine receptor. Nature 2007; 450:435 - 9; http://dx.doi.org/10.1038/nature06307; PMID: 17960135
- Segawa K, Suzuki J, Nagata S. Constitutive exposure of phosphatidylserine on viable cells. Proc Natl Acad Sci U S A 2011; 108:19246 - 51; http://dx.doi.org/10.1073/pnas.1114799108; PMID: 22084121
- Gardai SJ, McPhillips KA, Frasch SC, Janssen WJ, Starefeldt A, Murphy-Ullrich JE, Bratton DL, Oldenborg PA, Michalak M, Henson PM. Cell-surface calreticulin initiates clearance of viable or apoptotic cells through trans-activation of LRP on the phagocyte. Cell 2005; 123:321 - 34; http://dx.doi.org/10.1016/j.cell.2005.08.032; PMID: 16239148
- Chao MP, Jaiswal S, Weissman-Tsukamoto R, Alizadeh AA, Gentles AJ, Volkmer J, Weiskopf K, Willingham SB, Raveh T, Park CY, et al. Calreticulin is the dominant pro-phagocytic signal on multiple human cancers and is counterbalanced by CD47. Sci Transl Med 2010; 2:63ra94; http://dx.doi.org/10.1126/scitranslmed.3001375; PMID: 21178137
- Grugan KD, McCabe FL, Kinder M, Greenplate AR, Harman BC, Ekert JE, van Rooijen N, Anderson GM, Nemeth JA, Strohl WR, et al. Tumor-associated macrophages promote invasion while retaining Fc-dependent anti-tumor function. J Immunol 2012; 189:5457 - 66; http://dx.doi.org/10.4049/jimmunol.1201889; PMID: 23105143
- Oldenborg PA, Gresham HD, Lindberg FP. CD47-signal regulatory protein alpha (SIRPalpha) regulates Fcgamma and complement receptor-mediated phagocytosis. J Exp Med 2001; 193:855 - 62; http://dx.doi.org/10.1084/jem.193.7.855; PMID: 11283158
- Oldenborg PA, Zheleznyak A, Fang YF, Lagenaur CF, Gresham HD, Lindberg FP. Role of CD47 as a marker of self on red blood cells. Science 2000; 288:2051 - 4; http://dx.doi.org/10.1126/science.288.5473.2051; PMID: 10856220
- Jaiswal S, Jamieson CH, Pang WW, Park CY, Chao MP, Majeti R, Traver D, van Rooijen N, Weissman IL. CD47 is upregulated on circulating hematopoietic stem cells and leukemia cells to avoid phagocytosis. Cell 2009; 138:271 - 85; http://dx.doi.org/10.1016/j.cell.2009.05.046; PMID: 19632178
- Majeti R, Chao MP, Alizadeh AA, Pang WW, Jaiswal S, Gibbs KD Jr., van Rooijen N, Weissman IL. CD47 is an adverse prognostic factor and therapeutic antibody target on human acute myeloid leukemia stem cells. Cell 2009; 138:286 - 99; http://dx.doi.org/10.1016/j.cell.2009.05.045; PMID: 19632179
- Leek RD, Lewis CE, Whitehouse R, Greenall M, Clarke J, Harris AL. Association of macrophage infiltration with angiogenesis and prognosis in invasive breast carcinoma. Cancer Res 1996; 56:4625 - 9; PMID: 8840975
- Onita T, Ji PG, Xuan JW, Sakai H, Kanetake H, Maxwell PH, Fong GH, Gabril MY, Moussa M, Chin JL. Hypoxia-induced, perinecrotic expression of endothelial Per-ARNT-Sim domain protein-1/hypoxia-inducible factor-2alpha correlates with tumor progression, vascularization, and focal macrophage infiltration in bladder cancer. Clin Cancer Res 2002; 8:471 - 80; PMID: 11839666
- Lin EY, Li JF, Gnatovskiy L, Deng Y, Zhu L, Grzesik DA, Qian H, Xue XN, Pollard JW. Macrophages regulate the angiogenic switch in a mouse model of breast cancer. Cancer Res 2006; 66:11238 - 46; http://dx.doi.org/10.1158/0008-5472.CAN-06-1278; PMID: 17114237
- Carmi Y, Voronov E, Dotan S, Lahat N, Rahat MA, Fogel M, Huszar M, White MR, Dinarello CA, Apte RN. The role of macrophage-derived IL-1 in induction and maintenance of angiogenesis. J Immunol 2009; 183:4705 - 14; http://dx.doi.org/10.4049/jimmunol.0901511; PMID: 19752225
- De Palma M, Naldini L. Angiopoietin-2 TIEs up macrophages in tumor angiogenesis. Clin Cancer Res 2011; 17:5226 - 32; http://dx.doi.org/10.1158/1078-0432.CCR-10-0171; PMID: 21576085
- Mazzieri R, Pucci F, Moi D, Zonari E, Ranghetti A, Berti A, Politi LS, Gentner B, Brown JL, Naldini L, et al. Targeting the ANG2/TIE2 axis inhibits tumor growth and metastasis by impairing angiogenesis and disabling rebounds of proangiogenic myeloid cells. Cancer Cell 2011; 19:512 - 26; http://dx.doi.org/10.1016/j.ccr.2011.02.005; PMID: 21481792
- Murdoch C, Tazzyman S, Webster S, Lewis CE. Expression of Tie-2 by human monocytes and their responses to angiopoietin-2. J Immunol 2007; 178:7405 - 11; PMID: 17513791
- Huang Y, Yuan J, Righi E, Kamoun WS, Ancukiewicz M, Nezivar J, Santosuosso M, Martin JD, Martin MR, Vianello F, et al. Vascular normalizing doses of antiangiogenic treatment reprogram the immunosuppressive tumor microenvironment and enhance immunotherapy. Proc Natl Acad Sci U S A 2012; 109:17561 - 6; http://dx.doi.org/10.1073/pnas.1215397109; PMID: 23045683
- Du R, Lu KV, Petritsch C, Liu P, Ganss R, Passegué E, Song H, Vandenberg S, Johnson RS, Werb Z, et al. HIF1alpha induces the recruitment of bone marrow-derived vascular modulatory cells to regulate tumor angiogenesis and invasion. Cancer Cell 2008; 13:206 - 20; http://dx.doi.org/10.1016/j.ccr.2008.01.034; PMID: 18328425
- Roda JM, Sumner LA, Evans R, Phillips GS, Marsh CB, Eubank TD. Hypoxia-inducible factor-2α regulates GM-CSF-derived soluble vascular endothelial growth factor receptor 1 production from macrophages and inhibits tumor growth and angiogenesis. J Immunol 2011; 187:1970 - 6; http://dx.doi.org/10.4049/jimmunol.1100841; PMID: 21765015
- Chen W, Ma T, Shen XN, Xia XF, Xu GD, Bai XL, Liang TB. Macrophage-induced tumor angiogenesis is regulated by the TSC2-mTOR pathway. Cancer Res 2012; 72:1363 - 72; http://dx.doi.org/10.1158/0008-5472.CAN-11-2684; PMID: 22287548
- Del Vecchio M, Bajetta E, Canova S, Lotze MT, Wesa A, Parmiani G, Anichini A. Interleukin-12: biological properties and clinical application. Clin Cancer Res 2007; 13:4677 - 85; http://dx.doi.org/10.1158/1078-0432.CCR-07-0776; PMID: 17699845
- Strasly M, Cavallo F, Geuna M, Mitola S, Colombo MP, Forni G, Bussolino F. IL-12 inhibition of endothelial cell functions and angiogenesis depends on lymphocyte-endothelial cell cross-talk. J Immunol 2001; 166:3890 - 9; PMID: 11238633
- Sgadari C, Angiolillo AL, Tosato G. Inhibition of angiogenesis by interleukin-12 is mediated by the interferon-inducible protein 10. Blood 1996; 87:3877 - 82; PMID: 8611715
- Guiducci C, Vicari AP, Sangaletti S, Trinchieri G, Colombo MP. Redirecting in vivo elicited tumor infiltrating macrophages and dendritic cells towards tumor rejection. Cancer Res 2005; 65:3437 - 46; PMID: 15833879
- Murray PJ, Wynn TA. Protective and pathogenic functions of macrophage subsets. Nat Rev Immunol 2011; 11:723 - 37; http://dx.doi.org/10.1038/nri3073; PMID: 21997792
- Dvorak HF. Tumors: wounds that do not heal. Similarities between tumor stroma generation and wound healing. N Engl J Med 1986; 315:1650 - 9; http://dx.doi.org/10.1056/NEJM198612253152606; PMID: 3537791
- Duffield JS, Forbes SJ, Constandinou CM, Clay S, Partolina M, Vuthoori S, Wu S, Lang R, Iredale JP. Selective depletion of macrophages reveals distinct, opposing roles during liver injury and repair. J Clin Invest 2005; 115:56 - 65; PMID: 15630444
- Fallowfield JA, Mizuno M, Kendall TJ, Constandinou CM, Benyon RC, Duffield JS, Iredale JP. Scar-associated macrophages are a major source of hepatic matrix metalloproteinase-13 and facilitate the resolution of murine hepatic fibrosis. J Immunol 2007; 178:5288 - 95; PMID: 17404313
- Ramachandran P, Pellicoro A, Vernon MA, Boulter L, Aucott RL, Ali A, Hartland SN, Snowdon VK, Cappon A, Gordon-Walker TT, et al. Differential Ly-6C expression identifies the recruited macrophage phenotype, which orchestrates the regression of murine liver fibrosis. Proc Natl Acad Sci U S A 2012; 109:E3186 - 95; http://dx.doi.org/10.1073/pnas.1119964109; PMID: 23100531
- Khokha R, Murthy A, Weiss A. Metalloproteinases and their natural inhibitors in inflammation and immunity. Nat Rev Immunol 2013; 13:649 - 65; http://dx.doi.org/10.1038/nri3499; PMID: 23969736
- Roberts AB, Sporn MB, Assoian RK, Smith JM, Roche NS, Wakefield LM, Heine UI, Liotta LA, Falanga V, Kehrl JH, et al. Transforming growth factor type beta: rapid induction of fibrosis and angiogenesis in vivo and stimulation of collagen formation in vitro. Proc Natl Acad Sci U S A 1986; 83:4167 - 71; http://dx.doi.org/10.1073/pnas.83.12.4167; PMID: 2424019
- Netti PA, Berk DA, Swartz MA, Grodzinsky AJ, Jain RK. Role of extracellular matrix assembly in interstitial transport in solid tumors. Cancer Res 2000; 60:2497 - 503; PMID: 10811131
- Choi J, Credit K, Henderson K, Deverkadra R, He Z, Wiig H, Vanpelt H, Flessner MF. Intraperitoneal immunotherapy for metastatic ovarian carcinoma: Resistance of intratumoral collagen to antibody penetration. Clin Cancer Res 2006; 12:1906 - 12; http://dx.doi.org/10.1158/1078-0432.CCR-05-2141; PMID: 16551876
- Olive KP, Jacobetz MA, Davidson CJ, Gopinathan A, McIntyre D, Honess D, Madhu B, Goldgraben MA, Caldwell ME, Allard D, et al. Inhibition of Hedgehog signaling enhances delivery of chemotherapy in a mouse model of pancreatic cancer. Science 2009; 324:1457 - 61; http://dx.doi.org/10.1126/science.1171362; PMID: 19460966
- Provenzano PP, Cuevas C, Chang AE, Goel VK, Von Hoff DD, Hingorani SR. Enzymatic targeting of the stroma ablates physical barriers to treatment of pancreatic ductal adenocarcinoma. Cancer Cell 2012; 21:418 - 29; http://dx.doi.org/10.1016/j.ccr.2012.01.007; PMID: 22439937
- Jacobetz MA, Chan DS, Neesse A, Bapiro TE, Cook N, Frese KK, Feig C, Nakagawa T, Caldwell ME, Zecchini HI, et al. Hyaluronan impairs vascular function and drug delivery in a mouse model of pancreatic cancer. Gut 2013; 62:112 - 20; http://dx.doi.org/10.1136/gutjnl-2012-302529; PMID: 22466618
- Hingorani SR, Wang L, Multani AS, Combs C, Deramaudt TB, Hruban RH, Rustgi AK, Chang S, Tuveson DA. Trp53R172H and KrasG12D cooperate to promote chromosomal instability and widely metastatic pancreatic ductal adenocarcinoma in mice. Cancer Cell 2005; 7:469 - 83; http://dx.doi.org/10.1016/j.ccr.2005.04.023; PMID: 15894267
- Beatty GL, Torigian DA, Chiorean EG, Saboury B, Brothers A, Alavi A, Troxel AB, Sun W, Teitelbaum UR, Vonderheide RH, et al. A phase I study of an agonist CD40 monoclonal antibody (CP-870,893) in combination with gemcitabine in patients with advanced pancreatic ductal adenocarcinoma. Clin Cancer Res 2013; In press http://dx.doi.org/10.1158/1078-0432.CCR-13-1320; PMID: 23983255
- Frey AB. Myeloid suppressor cells regulate the adaptive immune response to cancer. J Clin Invest 2006; 116:2587 - 90; http://dx.doi.org/10.1172/JCI29906; PMID: 17016554
- Pardoll DM. The blockade of immune checkpoints in cancer immunotherapy. Nat Rev Cancer 2012; 12:252 - 64; http://dx.doi.org/10.1038/nrc3239; PMID: 22437870
- Pyonteck SM, Akkari L, Schuhmacher AJ, Bowman RL, Sevenich L, Quail DF, Olson OC, Quick ML, Huse JT, Teijeiro V, et al. CSF-1R inhibition alters macrophage polarization and blocks glioma progression. Nat Med 2013; 19:1264 - 72; http://dx.doi.org/10.1038/nm.3337; PMID: 24056773
- DeNardo DG, Brennan DJ, Rexhepaj E, Ruffell B, Shiao SL, Madden SF, Gallagher WM, Wadhwani N, Keil SD, Junaid SA, et al. Leukocyte complexity predicts breast cancer survival and functionally regulates response to chemotherapy. Cancer Discov 2011; 1:54 - 67; http://dx.doi.org/10.1158/2159-8274.CD-10-0028; PMID: 22039576
- Chaudhuri AA, So AY, Sinha N, Gibson WS, Taganov KD, O’Connell RM, Baltimore D. MicroRNA-125b potentiates macrophage activation. J Immunol 2011; 187:5062 - 8; http://dx.doi.org/10.4049/jimmunol.1102001; PMID: 22003200
- O’Connell RM, Taganov KD, Boldin MP, Cheng G, Baltimore D. MicroRNA-155 is induced during the macrophage inflammatory response. Proc Natl Acad Sci U S A 2007; 104:1604 - 9; http://dx.doi.org/10.1073/pnas.0610731104; PMID: 17242365
- Martinez-Nunez RT, Louafi F, Sanchez-Elsner T. The interleukin 13 (IL-13) pathway in human macrophages is modulated by microRNA-155 via direct targeting of interleukin 13 receptor alpha1 (IL13Ralpha1). J Biol Chem 2011; 286:1786 - 94; http://dx.doi.org/10.1074/jbc.M110.169367; PMID: 21097505
- Zonari E, Pucci F, Saini M, Mazzieri R, Politi LS, Gentner B, Naldini L. A role for miR-155 in enabling tumor-infiltrating innate immune cells to mount effective antitumor responses in mice. Blood 2013; 122:243 - 52; http://dx.doi.org/10.1182/blood-2012-08-449306; PMID: 23487026
- Banerjee S, Xie N, Cui H, Tan Z, Yang S, Icyuz M, Abraham E, Liu G. MicroRNA let-7c regulates macrophage polarization. J Immunol 2013; 190:6542 - 9; http://dx.doi.org/10.4049/jimmunol.1202496; PMID: 23667114
- Locati M, Mantovani A, Sica A. Macrophage activation and polarization as an adaptive component of innate immunity. Adv Immunol 2013; 120:163 - 84; http://dx.doi.org/10.1016/B978-0-12-417028-5.00006-5; PMID: 24070384
- Montagner S, Orlandi EM, Merante S, Monticelli S. The role of miRNAs in mast cells and other innate immune cells. Immunol Rev 2013; 253:12 - 24; http://dx.doi.org/10.1111/imr.12042; PMID: 23550635
- Sonda N, Simonato F, Peranzoni E, Calì B, Bortoluzzi S, Bisognin A, Wang E, Marincola FM, Naldini L, Gentner B, et al. miR-142-3p prevents macrophage differentiation during cancer-induced myelopoiesis. Immunity 2013; 38:1236 - 49; http://dx.doi.org/10.1016/j.immuni.2013.06.004; PMID: 23809164
- Xu J, Escamilla J, Mok S, David J, Priceman S, West B, Bollag G, McBride W, Wu L. CSF1R signaling blockade stanches tumor-infiltrating myeloid cells and improves the efficacy of radiotherapy in prostate cancer. Cancer Res 2013; 73:2782 - 94; http://dx.doi.org/10.1158/0008-5472.CAN-12-3981; PMID: 23418320