Abstract
Peptide vaccines derived from CD8+ T-cell epitopes have shown variable efficacy in cancer patients. Thus, some peptide vaccines are capable of activating CD8+ T-cell responses, even in the absence of CD4+ T-cell epitopes or dendritic cell (DC)-activating adjuvants. However, the mechanisms underlying the clinical activity of these potent peptides are poorly understood. Using CT26 and ovalbumin-expressing B16 murine allograft tumor models, we found that the antitumor effect of helper cell-independent CD8 T-cell peptide vaccines is inhibited by the blockade of CD40 ligand (CD40L) in vivo. Furthermore, in vitro stimulation with antigenic peptides of cells derived from immunized mice induced the expression of CD40L on the surface of CD8+ T cells and fostered DC maturation, an effect that was partially inhibited by CD40L-blocking antibodies. Interestingly, CD40L blockade also inhibited CD8+ T-cell responses, even in the presence of fully mature DCs, suggesting a role for CD40L not only in promoting DC maturation but also in mediating CD8+ T-cell co-stimulation. Importantly, these potent peptides share features with bona fide CD4 epitopes, since they foster responses against less immunogenic CD8+ T-cell epitopes in a CD40L-dependent manner. The analysis of peptides used for the vaccination of cancer patients in clinical trials showed that these peptides also induce the expression of CD40L on the surface of CD8+ T cells. Taken together, these results suggest that CD40L expression induced by potent CD8+ T-cell epitopes can activate antitumor CD8+ T-cell responses, potentially amplifying the immunological responses to less immunogenic CD8+ T-cell epitopes and bypassing the requirement for CD4+ helper T cells in vaccination protocols.
Introduction
The vaccination of cancer patients to induce antitumor CD8+ T-cell responses is considered a promising immunotherapeutic strategy. To achieve such an anticancer immunological response a number of vaccines comprising many different antigenic formats have been previously tested, as recently reviewed by Vacchelli et al.Citation1 The initial characterization of tumor-associated antigens (TAAs) led to the development of vaccination protocols based on the administration of synthetic peptides encompassing minimal CD8+ T-cell epitopes.Citation2,Citation3 However, in contrast to microbial components that activate dendritic cells (DCs) sufficiently to induce their maturation and efficient antigen presentation, minimal CD8+ epitopes lack such DC activation signals. Moreover, complex antigens such as those derived from pathogens also bear epitopes recognized by CD4+ T cells. The presentation of these epitopes to antigen-specific CD4+ T cells leads to the upregulation of CD40 ligand (CD40L), thereby providing an additional signal for DC maturation.Citation4 Thus, despite presentation in complex with MHC class I molecules on the surface of tumor cells, peptide epitopes are typically poorly immunogenic when administered without adjuvants that promote the activation of DCs such as pathogen components or CD4+ T-cell epitopes.
Despite these common features related to low immunogenicity of peptide vaccines, there are some exceptions. For example, even when administered in a relatively weak adjuvant that is unable to activate DCs, such as the incomplete Freund’s adjuvant (IFA), some highly immunogenic peptides corresponding to immunodominant CD8+ T-cell epitopes can activate CD8+ T-cell responses independently of signals coming from CD4+ T cells.Citation5 Numerous clinical trials have been performed to date to test peptide vaccines in cancer patients, with variable results regarding the induction of immune responses. Interestingly, robust antitumor immune responses have been detected in vaccinated patients in some studies,Citation6-Citation13 whereas poor responses have been reported in other cases.Citation14 Importantly, the mechanisms underlying the efficacy of such peptides to induce helper cell-independent, antitumor CD8+ T-cell responses remain poorly understood. Further insights into these mechanisms may assist the selection of optimal candidate peptides for the development of effective antitumor vaccines as well as the design of improved vaccination protocols incorporating the complete range of immune elements that are involved in the activity of highly immunogenic epitopes.
Several mechanisms have been proposed to explain the ability of some peptides to promote helper cell-independent CD8+ T-cell responses against cancer cells, including: an elevated affinity for MHC class I molecules or T-cell receptors (TCRs), a high frequency of peptide-specific T-cell precursors, and the propensity of peptide-specific T cells to secrete high levels of pro-inflammatory cytokines.Citation5,Citation15-Citation18 However, the molecular basis of peptide-induced, helper cell-independent CD8+ T-cell responses remains obscure. Considering the critical role that the reciprocal interaction between CD40 and its ligand (CD40L) plays in the induction of helper cell-dependent CD8+ T cells, we analyzed its role in helper cell-independent responses to TAA-derived peptides. Here, we demonstrate that highly immunogenic peptides induce the expression of CD40L by activated TAA-specific CD8+ T cells, mediating CD40/CD40L interactions and fostering helper cell-independent, antitumor CD8+ T-cell responses. Our findings reinforce the importance of CD40L in efficacious vaccination strategies and reveal that, in addition to their classical function as cytotoxic effector cells, CD8+ T cells may also function as modulators of immune responses.
Results
Immunization with potent CD8+ T cell epitope peptides induces antitumor and helper cell-independent CD8+ T-cell responses
Curiously, it has been reported that some TAA-derived epitopes can induce T-cell responses in cancer patients even when administered with adjuvants (e.g., IFA or its equivalent Montanide) that fail to provide DC-activating signals.Citation6-Citation9 In order to understand the physiological mechanisms responsible for these enigmatic properties, we first set out to characterize CD8+ T-cell peptide vaccines derived from TAAs that had previously been observed to possess antitumor effects in murine models in vivo. In a prior study,Citation19 we showed that the CD8+ T-cell peptide AH1 (which derives from gp70, a TAA expressed by CT26 murine colorectal carcinoma cells) is unable to elicit CD8+ T-cell responses and consequently does not negatively impact tumor growth. Thus, we utilized an improved variant of this peptide (AH1-A5),Citation20 corresponding to AH1 with an amino acid substitution (A) at position 5. Both AH1 and AH1-A5 have the same affinity for Ld MHC class I molecules, but the bipartite Ld/AH1-A5 complex has a stronger avidity for TCRs yielding a more stable MHC-peptide-TCR complex than unmodified AH1.
Using a conventional allograft model, we found that the administration of AH1-A5 delays the growth of CT26 cells in vivo and increases the survival of tumor-bearing mice, whereas unmodified AH1 has negligible antineoplastic activity (; P < 0.05). The antitumor effects of AH1-A5 correlated with its ability to induce strong T-cell responses, as documented by the expression of interferon γ (IFNγ) by total splenocytes, whereas AH1 elicited no significant immune responses (). To characterize which specific T-cell populations were responding to AH1-A5, we used flow cytometry and assayed the responses of various T-cell subsets in vaccinated mice. With this approach, we avoided the depletion of CD4+ regulatory T cells, a setting that has previously been shown to allow for the elicitation of CD8+ T-cell antitumor responses even by weak antigenic stimuli such as AH1.Citation21 As shown in , the administration of AH1-A5 stimulated IFNγ production exclusively within CD8+ T-cell subsets, thereby increasing the percentage of IFNγ+CD8+ T cells. In contrast, vaccination induced no significant differences in the percentage of IFNγ-expressing CD4+ T cells. AH1-A5 elicited various activities associated with CD8+ T-cell effectors, such as the release of interleukin (IL)-2 or the execution of cytotoxic functions (Fig. S1A and B). Similar to what we observed for IFNγ, AH1-A5 promoted the secretion of IL-2 and tumor necrosis factor α (TNFα) only by CD8+ T cells (Fig. S1C and D). These results suggest that AH1-A5 specifically activates CD8+ T cells independently of CD4+ T cells.
Figure 1. Strong CD8+ T-cell peptide vaccines induce helper-independent, CD8+ T-cell antitumor responses. (A–C) BALB/c mice (n = 5 to 6) were immunized subcutaneously with 100 μg of peptides AH1 or AH1-A5 emulsified in incomplete Freund’s adjuvant (IFA). Control mice were administered IFA alone. Ten days later the animals were challenged with 5 × 105 CT26 tumor cells implanted s.c. (A) Tumor growth (left panel) and animal survival (right panel) was monitored twice per week. (B) Splenocytes were harvested 10 d after immunization and stimulated ex vivo for 2 d with AH1 or AH1-A5 and the number of interferon-γ (IFNγ) spot-forming cells (SFC) was measured by ELISPOT. A no antigen (Ag) control was used for comparison. (C) The expression of IFNγ by CD4+ and CD8+ T cell subsets was analyzed by immunostaining and cytofluorometric analysis of cells cultured with or without AH1-A5. Left, dot plots showing the results of the analysis of a representative mouse relative to a no peptide (pep) control. Right, bar graphs showing the mean ± SEM (n = 5) of a single experiment. (D–F) C57BL/6 mice (n = 6) were immunized s.c. with 100 μg of peptides TRP2180–188 or OVA257–264 in IFA or IFA alone and 10 d later they were challenged s.c. with 105 B16-OVA tumor cells. (D) Tumor growth (left panel) and animal survival (right panel) was monitored twice per week. (E) Splenocytes were harvested from immunized animals 10 d later and IFNγ production was measured by ELISPOT. (F) Cytofluorometric analysis and percent IFNγ expressing cells in CD4+ and CD8+ T cell subsets. Results are representative of 2–3 independent experiments. Statistical analyses of immune responses were performed by nonparametric Kruskal–Wallis and Mann–Whitney U tests. Survival curves were plotted according to the Kaplan–Meier method and the log-rank test was used to measure statistical significance. In all cases, *P < 0.05 was considered statistically significant.

In a second tumor model based on ovalbumin (OVA)-expressing B16 (B16-OVA) melanoma cells, similar immunization experiments were performed using 2 other well-characterized epitopes which are presented by Kb MHC class I molecules: OVA257–264 and the immunodominant peptide TRP2180–188, which belongs to the endogenous melanoma-associated antigen dopachrome tautomerase (DCT, best known as TRP2). The administration of neither of them could completely prevent tumor growth, although some delay in tumor progression was promoted by TRP2180–188 (). Similar to AH1-A5, TRP2180–188 stimulated IFNγ production by splenocytes (), specifically the CD8+ subset, a response that was not observed among CD4+ T cells (). In contrast, OVA257–264 induced a much weaker IFNγ response ().
Helper cell-independent CD8+ T-cell responses to peptide vaccines are contingent upon CD40L
Ahlers et al. have previously reported that peptide vaccines containing modified CD4+ T-cell epitopes with enhanced affinity for MHC class II molecules induce the expression of high levels of CD40L on the surface of CD4+ T cells, leading to robust DC activation and hence to the priming of TH1 immune responses.Citation22 Thus, we set out to address the role of CD40L in the activity of our CD8+ T cell epitope peptides. To this end, mice were immunized with AH1-A5 in the presence or absence of CD40L-blocking antibodies. As measured by reduced IFNγ production, the blockade of CD40L significantly decreased the magnitude of AH1-A5-driven CD8+ T-cell responses as compared with immunized mice treated with isotype control antibodies (, left panel; P < 0.01). A similar impairment of CD8+ T-cell responses was observed when CD40L was blocked in mice immunized with TRP2180–188 (, right panel; P < 0.0001). Due to the important role that CD8+ T cells play in the antitumor immune responses induced by peptide vaccines, we tested the requirement for CD40L in this setting. In this particular experiment based on AH1-A5 as a vaccine against CT26 cancer cells, higher survival rates were documented as compared with those observed in the experiment depicted in . Nevertheless, the blockade of CD40L significantly decreased the survival of vaccinated, tumor-bearing mice from around 90% to 50% (, left panel). CD40L-blocking antibodies also significantly inhibited the antitumor effect of TRP2180–188 in B16-OVA-bearing mice (, right panel). These results suggest that CD40L plays an important role in the induction of helper cell-independent antitumor responses elicited by potent peptide vaccines.
Figure 2. Antitumor CD8+ T-cell responses induced by potent peptide vaccines are dependent on CD40L. (A) BALB/c or C57BL/6 mice (n = 3/group) were immunized subcutaneously with 100 μg of AH1-A5 or TRP2180–188 peptide, respectively. In both cases, mice also received 0.25 mg anti-CD40L or isotype control antibodies by i.p. injection at days −2, 0, and +2 relative to the day of immunization. Ten days later, splenocytes were stimulated with the corresponding peptide and T-cell responses were measured using an IFNγ ELISPOT assay. Results correspond to the difference between antigen-stimulated and unstimulated cells in each group. Data are representative of at least 2 independent experiments. Statistical analyses were performed by Mann–Whitney U tests; ** P < 0.01, ***; P < 0.0001. (B) Mice (n = 6) vaccinated as above were challenged with CT26 or B16-OVA tumor cells 10 d after vaccination. Animal survival was monitored twice per week. Survival curves were plotted according to the Kaplan–Meier method and the log-rank test was used to measure statistical significance; * P < 0.05.
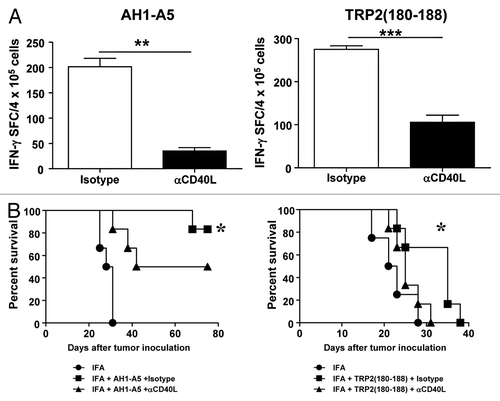
Potent peptide vaccines upregulates CD40L on CD8+ T cells
We had observed that CD40L-blocking antibodies inhibited the ability of peptides to elicit antitumor T-cell responses in the absence of CD4+ T-cell epitopes, and thus in the absence of activated CD4+ T cells. With this in mind, we surmised that the peptide-induced activation of CD8+ T cells would result in the expression of CD40L by these cells. To address this hypothesis, cells obtained from AH1-A5-immunized mice were stimulated with antigenic peptides in vitro and the expression of CD40L was analyzed 5 h later by immunostaining and flow cytometry. Immunogenic peptides indeed increased the expression of CD40L on the surface of CD8+ T cells, with the majority of CD40L-expressing CD8+ T cells being also IFNγ+ (). A similar pattern of CD40L upregulation was observed in CD8+ T lymphocytes isolated from TRP2180–188-receiving mice and stimulated with TRP2180–188 in vitro (). Interestingly, in response to this particular peptide, CD40L was also upregulated in a group of CD8+IFNγ− T cells (). CD40L expression was not detected among CD4+ T cells isolated from immunized mice and stimulated with either AH1-A5 or TRP2180–188 (), nor in cells obtained from naïve mice and stimulated in vitro with these peptides (data not shown). Of note, the CD8+ T cells obtained from AH1-A5-receiving mice and subsequently stimulated with AH1 in vitro exhibited much lower levels of CD40L than those induced in response to secondary exposure to AH1-A5 peptide (). Although we failed to detect CD4+ T-cell activation upon peptide stimulation, we wanted to discard the possibility that CD8+ T cells could acquire CD40L by trogocytosis from CD4+ T cells. To accomplish this, we performed immunization experiments in mice previously depleted of CD4 cells by anti-CD4 antibody administration. Under these circumstances, in which mice had less than 0.1% of native CD4+ T cells (data not shown), AH1-A5 and TRP2180–188 were still able to induce strong T-cell responses, as documented by IFNγ production (Fig. S2A). Importantly, the administration of antigenic peptides to splenocytes derived from CD4+ T cell-depleted mice also resulted in the expression of CD40L on CD8+ T cells (Fig. S2B).
Figure 3. Potent peptide vaccines induce the upregulation of CD40L on CD8+ T cells. (A and B) BALB/c (A) or C57BL/6 (B) mice (n = 3/group) were immunized with 100 μg of AH1-A5 or TRP2180–188 peptide, respectively. In both cases, after 10 d splenocytes derived from treated mice were cultured with or without the corresponding peptide and 5 h later IFNγ production and expression of CD40L was analyzed on CD8+ or CD4+ T cells by flow cytometry. Left, dot plots showing the results of the analysis of a representative mouse relative to a no peptide (pep) control. Right, bar graphs showing the mean ± SEM (n = 3) of a single experiment. Results are representative of 3 independent experiments. (C) Cytofluorometric analysis of expression of IFNγ and CD40L in splenocytes derived from AH1-A5-immunized mice and stimulated in vitro with peptide AH1 for 5 h. (D and E) CD8+ T cell lines specific for AH1 or AH1-A5 were stimulated for 5 h with peptide epitopes and expression IFNγ (D) or IFNγ and CD40L (E) were analyzed by immunofluorescence staining and flow cytometry. Statistical analyses were performed by Mann–Whitney U tests; * P < 0.05; ** P < 0.01.
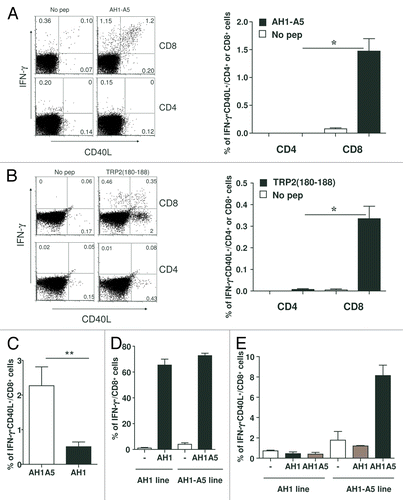
We next sought to determine whether CD40L expression was associated with CD8+ T cell activation irrespective of the nature of the immunogenic peptide or, conversely, it dependent on the strength of these epitopes. To this end, we first immunized mice with OVA257–264, which has previously shown to induce a small proportion of IFNγ-producing cells. In this case, we did not observe the upregulation of CD40L on splenocytes (Fig. 3SA). When this peptide was injected together with adjuvants such as polyinosinic–polycytidylic acid (polyI:C) and agonistic anti-CD40 antibodies, an increased proportion of IFNγ-producing cells was observed. However, even under these conditions only marginal CD40L expression was maintained (Fig. S3B). When the same approach was used to assay TRP2180–188 in combination with polyI:C and agonistic anti-CD40 antibodies, the peptide elicited not only enhanced IFNγ production (Fig. S3C; compare with ), but also increased levels of CD40L (Fig. S3D; compare with ).
In a separate set of experiments aimed at analyzing cultures enriched in antigen-specific CD8+ T cells, we took advantage of short-term T-cell lines obtained from AH1-A5-immunized mice. For comparison, we derived T-cell lines from AH1-immunized mice that had rejected CT26 tumors, as AH1 vaccination typically does not induce effective CD8+ T-cell antitumor responses. The stimulation of these T cell lines with their corresponding peptides resulted in more than 60% of CD8+ T cells that produced IFNγ regardless, of the identity of the antigenic stimulus (). However, despite the elevated antigen specificity of both these lines, AH1-A5 induced CD40L upregulation in about 8% of peptide-specific cells, but not CD40L upregulation was observed in AH1-specific CD8+IFNγ+ T cells (). Of potential importance, CD40L expression was markedly reduced when the AH1-A5-specific lymphocyte line was stimulated with AH1 ex vivo. Taken together, these results suggest that the interaction of CD8+ T cells with potent peptide vaccines induces CD40L expression, a phenotype that is not observed with weaker antigenic stimuli.
CD40L expressed by vaccine-specific CD8+ T cells induces DC maturation and co-stimulation of CD8+ T cells
Since CD40L expressed by CD4+ T helper cells is a known DC maturation factor, we studied whether the expression of CD40L on peptide-vaccine stimulated CD8+ T cells would also induce DC maturation. Splenocytes from mice immunized with AH1-A5 or TRP2180–188 were treated with the corresponding peptide in vitro and the expression of the co-stimulation and maturation marker CD86 was measured on DCs (defined as CD11c+I-Ad+ or CD11c+I-Ab+ cells). CD86 expression on DCs did not change 5 h after peptide stimulation (Fig. S4A). However, after 24 h of stimulation, CD86 was upregulated on peptide-treated, but not on untreated, DCs (). In contrast, this maturation marker was not upregulated when the splenocytes of naive mice were exposed to AH1-A5 (Fig. S4B) as well when the splenocytes of TRP2180–188-vaccinated were treated with OVA257–264 (Fig. S4C). We observed the upregulation of another DC maturation marker, CD80, in response to AH1-A5 or TRP2180–188 (Fig. S4D and E). Of note, the peptide-elicited upregulation of CD86 on DCs was partially dependent upon CD40L, as anti-CD40L antibodies had an inhibitory effect as compared with isotype-matched control antibodies (). The analysis of IL-12 production among splenocytes derived from AH1-A5-immunized mice showed that a secondary stimulation with AH1-A5 in vitro increases the number of IL-12+ DCs. However, at odds with what we observed about the CD40L-mediated upregulation of CD86 on DCs, no inhibitory effects on the levels of IL-12+ DCs were mediated by CD40L-blocking antibodies (Fig. S3F).
Figure 4. Peptide-induced CD40L expression leads to dendritic cell maturation. (A) Splenocytes from AH1-A5 or TRP2180–188-immunized mice (n = 3) were stimulated for 24 h with the corresponding peptide and CD86 expression was measured in CD11c+, I-Ad+ or I-Ab+ dendritic cells (DCs) by immunofluorescence staining and flow cytometry. (B) Splenocytes from peptide-immunized mice (n =3 ) were stimulated as above in the presence of anti-CD40L or isotype control antibodies and CD86 expression on DC was analyzed. Data are representative of 3 independent experiments. Statistical analyses were performed by Mann–Whitney U tests; * P < 0.05, **; P < 0.01)
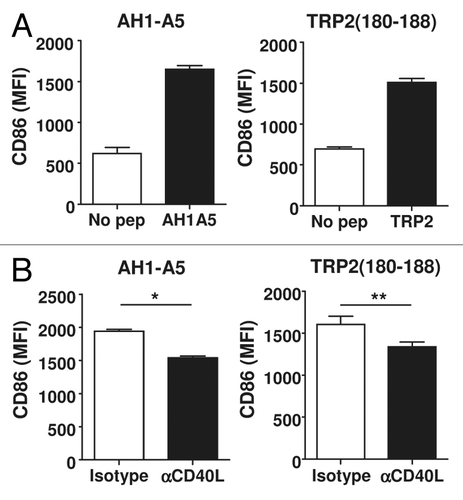
To study the biological relevance of CD40L-induced DC maturation on CD8+ T-cell responses, we measured CD8+ T-cell activation in the presence of anti-CD40L antibodies among splenocytes obtained from mice immunized with AH1-A5 or TRP2180–188. The blockade of CD40L during the exposure of splenocytes to either AH1-A5 or TRP2180–188 for 48 h clearly decreased IFNγ production (). To discard the possibility that our observations would reflect a DC maturation-unrelated, unknown role of CD40L, fully mature DCs were pulsed with AH1-A5 and used to stimulate purified CD8+ T cells derived from AH1-A5-immunized mice, either in the presence or in the absence of CD40L-blocking antibodies. Surprisingly, anti-CD40L antibodies inhibited IFNγ production by purified CD8+ T cells that had been stimulated by mature DCs (), suggesting that, besides promoting DC maturation, CD40L-CD40 interactions between CD8+ T cells may provide co-stimulatory signals. In order to test this hypothesis, the AH1-A5-specific CD8+ T cell line, a proportion of which expresses CD40 and CD40L, was exposed to plate-bound anti-CD3 antibody in vitro in the absence of antigen-presenting cells (APCs) but in the presence of Fc-CD40 or CD40L as a source of co-stimulation. CD40L, but not Fc-CD40, increased IFNγ production by CD8+ T cells exposed to anti-CD3 antibodies in a dose-dependent fashion, suggesting that CD40L-expressing cells may provide additional co-stimulatory signals to CD8+ T cells ().
Figure 5. CD40L induced by peptide stimulation amplifies CD8+ T-cell responses. (A and B) Splenocytes from AH1-A5 (A) or TRP2180–188 (B) -immunized mice (n = 3/group) were stimulated with their corresponding peptide in the presence of anti-CD40L or isotype control antibodies and IFNγ production was measured 48 h later by ELISA. (C) Flow cytometry purified CD8+ T cells from AH1-A5-immunized mice were stimulated using mature antigen presenting cells pulsed with AH1-A5 peptide in the presence of anti-CD40L blocking or isotype control antibodies and IFNγ production was measured 48 h later by ELISA. (D) CD8+ T cells obtained from an AH1-A5-specific T cell line were stimulated in vitro with plate-bound anti-CD3 antibody with or without recombinant Fc-CD40 or soluble, trimeric CD40L molecules, and IFNγ production was measured as above. Data are representative of 2 independent experiments. Statistical analyses were performed by Mann–Whitney U tests; * P < 0.05.
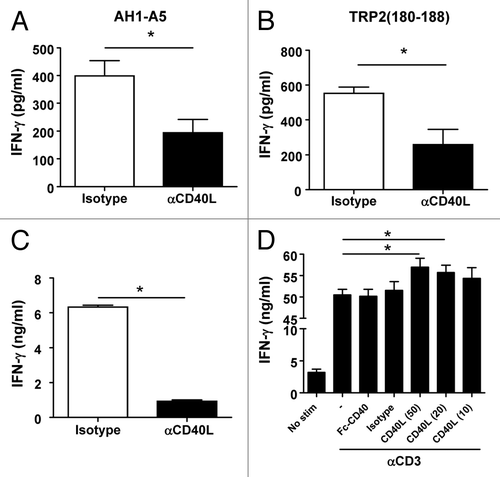
CD40L expression induced in CD8+ T cells by potent peptide vaccines confers them helper cell functional properties
The co-administration of CD4+ T-cell epitopes promotes immune responses to CD8+ T cell epitopes.Citation23,Citation24 As we had found that potent peptide vaccines elicit CD8+ T-cell responses in a helper cell-independent fashion by inducing CD40L expression, we analyzed whether the upregulation of CD40L could confer helper cell functional properties to CD8+ T cells. With this in mind, we sought to test the capacity of potent CD8+ T cell epitope peptides to boost immune responses against the poorly immunogenic CD8+ T cell epitope OVA257–264 when administered simultaneously in vivo. As previously shown (), the administration of OVA257–264 alone to B16-OVA-bearing mice induced poor immune and antitumor responses. However, the immunization of these animals with OVA257–264 in the presence of TRP2180–188 significantly enhanced OVA257–264-specific IFNγ production in T lymphocytes (; P < 0.05), an immunological response that decreased upon the blockade of CD40L (; P < 0.0001). In order to assay the activity of AH1-A5, we next immunized CB6F1 hybrid mice (expressing both H-2d and H-2b MHC class I molecules) to simultaneously present AH1-A5 and OVA257–264. Similar to the results achieved with TRP2180–188, the co-administration of AH1-A5 clearly enhanced the production of IFNγ elicited by OVA257–264 alone (), a phenomenon that was inhibited by CD40L blockade (). Taken together, these results suggest that potent CD8+ T-cell epitopes can behave as helper peptides in a CD40L-dependent manner.
Figure 6. Strong CD8+ T-cell peptide epitopes behave as helper peptides in a CD40L-dependent manner. (A–D) C57BL/6 mice (A and B) or CB6F1-hybrid mice (C and D) (n = 4/group) were immunized with 100 μg of OVA257–264 peptide epitope alone or in combination with (A and B) TRP2180–188 or (C and D) AH1-A5 peptide. In some experiments (B and D) mice receiving peptide combinations also received i.p. injection with 0.25 mg anti-CD40L blocking or isotype control antibodies at day −2, 0, and +2, with d 0 corresponding to the day of immunization. In all cases, 10 d later harvested splenocytes were stimulated with OVA257–264in vitro and T-cell responses were measured using an IFNγ ELISPOT assay. Results correspond to the difference between antigen-stimulated and unstimulated cells in each group. Data are representative of 2 independent experiments. Statistical analyses were performed by Mann–Whitney U tests; * P < 0.05; ** P < 0.01; *** P < 0.0001.
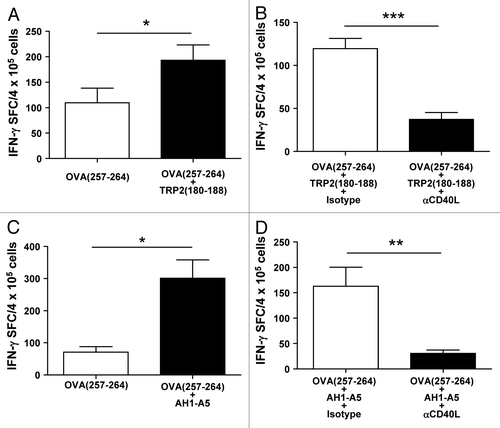
Potent peptides used in clinical trials induce CD40L expression on CD8 T+ cells
Having established that the upregulation of CD40L on antitumor CD8+ T cells responding to potent peptide vaccines contributes to their immunogenicity in murine models, we next wanted to determine the translational value of our findings. Thus, we analyzed CD40L expression on CD8+ T cells responding to peptides that had previously been shown to induce consistent CD8+ T-cell activity in cancer patients enrolled in clinical trials. Considering that most of these epitopes are presented by HLA-A2 molecules, we used human HLA-A2 and β-2 microglobulin-expressing HHD transgenic mice for our studies. As antigenic peptides, we employed the Melan A/MART-126–35 epitope (from now on EAA) and its highly immunogenic mutated variant containing a L at position 27 (from now on ELA).Citation25 We found that when these epitopes are administered in IFA, a high number of splenocytes is activated to produce IFNγ by ELA, but not by EAA (). We also found that the immunization of mice with the immunodominant peptide Tyr369, spanning tyrosinase residues 369–377,Citation26 could activate similar T-cell responses (). As shown by cytofluorometric analyses, these responses turned out to be specifically mediated by CD8+ T cells that upregulated CD40L upon peptide stimulation (), as we had observed in our murine models (). Thus, we confirmed the expression of CD40L by antigen-specific CD8+ T cells responding to highly immunogenic peptides currently used in clinical trials.
Figure 7. Potent peptides used in clinical trials induce CD40L-expressing CD8+ T cells in mice. (A and B) HHD mice (n = 3/group) transgenic for HLA-A2 were immunized with HLA-A2-restricted peptide epitope Melan A/MART-126–35 (EAA) or with its highly immunogenic mutated version containing a leucine at position 27 (ELA) (A), or with epitope 369–377 from tyrosinase (Tyr369) (B). Ten days later splenocytes from immunized mice were stimulated with or without the corresponding peptide and T-cell responses were measured using an IFNγ ELISPOT assay. (C–D) After 5 h of peptide stimulation CD8+ and CD4+ T cells were analyzed by immunofluorescence staining and flow cytometry for expression of CD40L and IFNγ. Data are representative of 2 independent experiments.
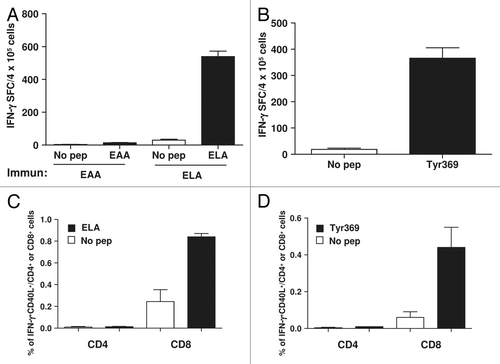
Discussion
In the present study, we considered whether CD40L could play a mechanistic role in the helper cell-independent immunogenic activity of CD8+ T cell epitope peptide vaccines. Vaccines based on the administration of CD8+ T-cell epitopes have been used with variable efficacy, but the causes of such distinct outcomes are obscure. Some synthetic peptides based on minimal CD8+ T-cell epitopes, despite the absence of DC-activating signals (adjuvants) or CD4+ T-cell epitopes, are indeed sufficient to trigger robust CD8+ T-cell responses. Several mechanismsCitation27 have been proposed to explain the ability of some peptides to activate CD8+ T lymphocytes in a helper cell-independent fashion, including: an elevated affinity for MHC moleculesCitation5 or TCRs,Citation15 the capacity to elicit abundant cytokine secretion,Citation16 and the increased frequency of peptide-specific T-cell precursors.Citation17,Citation18
In the classical paradigm, a strong interaction between APCs and CD8+ T cells is essential to activate antigen-specific immune responses. Such an interaction typically requires various soluble and contact-dependent signals provided by CD4+ T cells. One of the immunoregulatory molecules expressed by CD4+ T cells upon antigen recognition is CD40L. Here, we demonstrate both in vitro and in vivo, that the activation of CD8+ T cells by potent peptide vaccines depends on CD40L and that the levels of this molecule are increased upon peptide recognition. Although the expression of CD40L is traditionally associated with activated CD4+ helper T cells, CD40L-expressing mitogen-activated CD8+ T cells have also been reported.Citation28,Citation29 Moreover, a role for CD40/CD40L interactions has been demonstrated in optimal CD8+ T-cell activation in the absence of CD4+ lymphocytes upon immunization with antigen-pulsed DCs,Citation30 as well as in assays based on transgenic CD8+ T cells in vitro.Citation31 By a series of elegant experiments, it has been recently demonstratedCitation32 that strong TCR signals and IL-12 promote the expression of CD40L on CD8+ T cells isolated from OVA-specific OT-I transgenic mice, both in vitro and in vivo. Here, using wild-type mice (bear physiological T-cell numbers), we demonstrate that potent peptide vaccines can trigger helper cell-independent CD8+ T-cell responses that are inhibited by CD40L-blocking antibodies. The recognition of these potent peptides, as opposed to that of weaker ones, induces the expression of CD40L on CD8+, but not on CD4+, T cells. Contrarily to what observed by Stark and coworkers,Citation32 we documented poor CD8+ T-cell to OVA257–264, which induced limited levels of CD40L. These differences may be explained by the elevated amounts of precursor T cellsCitation17,Citation18 that result from the adoptive transfer of OT-I cells prior to immunization, as well as by the increased affinity of transgenic OT-I cells for their antigen relative to that of the wild-type T-cell repertoire. We also show that CD40L-inducing vaccines promote DC maturation, although this is not totally dependent on CD40L. Other CD8+ T cell-related molecules induced by antigen recognition, including cytokines like granulocyte macrophage colony-stimulating factor (GM-CSF),Citation33 may contribute to DC maturation in this setting. Indeed, CD40L blockade afforded only a partial inhibition of CD8+ T-cell responses and DC maturation, both in vivo and in vitro. Regarding IL-12, we have detected IL-12-producing DCs in AH1-A5-stimulated cultures, but this phenomenon does not seem to be contingent upon CD40L in our model. Thus, it is not completely clear which is the actual initiating factor: although CD40L expressed by CD8+ T cells, in combination with IFNγ, may lead to IL-12 production by DCs,Citation31 IL-12 and TCR signaling may induce CD40L expression, as seen in other models,Citation32 which could create a feedback loop to amplify TH1 cell responses.
Of note, some robust peptide vaccines exert CD40L-dependent functions not only when co-administered with adjuvants like IFA or Montanide, but also when admixed with other stronger adjuvants. Indeed, we have seen that TRP2180–188 combined with polyI:C and anti-CD40 agonistic antibodies induces very potent CD8+ T-cell responses, and an important fraction of these cells express CD40L (Fig. S3). In further support of this notion, it has recently been reportedCitation34 that the BiVax immunization strategy—combining selected peptides and polyI:C—generates CD8+ T-cell responses in the absence of CD4+ T cells. In this context, activation of CD8+ T lymphocytes is diminished in CD40-deficient mice, supporting a role for the CD40/CD40L signaling axis in this helper cell-independent therapeutic approach.
Blocking CD40L during the secondary stimulation of primed CD8+ T cells in vitro for 48 h lowered IFNγ production. This is in accordance with the partial decrease in DC maturation observed 24 h after blockade, a kinetic suggesting that initial antigen presentation and activation of CD8+ T cells performed by immature DCs may induce CD40L expression on these CD8+ T cells, thereby “licencing” DCs to further amplify a “second wave” of CD8+ T-cell activation. In this scenario, initial antigen recognition by CD8+ T cells would mimic the role of CD4+ helper T cells. Importantly, the partial inhibition of CD8+ T-cell activation observed upon the blockade of CD40L, which persisted even in the presence of mature DCs, suggests that other mechanisms besides DC licensing regulate CD8+ T-cell activation. Indeed, our co-stimulation experiments performed with anti-CD3 antibody in the absence of APCs demonstrate that CD40L contributes to CD8+ T-cell activation cell autonomously. In this scenario, the mutual co-stimulation of adjacent CD8+ T cells through juxtacrine CD40-CD40L interactions, resembling that proposed for CD4+ and CD8+ T cells,Citation35-Citation37 may (at least in part) underpin the potent immunogenicity of some peptides.
The overlapping role played by CD40L, be it expressed by CD8+ or CD4+ T cells, in the regulation of immune functions is further underscored by the analysis of the helper functional properties of peptides that successfully induced the expression of CD40L. Co-immunization experiments involving the weakly immunogenic peptide OVA257–264 demonstrated that potent CD8+ T-cell peptide vaccines can behave as helper epitopes. Interestingly, as in the case of CD4+ T-cell epitopes, the helper cell activity mediated by potent CD8+ T-cell vaccines is dependent upon CD40L. These results suggest that CD8+ T cell epitope peptides of sufficient potency operate as helper epitopes, mimicking the functional activities of CD4+ T-cell epitopes via a CD40L-dependent mechanism, thus allowing for the induction of CD8+ T-cell responses even in the absence of CD4+ T helper cells. Helper-like features have been attributed to CD8+ T cells in a heterologous system in which memory CD8+ T cells producing IFNγ have been shown to induce DCs to express IL-12. In turn, this resulted in the activation of new CD8+ T-cell responses against unrelated antigens,Citation38 a phenotype that has recently been observed in human and murine systems.Citation39
Multiple clinical trials have recently been performed to test short CD8+ T-cell epitopes in cancer patients.Citation40 In these settings, the administration of these peptides in IFA in the absence of Toll-like receptor (TLR) ligands was not always capable of inducing CD8+ T-cell responses. The analysis of the immunogenicity of some of these peptides in HLA-A2-expressing transgenic mice has previously demonstrated that the most immunogenic epitopes in humans are also the most immunogenic ones in mice.Citation13,Citation25 Here, we demonstrate that such highly immunogenic peptides are capable of inducing the expression of CD40L on CD8+ T cells. Thus, the ability of peptide vaccines to induce the expression of CD40L may underlie the marked variability in the therapeutic responses to these agents observed in clinical trials.
In summary, we demonstrated here that potent peptide vaccines induce CD8+ T cells to express CD40L, hence allowing for the induction of helper cell-independent antitumor responses. Since these peptides display (at least in part) immunological features of CD4+ T-cell epitopes, characterizing the ability of a candidate vaccine to induce CD8+CD40L+ T cells could provide a useful selection criterion for vaccine-based immunotherapeutic approaches. The identification of optimal peptides for vaccination strategies is important to treat not only cancer but also other diseases driven or associated with specific antigens, particularly in settings in which CD4+ T-cell functions are compromised.
Materials and Methods
Reagents and cancer cell lines
The peptides AH1, AH1-A5, TRP2180–188, OVA257–264, and EAA were purchased from Genecust and had a purity above 98%. as determined by high pressure liquid chromatography (HPLC) and mass spectrometry. CD40L-blocking (BE-0017–1) or hamster isotype-matched (IgG) control antibodies (BE-0091) were obtained from BioXCell. CT26 colon cancer cells, obtained from ATCC, were grown in complete medium (CM): RPMI medium containing 10% fetal bovine serum (FBS), 4 mM glutamine, 100 U/mL penicillin, 100 µg/mL streptomycin and 50 µM 2-mercaptoethanol). B16-OVA melanoma cells, obtained from Dr G Kroemer, were grown in DMEM containing 10% FBS and antibiotics. All antibodies and reagents were from BD-Biosciences unless otherwise stated.
Mouse strains and transgenic mice
BALB/c, C57BL/6, and CB6F1 mice were obtained from Harlan. B2mtm1Unc H2-Ab1tm1Doi Tg(HLA-A2/H2-D/B2M)1Bpe Tg(HLA-DR1)/Orl (HHD-DR1) mice,Citation41 transgenic for human HLA-A2 and HLA-DR1 molecules, were obtained from Dr F Lemmonier. Mice were maintained in pathogen-free conditions according to the guidelines for animal care of our Institutional Review Board.
Immunization
Mice were injected subcutaneously with 100 μg of the CD8+ T-cell epitope to be tested emulsified in IFA. In co-immunization experiments, 100 μg of OVA257–264 were also co-administered. Inhibition experiments in vivo were performed by i.p. injection of 0.25 mg of anti-CD40L or isotype-matched antibodies at day -2, 0, and +2, with d 0 corresponding to the day of immunization. In some cases, mice were depleted of CD4 cells by three i.p. injections on days -2, -1 and 0 with 0.3 mg of GK1.5 anti-CD4 antibodies.
Peptide stimulation of primed immune cells to evaluate cytokine production ex vivo
Ten days after immunization mice were sacrificed and their spleens were removed for immunological analyses. Splenocytes were resuspended in CM and plated at 8 × 105 cells/well in 0.2 mL in U-bottomed 96-well plates with or without 10 μM peptide. Two days later, supernatants were harvested and IFNγ was measured by ELISA (555138; BD-Biosciences). In selected experiments, 105 CD8+ T cells that had been purified from splenocytes of immunized mice using a negative selection kit (130–095–236; MiltenyiBiotec) and a cell sorter (FACSAria, BD-Biosciences) were stimulated with 5 × 105 mature APCs. APCs were obtained by culturing splenocytes from naive mice over a monolayer of CD40L-transfected NIH-3T3 fibroblasts for 1 d. In some wells, CD40L-blocking or isotype-matched, control antibodies were also added at 25 μg/mL. In experiments analyzing co-stimulation provided by interaction of CD40/CD40L, 105 T cells from the AH1-A5-specific CD8+ T cell line were stimulated with plate-bound anti-CD3 antibodies (BD-Biosciences) in the absence or presence of plate-bound recombinant Fc-CD40 (50324-M03H-5; Life Technologies) or 10–50 μg/mL soluble trimeric CD40L.Citation42 In other experiments IFNγ-producing cells were enumerated after stimulation of 4 × 105 splenocytes as above using an ELISPOT set (551083; BD-Biosciences) according to manufacturer’s instructions.
Flow cytometry
For analysis of T cells, splenocytes were incubated for 5 h with or without 10 μg/mL of the corresponding peptide in the presence of Fc Block™ (553142), anti-CD40L-APC (17–1541–81; eBiosciences) and 2 μg/mL GolgiStop (554724). Next, cells were stained in phosphate buffered saline (PBS) containing 2% FBS with anti-CD3-PECy5 (MCA2690C; AbD Serotec) or anti-CD3-FITC (553061) and anti-CD8-PeCy7 (552877), or anti-CD8-Pacific Blue (MCA609PBT; AbD Serotec) and anti-CD4-Brilliant Violet 570 (100541; Biolegend) or anti-CD4-Alexa Fluor 647 (557681) for 15 min at 4 °C. Finally, cells were fixed and permeabilized using a Cytofix/Cytoperm kit (554714; BD Biosciences) for 15 min at 4 °C and then stained with anti–IFNγ-PE (554412). In some cases, anti-TNF-PeCy7 (557644) and anti-IL-2-APC (554429) were also included. DC maturation was analyzed after incubating 2 × 106 cells during 24 h with or without the corresponding peptide at 37 °C in CM. In some experiments, 25 μg/mL CD40L-blocking or isotype-matched control antibodies were also added to the culture. Cells were then stained with APC-labeled anti-CD11c (550261) and anti-I-Ad-PE (553548) or anti-I-Ab-PE (06355A) antibodies in combination with FITC-labeled anti-CD80 (553768) or anti-CD86 (553691) antibodies. All antibodies and reagents were from BD-Biosciences unless otherwise stated. Samples were analyzed using a FACS Canto flow cytometer (BD-Biosciences).
Generation of T-cell lines
Splenocytes from mice immunized with AH1-A5 or from mice in which CT26 tumors had regressed were plated at 7.5 × 106 cells/well in 1.5 mL of CM in the presence of 10 μg/mL AH1-A5 or AH1, respectively. Five days later, 25 U/mL of IL-2 was added and cells were cultured for 2 additional days. On d 7 and thereafter, 106 growing cells were weekly stimulated with 5 × 106 irradiated peptide-pulsed splenocytes in the presence of 100 U/mL of IL-2. T-cell lines were routinely tested 1 wk after the last stimulation.
Allograft experiments
Ten days after administration of peptide vaccines, tumor challenge was performed by subcutaneous injection of either 5 × 105 CT26 cells into BALB/c recipient mice or 105 B16-OVA cells into C57BL/6 recipient mice. Tumor volume was calculated according to the formula: V = (length × widthCitation2)/2. Mice were sacrificed when the tumor diameter reached 17 mm.
Statistical analyses
Survival curves of animals treated with different protocols were plotted according to the Kaplan–Meier method and the log-rank test was used to measure statistical significance. Statistical analyses of immune responses were performed by nonparametric Kruskal–Wallis and Mann–Whitney U tests. In all cases, P < 0.05 was considered statistically significant.
Abbreviations: | ||
CM | = | complete medium |
DC | = | dendritic cell |
IFA | = | incomplete Freund’s adjuvant |
IFNγ | = | interferon γ |
SFC | = | spot-forming cell |
TCR | = | T-cell receptor |
Additional material
Download Zip (124.9 KB)Disclosure of Potential Conflicts of Interest
No potential conflicts of interest were disclosed.
Acknowledgments
The authors thank F Lemonnier for providing HHD-DR1 mice. This study is supported by “UTE project CIMA” to all authors. PS and JJL are recipients of grants SAF2010–15074 and SAF2010–15060, respectively, from Ministerio de Ciencia e Innovación. This work is dedicated to the memory of our friend and colleague F Borrás-Cuesta.
Supplemental Materials
Supplemental materials may be found here: http://www.landesbioscience.com/journals/oncoimmunology/article/27009
Citation: Llopiz D, Huarte E, Ruiz M, Bezunartea J, Belsue V, Zabaleta A, Lasarte J, Prieto J, Borrás-Cuesta F, Sarobe P. Helper cell-independent antitumor activity of potent CD8+ T cell epitope peptide vaccines is dependent upon CD40L. OncoImmunology 2013; 2:e27009; 10.4161/onci.27009
References
- Vacchelli E, Martins I, Eggermont A, Fridman WH, Galon J, Sautès-Fridman C, Tartour E, Zitvogel L, Kroemer G, Galluzzi L. Trial watch: Peptide vaccines in cancer therapy. Oncoimmunology 2012; 1:1557 - 76; http://dx.doi.org/10.4161/onci.22428; PMID: 23264902
- Tindle RW, Fernando GJ, Sterling JC, Frazer IHA. A “public” T-helper epitope of the E7 transforming protein of human papillomavirus 16 provides cognate help for several E7 B-cell epitopes from cervical cancer-associated human papillomavirus genotypes. Proc Natl Acad Sci U S A 1991; 88:5887 - 91; http://dx.doi.org/10.1073/pnas.88.13.5887; PMID: 1712110
- Feltkamp MC, Smits HL, Vierboom MP, Minnaar RP, de Jongh BM, Drijfhout JW, ter Schegget J, Melief CJ, Kast WM. Vaccination with cytotoxic T lymphocyte epitope-containing peptide protects against a tumor induced by human papillomavirus type 16-transformed cells. Eur J Immunol 1993; 23:2242 - 9; http://dx.doi.org/10.1002/eji.1830230929; PMID: 7690326
- Ridge JP, Di Rosa F, Matzinger P. A conditioned dendritic cell can be a temporal bridge between a CD4+ T-helper and a T-killer cell. Nature 1998; 393:474 - 8; http://dx.doi.org/10.1038/30989; PMID: 9624003
- Franco A, Tilly DA, Gramaglia I, Croft M, Cipolla L, Meldal M, Grey HM. Epitope affinity for MHC class I determines helper requirement for CTL priming. Nat Immunol 2000; 1:145 - 50; http://dx.doi.org/10.1038/77827; PMID: 11248807
- Jäeger E, Bernhard H, Romero P, Ringhoffer M, Arand M, Karbach J, Ilsemann C, Hagedorn M, Knuth A. Generation of cytotoxic T-cell responses with synthetic melanoma-associated peptides in vivo: implications for tumor vaccines with melanoma-associated antigens. Int J Cancer 1996; 66:162 - 9; http://dx.doi.org/10.1002/(SICI)1097-0215(19960410)66:2<162::AID-IJC4>3.0.CO;2-0; PMID: 8603805
- Salgaller ML, Marincola FM, Cormier JN, Rosenberg SA. Immunization against epitopes in the human melanoma antigen gp100 following patient immunization with synthetic peptides. Cancer Res 1996; 56:4749 - 57; PMID: 8840994
- Pass HA, Schwarz SL, Wunderlich JR, Rosenberg SA. Immunization of patients with melanoma peptide vaccines: immunologic assessment using the ELISPOT assay. Cancer J Sci Am 1998; 4:316 - 23; PMID: 9815296
- Rosenberg SA, Yang JC, Schwartzentruber DJ, Hwu P, Marincola FM, Topalian SL, Restifo NP, Dudley ME, Schwarz SL, Spiess PJ, et al. Immunologic and therapeutic evaluation of a synthetic peptide vaccine for the treatment of patients with metastatic melanoma. Nat Med 1998; 4:321 - 7; http://dx.doi.org/10.1038/nm0398-321; PMID: 9500606
- Jäger E, Gnjatic S, Nagata Y, Stockert E, Jäger D, Karbach J, Neumann A, Rieckenberg J, Chen YT, Ritter G, et al. Induction of primary NY-ESO-1 immunity: CD8+ T lymphocyte and antibody responses in peptide-vaccinated patients with NY-ESO-1+ cancers. Proc Natl Acad Sci U S A 2000; 97:12198 - 203; http://dx.doi.org/10.1073/pnas.220413497; PMID: 11027314
- Muderspach L, Wilczynski S, Roman L, Bade L, Felix J, Small LA, Kast WM, Fascio G, Marty V, Weber J. A phase I trial of a human papillomavirus (HPV) peptide vaccine for women with high-grade cervical and vulvar intraepithelial neoplasia who are HPV 16 positive. Clin Cancer Res 2000; 6:3406 - 16; PMID: 10999722
- Weber J, Sondak VK, Scotland R, Phillip R, Wang F, Rubio V, Stuge TB, Groshen SG, Gee C, Jeffery GG, et al. Granulocyte-macrophage-colony-stimulating factor added to a multipeptide vaccine for resected Stage II melanoma. Cancer 2003; 97:186 - 200; http://dx.doi.org/10.1002/cncr.11045; PMID: 12491520
- Liénard D, Rimoldi D, Marchand M, Dietrich PY, van Baren N, Geldhof C, Batard P, Guillaume P, Ayyoub M, Pittet MJ, et al. Ex vivo detectable activation of Melan-A-specific T cells correlating with inflammatory skin reactions in melanoma patients vaccinated with peptides in IFA. Cancer Immun 2004; 4:4; PMID: 15149168
- Cormier JN, Salgaller ML, Prevette T, Barracchini KC, Rivoltini L, Restifo NP, Rosenberg SA, Marincola FM. Enhancement of cellular immunity in melanoma patients immunized with a peptide from MART-1/Melan A. Cancer J Sci Am 1997; 3:37 - 44; PMID: 9072306
- Heath WR, Kjer-Nielsen L, Hoffmann MW. Avidity for antigen can influence the helper dependence of CD8+ T lymphocytes. J Immunol 1993; 151:5993 - 6001; PMID: 8245444
- Wiesel M, Kratky W, Oxenius A. Type I IFN substitutes for T cell help during viral infections. J Immunol 2011; 186:754 - 63; http://dx.doi.org/10.4049/jimmunol.1003166; PMID: 21160039
- Wang B, Norbury CC, Greenwood R, Bennink JR, Yewdell JW, Frelinger JA. Multiple paths for activation of naive CD8+ T cells: CD4-independent help. J Immunol 2001; 167:1283 - 9; PMID: 11466344
- Mintern JD, Davey GM, Belz GT, Carbone FR, Heath WR. Cutting edge: precursor frequency affects the helper dependence of cytotoxic T cells. J Immunol 2002; 168:977 - 80; PMID: 11801627
- Casares N, Lasarte JJ, de Cerio AL, Sarobe P, Ruiz M, Melero I, Prieto J, Borrás-Cuesta F. Immunization with a tumor-associated CTL epitope plus a tumor-related or unrelated Th1 helper peptide elicits protective CTL immunity. Eur J Immunol 2001; 31:1780 - 9; http://dx.doi.org/10.1002/1521-4141(200106)31:6<1780::AID-IMMU1780>3.0.CO;2-I; PMID: 11385623
- Slansky JE, Rattis FM, Boyd LF, Fahmy T, Jaffee EM, Schneck JP, Margulies DH, Pardoll DM. Enhanced antigen-specific antitumor immunity with altered peptide ligands that stabilize the MHC-peptide-TCR complex. Immunity 2000; 13:529 - 38; http://dx.doi.org/10.1016/S1074-7613(00)00052-2; PMID: 11070171
- Casares N, Arribillaga L, Sarobe P, Dotor J, Lopez-Diaz de Cerio A, Melero I, Prieto J, Borrás-Cuesta F, Lasarte JJ. CD4+/CD25+ regulatory cells inhibit activation of tumor-primed CD4+ T cells with IFN-gamma-dependent antiangiogenic activity, as well as long-lasting tumor immunity elicited by peptide vaccination. J Immunol 2003; 171:5931 - 9; PMID: 14634104
- Ahlers JD, Belyakov IM, Thomas EK, Berzofsky JA. High-affinity T helper epitope induces complementary helper and APC polarization, increased CTL, and protection against viral infection. J Clin Invest 2001; 108:1677 - 85; PMID: 11733563
- Lasarte JJ, Sarobe P, Gullón A, Prieto J, Borrás-Cuesta F. Induction of cytotoxic T lymphocytes in mice against the principal neutralizing domain of HIV-1 by immunization with an engineered T-cytotoxic-T-helper synthetic peptide construct. Cell Immunol 1992; 141:211 - 8; http://dx.doi.org/10.1016/0008-8749(92)90140-K; PMID: 1532538
- Shirai M, Pendleton CD, Ahlers J, Takeshita T, Newman M, Berzofsky JA. Helper-cytotoxic T lymphocyte (CTL) determinant linkage required for priming of anti-HIV CD8+ CTL in vivo with peptide vaccine constructs. J Immunol 1994; 152:549 - 56; PMID: 8283036
- Valmori D, Fonteneau JF, Lizana CM, Gervois N, Liénard D, Rimoldi D, Jongeneel V, Jotereau F, Cerottini JC, Romero P. Enhanced generation of specific tumor-reactive CTL in vitro by selected Melan-A/MART-1 immunodominant peptide analogues. J Immunol 1998; 160:1750 - 8; PMID: 9469433
- Skipper JC, Hendrickson RC, Gulden PH, Brichard V, Van Pel A, Chen Y, Shabanowitz J, Wolfel T, Slingluff CL Jr., Boon T, et al. An HLA-A2-restricted tyrosinase antigen on melanoma cells results from posttranslational modification and suggests a novel pathway for processing of membrane proteins. J Exp Med 1996; 183:527 - 34; http://dx.doi.org/10.1084/jem.183.2.527; PMID: 8627164
- Malek TR. T helper cells, IL-2 and the generation of cytotoxic T-cell responses. Trends Immunol 2002; 23:465 - 7; http://dx.doi.org/10.1016/S1471-4906(02)02308-6; PMID: 12297409
- Roy M, Waldschmidt T, Aruffo A, Ledbetter JA, Noelle RJ. The regulation of the expression of gp39, the CD40 ligand, on normal and cloned CD4+ T cells. J Immunol 1993; 151:2497 - 510; PMID: 8103067
- Cronin DC 2nd, Stack R, Fitch FW. IL-4-producing CD8+ T cell clones can provide B cell help. J Immunol 1995; 154:3118 - 27; PMID: 7897202
- Hernandez MG, Shen L, Rock KL. CD40-CD40 ligand interaction between dendritic cells and CD8+ T cells is needed to stimulate maximal T cell responses in the absence of CD4+ T cell help. J Immunol 2007; 178:2844 - 52; PMID: 17312128
- Wong KL, Lew FC, MacAry PA, Kemeny DM. CD40L-expressing CD8 T cells prime CD8alpha(+) DC for IL-12p70 production. Eur J Immunol 2008; 38:2251 - 62; http://dx.doi.org/10.1002/eji.200838199; PMID: 18600823
- Stark R, Hartung A, Zehn D, Frentsch M, Thiel A. IL-12-mediated STAT4 signaling and TCR signal strength cooperate in the induction of CD40L in human and mouse CD8+ T cells. Eur J Immunol 2013; 43:1511 - 7; http://dx.doi.org/10.1002/eji.201243218; PMID: 23765345
- Min L, Mohammad Isa SA, Shuai W, Piang CB, Nih FW, Kotaka M, Ruedl C. Cutting edge: granulocyte-macrophage colony-stimulating factor is the major CD8+ T cell-derived licensing factor for dendritic cell activation. J Immunol 2010; 184:4625 - 9; http://dx.doi.org/10.4049/jimmunol.0903873; PMID: 20357255
- Cho HI, Barrios K, Lee YR, Linowski AK, Celis E. BiVax: a peptide/poly-IC subunit vaccine that mimics an acute infection elicits vast and effective anti-tumor CD8 T-cell responses. Cancer Immunol Immunother 2013; 62:787 - 99; http://dx.doi.org/10.1007/s00262-012-1382-6; PMID: 23266830
- Bourgeois C, Rocha B, Tanchot C. A role for CD40 expression on CD8+ T cells in the generation of CD8+ T cell memory. Science 2002; 297:2060 - 3; http://dx.doi.org/10.1126/science.1072615; PMID: 12242444
- Xydia M, Ge Y, Quitsch U, Beckhove P. CD40L co-stimulation from CD8+ to CD4+ effector memory T cells supports CD4+ expansion. Immunol Cell Biol 2011; 89:670 - 80; http://dx.doi.org/10.1038/icb.2010.153; PMID: 21151195
- de Goër de Herve MG, Dembele B, Vallée M, Herr F, Cariou A, Taoufik Y. Direct CD4 help provision following interaction of memory CD4 and CD8 T cells with distinct antigen-presenting dendritic cells. J Immunol 2010; 185:1028 - 36; http://dx.doi.org/10.4049/jimmunol.0904209; PMID: 20562265
- Nakamura Y, Watchmaker P, Urban J, Sheridan B, Giermasz A, Nishimura F, Sasaki K, Cumberland R, Muthuswamy R, Mailliard RB, et al. Helper function of memory CD8+ T cells: heterologous CD8+ T cells support the induction of therapeutic cancer immunity. Cancer Res 2007; 67:10012 - 8; http://dx.doi.org/10.1158/0008-5472.CAN-07-1735; PMID: 17942935
- Frentsch M, Stark R, Matzmohr N, Meier S, Durlanik S, Schulz AR, Stervbo U, Jürchott K, Gebhardt F, Heine G, et al. CD40L expression permits CD8+ T cells to execute immunologic helper functions. Blood 2013; 122:405 - 12; http://dx.doi.org/10.1182/blood-2013-02-483586; PMID: 23719298
- Speiser DE, Pittet MJ, Rimoldi D, Guillaume P, Luescher IF, Liénard D, Lejeune F, Cerottini JC, Romero P. Evaluation of melanoma vaccines with molecularly defined antigens by ex vivo monitoring of tumor-specific T cells. Semin Cancer Biol 2003; 13:461 - 72; http://dx.doi.org/10.1016/j.semcancer.2003.09.010; PMID: 15001165
- Pascolo S, Bervas N, Ure JM, Smith AG, Lemonnier FA, Pérarnau B. HLA-A2.1-restricted education and cytolytic activity of CD8(+) T lymphocytes from beta2 microglobulin (beta2m) HLA-A2.1 monochain transgenic H-2Db beta2m double knockout mice. J Exp Med 1997; 185:2043 - 51; http://dx.doi.org/10.1084/jem.185.12.2043; PMID: 9182675
- Echeverria I, Pereboev A, Silva L, Zabaleta A, Riezu-Boj JI, Bes M, Cubero M, Borras-Cuesta F, Lasarte JJ, Esteban JI, et al. Enhanced T cell responses against hepatitis C virus by ex vivo targeting of adenoviral particles to dendritic cells. Hepatology 2011; 54:28 - 37; http://dx.doi.org/10.1002/hep.24325; PMID: 21452282