Abstract
Key physiological functions of the intestine are governed by nerves and neurotransmitters. This complex control relies on two neuronal systems: an extrinsic innervation supplied by the two branches of the autonomic nervous system and an intrinsic innervation provided by the enteric nervous system. As a result of constant exposure to commensal and pathogenic microflora, the intestine developed a tightly regulated immune system. In this review, we cover the current knowledge on the interactions between the gut innervation and the intestinal immune system. The relations between extrinsic and intrinsic neuronal inputs are highlighted with regards to the intestinal immune response. Moreover, we discuss the latest findings on mechanisms underlying inflammatory neural reflexes and examine their relevance in the context of the intestinal inflammation. Finally, we discuss some of the recent data on the identification of the gut microbiota as an emerging player influencing the brain function.
Introduction
Key functions of the gastrointestinal tract such as motility, secretion and vasoregulation are regulated by neural mechanisms provided by the autonomic nervous system (ANS). The extrinsic control of the intestine is provided by two branches: the sympathetic and parasympathetic branches. Sympathetic preganglionic fibers arise from cholinergic neurons located in the thoracolumbar intermediolateral nucleus of the spinal cord and synapse with noradrenergic postganglionic neurons in para- and prevertebral ganglia. Parasympathetic preganglionic fibers originate from motor neurons of the dorsal nucleus of the vagus (DMV) and make synaptic contact with postganglionic neurons within the intestine (i.e., the myenteric plexus) (). The density of the vagal innervation displays a proximodistal gradient along the intestine with the highest density observed in the duodenum and the lowest density observed in the distal part of the ileum.Citation1 The large intestine differs from the rest of the gastrointestinal tract as it receives a parasympathetic innervation from two distinct sources. The vagus nerve innervates the proximal colon whereas preganglionic neurons located in parasympathetic nuclei of sacral vertebrae (S2 to 4) contact post-ganglionic neurons in pelvic ganglia. These postganglionic neurons then give rise to rectal nerves providing parasympathetic input to the distal colon. Of note, in some species, direct sacral parasympathetic innervation of the distal colon is also provided by nerves arising from sacral parasympathetic nuclei.Citation2
Figure 1. Innervation and interactions between nervous and immune system in the intestine. (A) Sympathetic (red) and vagal (blue) innervation of the small and large intestine. Sympathetic postganglionic neurons are found in prevertebral ganglia while a pool of enteric neurons (in green) constitutes postganglionic neurons of the vagal innervation. The colon receives two parasympathetic inputs: one of them originates from the brainstem (DMV) while the other rises from sacral parasympathetic nuclei. Of note, the distal colon receives either a direct sacral parasympathetic innervation or an indirect innervation (synapse with postganglionic neurons located in pelvic ganglia, not shown). (B) Vagal inputs (in blue) solely innervate myenteric neurons. Sympathetic inputs (in red) make synaptic contact with enteric neurons and immune cells in both smooth muscle layers and PPs. Enteric fibers (in green) also project to PPs and are found in the close vicinity of macrophages. CM, circular muscularis; LM, longitudinal muscularis; MP, myenteric plexus; SP, submucosal plexus
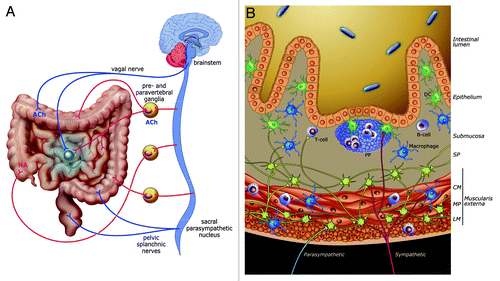
In addition to the extrinsic neuronal control, the gastrointestinal tract possesses an intrinsic nervous system, the Enteric Nervous System (ENS), able to regulate intestinal motility independently of the presence of the central nervous system (CNS).Citation3 The ENS is composed of 2 plexuses: the myenteric plexus located between the longitudinal and circular muscle layers of the muscularis externa and the submucosal plexus.
A tightly regulated immune system has developed in the intestine in response to a constant exposure to pathogenic and commensal microbiota. This system allows microbe sampling and surveillance and a quick response to microbial threat. The CNS, supported by the proximity of its terminals to immune cells, plays a major role in the regulation of this level of host defense. These interactions between nervous and immune system allow the modulation of the inflammatory response and offer new therapeutic targets to tackle inflammatory disorders where a deregulation of the immune reaction constitutes the key element.
In this review, we address the impact of neuro-immune interactions in the context of the intestine. The relevance of neural reflexes is discussed with regards to the regulation of intestinal inflammatory disorders. Finally, the capacity of the intestinal microbiota to influence the bi-directional communication of the gut-brain axis and its relevance in the treatment of inflammatory bowel disease (IBD) is highlighted.
Intestinal Neuro-immune Interactions
Sympathetic innervation of the gut and interactions with immune cells
The sympathetic regulation of the immune response relies on the binding of noradrenalin (NA) with α (subtypes α1 and α2) and β (subtypes β1, β2, β3) adrenergic receptors. With a half-life of 1 to 2 min and a capacity to diffuse on a long distance (up to 1 μm),Citation4 a wide range of cell types can be affected by NA release without need of direct contact with neuronal fibers.Citation5 Sympathetic efferent fibers densely innervate the intestine (). Terminals are found in the serosaCitation6 and the mucosa and an important network of sympathetic fibers contacts neurons of the myenteric plexus.Citation7,Citation8 Networks of fibers are also found in the circular muscularis while some fibers pass through the longitudinal muscle layer.Citation9,Citation10 Evidence of contacts between sympathetic terminals and non-vascular intestinal myocytes is however lacking. This innervation of the different intestinal compartments by noradrenergic fibers may therefore affect many immune cell types and thereby influence the innate and adaptive immune response. A good example of sympathetic immune regulation via such contacts is found in the lymphoid system of the gut.
The intestinal mucosa exhibits a dense network of immune cells particularly in organized lymphoid structures such as Peyer’s patches (PPs). This component of the gut-associated lymphoid tissue (GALT), constituted by B and T-cell follicles and a subepithelial dome rich in dendritic cells (DCs), participates in the response to microbial threats. The presence of noradrenergic fibers was reported in PPs implying that the sympathetic nerve could play a role in the regulation of the immune response. Noradrenergic axonal fibers are found in close proximity to DCs,Citation11 plasma cells and in T cell zones.Citation12 NA can affect DCs migration as well as their cytokine production (i.e., IL12, IL-6Citation13) through the binding to adrenoceptors (β2Citation13, α1,Citation14 α2Citation15,Citation16). The potential of NA to dictate the cytokine profile secreted by DCs allows the sympathetic innervation to affect the skewing of naïve T cells and thereby indirectly influences the shaping of the adaptive immune response.
In vitro studies reported that NA can influence the skewing of naïve T cells expressing β-adrenoceptors and thereby their cytokine secretion (IFN-γ, IL-12 for Th1 and IL-4 for Th2) (for a review see ref. Citation17). B cell proliferationCitation18 and immunoglobulin secretionCitation19 are triggered by NA treatment through β2-adrenoceptors signaling. In vivo studies using 6-OHDA (a chemical sympathectomy) confirmed the effects of NA on T and B cellsCitation20 and revealed the capacity of NA to modulate the migration and accumulation of lymphocytes in the GALTCitation21 during inflammation. Of note, the method used to deplete sympathetic inputs presents limitations as chemical sympathectomy not only depletes the noradrenergic input to the intestine but also affects the catecholamine content of immune cells.Citation22 Additional in vivo approaches are therefore required to determine the exact contribution of the intestinal sympathetic innervation in the modulation of the immune system.
The intestinal muscularis represents another compartment where neuro-immune interactions are observed. Recently, a direct contact between varicosities of sympathetic axons and resident intestinal macrophages was reported.Citation23 NA, through β-adrenoceptors signaling, can suppress TNF-α secretionCitation24 and phagocytosis of macrophagesCitation25 thereby affecting their clearance capacity. Of note, sympathetic fibers synapsing with motor neurons of the enteric nervous systemCitation26,Citation27 could modulate immune functions via an indirect effect on enteric neurons.
Vagal innervation of the gut and interactions with immune cells
The vagus nerve modulates immune cells through the release of Acetylcholine (ACh). In contrast to catecholamines, ACh exhibits a very short half-life of 1 to 2 ms, due to the presence of ACh esterase which hydrolyzes ACh at a high rate. Close contact between cholinergic nerve terminals and cells expressing ACh receptors is therefore required for the cholinergic control of these cells.
Receptors for Ach are of two types: muscarinic receptors (mAChR) comprising of 5 subtypes (M1-M5) and nicotinic receptors (nAChR) constituted of homomeric or heteromeric combinations of 5 subunits (among 17 subunits. On a cellular level, ACh binding on one or the other type of receptors leads to significantly different mechanisms. Activation of muscarinic receptors, belonging to the G-protein-coupled receptors, leads to the activation of a cascade of secondary messengers only allowing a slow response from the target cell.Citation28 On the contrary, nicotinic receptors belonging to the ligand-gated ion channel family allow a fast transmission of the cholinergic signal.Citation29,Citation30 In immune cells however, these variations in transmission don’t imply differences in the onset or length of the cholinergic signal as both nicotinic and muscarinic receptors trigger long-lasting effects.Citation31
The vast majority of immune cells express one or both types of receptors. Bone-Marrow-derived Dendritic cells, as well as PBMC-derivedCitation32 and peritoneal macrophagesCitation33 express both mAChR (M1-M5) and nAChR (α2, α5, α6, α7, α10, β2). T and B cells also exhibit receptors for ACh (nAChR α2, α5, α9, α10, β1, β2, β4 and M1, M3, M4, M5 mAChR for T cells; α4, α7, β2 nAChR and M2 mAChR for B cells).Citation33 Interestingly, the expression of these different subunits on both innate and adaptive immune cells varies with their maturation and development stage. Intracellular signaling triggered by the binding of ACh to these receptors has been shown to modify greatly the function of these cells. In vitro studies demonstrated the capacity of ACh to modulate both the innate and adaptive compartments of the immune system. α7nAChR agonists suppress TNF-α secretion by peritoneal macrophages exposed to LPS. Moreover, treatment of freshly isolated murine T cells with both agonists and antagonists of nAChR and mAChR interferes with their skewing toward Th1, Th2 and Th17 profiles.Citation31 Finally, a suppressive effect of nicotine on B cells activation was reported and is mediated by α2, α4 and β2 subunits of nAChR.Citation34,Citation35 Importantly, no contact between vagal terminals and immune cells in the intestine has so far been reported. However, preganglionic efferent terminals make synaptic contact with neurons of the myenteric gangliaCitation36-Citation38 indicating that neural cholinergic influence on immune cells is solely provided by myenteric neurons (). Importantly, T and B cells were recently shown as a crucial source of Ach able to modulate the immune response.Citation39,Citation40
The enteric nervous system and interactions with immune cells
Enteric neurons display a wide variety of neurotransmitters and neuropeptides determining the chemical coding of the ENS (for a review see refs. Citation41 and Citation42). Enteric neurotransmitters and neuropeptides are able to bind and influence a variety of immune cells.
Cholinergic enteric neurons are targeted by both branches of the ANS.Citation36,Citation43,Citation44 Evidence of direct interactions between ‘basket-like” cholinergic endings and cholinergic myenteric neurons was recently reported (own unpublished data).Citation38 This vagal innervation of cholinergic enteric neurons stimulates the production of ACh and may represent the neuronal circuitry by which the vagus nerve mediates its anti-inflammatory signal. ChAT+ fibers, most likely originating from the enteric neurons, are found in close proximity to resident intestinal macrophages.Citation45 Phillips and Powley recently brought evidence of direct interactions between cholinergic fibers (i.e., axons and dendrites) and macrophages in the gut muscularis.Citation23 This neuronal source of ACh can suppress macrophage activity through its binding to α7nAChR, and can thereby inhibit the release of pro-inflammatory cytokines.Citation45 On the contrary, sympathetic inputs to cholinergic enteric neurons inhibit ACh release through the binding of NA to α2 adrenoceptors.Citation46,Citation47 This observation suggests that sympathetic inputs may counterbalance the cholinergic anti-inflammatory effect.
Of note, cholinergic enteric fibers are not restricted to the muscle layers. Some ChAT+ fibers are found in PPs, suggesting a possible cholinergic modulation of the immune reaction.Citation48 However, no neuronal contact with immune cells has been reported so far in PPs. Determining what immune cells are contacted by cholinergic fibers in PPs is of crucial importance to better understand the interplay between cholinergic and immune system in the intestinal inflammatory response to microbial threats.
Vasoactive intestinal peptide (VIP), a well-established immunomodulator (for review see ref. Citation49), is expressed by enteric neurons. Sympathetic and vagal efferent fibers make contact with VIP+ enteric neurons providing another neuronal mediator by which the brain can affect the gut immune response. VIP+ fibers can be found in close proximity to resident macrophages expressing VIP receptor VPAC1 (own unpublished data). In vitro and in vivo studies revealed the suppressive action of VIP on their activity as it inhibits their chemokine and cytokine production (e.g., MIP-1, MIP-2, KC and IL-12) during endotoxemia.Citation50 In addition, VIP treatment modulates DCs activityCitation51 in vitro and VPAC1 and VPAC2 expression was observed on DCs isolated from PPs.Citation52 The modulatory effect of VIP on those DCs could potentially influence the IgA production by B cells, in addition to its direct effect already reported.Citation53 These results imply that VIP released by enteric neurons could interfere with both innate and adaptive immune systems.
In addition, both vagalCitation38 (own unpublished data) and sympathetic fibersCitation54 target nitrergic neurons in the ENS. Nitric oxide (NO) exerts both protective (e.g., T cell suppressionCitation55) and pro-inflammatory effects (e.g., increase in IFN-γ production by natural killer cellsCitation56) and can greatly affect the inflammatory reaction (for review see ref. Citation57). Nitrergic fibers are found in the close vicinity of macrophages (own unpublished data) placing it as an addition neuromodulator of the innate immune system.
Serotoninergic enteric neurons receive inputs from both autonomic branches.Citation26,Citation52,Citation53 Serotonin triggers the release of pro-inflammatory cytokines (i.e., IL-1β, IL-8) by DCs in vitro.Citation60 Interestingly, in postoperative ileus (POI) serotonin activates cholinergic enteric neurons leading to the dampening of intestinal inflammation.Citation61 Thus, despite a lack of evidence of contact between serotoninergic fibers and immune cells, serotonin can indirectly modulate the innate immune response. Of note, enterochromaffin cells are a major source of gastrointestinal serotoninCitation62 suggesting that its effect on the immune system could also be mediated through a non-neuronal release.
Extrinsic sympathetic inputs also target secretomotor neurons immunoreactive for substance P.Citation63 Receptors for substance P (i.e NK) are found on a large variety of immune cells (e.g., mast cells, NK cells, macrophages, lymphocytes). SP induces the secretion of pro-inflammatory cytokines by macrophagesCitation64 and increases T cell proliferation.Citation65 Importantly, mast cells are found in the close vicinity of SP positive fibers in the intestineCitation66 and SP activates peritoneal mast cells leading to their degranulation.Citation67 The degranulation and consequent release of inflammatory mediators can in turn activate sensory nerves, a phenomenon involved in the pathophysiology of irritable bowel syndrome (IBS)Citation68 and POI. The use of mast cell stabilizer doxantrazoleCitation69 or an inhibitor of Syk,Citation70 a kinase involved in the downstream signaling of SP receptors, showed its efficacy in dampening the inflammation in POI in mice. Although the SP-mast cells interaction is a key event in IBS and POI, the neuronal source of this neurotransmitter (i.e., enteric neurons or vagal sensory afferents) remains unclear.Citation71
In addition, a dramatic alteration in the expression of enteric neurotransmitters was observed in animal models of IBS and IBD patients.Citation72 This phenomenon, most probably driven by the ongoing inflammationCitation73 is likely to influence the modulation of the intestinal immune system. Treatment with VIP in a TNBS-colitis model decreased the severity of the inflammationCitation74 suggesting a beneficial role of this neuropeptide on the colonic inflammation. In experimental colitisCitation75 and CD patients,Citation76 an increase in the number of VIP+ neurons was measured and correlated with an elevated colonic VIP content. However, contradictory results were found on VIP expression in ulcerative colitis and Crohn’s disease (CD) patientsCitation77 leaving under debate the role of VIP in the colonic inflammation.
CNS Control of the Immune Response: The Inflammatory Reflex
A fine tuning of the immune response is required to maintain the organism homeostasis. This refined control relies on interactions between the CNS and immune cells mediated through neural autonomic reflexes. The initiation of inflammatory neural reflexes is characterized by the activation of sensory nerve terminals by inflammatory mediators. This peripheral information is conveyed to the CNS where it triggers the activation of motor neurons which in turn provide signals to immune cells present in the target organ.
Sympathetic reflexes and involvement in intestinal inflammatory diseases
Sympathetic reflexes are involved in diverse intestinal pathophysiologies. In POI, sympathetic reflexes play a role in both stages of this inflammatory disorder: the early neurogenic and the late inflammatory phase.Citation78 In the early phase, the activation of spinal mechano-sensory afferent fibersCitation79 by handling of the intestine triggers NA release by sympathetic motor fibers. The inhibitory effect of NA on intestinal smooth muscle cells leads to an impaired gastrointestinal motility. In the late inflammatory phase, the activation of this sympathetic reflex is maintained through the release of NO and the induction of Cox-2 secretion by infiltrated leukocytesCitation80-Citation82 thereby resulting in a prolonged intestinal paralysis. This sympathetic feedback loop is proposed to participate in the disseminative nature of POI.Citation83
In other intestinal inflammatory disorders, activation of sympathetic reflexes showed to play an important role in modulating the intestinal immune system. In intestinal parasitic infection (e.g., Trichinella spiralis)Citation84 and experimental colitis models (TNBSCitation85 or DSSCitation86) motor sympathetic fibers are affected as shown by a decreased NA release in the gut. Importantly, observations of intestinal sympathetic innervation in CD patients showed a lack of TH+ fibers in every intestinal layer.Citation87 This result however remains debatable as other studies showed an increased amount of TH+ fibers in the myenteric plexus of CD patients.Citation76 Additionally, the α2-adrenoceptor antagonist RX821002 inhibited the colonic mRNA level of pro-inflammatory cytokines (TNF-α and IL-1β) in TNBS colitis.Citation88 In the same model, chemical sympathectomy showed to ameliorate colonic inflammation.Citation89 These findings underscore the pro-inflammatory role of NA and α2-adrenoceptors signaling in colitis. Interestingly, exposure to TNBS also led to an increase in the number of sympathetic ‘basket-like structures’ in the dorsal root ganglia showing that colonic inflammation triggers sprouting of sympathetic fibers. These structures surround sensory neurons of the dorsal root ganglia, suggesting that sympathetic sprouting could influence the plasticity of these sensory neurons and be partly responsible for the visceral hypersensitivity in IBS and the chronic pain observed in colitis patients.Citation90
Vagal reflexes in intestinal inflammatory diseases
The ability of the immune system to activate the sensory arm of the vagal nerve is well established. In the intestine, close anatomical contacts between vagal sensory fibers and mucosal granular cells resembling granulocytesCitation91 and mucosal mast cellsCitation92 have been reported. These cells can release inflammatory mediators such as IL-1β and prostaglandins which in turn activate vagal afferents fibers.Citation93 Only ten years ago, the motor part of the vagus nerve was discovered as a modulator of the immune response. Indeed, activation of the vagus nerve (by electrical stimulation) showed to suppress the pro-inflammatory cytokine release by macrophages in a rat model of endotoxemia.Citation94 This effect mediated by the α7nAChR was named ‘cholinergic anti-inflammatory pathway’ (CAIP) and brought to light the concept of a vagal inflammatory reflex.Citation95
In POI, vagal nerve stimulation (VNS) applied prior to the intestinal manipulation prevents the inhibition of the gastrointestinal motility by suppressing the activation of resident intestinal macrophages via α7nAChR. We recently provided neuroanatomical evidence of the activation of vagal sensory and motor vagal neurons in the NTS and DMV respectively in the late inflammatory phase of POICitation96 demonstrating the existence of an endogenous vago-vagal reflex directed toward the local inflamed zone. In the same line, endogenous activation of the vagal reflex by administration of enteral lipid-rich nutritionCitation97 dampens the pro-inflammatory cytokine secretion by resident macrophages therefore preventing ileus. Altogether, these observations suggest two distinct functions of the vagal nerve in the modulation of the immune response: a preventive role when the vagus nerve is activated (by VNS) prior the immune challenge or insult; a role in the restoration of homeostasis when the vagal reflex is activated after the inflammation is settled.
The cholinergic anti-inflammatory pathway was also demonstrated in experimental colitis. Subdiaphragmatic vagotomy in DSS-exposed mice enhanced pro-inflammatory cytokine levels (i.e., TNF-α, IL-6 and IL-1β) worsening colonic inflammation.Citation98 Although the α7nAChR plays a key role in mediating the cholinergic suppressive effect on macrophage activity, another cholinergic receptor, α5nAChR, was shown to participate in the vagal anti-inflammatory mechanism during colitis.Citation99 With regards to the colon innervation, further investigations are required to determine the exact involvement of direct vagal innervation and sacral parasympathetic inputs in the cholinergic anti-inflammatory pathway regulating colitis.
Spleen Innervation: Involvement in the Vagal Reflex Controlling Immune Responses
The spleen and its innervation were recently identified as key players in mediating the vagal anti-inflammatory effect in sepsis.Citation100,Citation101 Interestingly, no evidence of a direct vagal innervation to the spleen has been shown to date.Citation102,Citation103 The vagal control on the spleen was consequently proposed to rely on vagal innervation of splenic postganglionic sympathetic neurons expressing α7nAChR located in celiac ganglia.Citation100,Citation101
This vagal control of NA release by splenic sympathetic fibers was recently shown to target a specific memory T cell population producing ACh (as they express ChAT).Citation39 This non-neuronal source of ACh is proposed to suppress pro-inflammatory cytokines (i.e., TNF-α) secretion by macrophages. However, some controversy exists as recent studies demonstrate the absence of neuronal contact between vagal and splenic nerveCitation104 (own unpublished data), suggesting that the anti-inflammatory effect observed may be driven by a spinal sympathetic reflex rather than a vagal reflex.
Nevertheless, the discovery of these cholinergic T cells may suggest a role of these cells in other inflammatory disorders where cholinergic regulation of the immune system is involved. The presence of this cell population may indeed not be restricted to the spleen but could also be found in other lymphoid structures such as PPs or mesenteric lymph nodes which present a comparable distribution of noradrenergic fibers. It would therefore be of interest to consider the contribution of this ChAT positive T cell population in the cholinergic anti-inflammatory mechanisms regulating intestinal inflammatory diseases such as POI and IBD.
The Microbiota-Gut-Brain Axis
Recent studies shed light on the involvement of the microbiota as an important component able to modulate the brain chemistry and function. This so-called microbiota-gut-brain axis opens the way to a novel research axis in neurogastroenterology. The role of the intestinal microbiota in the modulation of the nervous system was recently shown by studies where alteration of the microflora was performed. Diet-induced alteration of the intestinal microbiota led to an amelioration in assessed memory and learning capacity.Citation105 Similarly, dysbiosis triggered by oral administration of antibiotics is associated with an increased hippocampal expression of Brain-Derived Neurotrophic Factor (BDNF) and an increased exploratory behavior.Citation106 Additionally, chronic exposure to probiotics ameliorated anxiety- and depression-like behaviors in parasite- and DSS-induced colitis models.Citation107,Citation108 Subdiaphragmatic vagotomy abolishes this beneficial effect demonstrating the importance of the neuronal (herein vagal) gut-brain axis in the action of probiotics.
IBD and IBS patients exhibit changes in the composition of their intestinal microflora.Citation109 Moreover, co-morbidities of anxiety and depression are frequently observed in these patients.Citation110 The ability of the gut microbiota to modulate the CNS activity and ameliorate depression-like behaviors in animal models provides an alternative approach to tackle the psychological disorders often affecting IBD patients but also the underlying cause of IBD, i.e., the inflammation. Indeed, depression and anxiety behaviors are associated with Hyptohalamic-Pituitary-Adrenal (HPA) axis hyperactivity (for a review see refs. Citation111, Citation112) and HPA axis activation is often associated with enhanced or relapse of gut inflammation. Reducing its activity in IBD patients by ameliorating the depression and anxiety behaviors may therefore be beneficial on the inflammation itself. Modulating the intestinal microbiota in order to ameliorate said behaviors would therefore be of particular interest and would provide a new approach to ameliorate both psychological and inflammatory symptoms in IBD.
Conclusion and Outreach
A large body of evidence points toward an integrated regulatory role of both extrinsic and intrinsic innervation in intestinal immunity. The importance of inflammatory reflexes in regulating acute and chronic intestinal inflammatory disorders is emerging and the ENS appears as a pivotal element linking sympathetic and more particularly vagal inputs to the immune system. The recent involvement of the intestinal microbiota as another component able to modulate the gut-brain axis offers a novel level of complexity in this regulation. Our understanding of the interactions between nervous and immune system in the context of the intestine has strongly increased during the past decade. However, the exact mechanisms underlying these neuro-immune interactions still remain partly unclear and numerous challenges need to be addressed to comprehend the functioning of this neuro-immune system. On the one hand, further anatomical evidence are required to determine precisely which neural axis is able to affect the different components of the immune system in the intestine. On the other hand, the clear role of the nervous system in the pathophysiology of intestinal inflammatory disorders is not yet clarified in the current literature and further investigations are necessary to unravel the therapeutic relevance of this neural component. From a clinical point of view, the prevalence of intestinal inflammatory disorders such as IBD, IBS and POI and the limitations and cost of current treatments call for therapeutic alternatives. Regulating the immune system via the modulation of the nervous system may provide us with a powerful tool to resolve the intestinal inflammation underlying these disorders.
Disclosure of Potential Conflicts of Interest
No potential conflicts of interest were disclosed.
References
- Berthoud HR, Bereiter DA, Jeanrenaud B. Role of the autonomic nervous system in the mediation of LHA electrical stimulation-induced effects on insulinemia and glycemia. J Auton Nerv Syst 1980; 2:183 - 98; http://dx.doi.org/10.1016/0165-1838(80)90044-2; PMID: 7021651
- Olsson C, Chen BN, Jones S, Chataway TK, Costa M, Brookes SJ. Comparison of extrinsic efferent innervation of guinea pig distal colon and rectum. J Comp Neurol 2006; 496:787 - 801; http://dx.doi.org/10.1002/cne.20965; PMID: 16628614
- Bayliss WM, Starling EH. The movements and innervation of the small intestine. J Physiol 1899; 24:99 - 143; PMID: 16992487
- Pack RJ, Richardson PS, Smith IC, Webb SR. The functional significance of the sympathetic innervation of mucous glands in the bronchi of man. J Physiol 1988; 403:211 - 9; PMID: 3253421
- Elenkov IJ, Wilder RL, Chrousos GP, Vizi ES. The sympathetic nerve--an integrative interface between two supersystems: the brain and the immune system. Pharmacol Rev 2000; 52:595 - 638; PMID: 11121511
- Furness JB, Costa M. Morphology and distribution of intrinsic adrenergic neurones in the proximal colon of the guinea-pig. Z Zellforsch Mikrosk Anat 1971; 120:346 - 63; http://dx.doi.org/10.1007/BF00324897; PMID: 5153840
- Phillips RJ, Rhodes BS, Powley TL. Effects of age on sympathetic innervation of the myenteric plexus and gastrointestinal smooth muscle of Fischer 344 rats. Anat Embryol (Berl) 2006; 211:673 - 83; http://dx.doi.org/10.1007/s00429-006-0123-z; PMID: 17024301
- Fu YY, Peng SJ, Lin HY, Pasricha PJ, Tang SC. 3-D imaging and illustration of mouse intestinal neurovascular complex. Am J Physiol Gastrointest Liver Physiol 2013; 304:G1 - 11; http://dx.doi.org/10.1152/ajpgi.00209.2012; PMID: 23086917
- Capurso L, Friedmann CA, Parks AG. Adrenergic fibres in the human intestine. Gut 1968; 9:678 - 82; http://dx.doi.org/10.1136/gut.9.6.678; PMID: 5717969
- Llewellyn-Smith IJ, Furness JB, O’Brien PE, Costa M. Noradrenergic nerves in human small intestine. Distribution and ultrastructure. Gastroenterology 1984; 87:513 - 29; PMID: 6745604
- Dorban G, Defaweux V, Demonceau C, Flandroy S, Van Lerberghe PB, Falisse-Poirrier N, et al. Interaction between dendritic cells and nerve fibres in lymphoid organs after oral scrapie exposure. Virchows Arch 2007; 451:1057 - 65; http://dx.doi.org/10.1007/s00428-007-0476-6; PMID: 17823814
- Felten DL, Felten SY, Carlson SL, Olschowka JA, Livnat S. Noradrenergic and peptidergic innervation of lymphoid tissue. J Immunol 1985; 135:Suppl 755s - 65s; PMID: 2861231
- Manni M, Granstein RD, Maestroni G. β2-Adrenergic agonists bias TLR-2 and NOD2 activated dendritic cells towards inducing an IL-17 immune response. Cytokine 2011; 55:380 - 6; http://dx.doi.org/10.1016/j.cyto.2011.05.013; PMID: 21683614
- Maestroni GJ. Dendritic cell migration controlled by alpha 1b-adrenergic receptors. J Immunol 2000; 165:6743 - 7; PMID: 11120793
- Maestroni GJ, Mazzola P. Langerhans cells beta 2-adrenoceptors: role in migration, cytokine production, Th priming and contact hypersensitivity. J Neuroimmunol 2003; 144:91 - 9; http://dx.doi.org/10.1016/j.jneuroim.2003.08.039; PMID: 14597102
- Yanagawa Y, Matsumoto M, Togashi H. Enhanced dendritic cell antigen uptake via alpha2 adrenoceptor-mediated PI3K activation following brief exposure to noradrenaline. J Immunol 2010; 185:5762 - 8; http://dx.doi.org/10.4049/jimmunol.1001899; PMID: 20935206
- Sanders VM. The beta2-adrenergic receptor on T and B lymphocytes: do we understand it yet?. Brain Behav Immun 2012; 26:195 - 200; http://dx.doi.org/10.1016/j.bbi.2011.08.001; PMID: 21855626
- Li YS, Kouassi E, Revillard JP. Differential regulation of mouse B-cell activation by beta-adrenoceptor stimulation depending on type of mitogens. Immunology 1990; 69:367 - 72; PMID: 2155873
- Kasprowicz DJ, Kohm AP, Berton MT, Chruscinski AJ, Sharpe A, Sanders VM. Stimulation of the B cell receptor, CD86 (B7-2), and the beta 2-adrenergic receptor intrinsically modulates the level of IgG1 and IgE produced per B cell. J Immunol 2000; 165:680 - 90; PMID: 10878340
- Kohm AP, Sanders VM. Norepinephrine and beta 2-adrenergic receptor stimulation regulate CD4+ T and B lymphocyte function in vitro and in vivo. Pharmacol Rev 2001; 53:487 - 525; PMID: 11734616
- González-Ariki S, Husband AJ. The role of sympathetic innervation of the gut in regulating mucosal immune responses. Brain Behav Immun 1998; 12:53 - 63; http://dx.doi.org/10.1006/brbi.1997.0509; PMID: 9570861
- Flierl MA, Rittirsch D, Huber-Lang M, Sarma JV, Ward PA. Catecholamines-crafty weapons in the inflammatory arsenal of immune/inflammatory cells or opening pandora’s box?. Mol Med 2008; 14:195 - 204; PMID: 18079995
- Phillips RJ, Powley TL. Macrophages associated with the intrinsic and extrinsic autonomic innervation of the rat gastrointestinal tract. Auton Neurosci 2012; 169:12 - 27; http://dx.doi.org/10.1016/j.autneu.2012.02.004; PMID: 22436622
- Chou RC, Stinson MW, Noble BK, Spengler RN. Beta-adrenergic receptor regulation of macrophage-derived tumor necrosis factor-alpha production from rats with experimental arthritis. J Neuroimmunol 1996; 67:7 - 16; PMID: 8707933
- Straub RH, Pongratz G, Weidler C, Linde HJ, Kirschning CJ, Glück T, et al. Ablation of the sympathetic nervous system decreases gram-negative and increases gram-positive bacterial dissemination: key roles for tumor necrosis factor/phagocytes and interleukin-4/lymphocytes. J Infect Dis 2005; 192:560 - 72; http://dx.doi.org/10.1086/432134; PMID: 16028124
- Gershon MD, Sherman DL. Noradrenergic innervation of serotoninergic neurons in the myenteric plexus. J Comp Neurol 1987; 259:193 - 210; http://dx.doi.org/10.1002/cne.902590203; PMID: 3584557
- Lundgren O.. Sympathetic input into the enteric nervous system. Gut2000; 47 (Suppl 4): iv33-5; discussion iv6.
- Tobin G, Giglio D, Lundgren O.. Muscarinic receptor subtypes in the alimentary tract. J Physiol Pharmacol 2009; 60:3-21.
- Xu Y, Luo X, Shen J, Zhu W, Chen K, Jiang H. Molecular dynamics of nicotinic acetylcholine receptor correlating biological functions. Curr Protein Pept Sci 2006; 7:195 - 200; http://dx.doi.org/10.2174/138920306777452321; PMID: 16787259
- Kawashima K, Fujii T, Moriwaki Y, Misawa H. Critical roles of acetylcholine and the muscarinic and nicotinic acetylcholine receptors in the regulation of immune function. Life Sci 2012; 91:1027 - 32; http://dx.doi.org/10.1016/j.lfs.2012.05.006; PMID: 22659391
- Qian J, Galitovskiy V, Chernyavsky AI, Marchenko S, Grando SA. Plasticity of the murine spleen T-cell cholinergic receptors and their role in in vitro differentiation of naïve CD4 T cells toward the Th1, Th2 and Th17 lineages. Genes Immun 2011; 12:222 - 30; http://dx.doi.org/10.1038/gene.2010.72; PMID: 21270829
- Wang H, Yu M, Ochani M, Amella CA, Tanovic M, Susarla S, et al. Nicotinic acetylcholine receptor alpha7 subunit is an essential regulator of inflammation. Nature 2003; 421:384 - 8; http://dx.doi.org/10.1038/nature01339; PMID: 12508119
- Kawashima K, Yoshikawa K, Fujii YX, Moriwaki Y, Misawa H. Expression and function of genes encoding cholinergic components in murine immune cells. Life Sci 2007; 80:2314 - 9; http://dx.doi.org/10.1016/j.lfs.2007.02.036; PMID: 17383684
- Skok M, Grailhe R, Changeux JP. Nicotinic receptors regulate B lymphocyte activation and immune response. Eur J Pharmacol 2005; 517:246 - 51; http://dx.doi.org/10.1016/j.ejphar.2005.05.011; PMID: 15963492
- Skok M, Grailhe R, Agenes F, Changeux JP. The role of nicotinic acetylcholine receptors in lymphocyte development. J Neuroimmunol 2006; 171:86 - 98; http://dx.doi.org/10.1016/j.jneuroim.2005.09.011; PMID: 16253349
- Kirchgessner AL, Gershon MD. Identification of vagal efferent fibers and putative target neurons in the enteric nervous system of the rat. J Comp Neurol 1989; 285:38 - 53; http://dx.doi.org/10.1002/cne.902850105; PMID: 2568999
- Holst MC, Kelly JB, Powley TL. Vagal preganglionic projections to the enteric nervous system characterized with Phaseolus vulgaris-leucoagglutinin. J Comp Neurol 1997; 381:81 - 100; http://dx.doi.org/10.1002/(SICI)1096-9861(19970428)381:1<81::AID-CNE7>3.0.CO;2-G; PMID: 9087421
- Walter GC, Phillips RJ, Baronowsky EA, Powley TL. Versatile, high-resolution anterograde labeling of vagal efferent projections with dextran amines. J Neurosci Methods 2009; 178:1 - 9; http://dx.doi.org/10.1016/j.jneumeth.2008.11.003; PMID: 19056424
- Rosas-Ballina M, Olofsson PS, Ochani M, Valdés-Ferrer SI, Levine YA, Reardon C, et al. Acetylcholine-synthesizing T cells relay neural signals in a vagus nerve circuit. Science 2011; 334:98 - 101; http://dx.doi.org/10.1126/science.1209985; PMID: 21921156
- Reardon C, Duncan GS, Brüstle A, Brenner D, Tusche MW, Olofsson PS, et al. Lymphocyte-derived ACh regulates local innate but not adaptive immunity. Proc Natl Acad Sci U S A 2013; 110:1410 - 5; http://dx.doi.org/10.1073/pnas.1221655110; PMID: 23297238
- Costa M, Brookes SJ, Hennig GW. . Anatomy and physiology of the enteric nervous system. Gut2000; 47 (Suppl 4):iv15-9; discussion iv26.
- Furness JB. The enteric nervous system and neurogastroenterology. Nature reviews. Gastroenterol Hepatol 2012; 9:286 - 94
- Berthoud HR, Jedrzejewska A, Powley TL. Simultaneous labeling of vagal innervation of the gut and afferent projections from the visceral forebrain with dil injected into the dorsal vagal complex in the rat. J Comp Neurol 1990; 301:65 - 79; http://dx.doi.org/10.1002/cne.903010107; PMID: 1706359
- Manber L, Gershon MD. A reciprocal adrenergic-cholinergic axoaxonic synapse in the mammalian gut. Am J Physiol 1979; 236:E738 - 45; PMID: 443427
- de Jonge WJ, van der Zanden EP, The FO, Bijlsma MF, van Westerloo DJ, Bennink RJ, et al. Stimulation of the vagus nerve attenuates macrophage activation by activating the Jak2-STAT3 signaling pathway. Nat Immunol 2005; 6:844 - 51; http://dx.doi.org/10.1038/ni1229; PMID: 16025117
- Vizi ES. Presynaptic modulation of neurochemical transmission. Prog Neurobiol 1979; 12:181 - 290; http://dx.doi.org/10.1016/0301-0082(79)90011-X; PMID: 39316
- Straub RH, Wiest R, Strauch UG, Härle P, Schölmerich J. The role of the sympathetic nervous system in intestinal inflammation. Gut 2006; 55:1640 - 9; http://dx.doi.org/10.1136/gut.2006.091322; PMID: 17047110
- Kulkarni-Narla A, Beitz AJ, Brown DR. Catecholaminergic, cholinergic and peptidergic innervation of gut-associated lymphoid tissue in porcine jejunum and ileum. Cell Tissue Res 1999; 298:275 - 86; http://dx.doi.org/10.1007/s004419900096; PMID: 10571116
- Delgado M, Abad C, Martinez C, Juarranz MG, Arranz A, Gomariz RP, et al. Vasoactive intestinal peptide in the immune system: potential therapeutic role in inflammatory and autoimmune diseases. J Mol Med (Berl) 2002; 80:16 - 24; http://dx.doi.org/10.1007/s00109-001-0291-5; PMID: 11862320
- Delgado M, Ganea D. Inhibition of endotoxin-induced macrophage chemokine production by vasoactive intestinal peptide and pituitary adenylate cyclase-activating polypeptide in vitro and in vivo. J Immunol 2001; 167:966 - 75; PMID: 11441105
- Delgado M, Gonzalez-Rey E, Ganea D. The neuropeptide vasoactive intestinal peptide generates tolerogenic dendritic cells. J Immunol 2005; 175:7311 - 24; PMID: 16301637
- Massacand JC, Kaiser P, Ernst B, Tardivel A, Bürki K, Schneider P, et al. Intestinal bacteria condition dendritic cells to promote IgA production. PLoS One 2008; 3:e2588; http://dx.doi.org/10.1371/journal.pone.0002588; PMID: 18596964
- Boirivant M, Fais S, Annibale B, Agostini D, Delle Fave G, Pallone F. Vasoactive intestinal polypeptide modulates the in vitro immunoglobulin A production by intestinal lamina propria lymphocytes. Gastroenterology 1994; 106:576 - 82; PMID: 8119526
- Phillips RJ, Powley TL. . Innervation of the gastrointestinal tract: patterns of aging. Auton Neurosci 2007; 136:1-19.
- Allione A, Bernabei P, Bosticardo M, Ariotti S, Forni G, Novelli F. Nitric oxide suppresses human T lymphocyte proliferation through IFN-gamma-dependent and IFN-gamma-independent induction of apoptosis. J Immunol 1999; 163:4182 - 91; PMID: 10510354
- Cifone MG, D’Alò S, Parroni R, Millimaggi D, Biordi L, Martinotti S, et al. Interleukin-2-activated rat natural killer cells express inducible nitric oxide synthase that contributes to cytotoxic function and interferon-gamma production. Blood 1999; 93:3876 - 84; PMID: 10339496
- Bogdan C. Nitric oxide and the immune response. Nat Immunol 2001; 2:907 - 16; http://dx.doi.org/10.1038/ni1001-907; PMID: 11577346
- Kirchgessner AL, Tamir H, Gershon MD. . Identification and stimulation by serotonin of intrinsic sensory neurons of the submucosal plexus of the guinea pig gut: activity-induced expression of Fos immunoreactivity. J Neurosci1992; 12:235-48.
- Grønstad KO, DeMagistris L, Dahlström A, Nilsson O, Price B, Zinner MJ, et al. The effects of vagal nerve stimulation on endoluminal release of serotonin and substance P into the feline small intestine. Scand J Gastroenterol 1985; 20:163 - 9; http://dx.doi.org/10.3109/00365528509089650; PMID: 2581309
- Idzko M, Panther E, Stratz C, Müller T, Bayer H, Zissel G, et al. The serotoninergic receptors of human dendritic cells: identification and coupling to cytokine release. J Immunol 2004; 172:6011 - 9; PMID: 15128784
- Tsuchida Y, Hatao F, Fujisawa M, Murata T, Kaminishi M, Seto Y, et al. Neuronal stimulation with 5-hydroxytryptamine 4 receptor induces anti-inflammatory actions via α7nACh receptors on muscularis macrophages associated with postoperative ileus. Gut 2011; 60:638 - 47; http://dx.doi.org/10.1136/gut.2010.227546; PMID: 21115544
- Ormsbee HS 3rd, Fondacaro JD. Action of serotonin on the gastrointestinal tract. Proc Soc Exp Biol Med 1985; 178:333 - 8; http://dx.doi.org/10.3181/00379727-178-42016; PMID: 3919396
- Brookes SJ, Steele PA, Costa M. Identification and immunohistochemistry of cholinergic and non-cholinergic circular muscle motor neurons in the guinea-pig small intestine. Neuroscience 1991; 42:863 - 78; http://dx.doi.org/10.1016/0306-4522(91)90050-X; PMID: 1720229
- Kimball ES, Persico FJ, Vaught JL. Neurokinin-induced generation of interleukin-1 in a macrophage cell line. Ann N Y Acad Sci 1988; 540:688 - 90; http://dx.doi.org/10.1111/j.1749-6632.1988.tb27213.x; PMID: 2462840
- Payan DG, Brewster DR, Goetzl EJ. Specific stimulation of human T lymphocytes by substance P. J Immunol 1983; 131:1613 - 5; PMID: 6194207
- Dong WZ, Zou DW, Li ZS, Zou XP, Zhu AY, Xu GM, et al. Study of visceral hypersensitivity in irritable bowel syndrome. Chin J Dig Dis 2004; 5:103 - 9; http://dx.doi.org/10.1111/j.1443-9573.2004.00168.x; PMID: 15612244
- Shanahan F, Denburg JA, Fox J, Bienenstock J, Befus D. Mast cell heterogeneity: effects of neuroenteric peptides on histamine release. J Immunol 1985; 135:1331 - 7; PMID: 2409146
- Feng B, La JH, Schwartz ES, Gebhart GF. Irritable bowel syndrome: methods, mechanisms, and pathophysiology. Neural and neuro-immune mechanisms of visceral hypersensitivity in irritable bowel syndrome. Am J Physiol Gastrointest Liver Physiol 2012; 302:G1085 - 98; http://dx.doi.org/10.1152/ajpgi.00542.2011; PMID: 22403791
- de Jonge WJ, The FO, van der Coelen D, Bennink RJ, Reitsma PH, van Deventer SJ, et al. Mast cell degranulation during abdominal surgery initiates postoperative ileus in mice. Gastroenterology 2004; 127:535 - 45; http://dx.doi.org/10.1053/j.gastro.2004.04.017; PMID: 15300586
- van Bree SH, Gomez-Pinilla PJ, van de Bovenkamp FS, Di Giovangiulio M, Farro G, Nemethova A, et al. Inhibition of spleen tyrosine kinase as treatment of postoperative ileus. Gut 2012; http://dx.doi.org/10.1136/gutjnl-2012-302615; PMID: 23242119
- Genton L, Kudsk KA. Interactions between the enteric nervous system and the immune system: role of neuropeptides and nutrition. Am J Surg 2003; 186:253 - 8; http://dx.doi.org/10.1016/S0002-9610(03)00210-1; PMID: 12946828
- Lakhan SE, Kirchgessner A. Neuroinflammation in inflammatory bowel disease. J Neuroinflammation 2010; 7:37; http://dx.doi.org/10.1186/1742-2094-7-37; PMID: 20615234
- Geboes K.. Immunopathological studies of the small intestinal intramural nervous system and of intramural vessels in Crohn's disease. Verh K Acad Geneeskd Belg1993; 55:267-301; discussion -3.
- Arranz A, Juarranz Y, Leceta J, Gomariz RP, Martínez C. VIP balances innate and adaptive immune responses induced by specific stimulation of TLR2 and TLR4. Peptides 2008; 29:948 - 56; http://dx.doi.org/10.1016/j.peptides.2008.01.019; PMID: 18359536
- Linden DR, Couvrette JRN, Ciolino A, McQuoid C, Blaszyk H, Sharkey KA, et al. Indiscriminate loss of myenteric neurones in the TNBS-inflamed guinea-pig distal colon. Neurogastroenterol Motil2005; 17:751-60.
- Belai A, Boulos PB, Robson T, Burnstock G. Neurochemical coding in the small intestine of patients with Crohn’s disease. Gut 1997; 40:767 - 74; http://dx.doi.org/10.1136/gut.40.6.767; PMID: 9245931
- Mazumdar S, Das KM. Immunocytochemical localization of vasoactive intestinal peptide and substance P in the colon from normal subjects and patients with inflammatory bowel disease. Am J Gastroenterol 1992; 87:176 - 81; PMID: 1370872
- Bueno L, Fioramonti J, Ruckebusch Y. Postoperative intestinal motility in dogs and sheep. Am J Dig Dis 1978; 23:682 - 9; http://dx.doi.org/10.1007/BF01072352; PMID: 685935
- Zittel TT, Reddy SN, Plourde V, Raybould HE. Role of spinal afferents and calcitonin gene-related peptide in the postoperative gastric ileus in anesthetized rats. Ann Surg 1994; 219:79 - 87; http://dx.doi.org/10.1097/00000658-199401000-00013; PMID: 8297181
- Kalff JC, Buchholz BM, Eskandari MK, Hierholzer C, Schraut WH, Simmons RL, et al. Biphasic response to gut manipulation and temporal correlation of cellular infiltrates and muscle dysfunction in rat. Surgery 1999; 126:498 - 509; http://dx.doi.org/10.1016/S0039-6060(99)70091-7; PMID: 10486602
- Kalff JC, Carlos TM, Schraut WH, Billiar TR, Simmons RL, Bauer AJ. Surgically induced leukocytic infiltrates within the rat intestinal muscularis mediate postoperative ileus. Gastroenterology 1999; 117:378 - 87; http://dx.doi.org/10.1053/gast.1999.0029900378; PMID: 10419919
- Kalff JC, Schraut WH, Simmons RL, Bauer AJ. Surgical manipulation of the gut elicits an intestinal muscularis inflammatory response resulting in postsurgical ileus. Ann Surg 1998; 228:652 - 63; http://dx.doi.org/10.1097/00000658-199811000-00004; PMID: 9833803
- de Jonge WJ, van den Wijngaard RM, The FO, ter Beek ML, Bennink RJ, Tytgat GN, et al. Postoperative ileus is maintained by intestinal immune infiltrates that activate inhibitory neural pathways in mice. Gastroenterology 2003; 125:1137 - 47; http://dx.doi.org/10.1016/S0016-5085(03)01197-1; PMID: 14517797
- Swain MG, Blennerhassett PA, Collins SM. Impaired sympathetic nerve function in the inflamed rat intestine. Gastroenterology 1991; 100:675 - 82; PMID: 1847118
- Jacobson K, McHugh K, Collins SM. The mechanism of altered neural function in a rat model of acute colitis. Gastroenterology 1997; 112:156 - 62; http://dx.doi.org/10.1016/S0016-5085(97)70230-0; PMID: 8978354
- Motagally MA, Neshat S, Lomax AE. Inhibition of sympathetic N-type voltage-gated Ca2+ current underlies the reduction in norepinephrine release during colitis. Am J Physiol Gastrointest Liver Physiol 2009; 296:G1077 - 84; http://dx.doi.org/10.1152/ajpgi.00006.2009; PMID: 19264956
- Straub RH, Grum F, Strauch U, Capellino S, Bataille F, Bleich A, et al. Anti-inflammatory role of sympathetic nerves in chronic intestinal inflammation. Gut 2008; 57:911 - 21; http://dx.doi.org/10.1136/gut.2007.125401; PMID: 18308830
- Bai A, Lu N, Guo Y, Chen J, Liu Z. Modulation of inflammatory response via alpha2-adrenoceptor blockade in acute murine colitis. Clin Exp Immunol 2009; 156:353 - 62; http://dx.doi.org/10.1111/j.1365-2249.2009.03894.x; PMID: 19250273
- McCafferty DM, Wallace JL, Sharkey KA. Effects of chemical sympathectomy and sensory nerve ablation on experimental colitis in the rat. Am J Physiol 1997; 272:G272 - 80; PMID: 9124351
- Xia CM, Colomb DG Jr, Akbarali HI, Qiao LY. . Prolonged sympathetic innervation of sensory neurons in rat thoracolumbar dorsal root ganglia during chronic colitis. Neurogastroenterol Motil2011; 23:801-e339.
- Berthoud HR, Kressel M, Raybould HE, Neuhuber WL. Vagal sensors in the rat duodenal mucosa: distribution and structure as revealed by in vivo DiI-tracing. Anat Embryol (Berl) 1995; 191:203 - 12; http://dx.doi.org/10.1007/BF00187819; PMID: 7771683
- Williams RM, Berthoud HR, Stead RH. Vagal afferent nerve fibres contact mast cells in rat small intestinal mucosa. Neuroimmunomodulation 1997; 4:266 - 70; PMID: 9650820
- Ek M, Kurosawa M, Lundeberg T, Ericsson A.. Activation of vagal afferents after intravenous injection of interleukin-1beta: role of endogenous prostaglandins. The Journal of neuroscience: the official journal of the Society for Neuroscience1998; 18:9471-9.
- Borovikova LV, Ivanova S, Zhang M, Yang H, Botchkina GI, Watkins LR, et al. Vagus nerve stimulation attenuates the systemic inflammatory response to endotoxin. Nature 2000; 405:458 - 62; http://dx.doi.org/10.1038/35013070; PMID: 10839541
- Tracey KJ. The inflammatory reflex. Nature 2002; 420:853 - 9; http://dx.doi.org/10.1038/nature01321; PMID: 12490958
- Cailotto C, Costes LM, van der Vliet J, van Bree SH, van Heerikhuize JJ, Buijs RM, et al. Neuroanatomical evidence demonstrating the existence of the vagal anti-inflammatory reflex in the intestine. Neurogastroenterol Motil2012; 24:191-200, e93.
- Lubbers T, De Haan JJ, Hadfoune M, Zhang Y, Luyer MD, Grundy D, et al. Lipid-enriched enteral nutrition controls the inflammatory response in murine Gram-negative sepsis. Crit Care Med 2010; 38:1996 - 2002; PMID: 20639744
- Ghia JE, Blennerhassett P, Kumar-Ondiveeran H, Verdu EF, Collins SM. The vagus nerve: a tonic inhibitory influence associated with inflammatory bowel disease in a murine model. Gastroenterology 2006; 131:1122 - 30; http://dx.doi.org/10.1053/j.gastro.2006.08.016; PMID: 17030182
- Orr-Urtreger A, Kedmi M, Rosner S, Karmeli F, Rachmilewitz D. Increased severity of experimental colitis in alpha 5 nicotinic acetylcholine receptor subunit-deficient mice. Neuroreport 2005; 16:1123 - 7; http://dx.doi.org/10.1097/00001756-200507130-00018; PMID: 15973160
- Rosas-Ballina M, Ochani M, Parrish WR, Ochani K, Harris YT, Huston JRN, et al. Splenic nerve is required for cholinergic antiinflammatory pathway control of TNF in endotoxemia. Proc Natl Acad Sci U S A 2008; 105:11008 - 13; http://dx.doi.org/10.1073/pnas.0803237105; PMID: 18669662
- Vida G, Peña G, Deitch EA, Ulloa L. α7-cholinergic receptor mediates vagal induction of splenic norepinephrine. J Immunol 2011; 186:4340 - 6; http://dx.doi.org/10.4049/jimmunol.1003722; PMID: 21339364
- Nance DM, Burns J. Innervation of the spleen in the rat: evidence for absence of afferent innervation. Brain Behav Immun 1989; 3:281 - 90; http://dx.doi.org/10.1016/0889-1591(89)90028-7; PMID: 2611414
- Bellinger DL, Lorton D, Hamill RW, Felten SY, Felten DL. Acetylcholinesterase staining and choline acetyltransferase activity in the young adult rat spleen: lack of evidence for cholinergic innervation. Brain Behav Immun 1993; 7:191 - 204; http://dx.doi.org/10.1006/brbi.1993.1021; PMID: 8219410
- Bratton BO, Martelli D, McKinley MJ, Trevaks D, Anderson CR, McAllen RM. Neural regulation of inflammation: no neural connection from the vagus to splenic sympathetic neurons. Exp Physiol 2012; 97:1180 - 5; PMID: 22247284
- Li W, Dowd SE, Scurlock B, Acosta-Martinez V, Lyte M. Memory and learning behavior in mice is temporally associated with diet-induced alterations in gut bacteria. Physiol Behav 2009; 96:557 - 67; http://dx.doi.org/10.1016/j.physbeh.2008.12.004; PMID: 19135464
- Bercik P, Denou E, Collins J, Jackson W, Lu J, Jury J, et al. The intestinal microbiota affect central levels of brain-derived neurotropic factor and behavior in mice. Gastroenterology2011; 141:599-609, e1-3.
- Bercik P, Park AJ, Sinclair D, Khoshdel A, Lu J, Huang X, et al. The anxiolytic effect of Bifidobacterium longum NCC3001 involves vagal pathways for gut-brain communication. Neurogastroenterol Motil 2011; 23:1132 - 9; http://dx.doi.org/10.1111/j.1365-2982.2011.01796.x; PMID: 21988661
- Bercik P, Verdu EF, Foster JA, Macri J, Potter M, Huang X, et al. Chronic gastrointestinal inflammation induces anxiety-like behavior and alters central nervous system biochemistry in mice. Gastroenterology2010; 139:2102-12e1.
- Chassaing B, Darfeuille-Michaud A. The commensal microbiota and enteropathogens in the pathogenesis of inflammatory bowel diseases. Gastroenterology 2011; 140:1720 - 8; http://dx.doi.org/10.1053/j.gastro.2011.01.054; PMID: 21530738
- DuPont AW, DuPont HL. The intestinal microbiota and chronic disorders of the gut. Nature reviews. Gastroenterol Hepatol 2011; 8:523 - 31
- Mawdsley JE, Rampton DS. Psychological stress in IBD: new insights into pathogenic and therapeutic implications. Gut 2005; 54:1481 - 91; http://dx.doi.org/10.1136/gut.2005.064261; PMID: 16162953
- Mawdsley JE, Rampton DS. The role of psychological stress in inflammatory bowel disease. Neuroimmunomodulation 2006; 13:327 - 36; http://dx.doi.org/10.1159/000104861; PMID: 17709955