Abstract
We have previously reported induction of transcriptional gene silencing (TGS) of HIV-1 by short hairpin RNA (shRNA) expressed in MOLT-4 cells. The shRNA (termed shPromA) targets the highly conserved tandem NF-kB binding sequences of the HIV-1 promoter. Recent articles have reported that TGS mediated by promoter-targeted siRNAs was exclusively the result of sequence non-specific off-target effects. Specifically, several mismatched siRNAs to the target promoter sequences were reported to also induce significant TGS, suggesting TGS was a consequence of off-target effects. Here, following extensive investigation, we report that shPromA induces sequence specific transcriptional silencing in HIV-1 infection in MOLT4 cells, while four shRNA variants, mismatched by 2-3 nucleotides, fail to suppress viral replication. We confirm similar levels of shRNA expression from the U6 promoter and the presence of processed/cleaved siRNAs for each construct in transduced MOLT-4 cells. HIV-1 sequence specific shPromA does not suppress HIV-2, which has an alternate NF-kB binding sequence. As a result of the unique sequence targeted, shPromA does not induce down-regulation of other NF-kB driven genes, either at the mRNA or protein level. Furthermore, we confirmed shPromA does not have sequence non-specific off-target effects through unaltered expression of CD4, CXCR4, and CCR5, which are used for viral entry. Additionally, shPromA does not alter PKR, IFN levels, and three downstream mediators of IFN-a response genes. Our data clearly shows that shPromA achieved highly specific TGS of HIV-1, demonstrating that effective TGS can be induced with minimal off-target effects.
Introduction
Small non-coding RNAs, including small interfering RNA (siRNA) and micro RNA (miRNA), have rapidly emerged as important regulators of gene expression.Citation1 Non-coding RNAs are involved in three distinct pathways of gene silencing: post transcriptional gene silencing (PTGS), transcriptional gene silencing (TGS), and translational silencing by miRNA.Citation1,Citation2 In PTGS Argonaute (Ago) proteins bind components of the siRNA duplex forming the RNA-induced silencing complex (RISC), which mediates cytoplasmic degradation of the targeted mRNA.Citation3–Citation5 TGS, previously described in plants, has been more recently observed in certain genes in mammalian cells.Citation6–Citation10 TGS is induced by siRNAs targeting promoter regions and occurs through a distinct mechanism, where the formation of the RNA-induced transcriptional silencing (RITS) complex mediates heterochromatin formation to induce repression of transcription.Citation1,Citation2 The mechanisms underlying siRNA-mediated TGS in mammalian cells are largely unknown. Recent articles have suggested that reported sequence specific TGS is an artifact resulting from off-target effects. This follows demonstrations that several siRNAs containing 2–5 nucleotide mismatches to the targeted promoter sequence were able to induce substantial TGS.Citation11,Citation12 In addition, it is well described that sequence non-specific off-target effects can be induced by certain siRNAs. This is especially the case with long single or double stranded RNA (dsRNA), which are recognized by endosomal receptors such as TLR3, TLR7 and TLR8, triggering interferon (IFN) pathways.Citation13–Citation18 Certain exogenous RNA species also trigger expression of dsRNA-dependent protein kinase R (PKR), resulting in an overall reduction of protein synthesis.Citation19,Citation20 A comprehensive evaluation of the specificity of any si/shRNA mediating TGS effects is essential prior to taking any construct forward towards development as a potential therapeutic strategy.
We have demonstrated previously that selected RNA duplexes targeting the promoter region of the integrated retroviruses of HIV-1 or SIV induce sustained suppression of viral replication through TGS.Citation21–Citation24 During this process we evaluated a number of siRNAs targeting the various sequences within retroviral promoter regions and revealed that most of the tested promoter-targeted siRNAs did not induce TGS.Citation21–Citation24 Among those tested siPromA, which targets a specific sequence within the tandem repeat of NFκB binding motifs in the U3 promoter region of the HIV 5′LTR, showed the most effective suppression. We have recently shown that prolonged HIV-1 gene suppression (>1 year) can be achieved in a T-cell line stably expressing an shRNA targeting the same sequence.Citation24 We also confirmed that the levels of integrated HIV-1 provirus DNA 24 hours post-infection were not different between MOLT-4 cells expressing shPromA and those expressing scrambled shPromA.Citation24 The silenced promoter region of the integrated viral genome is characterized by epigenetic modifications including, dimethylation of lysine 9 of histone 3 (H3K9me2), trimethylation of lysine 27 of histone 3 (H3K27me3), and the recruitment of histone deacetylase-1 (HDAC1), which is consistent with heterochromatin formation.Citation21,Citation23 Nuclear run-on assays definitively confirmed that shPromA induced transcriptional gene silencing.Citation21 Furthermore, the transcriptionally silenced virus is able to be reactivated by the histone deacetylase inhibitor, trichostatin-A (TSA).Citation24 Since HIV-1 has identical LTRs at the 5′ and 3′ ends of the integrated virus, it was important to document the relative contributions of TGS and PTGS to the observed silencing effect. We found that siPromA is a poor mediator of PTGS.Citation23 In combination these data strongly suggested that PromA siRNA/shRNA, targeting a conserved sequence within the HIV-1 promoter induces retroviral silencing by TGS.
In this study we aimed to demonstrate unequivocally that induction of TGS mediated by shRNA (shPromA) targeting the NFκB promoter region of HIV-1 is sequence specific. We hypothesized that sequence mismatched mutants of shPromA would not suppress HIV-1 transcription. Further, because the sequence targeted is distinct from that found in the promoters of human NFκB driven genes, we hypothesized that the level of expression of these genes would not be altered by shPromA. Finally, we sought to exclude sequence nonspecific effects by demonstrating a lack of change in the expression of PKR and type I IFNs or their downstream mediators.
Results
Sequence specific inhibition of HIV replication.
We achieved prolonged TGS in HIV-1 infected MOLT-4 that stably expressed shPromA, which targets a sequence within the NFκB binding region of HIV-1 promoter ().Citation24 We hypothesized the induced transcriptional suppression was sequence specific suppression rather than a consequence of induced off-target effects. In order to evaluate sequence specific suppression, we made four variant constructs with 2–3 nucleotide mismatches within the shPromA target sequences, designated shPromA-M1 to M4 (). Following retroviral transduction MOLT-4 cell lines stably expressing each of various shRNA were established, as well as MOLT-4 cell lines stably expressing shPromA and shPromA-Sc, and then each cell line was infected with HIV-1 IIIB.Citation24 HIV replication was measured by reverse transcriptase (RT) activity in culture supernatants. Only MOLT-4 cells expressing shPromA suppressed HIV-1 infection. None of the four mismatched variants (shPromA M1–4) or scrambled shRNA (shPromA-Sc) effectively suppressed HIV-1 replication, although, shPromA-M4 expressed in MOLT-4 cells showed transient suppression at day 7 post HIV-1 infection (). These data suggest that HIV-1 replication was suppressed only in MOLT-4 cells expressing shPromA, corresponding to the shRNA with perfectly matched sequence to the targeted sequence within the HIV-1 promoter.
Sequence specific viral suppression in MOLT-4 cells.
To further demonstrate the sequence specificity of shPromA-mediated TGS of HIV-1, we altered the virus used in the infectious model. Since HIV-2 possesses five different nucleotides within the sequence targeted by shPromA (), we investigated whether shPromA suppressed HIV-2 infection of MOLT-4 cells. As expected shPromA inhibited HIV-1 replication but failed to suppress HIV-2 replication, as determined by RT activity in culture supernatants (). We also monitored phenotypic changes due to HIV infection. Syncytium formation was inhibited in MOLT-4 shPromA infected with HIV-1 (Fig. S1A), however shPromA did not suppress syncytium formation resulting from HIV-2 infection (Fig. S1C). Importantly, neither HIV-1 nor HIV-2 viruses were inhibited in infected MOLT-4 cells stably expressing the scrambled shPromA sequence (Fig. S1B and D). These data further support the sequence specific nature of inhibition induced by shPromA. The data are also consistent with the previously observed specificity of viral suppression using a CD4+ HeLa cell line transfected with siRNAs in a HIV infection model.Citation21
Presence of siRNA in MOLT-4 cells.
Failure of the suppression of HIV-1 by MOLT-4 shPromA M1 to M4 mismatched variants and shPromA-Sc may result from defective or impaired expression of shRNA transcripts in the MOLT-4 cells. We sought to confirm the expression levels of mature/processed siRNAs generated from the shRNA transcripts (). We faced difficulties in detecting the short 21 bp siRNAs by standard northern blot procedure due to technical issues including, insufficient short RNA transfer to the blot membrane, inefficient hybridization ability of probes to the siRNAs already bound to the membrane, and difficulties in developing a single radio-labeled probe to detect all variants.Citation25,Citation26 Therefore we utilized a sensitive detection method based on cDNA synthesis of the extracted shRNAs, followed by PCR detection. We initially aimed to detect the antisense strand of shRNA transcripts expressed in the MOLT-4 cells by end-point PCR with primer sets consisting of antisense specific primers and a universal primer ( and B). Each antisense specific primer was able to amplify the perfectly matched corresponding antisense strand, but failed to detect any of the other antisense variants ().
Next, we investigated the presence of the mature/processed double-stranded RNAs in the stably transduced MOLT-4 cells. The shRNA transcript forms a loop structure via hybridization of the sense and antisense strands. The shRNA is transcribed by U6, RNA polymerase III, which terminates at poly(Ts) stretches in the DNA template.Citation27 The loop structure should then be processed by cellular ribonucleases to form mature/processed double-stranded RNA ().Citation28 We predicted that we would be able to detect cleaved strands of the sense strand and the loop using the same approach as for antisense strand detection. If the shRNAs were efficiently processed/cleaved, the size of the PCR amplicons generated by primer sets made up of each of sequence specific primers; asp, lsp or ssp, paired with the universal primer would be a similar size (). Indeed, the size of each of the PCR amplicons detected was approximately 120 bp (). These data indicate that mature/processed double-stranded RNAs were the dominant species in transduced MOLT-4 cells ( and C). We then asked whether the level of transcribed shRNA was relatively similar in each stably transduced cell. We employed Real-Time PCR quantification methodology to cDNA using miScript Reverse Transcription Kit. We used a single primer set of the universal loop specific primer (lsp) and the universal tag primer to assess shRNA expression across the experimental groups for this Real-Time PCR detection. We undertook this approach since individual asp and ssp may have different amplification efficiencies, which would lead to difficulties in comparing PCR quantification of products produced by different primer pairs. Using this methodology we found relatively similar amounts of the cleaved loop strand was present in the each of the MOLT-4 cell lines (), except shPromA-M3 transduced MOLT-4 cell, which produced higher levels of the processed loop strand compared with the other cell lines. However, as previously shown the shPromA-M3 variant did not result in any suppression of HIV-1 (). These data suggest that failure of the suppression of HIV-1 in MOLT-4 shPromA mismatched variants was not due to defective expression of mature/processed dsRNAs.
No significant change in expression of surface receptors by shPromA.
We investigated the possibility that the observed suppression of HIV-1 in MOLT-4 shPromA expressing cells may have resulted from off-target effects mediating reduced expression of the cell surface molecules that act as receptors for HIV-1 entry. We analyzed expression levels of CD4, CCR5 and CXCR4 on each of the MOLT-4 cell lines by flow cytometry and found there was no significant difference in the surface receptor expression across any of the transduced cells compared to untranduced MOLT-4 cells (). These results confirm that suppression of HIV-1 in MOLT-4 shPromA was not due to off-target effects that reduced expression of the cell surface receptors used for HIV-1 entry.
No significant alteration in expression of NFκB driven genes in shPromA in MOLT-4 cells.
Since shPromA targets the NFκB binding region of HIV-1, shPromA may potentially alter the expression of other NFκB driven genes as a sequence specific off-target effect. We assessed the expression of three surface receptors (CD54, CD38 and CD25), which are all dependent upon NFκB. No significant differences were observed in the expression of any of the three surface receptors (Fig. S2). To further exclude any off-target effects on NFκB driven genes, we employed a microtiter plate format PCR based assay to examine the expression levels of 86 NFκB driven genes, including IFNα, β, γ. We selected three stably transduced cell lines; shPromA, shPromA-M2 and shPromA-Sc, for this analysis. No significant difference in expression of was noted in any of these NFκB driven genes when comparing cells stably expressing shPromA and cells stably expressing shPromA-Sc () or shPromA-M2 (). Further, we did not detect any substantial induction of IFNα1, β1 or γ in shPromA expressing MOLT-4 cells (Table S1). In contrast, exposure to TNFα resulted in a significant overexpression of a large subset of NFκB driven genes, including greater than 18-fold upregulation of IFNα1, β1 and γ and over 120-fold upregulation of tumor necrosis factor receptor super family, member 10a (TNFRSF10A), consistent with previous reports in reference Citation29 and Citation30 ( and Table S1). These data strongly support the contention that HIV-1 transcriptional suppression induced by shPromA is sequence specific, and does not alter expression of other NFκB driven genes or induce IFN responses. We chose to also compare expression of these 86 NFκB driven genes between MOLT-4 cells transfected with or without siPromA, as stably expressing shRNA MOLT-4 cell lines are grown under the influence of the G418 antibiotic. MOLT-4 cells were siRNA-transfected using electroporation (Amaxa Nucleofector technology), since this approach has been recommended to assess IFN induction by RNA duplexes.Citation31,Citation32 The data confirmed that shPromA did not induce any substantial change in expression of the 86 NFκB driven genes compared to mock-transfected MOLT-4 cells (Fig. S3). Although there were slight reductions in several genes NFκB driven genes in MOLT-4 cells expressing shPromA, these changes were marginal, compared to ≥1,000 fold reduction in HIV-1 mRNA following transduction with shPromA.Citation21,Citation24
No significant sequence non-specific off-target induction by shPromA in MOLT-4 cells.
It is well established that exogenous RNA may induce sequence non-specific off-target effects. In particular long single or dsRNAs are recognized by endosomal innate immune receptors, such as TLR3, TLR7 and TLR8, which trigger type I interferon and interferon stimulated gene expression that may induce downstream non-specific off-target effects.Citation13,Citation14 Certain exogenous RNAs can also activate dsRNAdependent protein kinase R (PKR), whose function is global inhibition of protein synthesis.Citation19,Citation20 With this in mind we evaluated expression levels of three IFNα response genes; ISG20, Viperin and IFITM1, to determine IFN mediated off-target effects of shPromA. Consistent with the lack of increased IFNα expression in MOLT-4 shPromA expressing cells, there was no induction of expression in any of the three IFNα stimulated genes (). We also could not detect any induction of the activated form of PKR across all transduced MOLT-4 cells (). These data demonstrate that is highly unlikely the observed suppressive effects on HIV-1 transcription are due to the action of down-stream effects from IFNα or due to PKR.
Discussion
The induction of TGS by promoter-targeted siRNAs in mammalian cells remains a controversial subject, despite increasing reports of siRNA-mediated TGS of certain genes. The controversy arises to some extent because the process by which these siRNAs induce TGS is still very poorly understood. Identification of the RITS complex in mammalian cells remains problematical and is challenging because some components of the RITS complex and the RNA-directed RNA polymerase complex in plants and yeast do not have sequence homologues identifiable in the human genome.Citation33,Citation34 There is a contention in the literature that much of the observed siRNA induced TGS in mammalian cells is artificial, driven by consequence of off-target effects.Citation11,Citation12
In this study we have conducted an extensive investigation in MOLT-4 cells to explore whether our previously reported si/shRNA does indeed induce sequence specific TGS. We have previously reported that MOLT-4 cells expressing shPromA targeting a conserved sequence within the NFκB binding sequences of the HIV-1 promoter region achieved long-term suppression of viral replication through epigenetic modifications that result in heterochromatin formation.Citation24 In order to assess sequence specificity, we made MOLT-4 cells stably expressing four mismatched mutants (shPromA-M1 to M4) and confirmed essentially equivalent levels of expression and processing of shPromA, the four mismatched mutants of shPromA M1–M4 and a scrambled version of shPromA. We explored three main questions. Firstly, was the observed viral suppression sequence specific? Secondly, was there any evidence of sequence specific off-target effects? This was particularly important with the PromA construct as it targets a unique sequence of the tandem repeat of NFκB binding motifs within the 5′LTR. Although this sequence is different from the NFκB binding motifs found within the human genes,Citation21,Citation35,Citation36 we felt it was important to exclude any effect on NFκB driven genes. Finally, we wished to exclude any evidence of sequence non-sequence off-target effects mediated by the RNA species binding to innate surveillance mechanisms, such as RIG-I and TLRs 7 and 8, which strongly induce IFN.
There are two types of off-target effects associated with the use of dsRNAs: sequence specific off-target effects and sequence non-specific off-target effects. Sequence specific off-target effects are mediated by partial sequences of siRNA cross interfering with genes other than the targeted gene. This type of effect was reported initially in the cytoplasmic PTGS pathway inducing mRNA degradation,Citation37–Citation40 but also more recently has been reported in the nucleus acting through a TGS pathway.Citation11,Citation12 To address the sequence specificity of observed suppression we conducted three separate sets of experiments. Firstly, we varied the sequence expressed by the shRNAs by mutating 2 or 3 nucleotides within the sense/antisense sequences of shPromA. We created stable transfectants expressing each of these variants and then infected these MOLT-4 cells with HIV-1 IIIB. These mutations ablated the suppressive effects achieved by shPromA. This reduced efficacy was seen with as little as 2 nucleotide changes in the sequence of shPromA. We also tested the ability of the shPromA to inhibit HIV-2 replication, which has a sequence that differs by 5 nucleotides in the targeted NFκB binding region of its 5′LTR and showed that shPromA did not suppress HIV-2 replication.
Taken together these experiments strongly suggest that shPromA induces sequence specific TGS. However, these experiments did not absolutely exclude a sequence specific off-target effect. We next explored the hypothesis that because PromA sequence targets the HIV-1 NFκB binding motif, this RNA sequence might induce modulation of expression of other NFκB driven genes. We addressed this in several ways. We demonstrated that the surface expression of three of important immunomodulatory, NFκB dependent genes, CD54, CD38 and CD25, did not vary between the original MOLT-4 cell and those transduced with shPromA or the M1 to M4 variants. We also screened for the level of mRNA expression of 86 NFκB driven genes in MOLT-4 transduced with shPromA, mutant shPromA-M2 or scrambled shPromA, and again failed to show any substantial modulation of expression in any of these genes, strongly suggesting that the effect of shPromA is highly sequence specific.
While our current observations strongly support a sequence specific TGS effect of the shPromA, others have reported that siRNA-mediated TGS effects may be the result of sequence-specific off-target effects.Citation11,Citation12 These observations support the use of carefully chosen controls in these types of experiments. Clearly, it is possible within the one promoter to target sequences that will induce no effect and others that will induce an effect by off-target effects. Our previously published data demonstrated that sequences 60 base pairs up or downstream of the PromA target sequence fail to induce TGS,Citation21,Citation24 while sequences 100 base pairs upstream have been reported by others to induce TGS.Citation41 More recently, another article reported that TGS is a consequence of induction of sequence specific off-target effects.Citation11 This study used luciferase expression constructs as a read out for siRNA-induced TGS of human vascular endothelial growth factor (VEGF) promoter and the HIV promoter (HIVSF2-LTR). In this study siRNA with 2 nucleotides deleted from the target sequence in the VEGF promoter region and a five-base mismatched siRNA in HIVSF2-LTR promoter induced TGS at levels similar to that achieved by perfectly matched siRNAs. The investigators hypothesized that siRNA-mediated inhibition was not through a direct sequence specific effect, but rather VEGF and HIVSF2-LTR transcription was being regulated by modulation of expression of transcription factors, which was in turn controlled by the antisense strand of siRNAs acting as miRNA targeting “seed” sequences within the 3′ UTR sequence of these genes, inducing gene inhibition. However, the conclusions reported were based on experiments using an alternate and mismatched siRNA LTR247 sequence, with the 8th base from the 5′end of the sense strand changed from “U” to “A”, and not the sequence-specific LTR247 employed in previous reports.Citation11,Citation12,Citation41 Therefore, the conclusions regarding the mechanism of action of this siRNA must be questioned or at least interpreted with extreme caution. Due to the apparent controversy of siRNA-mediated TGS, it is critical that potential siRNAs are screened, not only for their sequence specificity within the targeted genome via searches such as basic local aliment search tool (BLAST), but should also be designed to avoid miRNA-like effects.
Further, both our group and others have repeatedly reported that only specific siRNAs are able to induce transcriptional suppression of the targeted promoter and that other siRNAs targeting the same promoter do not induce TGS.Citation12,Citation41–Citation43 It is also clearly reported that the efficacy of sequence specific induction of TGS by siRNA has been shown to be dependent upon the region or specific sequence targeted within a promoter. One of the clearest demonstrations of variable effect is described in the context of siRNA-mediated TGS of the progesterone receptor in breast cancer cells.Citation44
The other type of off-target-effect we considered was sequence non-specific off-target effects. Firstly, we considered whether there was modulation of molecules that could directly affect viral entry. There was no variation in the surface expression of CD4 or either of the two HIV-1 co-receptors, CCR5 and CXCR4, on the surface of MOLT-4 cells. This result is similar to what we have previously reported in MAGIC-5 cells.Citation21 We then looked for evidence of shRNAs triggering an innate host defense response through either RIG-ICitation45 or TLR3, 7 and 8.Citation13–Citation18 Firstly, the levels of IFNα, β and γ were not altered by shPromA, strongly suggesting no significant engagement of either RIG-I or the TLRs.Citation13–Citation16 Secondly, there was no induction of IFNα response genes: ISG20, Viperin or IFIT1. Further, we did not detect any activation of the PKR pathway. These data suggest that shPromA does not induce sequence non-specific off-target effects in MOLT-4 cells. These experiments strongly argue that the viral suppression observed in this model is not due to sequence non-specific off-target effects. It is well known that individual siRNA duplexes vary significantly in their capacity to induce these host defense pathways.Citation15,Citation46 shPromA does not contain the nucleic acid motifs that are known to predispose to engagement by TLRs, such as 5′-UGU-3′, triggering expression of IFNα or IL-6.Citation15,Citation46–Citation50 Additionally, both delivery of siRNA and shRNA via lentiviral vectors have been shown to reduce the propensity for induction of the IFN response.Citation42,Citation51–Citation53 Further study has shown that stable expression of shRNA does not induce an IFN response in CD34+ progenitor cells in vitroCitation54 or in an in vivo mouse model of siRNA-mediated gene silencing.Citation55 Additionally, more than a dozen siRNA lead discoveries are under evaluation in in vivo pharmacological studies and preclinical safety evaluations.Citation56 While this report is restricted to the effect of our shRNAs in MOLT-4 cells the results are supported by similar results obtained from the more limited analyses previously performed in HeLa cell lines.Citation21,Citation23 Clearly, further evaluation will be conducted using other T-cell lines and primary cells in order to assess sequence specific and non-specific off-target effects induced by siPromA/shPrmA.
Current combination antiretroviral therapy (cART) has limitations in HIV-1 treatment. Although cART can achieve marked reduction in HIV-1 plasma viral load and has markedly improved morbidity and mortality,Citation57–Citation63 it does not substantially impact upon the highly stable reservoir of latently infected resting CD4+ T cells.Citation64–Citation66 These resting memory CD4+ T cells survive for years, with a half-life estimated at 44 months.Citation67–Citation69 This rate of decay means that elimination of the virus from this compartment may need more than 60 years of continuous cART treatment, which is at least equivalent to the lifespan of most infected individuals.Citation70–Citation72 New approaches are required to combat HIV-1 reservoirs and shRNA-induced TGS holds substantial promise as one of these novel approaches. The data presented here definitively shows that the TGS effect can be induced with exquisite specificity and produce little or no off-targeted effects in these in vitro systems. For future therapeutic applications, the efficacy and specificity of the shRNA-induced TGS approach needs to be confirmed in vivo.
Materials and Methods
Construction of shRNA-expression retroviral vector.
The retroviral vectors (Retrovirus Packaging Kit Ampho, TAKARA Bio Inc.,) expressing four shRNAs: shPromA-M1, M2, M3, M4 were constructed using the same methodology to construct shPromA and shPromA-Sc as previously described in reference Citation24, using the following sense and antisense DNA oligomers: shPromA M1 sense; 5′-GAT CCG AAA CTT TCC GCT GGG GAC TTC TGT GAA GCC ACA GAT GGG AAG TCC CCA GCG GAA AGT TTC TTT TTT AT, shPromA M1 antisense; 5′-GCT ATT TTT TCT TTG AAA GGC GAC CCC TGA AGA CAC TTC GGT GTC TAC CCT TCA GGG GTC GCC TTT CAA AGC, shPromA M2 sense; 5′-GAT CCG GGA CTT TAA GCT GGG GAC TTC TGT GAA GCC ACA GAT GGG AAG TCC CCA GCT TAA AGT CCC TTT TTT AT, shPromA M2 antisense; 5′-GCC CTG AAA TTC GAC CCC TGA AGG GTA GAC ACC GAA GTG TCT TCA GGG GTC GAA TTT CAG GGA AAA AAT AGC, shPromA M3 sense; 5′-GAT CCG GGA CTT TCC ACT GGG GCG TTC TGT GAA GCC ACA GAT GGG AAC GCC CCA GTG GAA AGT CCC TTT TTT AT, shPromA M3 antisense; 5′-GCT ATT TTT TCC CTG AAA GGT GAC CCC GCA AGA CAC TTC GGT GTC TAC CCT TGC GGG GTC ACC TTT CAG GGC, shPromA M4 sense; 5′-GAT CCA GGA CTT CCG CTG GGG ACT TCT GTG AAG CCA CAG ATG GGA AGT CCC CAG CGG AAG TCC TTT TTT TAT, shPromA M4 antisense; 5′-GCT ATT TTT TTC CTG AAG GCG ACC CCT GAG GGT AGA CAC CGA AGT GTC TTT CAG GGG TCG CCT TCA GGA C.
Cell culture, HIV-1 infection and reverse transcriptase assay.
MOLT-4 cells were transduced as previously described in reference Citation24. The MOLT-4 cells expressing shRNA were cultured in RPMI 1640 medium with 10% fetal calf serum with 100 units/mL penicillin, 100 µg/mL streptomycin and 500 µg/mL G418. The stable transfectants were established for a month by culture under selection of G418. MOLT-4 cells (5 x 105 cells) were incubated with HIV-1 IIIB (infecting dose equivalent to 4.4 x 107 copies of HIV-RNA). After 2 h, the cells were washed with PBS three times and then cultured in 6-well plates. HIV-1 replication was detected by measuring reverse transcriptase (RT) activity in culture supernatants as described previously in references Citation73–Citation75.
Sense, loop and antisense strand detection in shRNA transcripts.
Extraction of cell associated RNA from the MOLT-4 cells was carried out using the SV Total RNA Isolation kit (Promega, Alexandria, Australia). cDNA was synthesized using miScript Reverse Transcription Kit (QIAGEN, Doncaster, Australia). The detection of the transcribed cDNA was carried out by end-point PCR reactions using a primer set consisting of the reverse primer targeting the universal tag and the forward primer targeting either the sense strand (ssp), the loop (lsp), or the antisense strand (asp) of the shRNA. These PCRs were performed with AccuPrime Taq DNA Polymerase (Invitrogen, Mulgrave, Australia) using Mastercycler PCR instrument (Eppendorf, North Ryde, Australia) with reaction conditions of 3 min at 94° C followed by 40 cycles of a three step PCR: 15 sec at 94° C, 20 sec at 58° C, 20 sec at 68° C. Sequences of ssp, lsp and asp were as follows. PromA ssp: 5′-TTC CGC TGG GGA CTT-3′, PromA M2 ssp: 5′-GGA CTT TAA GCT GGG GAC TT-3′, PromA M3 ssp: 5′-TTC CAC TGG GGC GTT-3′, PromA M4 ssp: 5′-TTC CGCTGG GGA CTT-3′, PromA Sc ssp: 5′-CTG GGA CGT GTG CCT G-3′, Universal lsp; 5′-CTG TGA AGC CAC AGA TGG G-3′, Prom-A asp: 5′-CCA GCG GAA AGT CCC-3′, Prom-A M1 asp: 5′-TCC CCA GCG GAA AGT TT-3′, Prom-A M2 asp: 5′-GGG AAG TCC CCA GCT T-3′, Prom-A M3 asp: 5′-GGA ACG CC CCA GTG3′ Prom-A M4 asp: 5′-CCC AGC GGA AGT CCT-3′, Prom-A Scram asp; 5′-GGC ACA CGT CCC AGC-3′. In addition, Real-Time PCR analysis was conducted for quantification detection of cleaved/matured siRNA. cDNA reaction was carried out with 84 ng of total RNA extracted from the stably transfected MOLT-4 cells, followed by RT-PCR analysis with miScript SYBR Green PCR Kit (QIAGEN, Doncaster, Australia) with the primer set of the universal tag and the loop specific primer using IQ5 thermocycler (Bio-Rad, Gladesville, Australia) with reaction conditions of 15 min 94° C followed by 40 cycle of three step PCR: 10 sec at 94° C, 20 sec at 58° C, 20 sec at 70° C.
Flow cytometry analysis.
Flow cytometric estimation of surface expression of CD4, CCR5, CXCR4, CD54, CD38 and CD25 on MOLT-4 cells was carried out as previously described using the following directly conjugated antibodies:Citation21 CD4-ECD, CCR5-FITC, CXCR4-APC, CD38-PE-Cy3, CD25-PE-Cy7, CD54-PE (Becton Dickinson, North Ryde, Australia) and analyzed on a BD LSR2 (Becton Dickinson, North Ryde, Australia).
Comparison of 86 NFκB driven genes by real-time PCR.
cDNA reactions were carried out using the First Strand cDNA Synthesis kit (QIAGEN, Doncaster, Australia) with 1.5 µg of RNA extracted from the stably transfected MOLT-4 expressing PromA, PromA-M2 or PromA Scrambled. After cDNA was generated, SYBR-Green based quantitative Real-Time PCR assays detected expression levels of 86 NFκB driven genes using a 96 well based kit (NFκB Signaling Pathway PCR Array, QIAGEN, Doncaster, Australia) using the IQ5 (Bio-Rad, Gladesville, Australia). Results were generated using a web-based analysis procedure available at: (www.sabiosciences.com/pcr/arrayanalysis.php). Each run was validated using several house-keeping genes, included DNA contamination controls. The expression of five house-keeping genes, including beta-2-microglobulin and beta-Actin, was used to normalize PCR array data. Ct values of the house keeping genes did not differ by more than one cycle across the compared samples. TNFα at final concentration of 10 ng/mL (R&D systems: Bio-Scientific, Gymea, Australia) was used as a positive control for activation of NFκB pathways, RNA was extracted 6 h post treatment of MOLT-4 cells.
Interferon stimulated gene expression by real-time PCR.
The mRNA expression levels of the IFNα response genes, ISG20, Viperin and IFIT1 were detected by SYBR-Green based quantitative Real-Time PCR assays, as previously described in reference Citation76, using the following primer pairs. Viperin; Fwd 5′-GTG AGC AAT GGA AGC CTG ATC-3′ and Rev 5′-GCT GTC ACA GGA GAT AGC GAG AA-3′, IFIT1; Fwd 5′-AAC TTA ATG CAG GAA GAA CAT GAC AA-3′ and Rev 5′-CTG CCA GTC TGC CCA TGT G-3′, ISG20; Fwd 5′-TCG TTG CAG CCT CGT GAA C-3′ and Rev 5′-TCC CTC AGG CCG GAT GA-3′. ISG20, Viperin and IFIT1 have been used to detect INFα response induced by RNA duplexes previously in reference Citation15 and Citation77.
PKR immunoblotting.
Phosphorylated dsRNA-dependent protein kinase R (PKR) was detected as previously described in reference Citation78. Briefly, cells were washed twice with PBS and lysed in cold lysis buffer (Reporter Gene Assay Lysis Buffer, Roche Diagnostics, Castle Hill, Australia) with protease inhibitor cocktail and phosphatase inhibitors (Roche Diagnostics, Castle Hill, Australia). The amount of protein within the whole cell extract was measured by the Bradford assay (Bio-Rad, Gladesville, Australia). 150 µg of cell extract was resolved by SDS-PAGE then analyzed by immunoblotting with antibodies against phosphorylated PKR (rabbit polyclonal antibody against PKR [pT451], BioSource; Invitrogen, Mulgrave, Australia) and β-Actin (rabbit polyclonal against β-actin, Sigma-Aldrich, Castle Hill, Australia).
Statistical analysis.
RT values are given as mean and SEM and were tested for significance using a paired, two-tailed t-test. A p-value < 0.05 was considered statistically significant. All analyses were performed using Graphpad Prism Version 5.0a (Graphpad Software, San Diego, CA).
Figures and Tables
Figure 1 Sequence specific inhibition of HIV-1 replication in MOLT-4 cells expressing shRNA targeting HIV-1 promoter region. (A) The sequence targeted by the shPromA within HIV-1 promoter region of U3 is indicated in blue. The alignment of shRNA targeting NFκB sites (shPromA), four variants (shPromA M1–4), and scrambled control of shPromA (shPromA Sc) is shown below. Mismatches are indicated in red. Underlining indicates NFκB binding sites in the HIV-1 promoter region. The start site of HIV-1 mRNA transcription is indicated by an arrow. (B) Sequence dependent inhibition of HIV-1 replication was achieved. HIV replication was detected by reverse transcriptase (RT) assay in culture supernatants post infection at days 4, 7, 11. The mean values of three independent experiments are plotted. (C) Alignment of HIV-1 and HIV-2 NFκB binding regions. NFκB binding regions are underlined. Sequence targeted by shPromA is highlighted in blue. The letters in red indicate sequence differences in HIV-2 U3 promoter region. (D) Sequence dependent inhibition of viral infection was achieved. HIV-1 and HIV-2 replication was detected by RT levels in culture supernatants post infection at days 2, 6, 10. MOLT-4 shPromA cells were able to suppress HIV-1 infection but failed to suppress HIV-2 replication. MOLT-4 cells not expressing shPromA showed increasing levels of HIV-1 and HIV-2 over the 10 day time course. The mean values of three independent experiments are plotted.
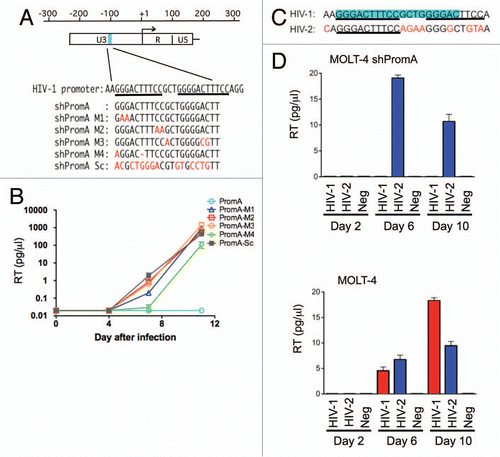
Figure 2 Evidence of shRNA transcription in MOLT-4 cells. (A) A schematic of the procedure used for detection of antisense strand expression following shRNA transcription. The shRNA expression unit consisting of sense, loop, and antisense strands of shRNA sequences is transcribed under the control of the U6 promoter. RNA was extracted from MOLT-4 cells expressing various shPromA constructs, followed by two step in vitro reaction of polyadenylation mediated by the polynucleotide adenylyltransferase and cDNA synthesis from 3′ end of the shRNA sequence using an oligo(dT) primer with a universal tag. Sequence specific PCR was used to detect the newly synthesized cDNA using the primer for the universal tag paired with specific antisense primers (asp). Detection of the loop strand and the sense strands employed a similar approach. (B) Alignment of the shPromA and variant sequences with the positions of specific 5′ PCR primers. The loop sequence is indicated by blue letters. The arrows indicate the positions of antisense specific primers (asp), sense specific primers (ssp) and loop specific primer (lsp). (C) Antisense specific primers specifically detect only the matched antisense strand of shRNA transcript. The ethidium bromide stained PCR bands were obtained after PCR with each specific antisense specific primer and universal primer. The antisense specific primers (asp) are listed on the left side of the gel image. Numbers 1–6 represent the MOLT-4 cells expressing shPromA or variants.
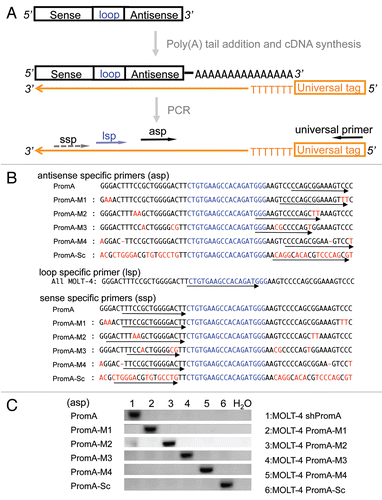
Figure 3 Evidence for efficient generation of mature/processed forms of double stranded siRNA from shRNA in MOLT-4 cells. (A) The schematic picture shows that the antisense strand and sense strand of shRNA transcripts with termination oligo-(U) sequences hybridize to form double-stranded with loop form of shRNA. A ribonuclease then cleaves the loop structure at indicated sites (indicated by red triangles). (B) The shRNA transcripts with loop structure can exist in four possible states without or with cleavage by ribonuclease as indicated in [1–4]. Estimated amplicon sizes are listed along with each of three specific primers. If the uncleaved shRNA [1] was dominant, the predicted size of PCR product amplified with ssp primer would be a longer amplicon (approximately 160 bp) compared with that detected with asp primer resulting in a band of approximately 120 bp in size. The predicted size of amplicon generated by the lsp primer would be intermediate in size (around 140 bp). If mature/processed double stranded siRNAs [2–4] are dominant, similar short amplicons would be generated by all each of ssp, asp and lsp primer sets, because polyadenylation is initiated at the 3′end of cleaved sense and loop strand.Citation79 (C) PCR amplicons generated using the strand specific primers in the MOLT-4 expressing shPromA and variants. Similar sized PCR amplicons were observed using each of three specific primer sets in the MOLT-4 cells. These results indicate complete processing of shRNA to siRNA. (D) Similar amounts processed/cleaved shRNA transcripts were expressed in all MOLT-4 cells. The cDNA reaction was carried out with 84 ng of total RNA as in , followed by Real-Time PCR analysis with universal loop specific primer. Estimated fold changes of cleaved loop structure of shRNA transcript in the MOLT-4 cells were shown relative to shPromA scrambled control based on delta-ct change. Means and standard errors of four independent experiments were shown. ns:Not significant, *p < 0.05.
![Figure 3 Evidence for efficient generation of mature/processed forms of double stranded siRNA from shRNA in MOLT-4 cells. (A) The schematic picture shows that the antisense strand and sense strand of shRNA transcripts with termination oligo-(U) sequences hybridize to form double-stranded with loop form of shRNA. A ribonuclease then cleaves the loop structure at indicated sites (indicated by red triangles). (B) The shRNA transcripts with loop structure can exist in four possible states without or with cleavage by ribonuclease as indicated in [1–4]. Estimated amplicon sizes are listed along with each of three specific primers. If the uncleaved shRNA [1] was dominant, the predicted size of PCR product amplified with ssp primer would be a longer amplicon (approximately 160 bp) compared with that detected with asp primer resulting in a band of approximately 120 bp in size. The predicted size of amplicon generated by the lsp primer would be intermediate in size (around 140 bp). If mature/processed double stranded siRNAs [2–4] are dominant, similar short amplicons would be generated by all each of ssp, asp and lsp primer sets, because polyadenylation is initiated at the 3′end of cleaved sense and loop strand.Citation79 (C) PCR amplicons generated using the strand specific primers in the MOLT-4 expressing shPromA and variants. Similar sized PCR amplicons were observed using each of three specific primer sets in the MOLT-4 cells. These results indicate complete processing of shRNA to siRNA. (D) Similar amounts processed/cleaved shRNA transcripts were expressed in all MOLT-4 cells. The cDNA reaction was carried out with 84 ng of total RNA as in Figure 1C, followed by Real-Time PCR analysis with universal loop specific primer. Estimated fold changes of cleaved loop structure of shRNA transcript in the MOLT-4 cells were shown relative to shPromA scrambled control based on delta-ct change. Means and standard errors of four independent experiments were shown. ns:Not significant, *p < 0.05.](/cms/asset/efced49e-9d30-43c5-ba48-a335ded47dc1/krnb_a_10916264_f0003.gif)
Figure 4 MOLT-4 cells expressing shPromA or variants display no change in surface expression of HIV receptor/coreceptor molecules CD4, CCR5, CXCR4, compared to untransduced MOLT-4 cells. Flow cytometry histograms (representative of 3 separate experiments) of CD4, CCR5 and CXCR4 revealed no difference in expression of these three surface molecules.
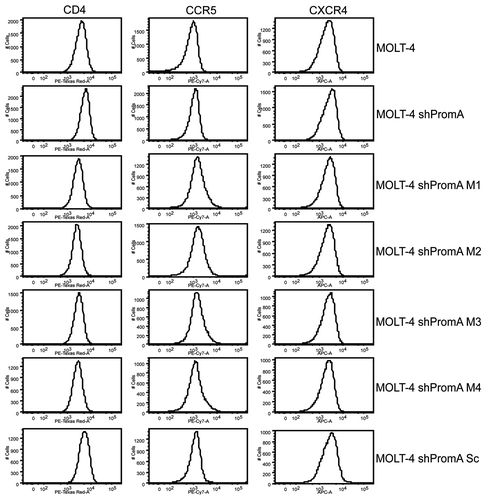
Figure 5 There is no significant difference in expression of mRNA from 86 NFκB driven genes in MOLT-4 cells stably transduced with shPromA, shPromA-M2 or shPromA-Sc. (A) Scatter plot of a log transformation of the relative expression of each gene (2 delta-Ct) comparing MOLT-4 expressing shPromA and MOLT-4 expressing shPromA-Sc. cDNA was synthesized from extracted RNA, followed by Real-Time PCR analysis. There was no significant difference in induction of NFκB driven genes by shPromA in MOLT-4 cells. (B) Scatter plot of a log transformation of the relative expression of each gene (2delta-Ct) between MOLT-4 expressing shPromA-M2 cells and MOLT-4 expressing shPromA-Sc cells. (C) Scatter plot of a log transformation of the relative expression of each gene (2delta-Ct) between TNFα stimulated MOLT-4 expressing shPromA cells and un-stimulated MOLT-4 shPromA cells. RNA was extracted 6 hours post stimulation for analysis. Most of NFκB driven genes were activated by TNFα stimulation. Plots for IFNα1, β1, γ, TNFRSF10A are indicated by arrows. The parallel two lines indicate a two-fold change in gene expression threshold. The mean of the triplicate experiments of each gene was used for this analysis.
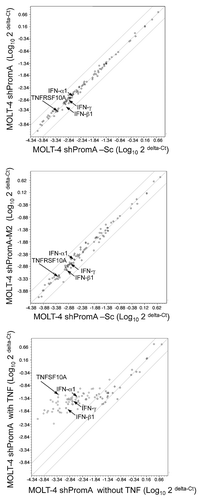
Figure 6 No significant off-target effects are induced by shPromA as determined by IFNα response genes and activation of PKR. (A) Three IFNα response genes (ISG20, Viperin and IFIT1) were not elevated in cells transduced with shPromA. The fold change for each mRNA is shown. Mean and standard error mean are plotted from triplicate experiments and the data was normalized to the value from MOLT-4 cells. The positive control was generated by treatment of cells for 24 h with IFNα (500 IU/mL). (B) Activation of phosphorylated PKR was not detected in shPromA-transduced cells. Western blot data were obtained from 150 µg of cellular extract. β-actin was used as loading control. Treatment with poly (I:C) (10 ng/mL for 6 h) was used as a positive control.
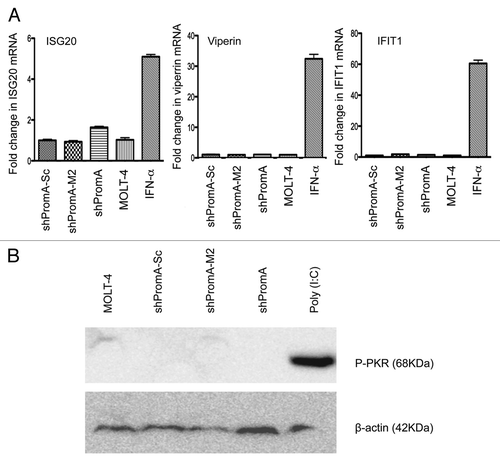
Additional material
Download Zip (3.7 MB)Acknowledgments
This paper was funded from the following sources: the Australian Government Department of Health and Ageing; grants from the National Health and Medical Research Council including a Practitioner Fellowship (A.K.) and a Project grant (K.S., C.H.). The views expressed in this publication do not necessarily represent the position of the Australian Government. The Kirby Institute is affiliated with the Faculty of Medicine, University of New South Wales.
References
- Hutvagner G, Simard MJ. Argonaute proteins: key players in RNA silencing. Nat Rev Mol Cell Biol 2008; 105:512 - 517
- Peters L, Meister G. Argonaute proteins: mediators of RNA silencing. Mol Cell 2007; 26:611 - 623
- Miyoshi K, Uejima H, Nagami-Okada T, Siomi H, Siomi MC. In vitro RNA cleavage assay for Argonautefamily proteins. Methods Mol Biol 2008; 442:29 - 43
- Jagannath A, Wood MJ. Localization of Double-stranded siRNA to Cytoplasmic P-Bodies Is Ago2dependent and Results in Upregulation of GW182 and Ago2. Mol Biol Cell 2008;
- Qi HH, Ongusaha PP, Myllyharju J, Cheng D, Pakkanen O, Shi Y, et al. Prolyl 4-hydroxylation regulates Argonaute 2 stability. Nature 2008;
- Morris KV, Santoso S, Turner AM, Pastori C, Hawkins PG. Bidirectional transcription directs both transcriptional gene activation and suppression in human cells. PLoS Genet 2008; 4:1000258
- Malecova B, Morris KV. Transcriptional gene silencing through epigenetic changes mediated by non-coding RNAs. Curr Opin Mol Ther 2010; 12:214 - 222
- Sibley CR, Seow Y, Wood MJ. Novel RNA-based strategies for therapeutic gene silencing. Mol Ther 2010; 18:466 - 476
- Suzuki K, Kelleher AD. Transcriptional regulation by promoter targeted RNAs. Curr Top Med Chem 2009; 9:1079 - 1087
- Suzuki K, Kelleher AD. Lessons from viral latency in T cells: manipulating HIV-1 transcription by siRNA. HIV Therapy 2010; 4:199 - 213
- Moses J, Goodchild A, Rivory LP. Intended transcriptional silencing with siRNA results in gene repression through sequence-specific off-targeting. RNA 2010; 16:430 - 441
- Weinberg MS, Barichievy S, Schaffer L, Han J, Morris KV. An RNA targeted to the HIV-1 LTR promoter modulates indiscriminate off-target gene activation. Nucleic Acids Res 2007; 35:1 - 10
- Olejniczak M, Galka P, Krzyzosiak WJ. Sequencenon-specific effects of RNA interference triggers and microRNA regulators. Nucleic Acids Res 2010; 38:1 - 16
- Reynolds A, Anderson EM, Vermeulen A, Fedorov Y, Robinson K, Leake D, et al. Induction of the interferon response by siRNA is cell type- and duplex length-dependent. RNA 2006; 12:988 - 993
- Judge AD, Sood V, Shaw JR, Fang D, McClintock K, MacLachlan I. Sequence-dependent stimulation of the mammalian innate immune response by synthetic siRNA. Nat Biotechnol 2005; 23:457 - 462
- Kim DH, Longo M, Han Y, Lundberg P, Cantin E, Rossi JJ. Interferon induction by siRNAs and ssRNAs synthesized by phage polymerase. Nat Biotechnol 2004; 22:321 - 325
- de Veer MJ, Sledz CA, Williams BR. Detection of foreign RNA: implications for RNAi. Immunol Cell Biol 2005; 83:224 - 228
- Sledz CA, Holko M, de Veer MJ, Silverman RH, Williams BR. Activation of the interferon system by short-interfering RNAs. Nat Cell Biol 2003; 5:834 - 839
- Tian B, Mathews MB. Functional characterization of and cooperation between the double-stranded RNA-binding motifs of the protein kinase PKR. J Biol Chem 2001; 276:9936 - 9944
- Su Q, Wang S, Baltzis D, Qu LK, Wong AH, Koromilas AE. Tyrosine phosphorylation acts as a molecular switch to full-scale activation of the eIF2alpha RNA-dependent protein kinase. Proc Natl Acad Sci USA 2006; 103:63 - 68
- Suzuki K, Shijuuku T, Fukamachi T, Zaunders J, Guillemin G, Cooper D, Kelleher A. Prolonged transcriptional silencing and CpG methylation induced by siRNAs targeted to the HIV-1 promoter regiony. J RNAi and Gene Silencing 2005; 1:66 - 78
- Lim HG, Suzuki K, Cooper DA, Kelleher AD. Promoter-targeted siRNAs Induce Gene Silencing of Simian Immunodeficiency Virus (SIV) Infection In Vitro. Mol Ther 2008; 16:565 - 570
- Suzuki K, Juelich T, Lim H, Ishida T, Watanebe T, Cooper DA, et al. Closed chromatin architecture is induced by an RNA duplex targeting the HIV-1 promoter region. J Biol Chem 2008; 283:23353 - 23363
- Yamagishi M, Ishida T, Miyake A, Cooper DA, Kelleher AD, Suzuki K, Watanabe T. Retroviral delivery of promoter-targeted shRNA induces long-term silencing of HIV-1 transcription. Microbes Infect 2009; 11:500 - 508
- Davis S, Propp S, Freier SM, Jones LE, Serra MJ, Kinberger G, et al. Potent inhibition of microRNA in vivo without degradation. Nucleic Acids Res 2009; 37:70 - 77
- Chomczynski P, Sacchi N. The single-step method of RNA isolation by acid guanidinium thiocyanatephenol-chloroform extraction: twenty-something years on. Nat Protoc 2006; 1:581 - 585
- Tiscornia G, Singer O, Verma IM. Design and cloning of lentiviral vectors expressing small interfering RNAs. Nat Protoc 2006; 1:234 - 240
- Brummelkamp TR, Bernards R, Agami R. A system for stable expression of short interfering RNAs in mammalian cells. Science 2002; 296:550 - 553
- Frankova D, Zidek Z. IFN gamma-induced TNFalpha is a prerequisite for in vitro production of nitric oxide generated in murine peritoneal macrophages by IFN gamma. Eur J Immunol 1998; 28:838 - 843
- Kuribayashi K, Krigsfeld G, Wang W, Xu J, Mayes PA, Dicker DT, et al. TNFSF10 (TRAIL), a p53 target gene that mediates p53-dependent cell death. Cancer Biol Ther 2008; 7:2034 - 2038
- Lenz P, Bacot SM, Frazier-Jessen MR, Feldman GM. Nucleoporation of dendritic cells: efficient gene transfer by electroporation into human monocyte-derived dendritic cells. FEBS Lett 2003; 538:149 - 154
- Landi A, Babiuk LA, van Drunen Littel-van den Hurk S. High transfection efficiency, gene expression and viability of monocyte-derived human dendritic cells after nonviral gene transfer. J Leukoc Biol 2007; 82:849 - 860
- Verdel A, Jia S, Gerber S, Sugiyama T, Gygi S, Grewal SI, Moazed D. RNAi-mediated Targeting of Heterochromatin by the RITS Complex. Science 2004; 303:672 - 676
- Motamedi MR, Verdel A, Colmenares SU, Gerber SA, Gygi SP, Moazed D. Two RNAi complexes, RITS and RDRC, physically interact and localize to noncoding centromeric RNAs. Cell 2004; 119:789 - 802
- Montano MA, Kripke K, Norina CD, Achacoso P, Herzenberg LA, Roy AL, Nolan GP. NFkappaB homodimer binding within the HIV-1 initiator region and interactions with TFII-I. Proc Natl Acad Sci USA 1996; 93:12376 - 12381
- Kashanchi F, Shibata R, Ross EK, Brady JN, Martin MA. Second-site long terminal repeat (LTR) revertants of replication-defective human immunodeficiency virus: effects of revertant TATA box motifs on virus infectivity, LTR-directed expression, in vitro RNA synthesis, and binding of basal transcription factors TFIID and TFIIA. J Virol 1994; 68:3298 - 3307
- Scacheri PC, Rozenblatt-Rosen O, Caplen NJ, Wolfsberg TG, Umayam L, Lee JC, et al. Short interfering RNAs can induce unexpected and divergent changes in the levels of untargeted proteins in mammalian cells. Proc Natl Acad Sci USA 2004; 101:1892 - 897
- Jackson AL, Bartz SR, Schelter J, Kobayashi SV, Burchard J, et al. Expression profiling reveals off-target gene regulation by RNAi. Nat Biotechnol 2003; 21:635 - 637
- Jackson AL, Burchard J, Schelter J, Chau BN, Cleary M, Lim L, Linsley PS. Widespread siRNA “off-target” transcript silencing mediated by seed region sequence complementarity. RNA 2006; 12:1179 - 1187
- Cullen BR. Enhancing and confirming the specificity of RNAi experiments. Nat Methods 2006; 3:677 - 681
- Weinberg MS, Villeneuve LM, Ehsani A, Amarzguioui M, Aagaard L, Chen ZX, et al. The antisense strand of small interfering RNAs directs histone methylation and transcriptional gene silencing in human cells. RNA 2006; 12:256 - 262
- Barichievy S, Saayman S, von Eije KJ, Morris KV, Arbuthnot P, Weinberg MS. The inhibitory efficacy of RNA POL III-expressed long hairpin RNAs targeted to untranslated regions of the HIV-1 5′ long terminal repeat. Oligonucleotides 2007; 17:419 - 431
- Castanotto D, Tommasi S, Li M, Li H, Yanow S, Pfeifer GP, Rossi JJ. Short hairpin RNA-directed cytosine (CpG) methylation of the RASSF1A gene promoter in HeLa cells. Mol Ther 2005; 12:179 - 183
- Janowski BA, Huffman KE, Schwartz JC, Ram R, Hardy D, Shames DS, et al. Inhibiting gene expression at transcription start sites in chromosomal DNA with antigene RNAs. Nat Chem Biol 2005; 1:216 - 222
- Marques JT, Devosse T, Wang D, Zamanian-Daryoush M, Serbinowski P, Hartmann R, et al. A structural basis for discriminating between self and nonself double-stranded RNAs in mammalian cells. Nat Biotechnol 2006; 24:559 - 565
- Sioud M. Induction of inflammatory cytokines and interferon responses by double-stranded and single-stranded siRNAs is sequence-dependent and requires endosomal localization. J Mol Biol 2005; 348:1079 - 1090
- Diebold SS, Kaisho T, Hemmi H, Akira S, Reis e Sousa C. Innate antiviral responses by means of TLR7mediated recognition of single-stranded RNA. Science 2004; 303:1529 - 1531
- Hornung V, Guenthner-Biller M, Bourquin C, Ablasser A, Schlee M, Uematsu S, et al. Sequence-specific potent induction of IFN alpha by short interfering RNA in plasmacytoid dendritic cells through TLR7. Nat Med 2005; 11:263 - 270
- Diebold SS, Massacrier C, Akira S, Paturel C, Morel Y, Reis e Sousa C. Nucleic acid agonists for Toll-like receptor 7 are defined by the presence of uridine ribonucleotides. Eur J Immunol 2006; 36:3256 - 3267
- Goodchild A, Nopper N, King A, Doan T, Tanudji M, Arndt GM, et al. Sequence determinants of innate immune activation by short interfering RNAs. BMC Immunol 2009; 10:40
- Konstantinova P, de Vries W, Haasnoot J, ter Brake O, de Haan P, Berkhout B. Inhibition of human immunodeficiency virus type 1 by RNA interference using long-hairpin RNA. Gene Ther 2006; 13:1403 - 1413
- Akashi H, Miyagishi M, Yokota T, Watanabe T, Hino T, Nishina K, et al. Escape from the interferon response associated with RNA interference using vectors that encode long modified hairpin-RNA. Mol Biosyst 2005; 1:382 - 390
- Saayman S, Barichievy S, Capovilla A, Morris KV, Arbuthnot P, Weinberg MS. The efficacy of generating three independent anti-HIV-1 siRNAs from a single U6 RNA Pol III-expressed long hairpin RNA. PLoS ONE 2008; 3:2602
- Robbins MA, Li M, Leung I, Li H, Boyer DV, et al. Stable expression of shRNAs in human CD34+ progenitor cells can avoid induction of interferon responses to siRNAs in vitro. Nat Biotechnol 2006; 24:566 - 571
- Heidel JD, Hu S, Liu XF, Triche TJ, Davis ME. Lack of interferon response in animals to naked siRNAs. Nat Biotechnol 2004; 22:1579 - 1582
- Vaishnaw AK, Gollob J, Gamba-Vitalo C, Hutabarat R, Sah D, Meyers R, et al. A status report on RNAi therapeutics. Silence 2010; 1:14
- Ho DD, Neumann AU, Perelson AS, Chen W, Leonard JM, Markowitz M. Rapid turnover of plasma virions and CD4 lymphocytes in HIV-1 infection. Nature 1995; 373:123 - 126
- Mellors JW, Rinaldo CRJ, Gupta P, White RM, Todd JA, Kingsley LA. Prognosis in HIV-1 infection predicted by the quantity of virus in plasma. Science 1996; 272:1167 - 1170
- Perelson AS, Neumann AU, Markowitz M, Leonard JM, Ho DD. HIV-1 dynamics in vivo: virion clearance rate, infected cell life-span and viral generation time. Science 1996; 271:1582 - 1586
- Gulick RM, Mellors JW, Havlir D, Eron JJ, Gonzalez C, McMahon D, et al. Treatment with indinavir, zidovudine and lamivudine in adults with human immunodeficiency virus infection and prior antiretroviral therapy. N Engl J Med 1997; 337:734 - 739
- Hammer SM, Squires KE, Hughes MD, Grimes JM, Demeter LM, Currier JS, et al. AIDS Clinical Trials Group 320 Study Team. A controlled trial of two nucleoside analogues plus indinavir in persons with human immunodeficiency virus infection and CD4 cell counts of 200 per cubic millimeter or less. N Engl J Med 1997; 337:725 - 733
- Perelson AS, Essunger P, Cao Y, Vesanen M, Hurley A, Saksela K, et al. Decay characteristics of HIV-1-infected compartments during combination therapy. Nature 1997; 387:188 - 191
- Palella FJJ, Delaney KM, Moorman AC, Loveless MO, Fuhrer J, Satten GA, et al. HIV Outpatient Study Investigators. Declining morbidity and mortality among patients with advanced human immunodeficiency virus infection. N Engl J Med 1998; 338:853 - 860
- Chun TW, Finzi D, Margolick J, Chadwick K, Schwartz D, Siliciano RF. In vivo fate of HIV-1infected T cells: quantitative analysis of the transition to stable latency. Nat Med 1995; 1:1284 - 1290
- Chun TW, Carruth L, Finzi D, Shen X, DiGiuseppe JA, Taylor H, et al. Quantification of latent tissue reservoirs and total body viral load in HIV-1 infection. Nature 1997; 387:183 - 188
- Finzi D, Hermankova M, Pierson T, Carruth LM, Buck C, Chaisson RE, et al. Identification of a reservoir for HIV-1 in patients on highly active antiretroviral therapy. Science 1997; 278:1295 - 1300
- Hammarlund E, Lewis MW, Hansen SG, Strelow LI, Nelson JA, Sexton GJ, et al. Duration of antiviral immunity after smallpox vaccination. Nat Med 2003; 9:1131 - 1137
- Lavergne E, Combadiere C, Iga M, Boissonnas A, Bonduelle O, Maho M, et al. Intratumoral CC chemokine ligand 5 overexpression delays tumor growth and increases tumor cell infiltration. J Immunol 2004; 173:3755 - 3762
- Riou C, Yassine-Diab B, Van grevenynghe J, Somogyi R, Greller LD, Gagnon D, et al. Convergence of TCR and cytokine signaling leads to FOXO3a phosphorylation and drives the survival of CD4+ central memory T cells. J Exp Med 2007; 204:79 - 91
- Finzi D, Blankson J, Siliciano JD, Margolick JB, Chadwick K, Pierson T, et al. Latent infection of CD4+ T cells provides a mechanism for lifelong persistence of HIV-1, even in patients on effective combination therapy. Nat Med 1999; 5:512 - 517
- Siliciano JD, Kajdas J, Finzi D, Quinn TC, Chadwick K, Margolick JB, et al. Long-term follow-up studies confirm the stability of the latent reservoir for HIV-1 in resting CD4+ T cells. Nat Med 2003; 9:727 - 728
- Strain MC, Gunthard HF, Havlir DV, Ignacio CC, Smith DM, Leigh-Brown AJ, et al. Heterogeneous clearance rates of long-lived lymphocytes infected with HIV: intrinsic stability predicts lifelong persistence. Proc Natl Acad Sci USA 2003; 100:4819 - 4824
- Suzuki K, Craddock BP, Okamoto N, Kano T, Steigbigel RT. Poly A-Linked Colorimetric Microtiter Plate Assay For HIV Reverse Transcriptase. J Virol Methods 1993; 44:189 - 198
- Suzuki K, Craddock BP, Kano T, Steigbigel RT. Chemiluminescent enzyme-linked immunoassay for reverse transcriptase, illustrated by detection of HIV reverse transcriptase. Anal Biochem 1993; 210:277 - 281
- Suzuki K, Saito T, Kondo M, Osanai M, Watanabe S, Kano T, et al. Poly A-linked non-isotopic microtiter plate reverse transcriptase assay for sensitive detection of clinical human immunodeficiency virus isolates. J Virol Methods 1995; 55:347 - 356
- Helbig KJ, Lau DT, Semendric L, Harley HA, Beard MR. Analysis of ISG expression in chronic hepatitis C identifies viperin as a potential antiviral effector. Hepatology 2005; 42:702 - 710
- Espert L, Rey C, Gonzalez L, Degols G, Chelbi-Alix MK, Mechti N, Gongora C. The exonuclease ISG20 is directly induced by synthetic dsRNA via NFkappaB and IRF1 activation. Oncogene 2004; 23:4636 - 4640
- Christensen HS, Daher A, Soye KJ, Frankel LB, Alexander MR, Laine S, et al. Small interfering RNAs against the TAR RNA binding protein, TRBP, a Dicer cofactor, inhibit human immunodeficiency virus type 1 long terminal repeat expression and viral production. J Virol 2007; 81:5121 - 5131
- Hurst DR, Edmonds MD, Scott GK, Benz CC, Vaidya KS, Welch DR. Breast cancer metastasis suppressor 1 upregulates miR-146, which suppresses breast cancer metastasis. Cancer Res 2009; 69:1279 - 1283