Abstract
MicroRNA (miRNA) genes produce three major RNA products; primary (pri-), precursor (pre-), and mature miRNAs. Each product includes sequences complementary to cognate targets, thus they all can in principle interact with the targets. In a recent study we showed that pri-miRNAs play a direct role in target recognition and repression in the absence of functional mature miRNAs. Here we examined the functional contribution of pri-miRNAs in target regulation when full-length functional miRNAs are present. We found that pri-let-7 loop nucleotides control the production of the 5’ end of mature miRNAs and modulate the activity of the miRNA gene. This insight enabled us to modulate biogenesis of functional mature miRNAs and dissect the causal relationships between mature miRNA biogenesis and target repression. We demonstrate that both pri- and mature miRNAs can contribute to target repression and that their contributions can be distinguished by the differences between the pri- and mature miRNAs’ sensitivity to bind to the first seed nucleotide. Our results demonstrate that the regulatory information encoded in the pri-/pre-miRNA loop nucleotides controls the activities of pri-miRNAs and mature let-7 by influencing pri-miRNA and target complex formation and the fidelity of mature miRNA seed generation.
Introduction
Animal genomes contain large number of microRNA (miRNA) genes that encode regulatory RNAs. At least three RNA species, including pri-miRNA, pre-miRNA, and mature miRNA, are made from miRNA genes through transcription and sequential endonucleolytic maturation steps.Citation1 Mature miRNAs are loaded into RNA-induced silencing complexes (RISC) and guide the RISC to cognate target mRNAs for degradation or translational repression.Citation2 Despite the progress in recent years, many important questions regarding mechanisms of action by miRNAs during target recognition and repression remain unresolved. In particular, one critical problem that has not been extensively studied is whether only the mature miRNA or whether multiple species produced by a miRNA gene contribute to target recognition and repression. The pri-, pre-, and mature miRNAs each contain identical seed sequences and, therefore, all have the potential to interact with target mRNAs. However, the majority of studies in the field have focused on the functional roles of mature miRNAs in target recognition and repression. The pri-miRNAs and premiRNAs are considered to be transitory intermediates in biogenesis of mature miRNAs and to have no direct role in target gene regulation. Could pri- and/or pre-miRNAs play critical roles in target recognition and repression given that they all contain the mature miRNA sequence? Clearly, structured pri- and pre-miR-NAs and linear ∼22 nucleotide mature miRNAs contain different sequence and structural information that can be utilized for gene regulation and these RNAs may act with distinct protein or RNA partners. Ignoring the function of structured pri- and/or pre-miRNAs may result in omission of critical regulatory information used in target recognition and the molecular steps required for target repression, thus hampering our understanding of the mechanisms of target recognition and repression.
Importantly, while mature miRNAs clearly function in target repression, so far there has been no evidence to rule out functional roles for pri- and/or pre-miRNAs in target recognition and repression. Deletions and mutations of specific miRNA genes result in defects in all three RNA species produced from the genes and thus the phenotypes observed for loss of miRNA genes cannot be definitively attributed to one of the miRNA species. Such possibility was thoroughly discussed in the paper describing the finding of prototype miRNA gene, lin-4.Citation3 Moreover, although transfected siRNA duplexes can silence targets,Citation4 such analyses do not demonstrate that these miRNA mimics are functionally equivalent to endogenous mature miRNAs, and can not rule out a function for pri/pre-miRNAs. Finally, although deletion of Drosha or Dicer has been used to attribute the function of miRNA genes to mature miRNAs and to rule out the function of pri- and pre-miRNA species, such analyses have intrinsic limitations. Both Dicer and Drosha play critical roles in biogenesis of nearly all miRNAs but are also known to have roles beyond those in miRNA biogenesis. Dicer-1 fragments chromosomal DNA during apoptosis,Citation5 and Drosha processes DGCR8 mRNA and many other cellular mRNAs.Citation6,Citation7 Thus, it is not possible to evaluate whether deletion of Dicer and Drosha may result in defects that compromise pathways required for precursor miRNA function.
Supporting the role of precursor miRNAs in target recognition and repression, we previously showed that regulatory information encoded outside the mature miRNA controls the distinct activities of murine mir-181a-1 and mir-181c independent of mature miRNA biogenesis.Citation8,Citation9 These findings raised the possibility that precursor forms of miRNAs, such as pri- and pre-miR-NAs, have direct roles in target recognition and repression. This possibility was further confirmed in a heterologous culture assay using the prototype miRNA gene C. elegans let-7 (cel-let-7) and a target reporter containing the lin-41 3′ UTR as a model.Citation9 In this system, pri-let-7 recognizes and represses target expression in the presence of truncated and non-functional mature let-7. Moreover, the pri-let-7 loop nucleotides modulate the formation of target and pri-miRNA complexes. These findings illustrate that the regulatory information encoded in primary miRNAs can be directly translated into function through interactions between structured pri-miRNAs and cognate targets in the absence of functional mature miRNAs. However, it is difficult to discern the functional contribution by pri- and mature miRNAs in the presence of full-length, functional mature miRNAs. In this study, we examined the functional contribution of pri-miRNAs to target recognition and repression when full-length functional miRNAs were present. We found that pri-let-7 loop nucleotides control the precision of the biogenesis of the 5′ end of mature miRNAs and modulate the activity of miRNA genes. We present evidence that both pri- and mature miRNA species contribute to target repression and show that their activities are distinguished by their differential sensitivity to pairing between the first seed nucleotide of the miRNA and the target.
Results
Pri/pre-let-7 loop nucleotides control target repression.
Given that pri-miRNA loops are important for activity of miRNA genes,Citation8,Citation9 we mutated pri-let-7 loop nucleotides () and tested the effects of these mutations on the activity of cel-let-7 in target repression (). Seed-dependent repression activity of cel-let-7 and its loop mutants were determined using Renilla luciferase reporters (Fig. S1) with a wild-type lin-41 3′ UTRCitation10 (lin-41_LCS-wt) or with a mutant UTR that abrogate pairings to the let-7 seed nucleotides (lin-41_LCS-sm). We found that pri-let-7 loop nucleotides have both positive and negative effects on the activity of cel-let-7 (). Some mutations (LP2, LP3, and LP5) resulted in slight decreases in repression, whereas LP6 mutations had no effect. Strikingly, the LP4 mutations resulted in a significant increase in target repression by cel-let-7 from ∼40% repression by wild type to ∼80% repression. These results demonstrate that nucleotides beyond the mature miRNA region play critical roles in controlling the activity of miRNA genes in target repression.
Pri-let-7 loop controls the precision of mature miRNA biogenesis.
How do loop nucleotides control the activity of miRNA genes? Previous studies indicated that various cellular proteins, such as Lin-28, hnRNPA1, and KSRP control the production of miRNAs through interaction with pri- and/or pre-miRNAs.Citation11–Citation16 However, these proteins may not play a role in determining whether or not mature let-7 is made since truncated pre- and mature let-7 that lack the first two nucleotides at the 5′ end are produced from cel-let-7-wt.Citation9 Indeed, northern blot analyses indicated that loop mutations have varied effects on the biogenesis of pre- and mature let-7 (). Expression of LP2, LP3, LP5 or LP6 did not result in mature miRNA profiles that were significantly different from that of the cel-let-7-wt, whereas expression of the cel-let-7-LP4 mutant resulted in a significant increase in the 22-nt mature let-7 species.
Primer extension analyses confirmed that the LP4 mutant produced significant amounts of mature and pre-let-7. Notably, much of this RNA had the same 5′ end as the endogenous human let-7 ( and ). Thus, unlike mature let-7 miRNAs made from cel-let-7-wt which lack the first canonical seed (denoted “SD1” here) nucleotide, cel-let-7-LP4 mutations partially restore the biogenesis of full-length pre- and mature let-7 RNAs. Other loop mutants tested (LP2, LP3, LP5 and LP6), which have minor detrimental or no effects on cel-let-7 activity, produce only truncated pre- and mature let-7 as does the cellet-7-wt (data not shown). These results demonstrate that primiRNA loop nucleotides control the precision of pri-miRNA processing to pre- and mature forms, which determines the fidelity of miRNA seeds. Thus, although previous studies showed that the pri-miRNA loop is dispensable for primary miRNA processing in vitro,Citation14 it may play critical roles in controlling precision of seed generation and the activity of miRNA genes in vivo.
Both loop structure and sequence affect mature miRNA biogenesis.
To test whether the UU to AA nucleotide changes are sufficient to restore the generation of full-length mature let-7 in cel-let-7-LP4 (), we further mutated the cel-let-7-LP4A by altering the GG dinucleotide at the 5′ end of the loop of LP4 to CC (). We also generated the LP4B mutant, which maintained the GG to CC change in LP4A, but contained compensatory mutations to restore the loop structure of LP4 (). Similar loop mutations have strong effects on the repression activity of pri-let-7-wt when no functional mature let-7 is produced.Citation9 LP4A and LP4B mutations both resulted in significant reduction in pre- and mature let-7 RNAs as indicated by northern blot analyses (): The full length∼22-nt mature let-7 produced by these double-mutant constructs was at background level (). Primer extension analyses of gel-purified small RNA () and pre-miRNA () fractions showed that LP4A and LP4B only produced truncated pre- and mature let-7 lacking two nucleotides at the 5′ end (the 20-nt primer extension product); there was no detectable full-length pre- or mature let-7. These results demonstrate that the precision of pri-let-7 processing, which determines the production of mature miRNA with proper seed nucleotides, is controlled by both the nucleotide sequence and the secondary structure of the pri-let-7 loop.
Loop mutagenesis reveals pri- and mature miRNA functions in target repression.
The effects of the LP4 mutations on mature miRNA biogenesis provided a unique opportunity to examine how loop nucleotides control the activity of the cel-let-7 gene in the presence and absence of properly processed mature let-7 RNAs. Since we have previously shown that loop nucleotides can control the target repression activity of pri-miRNAs in the absence of functional mature miRNAs,Citation9 it is possible that LP4 mutations may alter pri-miRNA function and contribute to the increase of activity of cel-let-7-LP4 independent of biogenesis of full-length mature miRNAs. Alternatively, given that a significant increase in the production of full-length mature let-7 coincides with enhanced target repression in cel-let-7_LP4, it is also logical to infer that the generation of full-length mature let-7 directly contributes to the enhanced activity of the mutant gene. The ability to manipulate mature miRNA biogenesis through loop mutagenesis () enabled us to further dissect the causal relationships between mature miRNA biogenesis and the target repression ().
Intriguingly, expression of cel-let-7, cel-let-7-LP4A, and cel-let-7-LP4B repressed target reporter gene expression by 40% (), 18%, and 55% (), respectively. In all three cases, target repression occurred despite the fact that full-length mature let-7 was not produced from any of these genes ( and ). Moreover, the approximately 6.7-fold increase in full-length mature let-7 expression by the cel-let-7-LP4 construct compared to that from the wild-type cel-let-7 resulted in an additional 36% absolute increase in repression. In contrast, without causing significant change in mature miRNA levels, cel-let-7-LP4B mutant led to a 15% absolute increase in repression activity when compared to cel-let-7-wt ( and ). Clearly, the activity of pri-let-7 can be controlled by the loop nucleotides in the absence of functional mature let-7 miRNAs. Unless the LP4 mutations completely abolish the contribution of pri-let-7-LP4, these findings strongly suggest that both pri- and mature miRNAs produced from cel-let-7-LP4 contribute to the increase in cel-let-7-LP4 repression activity. However, additional analyses are needed to discern their contributions and functional relationships in target recognition and repression.
Control of target regulation by pri-miRNA and target interaction.
Since in the absence of functional mature miRNAs target repression activity correlates strongly with the formation of primiRNA and target complexes,Citation9 we examined the complex formation between target mRNAs and pri-let-7-LP4 in the presence of full-length, functional mature let-7 miRNAs. In brief, Renilla reporters with the wild-type lin-41_LCS UTR (lin-41_LCS-wt), seed mutant lin-41_LCS UTR (lin-41_LCS-sm), or no lin-41_ LCS UTR were tagged with the S1 aptamer, thus allowing selective enrichment of target/pri-miRNA complexes by streptavidin affinity purification.Citation9 Using this pull-down assay, we showed that both pri-let-7-wt and pri-let-7-LP4 specifically bound to the S1-tagged target mRNA with the wild-type target sites but not to either the S1-tagged reporter mRNA without a UTR or with the lin-41_LCS-sm UTR (). Relative levels of pri-let7-wt and pri-let-7-LP4 RNAs associated with the lin-41_LCS-wt UTR were 3- to 5-fold higher than those associated with either of the control target mRNAs. There were an average of two copies of pri-let-7-LP4 RNA bound to each lin-41_LCS-wt UTR reporter mRNA as calculated from standard curve qPCR analyses using in vitro transcribed forms of pri-let-7 and Renilla reporter RNAs as standards (); this is comparable to the number of pri-let-7-wt molecules bound to each target mRNA Citation9. Thus, these results demonstrate that pri-let-7-LP4 directly interacts with target reporter miRNA in vivo, demonstrating that pri-let-7-LP4 directly recognizes and represses the expression of target mRNA in the presence of full-length functional mature let-7.
Neither cel-let-7-LP4A nor cel-let-7-LP4B produced significant amounts of functional mature let-7 but both inhibited target expression (by 20% and 55%, respectively, and ). Interestingly, formation of the pri-miRNA and target complex was inefficient when cel-let-7LP4A was expressed, but highly efficient when cel-let-7-LP4B was expressed. The efficiency of pri-miRNA and target complex formation may explain why cel-let-7-LP4B only slightly reduced repression activity compared to cel-let-7-LP4 ( and ), again indicating that pri-miRNAs can control target recognition and repression in the absence of functional mature let-7. It is also important to note that cel-let-7-LP4B represses target expression more efficiently than cel-let-7-wt (55% vs. 40%, and , p<0.01). Since neither cel-let-7-LP4B nor cel-let-7-wt produces significant amounts of functional mature let-7 ( and ) and pri-miRNAs from these genes form complexes with target mRNAs at similar levels (), these results suggest that the pri-miRNA loop nucleotides may influence the activity of these miRNA genes by controlling processes other than mature miRNA biogenesis and steady-state levels of target and pri-miRNA complexes.
Intriguingly, under the conditions used in the pull down assay, the amount of mature let-7 RNA associated with the S1-tagged target mRNA was not significant (). There are several reasons why mature let-7 might not be efficiently pulled down by S1-tagged target mRNA. One possible explanation is that targets bound to functional mature let-7 are degraded and, therefore, cannot be enriched in the pull-down fraction. In support of this idea, we observed that cel-let-7-LP4 expression resulted in significant degradation of target mRNAs (). Since we have shown that prilet-7-wt does not lead to target degradation in the absence of functional mature let-7,Citation9 these results suggest a strong correlation between target degradation and the presence of full-length functional mature miRNAs. Thus, pri-let-7 and mature let-7 may have distinct effects on target stability, further supporting the hypothesis that both pri-let-7 and full-length mature let-7 of cel-let-7-LP4 contribute to target repression.
Differential sensitivity of target regulation to SD1 pairing.
When both pri-miRNA and full-length functional mature miRNA are present, it is difficult to quantitatively discern the contributions of the two species on gene repression. These two molecules could function either independently or synergistically. Interestingly, we noted that cel-let-7-LP4 activity was only partially inhibited by mutation of the SD1 pairing nucleotide in the target UTR, although activity of cel-let-7-wt was completely abolished by this mutation ( and ). The activities of cellet-7-LP4A and cel-let-7-LP4B, both of which failed to produce detectable amounts of full-length mature let-7, were also completely abolished by this mutation (). This observation is consistent with our previous observation that in the absence of functional mature let-7, the activity of pri-let-7 is completely abolished by mutation of the SD1 nucleotide.Citation9 Thus, unlike that of the cel-let-7-wt gene and mutants that do not produce full-length mature let-7 (cel-let-7-LP4A and cel-let-7-LP4B), a portion of the cel-let-7_LP4 activity is SD1-insensitive.
Since the appearance of full-length functional mature let-7 is accompanied by enhanced cel-let-7_LP4 activity, these findings raised the possibility that SD1-insensitive regulation might be mediated by the full-length mature let-7. In support of this hypothesis, we found that synthetic mature 22-nt mature let-7 duplexes (si-let-7) effectively repress reporters with the SD1 pairing nucleotide mutated, albeit at a slightly lower efficacy than repression of reporters with wild-type let-7 binding sites (). Thus, mutations disrupting SD1 pairing have minor effects on target repression by synthetic mature let-7. Further, we noted a significant decrease in reporter mRNA in cells expressing cel-let-7_LP4, indicating that this SD1-insensitive pathway may trigger degradation of the target transcript (). In contrast, no target degradation is observed upon expression of cel-let-7-wt, which produces no full-length mature miRNAs Citation9. These analyses support the idea that both pri-let-7 and mature let-7 made from cel-let-7_LP4 can contribute to target recognition and repression and their activities are differentiated by their differential sensitivity to the paring to the first seed nucleotide.
Discussion
The finding that pri-miRNAs function in target recognition raises many fundamental questions: Do pri-miRNAs act as functional species for some or all miRNA genes? How do pri-miRNAs repress target expression? Do pri-miRNAs use the same or different pathways as the mature miRNAs? Since mature miRNAs have been shown to function in target repression, what are the relationships between pri-miRNA and mature miRNA made from the same miRNA gene? Specifically, do pri-miRNA and mature miRNA species of a miRNA gene function independently, or in a mutually exclusive fashion, or in coordination? In this study we focused on resolving the relationship between pri- and mature let-7 and determining their functional contributions when both species are active. These studies revealed critical regulatory information used in target recognition and molecular steps required for target repression.
Given that miRNA genes generally produce mature miRNAs with correct 5′ ends, it is intrinsically difficult to discern their activities when both pri- and mature miRNA species are active. Our finding that the fidelity of mature miRNA 5′ end and seed nucleotides can be controlled by the pri-miRNA loop nucleotides provides the first evidence that pri-miRNA loops play critical roles in controlling the fidelity of mature miRNA biogenesis in vivo and also illustrates a novel approach to dissecting the causal relationship between mature miRNA biogenesis and target repression. Intriguingly, previous analyses mir-16-1 biogenesis in vitro suggested that the pri-miRNA loop is dispensable for pri-miRNA processing and mature miRNA biogenesis.Citation14 Clearly, our findings suggest that more thorough analyses may be needed to determine the role of loops in pri-miRNA processing and the fidelity of miRNA seed generation in vivo. Although certain protein factors have been shown to bind the pri-/pre-miRNA loops and control the production of mature miRNAs, none have been implicated in controlling the precision of mature miRNA 5′ end generation.Citation11–Citation13,Citation15,Citation16 Therefore, these findings suggest that there may exist a class of molecules that recognize pri-miRNA loops and control the fidelity of miRNA seed generation.
The ability to manipulate the processing and the activity of miRNA gene products enabled the dissection of the causal relationships between mature miRNA biogenesis and the target repression activities of pri- and mature miRNAs without causing major cellular defects. In contrast, given the broad function of Dicer and Drosha,Citation5-Citation7 it is not possible to rule out whether loss of Dicer or Drosha may result in unknown consequences that preclude the use of these cells in discerning the functional contribution by precursor miRNAs. Through manipulating mature miRNA biogenesis by loop mutagenesis we demonstrated that pri-miRNAs account for significant fraction of repression activity by the c-let-7-LP4 even in the presence of properly processed mature miRNAs. In particular, we showed that mutant c-let-7LP4B is more active than c-let-7-wt even though these mutations do not result in a significant increase in full-length mature let-7 (, , and ). In contrast, mutant c-let-7-LP4A, which also results in the loss of full-length mature let-7 ( and ), has little repression activity (). Thus, loop nucleotides can be modified to either increase or decrease the activity of pri-miRNAs in the absence of full-length functional mature let-7. We also observed a dramatic increase of repression activity with the c-let-7-LP4 mutation corresponding to a significant increase in full-length functional mature let-7 (). Intriguingly, whereas the repression activities of c-let-7-wt and c-let-7-LP4B were sensitive to the target paring to the first seed nucleotide, those of c-let-7-LP4 and synthetic full-length let-7 mimics was only partially sensitive (). This observation indicates that both pri-miRNA and full-length mature let-7 may contribute to the increased repression activity by the c-let-7-LP4 mutations. In support of this idea, we showed that pri-let-7-LP4 formed complexes with target mRNAs as efficiently as pri-let-7-wt and prilet-7-LP4B (). Using this system, it is still not possible to determine the fraction of activity contributed by the pri-miRNA and full-length mature let-7 when both are present. Nevertheless, these results strongly suggest that both and mature miRNAs have functional roles in target recognition and repression when mature miRNAs are properly processed.
Our results suggest that regulatory information encoded in pri-miRNAs may be translated into activities in target recognition and repression at two levels: (1) though direct interactions between pri-miRNAs and target mRNAs and (2) through control of pri-miRNA processing into mature miRNAs. These findings may explain some puzzling observations in target recognition and repression. For example, it is well known that many miRNAs are expressed at low levels, ranging from tens copies/cell to a few hundred copies/cells. Given that each miRNAs can regulate a few hundred targets, it is unclear how these low-abundance miRNAs regulate some but not all targets containing matching targets sites. The fact that both pri-miRNAs and full-length mature miRNAs can function in target repression raises the possibility that pri-miRNAs may function as the guiding molecules that enhance recognition of certain target mRNAs. Since we have shown that pri-miRNA and target RNA interactions occur within the nucleus during and after target mRNA transcription, these findings further suggest that nuclear events, such as target and pri-miRNA recognition, as well as co-transcriptional processing of pri-miRNAsCitation17 may constitute the early steps of target recognition by some miRNA gene products. Moreover, early engagement of targets by pri-miRNAs in the nucleusCitation9 may facilitate the coordination of mature miRNA biogenesis and guide mature miRNAs to the target sites that are recognized by primiRNAs but not those that are not recognized by pri-miRNAs. Such a mechanism may guide low-abundance mature miRNAs to their targets. However, whether pri-let-7-LP4 and mature let-7 function independently or in coordination when both are present remains unclear. In vivo kinetic models for gene regulation by miRNA genes may be required to resolve the relationships between the structure pri-miRNAs and linear mature miRNAs. Nevertheless, this model suggest that target repression may consist of sequential steps of interactions between targets and pri-, pre-, and mature miRNAs. Indeed, pre-miRNAs were found to bind to Ago2 and are active components of RISC.Citation18
Our findings may have broad implications for understanding the mechanisms of target recognition and repression by miRNA genes. From an evolutionary point of view, these results suggest that the activity of miRNA genes may be tuned and altered by the loop nucleotides. Intriguingly, many miRNA genes can be classified into large families, which produce largely identical mature miRNAs from precursor miRNAs with varied loop sequences. As an example, the let-7 gene expanded significantly during evolution from one gene in C. elegans to 11 genes in humans. These genes produce nearly identical mature miRNAs from precursor miRNAs with highly divergent loop sequences and structures Citation19. Often miRNAs of the same family are co-expressed in the same cell types. For example, as shown by miRNA deep sequencing analyses, the miR-181 family (miR-181a-d), let-7 family (let-7a-i), and many other miRNA families were found to have largely overlapping expression patterns in developing T and B lymphocytes.Citation20 Therefore, the role of divergent precursor miRNA loops is likely not limited only to the control of mature miRNA biogenesis. As illustrated by our loop mutagenesis analyses (–), precursor miRNA loop nucleotide sequences that diverged over the course of evolution might have varied activities in target repression. Finally, these findings also suggest that precursor miRNA loop nucleotide structure and sequences may be optimized to improve the suppressive activity of small interference RNAs based on primiRNA miRNA or short hairpin precursors.
The finding that pri-let-7 loop nucleotides control the precision of mature miRNA processing revealed unexpected levels of regulation that determine the fidelity of miRNA seed that in turn control target specificity and the activity of mature miRNAs. miRNA cloning and sequencing analyses have revealed polymorphisms at the 5′ end of mature miRNAs, albeit less prevalent than 3′ polymorphisms,Citation21 suggesting that loop nucleotides may control activities of miRNA gene products under physiological conditions. Identification of protein and/or RNA factors that control the precision of mature miRNA biogenesis at the primiRNA level will provide insights into the mechanisms that control primary and mature miRNA activity. Furthermore, the fact that structure and sequence elements of pri-miRNAs can control the activity of these structured RNAs suggests that the information encoded in these regulatory elements but absent in mature miRNAs may be incorporated into target analyses algorithms to further improve the accuracy of prediction. Our findings provide an exception to the canonical model that considers pri- and pre-miRNAs as transitory intermediates with no functional roles. Since interactions mediated by the structural motifs of RNA molecules represent a primordial mode of regulation by many RNAs,Citation22–Citation24 the possibility that pri-miRNAs of other miRNA genes may also have functions in target recognition and repression should be further tested.
Materials and Methods
See Trujillo et al.Citation9 and supplemental material for more detailed descriptions of vectors and luciferase reporter assay and northern blot and primer extension analyses.
Plasmid constructs.
cel-let-7 and its variants were cloned into a bi-cistronic vector that allows for co-expression of the miRNA gene with a GFP reporter.Citation9,Citation25 A 102-nt lin-41 3′ UTR containing the let-7 complementary sites (LCS) was cloned into the UTR of the Renilla luciferase reporter vector phRL-TK (Promega).
Luciferase reporter assay.
BOSC 23 cells were co-transfected with firefly luciferase control reporter plasmid, Renilla luciferase target reporter, and miRNA expression vector. Cells were harvested 48 hours post-transfection and assayed using the Dual-Luciferase system (Promega). Seed-dependent repression was determined by normalizing the miRNA-mediated repression of wild-type reporter to that of corresponding seed-mutant reporter.
Northern blot analyses.
Northern blot analyses were used to determine the levels of mature miRNA expression as well as levels of processing of pre- and mature miRNA. Quantitative northern blot analyses were carried out as described previously.Citation8,Citation9 Total RNA was prepared from BOSC 23 cells transfected with equal masses of miRNA expression vector. Data was normalized by FACS analysis of GFP transfection marker signal, reprobing of blots for U6 RNA, or ethidium bromide gel staining. Serial dilutions of synthetic mature miRNA were resolved alongside total RNA samples to generate standard curves. Band intensity was determined by phospho-imaging and copies/pg total RNA were determined by comparison to standard curves. Representative blots and averaged data from three or more repetitions from cells transfected with independent DNA preparations are shown.
Primer extension analyses.
Pre- and mature miRNA fractions were obtained by fractionating total RNA samples (100 µg) from cells transfected with various miRNA expression vectors by 15% PAGE. Synthetic pre-miR-181a-1 and mature miR-181a were spiked into total RNA samples before gel fractionation. Primer extension analyses were then carried out map the 5′ ends of pre- and mature miRNAs.. LNA primers were used to prime pre-let-7 and pre-miR-181a-1. Synthetic oligonucleotides with the let-7 sequence in single nucleotide increments (16 to 22 nt) labeled with 32P were used as size ladders. The products of reactions with LNA primers migrate at a rate ∼1 nt longer than corresponding DNA primer reactions. Quantitative analyses were performed by normalizing the intensities of pre- or mature let-7 products to that of pre- or mature miR-181 internal controls, respectively.
Quantitative PCR analyses (qPCR).
Cells transfected with target reporter and designated miRNA expression constructs were collected, spiked with 22.5 fmol synthetic miR-223, and lysed with Trizol reagent (Invitrogen) for total RNA preparation. miRNA qPCR analyses were carried out for let-7, miR-223, or target mRNAs. Numbers of copies of test and spiked miRNAs in a defined amount of total RNA were determined by using standard curves for let-7 and spiked miR-223.
Figures and Tables
Figure 1 Identification of pri/pre-miRNA loop mutations that potentiate cel-let-7 activity and partially rescue defective mature let-7 biogenesis of cel-let-7 miRNAs in human cells. (A) Schematic diagrams depict the pre-miRNA sequences and structures of the wild-type cel-let-7 gene and loop mutants. (B) Seed-dependent repression of target reporter by the cel-let-7 and loop mutants. Average results of at least six independent trials ± S.D. are shown. Statistical significance was determined by an unpaired two-tailed Student's t test. (C) Expression of pre- and mature miRNA made from wild-type and loop mutant cel-let-7 genes was determined by quantitative northern blot. Representative results of four northern blot analyses from independent transfections are shown. Blots were also probed for U6 small nuclear RNA as a loading control. (D & E) LP4 mutations in cel-let-7 result in increases in pre- (n=2) and mature let-7 (n=3 to 6) with correct 5′ ends. The 5′ ends of (D) mature let-7 and (E) pre-let-7 made from the wild-type cel-let-7 and cel-let-7_LP4 genes were determined by primer extension analyses.
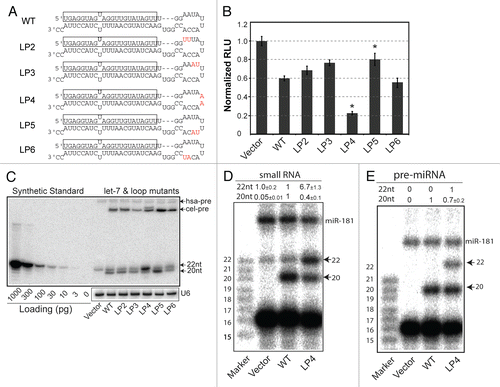
Figure 2 Loop mutations that inhibit biogenesis of full-length mature let-7. (A) Schematic diagrams depicting the pre-miRNA sequences and structures of cel-let-7_LP4 and loop mutants. (B) A northern blot analysis representative of three experiments shows pre-miRNA and mature miRNA expression from cel-let-7-LP4 and loop mutants. (C) The 5′ ends of mature let-7 miRNAs made from the type cel-let-7 and pri-let-7 loop mutants were determined by primer extension analyses (n=6). (D) The 5′ ends of pre-let-7 miRNAs made from the type cel-let-7 and pri-let-7 loop mutants were determined by primer extension analyses (n=2).
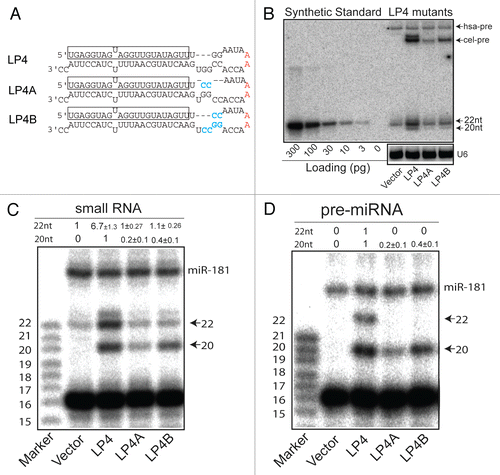
Figure 3 The activities of cel-let-7_LP4 and loop mutants in target repression correlate with the formation of specific pri-let-7 RNAs and target complexes. (A) Repression of luciferase reporter expression by cel-let-7_LP4 and loop mutants. Representative results of at least six independent trials (± S.D.) are shown (*, p<0.0001 except as indicated). (B) Formation of pri-let-7 RNAs and target complexes predicts the activity of cel-let-7 and loop mutants in target repression. Lin-41_LCS reporter mRNAs were tagged with S1 aptamers and used to pull-down associated pri-let-7 RNA. S1-tagged reporter mRNAs with the Lin41-LCS_sm UTR or without the Lin41-LCS UTR were used as negative controls. The ratios of wild-type to mutant pri-let-7 RNA and corresponding S1-tagged target RNAs with or without the wild-type lin-41-LCS UTR or with a seed mutant lin-41-LCS UTR in the pull-down RNA samples were determined by using qPCR analyses (n=4, ±S.D., *, p< 0.001). (C & D) Standard curve-based qPCR was carried out to determine the average copy numbers of (C) pri-let-7 and (D) mature let-7 per target in the pull-down samples (n=4, ±S.D.). (E) The effects of cel-let-7-wt and cel-let-7-LP4 expression on the levels of target mRNA were determined by qPCR analyses (n=4, ±S.D., *, p< 0.001).
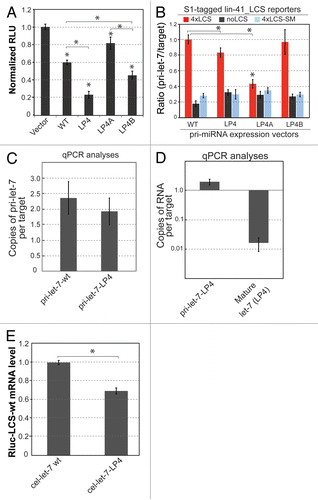
Figure 4 Repression activity by cel-let-7 and loop mutants may be controlled by both SD1-sensitive and SD1-insensitive components. (A) Schematic diagrams showing the predicted base parings between let-7 and modified lin-41_LCS. Altered nucleotides are indicated in blue, missing as underlined. (B & C) Repression of mutant lin-41_LCS reporters that abolish SD1 pairing by (B) wild-type cel-let-7 and cel-let-7-LP4 and (C) double loop mutant cel-let-7-LP4 genes. (D) siRNA duplexes of 20 base pairs (truncated let-7) and 22 base pairs (full-length mature let-7) were transfected into cells expressing mutant lin-41_LCS reporters that abolish SD1 pairing and reporter activity was measured. Representative results of at least six independent trials (± S.D.) are shown in panels B, C, and D (*, p< 0.01).
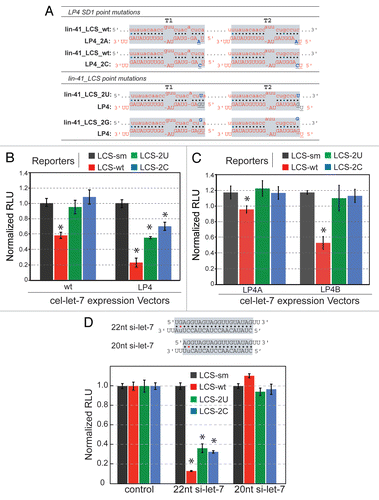
Additional material
Download Zip (338.7 KB)Acknowledgements
We thank the members of Chen lab for the comments and suggestions.
Funding
This work was supported by grants from National Institute of Health (HL081612 and 1DP1 OD00643501) and the W. M. Keck foundation to C.-Z. C.
References
- Kim VN. MicroRNA biogenesis: coordinated cropping and dicing. Nat Rev Mol Cell Biol 2005; 6:376 - 385
- Ghildiyal M, Zamore PD. Small silencing RNAs: an expanding universe. Nat Rev Genet 2009; 10:94 - 108
- Lee RC, Feinbaum RL, Ambros V. The C. elegans heterochronic gene lin-4 encodes small RNAs with antisense complementarity to lin-14. Cell 1993; 75:843 - 854
- Doench JG, Peterson CP, Sharp PA. siRNAs can function as miRNAs. Genes Dev 2003; 17:438 - 442
- Nakagawa A, Shi Y, Kage-Nakadai E, Mitani S, Xue D. Caspase-dependent conversion of Dicer ribonuclease into a death-promoting deoxyribonuclease. Science 2010; 328:327 - 334
- Han J, Pedersen JS, Kwon SC, Belair CD, Kim YK, Yeom KH, et al. Posttranscriptional crossregulation between Drosha and DGCR8. Cell 2009; 136:75 - 84
- Kadener S, Rodriguez J, Abruzzi KC, Khodor YL, Sugino K, Marr MT 2nd, et al. Genome-wide identification of targets of the drosha-pasha/DGCR8 complex. RNA 2009; 15:537 - 545
- Liu G, Min H, Yue S, Chen CZ. Pre-miRNA loop nucleotides control the distinct activities of mir-181a-1 and mir-181c in early T cell development. PLoS ONE 2008; 3:e3592
- Trujillo RD, Yue SB, Tang Y, O'Gorman WE, Chen CZ. The potential functions of primary microRNAs in target recognition and repression. EMBO J 2010; 29:3272 - 3285
- Vella MC, Reinert K, Slack FJ. Architecture of a validated microRNA::target interaction. Chem Biol 2004; 11:1619 - 1623
- Newman MA, Thomson JM, Hammond SM. Lin-28 interaction with the Let-7 precursor loop mediates regulated microRNA processing. RNA 2008; 14:1539 - 1549
- Heo I, Joo C, Cho J, Ha M, Han J, Kim VN. Lin28 mediates the terminal uridylation of let-7 precursor MicroRNA. Mol Cell 2008; 32:276 - 284
- Viswanathan SR, Daley GQ, Gregory RI. Selective blockade of microRNA processing by Lin28. Science 2008; 320:97 - 100
- Han J, Lee Y, Yeom KH, Nam JW, Heo I, Rhee JK, et al. Molecular basis for the recognition of primary microRNAs by the Drosha-DGCR8 complex. Cell 2006; 125:887 - 901
- Guil S, Caceres JF. The multifunctional RNA-binding protein hnRNP A1 is required for processing of miR18a. Nat Struct Mol Biol 2007; 14:591 - 596
- Trabucchi M, Briata P, Garcia-Mayoral M, Haase AD, Filipowicz W, Ramos A, et al. The RNA-binding protein KSRP promotes the biogenesis of a subset of microRNAs. Nature 2009; 459:1010 - 1014
- Pawlicki JM, Steitz JA. Primary microRNA transcript retention at sites of transcription leads to enhanced microRNA production. J Cell Biol 2008; 182:61 - 76
- Tan GS, Garchow BG, Liu X, Yeung J, Morris JPt, Cuellar TL, et al. Expanded RNA-binding activities of mammalian Argonaute 2. Nucleic Acids Res 2009; 37:7533 - 7545
- Griffiths-Jones S. The microRNA Registry. Nucleic Acids Res 2004; 32:Database issue D109 - D111
- Kuchen S, Resch W, Yamane A, Kuo N, Li Z, Chakraborty T, et al. Regulation of microRNA expression and abundance during lymphopoiesis. Immunity 2010; 32:828 - 839
- Ruby JG, Jan C, Player C, Axtell MJ, Lee W, Nusbaum C, et al. Large-scale sequencing reveals 21U-RNAs and additional microRNAs and endogenous siRNAs in C. elegans. Cell 2006; 127:1193 - 1207
- Eisinger J, Gross N. The anticodon-anticodon complex. J Mol Biol 1974; 88:165 - 174
- Eguchi Y, Itoh T, Tomizawa J. Antisense RNA. Annu Rev Biochem 1991; 60:631 - 652
- Paillart JC, Marquet R, Skripkin E, Ehresmann B, Ehresmann C. Mutational analysis of the bipartite dimer linkage structure of human immunodeficiency virus type 1 genomic RNA. J Biol Chem 1994; 269:27486 - 27493
- Mao TK, Chen CZ. Dissecting microRNA-mediated gene regulation and function in T-cell development. Methods Enzymol 2007; 427:171 - 189