Abstract
Plants possess a single subfamily of Rho GTPases, ROP, which does usual things as do Rho-family GTPases in animal and fungal systems, namely participating in the spatial control of cellular processes by signaling to the cytoskeleton and vesicular trafficking. As one would expect, ROPs are modulated by conserved regulators such as DHR2-type GEFs, RhoGAPs, and Rho GDIs. What is surprising is that plants have invented new regulators such as PRONE-type GEFs (known as RopGEFs) and effectors such as RICs and ICRs/RIPs in the regulation of the cytoskeleton and vesicular trafficking. This review will discuss recent work on characterizing ROP regulators and effectors as well as addressing why and how a mixture of conserved and novel Rho signaling mechanisms is utilized to modulate fundamental cellular processes such as cytoskeletal dynamics/reorganization and vesicular trafficking.
Introduction
Rho-family small GTPases are highly conserved molecular switches that typically function as “hubs” in signaling networks that control fundamental cellular processes depending on cytoskeletal reorganization/dynamics and/or polarized vesicular trafficking in eukaryotes, such as cell polarity generation, cell morphogenesis, cell movement, etc. In spite of the overall conserved cellular function, members of the Rho family have evolved to diverge to Rho, CDC42 and Rac subfamilies in animals and filamentous fungi and CDC42 and Rho in yeasts. Interestingly, plants possess ROP (Rho-like GTPases from plants) as a sole counterpart of the Rho family. ROPs sometimes are also referred to as RACs, because they share highest identity at the amino acid sequence level.Citation1–Citation3 However, phylogenetic analysis suggests that all ROPs evolved from the common ancestor of Rho, CDC42 and Rac proteins (). For this purpose, plants Rho-family small GTPases are referred to as ROPs in this review.
Since plants lack the Ras family of small GTPases, which participates in transmission of extracellular signals along with Rho GTPases in yeast and animals, ROPs are the sole signaling small GTPases in plants. Thus, it is expected that ROPs participate in wide variety of signaling events in plants. As their counterparts in yeast and animals, ROPs participate in signaling pathways that regulate cytoskeletal organization/dynamics and vesicular trafficking, consequently impacting cell polarization, polar growth and cell morphogenesis in plants.Citation4–Citation18 Moreover, much of our knowledge of ROP GTPase signaling can be linked to the study of the mechanisms underlying tip growth in pollen tubes and cell morphogenesis in leaf epidermal pavement cells ().Citation19–Citation24 Another area of intensive study concerning ROP GTPases is plant defense responses.Citation2,Citation25–Citation36 ROPs have also been reported to regulate gene expression involved in hormone responses and responses to abiotic stresses.Citation37–Citation41 Finally ROP signaling has been implicated in light-mediated guard cell movement.Citation42 Given the functional conservation for plant Rho GTPases, it is surprising to discover plant-specific downstream effectors that plant use to modulate these fundamental cellular processes. Plants do retain conserved regulators such as DHR2-type guanine nucleotide exchange factors (GEFs), conventional Rho GTPase-activiting proteins (RhoGAPs) and guanine nucleotide dissociation inhibitors (GDIs). Yet plants also invented their own regulators such as a novel family of GEFs termed RopGEFs. The comparison and contrast of ROP GTPase effectors () and regulators with the counterparts from fungi and animals, and the implication of these new functional partners in the capability of ROP GTPases to orchestrate fundamental cellular processes in the plant cell environment will be discussed.
ROP Effector Proteins
A wide variety of Rho GTPase effector proteins is known in fungi and animals, ranging from protein kinases (e.g., PAKs) through cytoskeleton associated proteins (formins) to scaffold proteins like WAVE/SCAR.Citation43,Citation44 The majority of those effector proteins from other systems are missing in plants, and in those cases where homologs of animal or fungal Rho effectors are present in plants, e.g., formins, no evidence is available to support their function as direct effectors of ROPs. Instead, several families of plant-specific effector proteins for ROPs have been identified.
CRIB motif containing proteins.
The first example of ROP effector proteins is a class of plant-specific proteins containing a CRIB motif, named as ROP INTERACTIVE CRIB MOTIF-CONTAINING PROTEINS (RICs).Citation45 The CRIB (Cdc42/Rac-interactive binding) motif is commonly found in Cdc42/Rac effector proteins such as WASPs and p21PAK, and is responsible for their interactions with the GTP-bound form of Cdc42 and Rac GTPases. As in CRIB-containing Cdc42 and Rac effectors, the CRIB motif allows RICs to interact with activated ROPs. Although there are several residues that show variation among RICs, residues within CRIB domain are highly conserved as shown in . Although RICs are highly variable, residues within CRIB motifs are highly conserved as shown in . Another family of plant ROP-interacting proteins containing the CRIB motif is the RopGAP proteins.Citation46 Interestingly these CRIB motifs found in ROP effectors and regulators, respectively, were probably originated from the same plant ancestor, because all plant CRIB motifs are show higher similarities with each other than with CRIB motifs in proteins from other kingdoms. Outside of the CRIB motif, which is localized in the central region of RICs, those proteins do not have any similarity to known proteins. The Arabidopsis genome encodes 11 RICs that are highly divergent, implying that they could have evolved to modulate a wide range of downstream signaling pathways. Subsequent functional analysis of RICs in Arabidopsis and other plants demonstrated that different RICs indeed exhibit distinct functions.Citation11,Citation13,Citation45,Citation47 Here we focus on three RICs that have been extensively characterized and are all linked to the regulation of cytoskeletal organization and dynamics.
Regulation of Actin Dynamics by Counteractive RIC4 and RIC3: Roles in Tip Growth
The tip-growing pollen tube turns out to be an excellent system for investigating the function and regulation of ROP-dependent actin dynamics (). Three closely related members of Arabidopsis ROP (ROP1, ROP3, ROP5) are expressed in pollen, and are functionally redundant in the regulation of tip growth in pollen tubes.Citation48 Using a live F-actin marker, a highly dynamic form of F-actin localized to the apex of pollen tubes was observed and was shown to be regulated by ROP1 localized to the apical plasma membrane.Citation5 The ROP-dependent dynamics of the apical F-actin is implicated in the modulation of tip-targeted exocytosis required for pollen tube tip growth.Citation14
Actin dynamics involves the assembly and the subsequent disassembly of F-actin, which are traditionally thought to be activated by two independent signaling pathways, respectively. The dilemma is how could a single tip-localized ROP GTPase modulate the dynamics of F-actin at the tip of pollen tubes. The investigation of RICs reveals a mechanism by which a single ROP controls the tip actin dynamics. When overexpressed (OX), two structurally unrelated RICs, RIC3 and RIC4, both induced depolarized growth in tobacco pollen tubes associated with distinct changes in the dynamic tip F-actin; RIC3 OX caused disappearance of this actin, whereas RIC4 OX led to its stabilization.Citation13,Citation45 Their co-overxpression recovered normal polarized tip growth and the dynamics of the tip F-actin. These observations prompted Gu and his colleagues to test the hypothesis that these two ROP effectors act together coordinately to control actin dynamics.Citation13 Additional experimentation by Gu et al. show that whether RIC4 promotes the assembly of F-actin by acting as a ROP1 effector in pollen tube tips. It was further found that RIC4 acts as an effector of ROP2 in promoting the formation of diffuse cortical F-actin also in the lobe of jigsaw-puzzle-shaped leaf epidermal pavement cells.Citation11 Thus RIC4 appears to have a conserved function in the promotion of actin assembly in different cell types, but the mode of action for RIC4 regulation of actin assembly remains mysterious. Interestingly RIC4-dependent cortical F-actin is different from ARP2/3-dependent F-actin, and RIC4 might regulate a new type of actin nucleation.Citation11
Gu et al. found that overexpression of RIC3 in pollen tubes induced changes in the actin cytoskeleton similar to those induced by high levels of cytosolic Ca2+. It turned out that RIC3 promotes the level of cytosolic Ca2+ at the tip of the tubes. Moreover they found that RIC3-dependent cytosolic Ca2+ activates the disassembly of the apical F-actin in pollen tubes. Furthermore, they showed that RIC3 also acts as an ROP1 effector in its action to regulate cytosolic Ca2+ levels and subsequently actin disassembly. Therefore, both acting downstream of ROP1, the RIC3 and RIC4 pathways counteract coordinately to control actin dynamics at the tip of pollen tubes.Citation13
Being the first example of two counteracting pathways acting downstream of a single Rho GTPase to modulate actin dynamics, this finding raises some interesting questions. First, could this be a common mechanism for the regulation of actin dynamics in different systems? Although studies directly showing similar mode of action are yet to be obtained, several observations hint its generality in other systems accompanying tip growth such as root hair tip growth in plants, neurogenetic processes in animals and fungal hyphal growth.Citation13 In those processes, Rho GTPases as well as F-actin dynamics and calcium gradient also play an essential role. As there are examples of promotion of both entry and release of calcium into and out of animal cells by Rho GTPases,Citation49,Citation50 coordination of polymerization and calcium-mediated depolymerization of F-actin may be achieved by single upstream Rho GTPase as in pollen tubes. Second, what is the significance of this mechanism in the regulation of polarized tip growth? A recent study generated some insights into the mechanism by which ROP1-dependent F-actin dynamics participates in the tip growth of pollen tubes.Citation14 By using FRAP method with an RLK-GFP as a tracer, Lee et al. found that both the RIC4-dependent actin assembly and RIC3-dependent disassembly promotes transport and docking/fusion of exocytic vesicles to the apical PM, respectively, spatiotemporally coordinating targeted exocytosis for tip growth.Citation14 Furthermore, these two pathways are counteractive in influencing the targeting and docking/fusion of exocytic vesicles, consistent with the observation that RIC3-mediated calcium accumulation and actin disassembly lag behind RIC4-mediated actin assembly. This temporal coordination of the two counteractive pathways can also explain F-actin's and calcium's participation of positive and negative feedback regulations of the apical ROP1 activity, respectively.Citation51
RIC1 Regulation of Microtubule Organization
RIC1 has been shown to regulate microtubule organization important for the morphogenesis of pavement cells with interdigitated lobes and indentationsCitation11,Citation12 (). This process also requires two coordinated counteractive pathways: The ROP2-RIC4 pathway localizes to lobe tips and activates the accumulation of F-actin there and the ROP6-RIC1 pathway that organize cortical microtubules (MTs) at the indenting regions.Citation11,Citation12 RIC1 is MT associated protein that promotes parallel arrangements of MTs, as overexpression of RIC1 caused dense parallel cortical MTs and consequently inhibition of lobe formation, whereas loss of function of RIC1 resulted in randomized cortical MTs.Citation11 RIC1's association with MTs and function in MT organization is dependent on the activation of ROP6, which directly binds and activates RIC1. In the absence of ROP6, RIC1 is dissociated from MTs.Citation12 How ROP6 activation induces RIC1 association and how RIC1 promotes MT organization once it is associated with MTs are interesting questions yet to be addressed.
ICR/RIP Family Members Act as Scaffold Protein Linking ROPs to Exocyst
Another family of ROP effector proteins in Arabidopsis, represented by Interactor of Constitutively Active ROP 1 (ICR1)/ROP-interactive Partner 1 (RIP1), was found by two independent studies involving yeast 2 hybrid screen for proteins interacting with an activated form of ROPs.Citation15,Citation52 The ICR/RIP family, composed of 5 members in Arabidopsis, is conserved in the plant kingdom, but does not exist in other eukaryotes. Moreover, unlike RICs ICRs/RIPs do not contain any motifs known to interact with other Rho GTPases. However, motif invariable among ICR/RIP members near the c-terminus of those proteins was shown to be necessary for binding to active ROPCitation15 (). This plant-specific ROP-interactive motif suggests that plants have invented a completely new ICR/RIP family of Rho effectors. This is particularly interesting given the evidence that ICR1/RIP1 acts as a scaffold protein linking ROPs to exocyst, an exocytic vesicle-tethering complex highly conserved in eukaryotes.
Lavy et al. found that ICR1/RIP1 binds AtSEC3A, a subunit of the exocyst complex.Citation15,Citation53 In yeast, CDC42 directly interacts with SEC3 to recruit the exocyst complex to the site of CDC42 activationCitation54 (), and thus yeast SEC3 contains a Rho GTPase binding motif. However, in plants and animals, SEC3 homologs lack the Rho interaction domain. In mammalian cells, CDC42/RhoA failed to bind SEC3 homolog, while the CDC42/RhoA effector protein IQGAP1 was shown to interact with Sec3/Sec8.Citation55 Therefore, it appears that plants and mammals have evolved parallel mechanisms by adopting scaffolding proteins to link Rho-family GTPases to the exocyst.
Lavy et al. also found that T-DNA insertional mutant of ICR1 (icr1) show defects in plant morphogenesis. In the mutant, pavement cells became cubical, suggesting that these cells fail to expand, which is consistent with the essential role for the exocyst in cell expansion in plants.Citation56,Citation57 Interestingly, ICR1 is implicated in polar transport of auxin by regulating polar localization of PIN proteins that export auxin out of cells. PIN polarization is required for the establishment of auxin gradients that regulate various plant developmental processes and pattern formation.Citation58,Citation59–Citation63 Root meristem in icr1 mutant collapses, a phenotype resembling root patterning defect induced by a block in polar auxin transport.Citation15 Furthermore, ROPs contribute to polar PIN targetingCitation64 as well as vesicle trafficking that is essential for polar PIN targeting.Citation65 Indeed, recent work by the same group showed that ICR1/RIP1 contributes to the regulation of polar localization of PIN auxin efflux transporters to produce sites of auxin maxima in roots and embryos.Citation17 Polarization of PINs involves both localized inhibition of PIN endocytosis and localized recycling.Citation66 Interestingly ICR1 is polarly localized to the site of PIN and appears to be required for endocytic PIN recycling to the same site.Citation17 This is consistent with the notion that ICR1 is involved in tethering of endocytic recycling vesicles to the ICR1 site. PIN protein expression is induced by auxin, suggesting that ICR1/RIP1 pathway may contribute to a positive feedback loop consisting of auxin and accumulation of PIN proteins at the sites of high auxin in roots. Evidence also suggests auxin inhibits PIN endocytosis to form feedback regulation of PIN at the sites of auxin maxima in roots.Citation67,Citation68 Thus, it seems that there are at least two pathways regulating PIN trafficking to produce the polar distribution of PIN proteins: one that inhibits their endocytosis and the other that promotes their recycling mediated by ICR1/RIP1. Interestingly a recent study suggests that extracellular auxin activates lobe-localized ROP2, which in turn is required for PIN1 polarization to the lobes in pavement cells, suggesting that auxin activation of ROP2 and PIN1 polarization form a positive feedback loop.Citation69 Therefore it is reasonable to speculate that auxin activation of ROP2 promotes PIN1 polarization via coordination of two downstream pathways: an unknown pathway that inhibits PIN1 endocytosis and the ICR1/RIP1 pathway that promotes its recycling (). Clearly future work should test this possibility and determine whether this could be a common mechanism for the polarization of PIN proteins in various cell types.
The work by Li et al. also supports the notion that ICR1/RIP1 is involved in the exocytic process. They showed that ICR1/RIP1 localizes to the cell cortex of future germination sites and to the apical cortex of growing pollen tubes where exocytic activity in an active ROP1-dependent manner.Citation52 Furthermore, overexpression of ICR1/RIP1 cause depolarization of pollen tubes, and enhance accumulation of GFP-ROP1 to the PM. Thus, ROP1 and ICR1/RIP1 could form another positive feedback loop to maintain high ROP activity at the tip of growing pollen tubes. Since the exocyst complex is required for tip growth in pollen tubes,Citation56 ICR1/RIP1 may also act as scaffold protein to recruit exocyst to the apical PM where ROP1 is activated.
Other ROP Effector Proteins
Recently, a family of receptor-like cytoplasmic kinases and a cysteine-rich receptor kinase are identified as ROP interactors.Citation70,Citation71 Also, several proteins were identified as ROP effector proteins from studies of ROP proteins from Rice, named OsRAC1. OsRAC1 is involved in defense signaling in rice through multiple pathways including the regulation of NADPH oxidase dependent ROS production and the regulation of MAP kinase signaling.Citation2,Citation18,Citation34,Citation72
Of these, respiratory burst oxidase homolog (Rboh), catalytic subunit of NADPH oxidase involved in ROS production, is particularly interesting. Rboh proteins were shown to bind OsRAC1 directly.Citation32 In mammalian cells, NADPH oxidase exists as the multi-protein complex including Rac. Activated Rac activates the NADPH oxidase complex by translocating p67 subunit to the complex.Citation73 However, plant lacks subunits other than homologs to the catalytic subunit protein (Rboh) and OsRac1. Rboh possess N-terminal extension containing the EF-hand motifs that is absent from animal homologs. Wong et al. tested bindings between Rboh and OsRac1, and showed that OsRac1 binds Rboh at the N-terminal region in a GTP dependent manner. Moreover, they found that increase in cytosolic Ca2+ inhibits interaction between Rboh and OsRac1, and the EF-hand motif may contribute to the suppression mediated by Ca2+.
ROP Regulators: Tuning in Spatial Signals to ROPs
A key hallmark of Rho GTPase signaling is its spatial control of cellular processes. In this capacity, Rho GTPases are typically activated in local domains of the plasma membrane, such that they are capable of regulating the polarization of the cytoskeleton and of vesicular trafficking as discussed above for ROP effectors. Given the conservation of this spatial control in all eukaryotic cells, it is not surprising that some Rho regulators such as RhoGAPs (Rho GTPase activating proteins) and RhoGDIs (Rho guanine nucleotide dissociation inhibitors) are conserved for the spatial regulation of ROPs in plants. Investigation of these regulators in plant cellular systems may reveal paradigms for the spatial control of Rho GTPases (e.g., see REN1 below). Since types of cues that determine spatial activation of ROPs are likely plant cell-specific, one might expect that unique ROP activators could have evolved for the perception and transmission of these cues. Indeed as discussed below a plant-specific class of RhoGEFs (Rho guanine nucleotide exchange factors).
In addition to ROP regulators, posttranslational lipid modification of ROP proteins that affect their membrane attachment has also been implicated in the regulation of ROP activity. ROPs are categorized to 2 groups according to the type of lipid modifications on hyper-variable C-terminal regions. Type I ROPs are thought to be prenylated primarily by geranylgeranyltransferase, while type II ROPs are subject to S-acylation.Citation74–Citation76 A recent exciting study suggests that activated ROPs undergo S-acetylation at G-domain, which appears to be important for their association with lipid rafts.Citation77,Citation78 In-depth analysis of lipid modification of ROPs can be found in recent reviews.Citation78–Citation80
RhoGEFs
Animal and fungal RhoGEFs fall into two types: one containing a DBL homology (DH) domain and plestrin homology (PH) domain, the other containing Dock homology region (DHR) region.Citation81 In mammals, each type of RhoGEFs is encoded by a large gene family. However plants lack DH-type RhoGEFs, which are often activated by receptor tyrosine kinase (RTK) or G protein coupled receptor (GPCR) signaling in mammalian systems.Citation82–Citation85 This is consistent with the fact that RTK is missing in plants and that a single Gα in Arabidopsis only has a minimal effect on plant growth and development.Citation86 Furthermore, only a single DHR-type RhoGEF, named SPIKE1 (SPK1) is found in Arabidopisis.Citation87 Knocking out SPK1 in Arabidopsis caused adult lethality and defects in the morphogenesis of leaf epidermal cells.Citation87 Some of these changes such as the loss of jigsaw puzzle piece shape in pavement cells are similar to those induced by downregulation of ROPs.Citation11,Citation87,Citation88 Recent work showed SPIKE1 could bind to ROPs in a DHR2-dependent manner and act as a RhoGEF.Citation89 SPIKE1 may have a function not observed in animals, as it seems to act in the WAVE and ARP2/3 complexes to control the actin cytoskeleton.Citation89 SPK1 does not affect other ROP-dependent processes such as tip growth in pollen tubes and root hairs, suggesting the existence of other types of RhoGEFs in plants.
Indeed a novel and plant-specific RhoGEF family, termed RopGEF, was identified.Citation19,Citation90 The RopGEF family contains 14 members in Arabidopsis which share a conserved PRONE (plant-specific Rop nucleotide exchanger) domain with all RopGEFs from other plant species that functions as the GEF catalytic domain.Citation19,Citation90 Although they do not share amino acid sequence similarity with animal and fungal RhoGEFs, the crystal structure analysis shows that their 3-D structure resembles other RhoGEFs, and they function as a dimer like other RhoGEFs.Citation91 In vitro experiments suggest that the N- and C-termini of RhoGEF1 contains structural domains that interact with and inhibit the central PRONE domain, a regulatory feature that appears to be common among various RhoGEFs.Citation19
The cellular function of the RhoGEF family is largely uncharacterized. Overexpression of RopGEF1 or RopGEF12 induced depolarization of pollen tube growth, similar to that induced by ROP1 overexpression, suggesting that these RopGEFs may act as an activator of ROP1 in controlling polar growth in pollen tubes.Citation19,Citation22 Growth depolarization is similarly induced by the overexpression of the full-length RopGEF1 and the PRONE domain, from which the autoinhibitory domains were removed, implying the existence of cellular mechanism for releasing the autoinhibition.Citation19 A possible mechanism for removing C-terminal autoinhibiton was also suggested for RopGEF12.Citation22 Interestingly, a tomato RopGEF homolog was independently identified from a yeast two-hybrid screen for proteins that interact with the kinase domain of pollen-expressed receptor-like protein kinases, LePRK1 and LePRK2.Citation22,Citation92,Citation93 This led to testing a physical and functional interaction between the pollen-specific Arabidopsis RopGEF12 and PRK2a, a homolog of LePRK2.Citation22 Indeed the C-terminal region of RopGEF12 was shown to interact with the kinase domain of the Arabidopsis PRK2a. A series of overexpression experiments suggest that this interaction recruits RopGEF12 to the plasma membrane and releases the inhibition of the C-terminal region on the GEF activity of the PRONE domain.Citation22 A subclass of RopGEFs, all of which are expressed in pollen, contains conserved phosphorylation site at the C-terminal region, and S510D mutation has an impact on the function of RopGEF12 in pollen tubes.Citation22 Thus PRK2a may regulate RopGEF12 via PRK2a-mediated phosphorylation of the C-terminal region.
These observations suggest that plant-specific RopGEFs might have evolved to transmit plant-specific signals that are perceived by the RLK superfamily (>400 members in Arabidopsis), which is the predominant cell-surface receptor ser/thr kinases in plants, given lack of RTKs and scarcity of GPCRs (if any) in plants. Thus it is anticipated that RLKs could be a primary mechanism by which extracellular signals activate ROPs in plants. Another RLK candidate for ROP activation has been reported in maize.Citation94 The PAN1 RLK is required for the accumulation of a membrane-associated phosphoprotein, and also the accumulation of actin patches that is essential to polarize subsidiary mother cell (SMC) for asymmetric cell division toward guard mother cell (GMC).Citation94 A recent study suggests that exracellular auxin activates both ROP2 and ROP6, respectively localized to the complementary lobing and indenting regions (see ).Citation69 Auxin activation of these two ROP signaling pathways requires auxin-binding protein 1 (ABP1), which apparently acts in the cell surface and does not contain a transmembrane domain. Presumably a cell surface protein such as an RLK could act as with ABP1 as auxin coreceptor to transmit extracellular auxin signals to ROPs. ROP GTPase signaling in this system has been suggested to coordinate cell polarization and cell morphogenesis between adjacent cells.Citation69 Having a RLK cell surface receptor to perceive a ROP activating signal from adjacent cells could be a common mechanism for cell-cell coordination for the spatial control of cellular processes in multicellular plant tissues consisting of walled cells. Therefore we predict that identification of RLKs that regulate RopGEFs will be a fertile field for future studies of ROP signaling in connection to developmental mechanisms in plants.
RhoGAP and RhoGDI are Critical for Spatial and Temporal Control of Rho GTPase
Two types of RhoGAPs, both of which contain conserved RhoGAP domain, are known in plants, and both of them have been implicated in the spatial control of ROP activity.Citation21,Citation95 One family of Arabidopsis RhoGAPs with five members, termed RopGAPs, was initially identified in a yeast two-hybrid system for proteins interacting with constitutive active ROP1 (G15V).Citation46 These RopGAPs contain a conserved GAP-like domain and a N-terminal CRIB motif that enhances their affinity for the GTP-bound form of ROP and the GAP activity.Citation46 In tobacco pollen tubes, NtRhoGAP1 overexpression inhibited pollen tube elongation and caused narrower tubes, and GFP-tagged NtRhoGAP1 was localized preferentially in the shoulder of the tube apex.Citation21 These observations led to the proposal that RopGAPs restrict ROP signaling to the apex of pollen tubes by lateral inhibition,Citation21 as shown for several RhoGAPs in animal systems.Citation96–Citation99
Another type of RhoGAPs with three members in Arabidopsis was identified from genetic screen for ren mutations that enhance pollen tube tip swelling induced by ROP1 overexpression in Arabidopsis. REN1 contains an N-terminal PH domain and a RhoGAP domain, and two coiled-coil (CC) domains at the C-terminus (). This novel RhoGAP doesn't contain CRIB domain and is distinct from RhoGAPs in its cellular function.Citation95 Knocking out REN1 causes swollen pollen tubes that are reminiscent of those induced by the constitutively active form of ROP1. The ren1 phenotype together with biochemical data indicates that REN1 acts as ROP1 RhoGAP to negatively regulate tip-localized ROP1. REN1 is localized to exocytic vesicles in the pollen-tube apex and the apical PM region where ROP1 is activated. These results suggest that REN1 act as a global inhibitor to spatially restrict ROP1 activity to the apical PM.Citation95 Analysis of sptiotemporal dynamics of ROP1 activity in tobacco pollen tube tips also supports the existence of a global inhibitor in the restriction of ROP1 activity to the pollen tube apex.Citation99 These findings reveal a new paradigm for the spatial regulation of Rho GTPase activity by RhoGAPs, which is distinct from the lateral inhibition known for several RhoGAPs found in animal systems.Citation96–Citation98
It was proposed that the global mode of action for the REN RhoGAP facilitates the self-regulation of Rho GTPases such as that found in pollen tube tips.Citation99,Citation100 Indeed, it was shown that the apical PM localization of REN1 oscillates behind ROP1 activation and ahead of ROP1 deactivation.Citation95 Furthermore, REN1 function is dependent upon its targeting to the apical PM through its association with the exocytic vesicles, implying the presence of a negative feedback mechanism, in which ROP1-mediated polar exocytosis is important for REN1-mediated downregulation of ROP1.Citation95 Thus the REN1 RhoGAP action appears to couple the temporal regulation with the spatial regulation of ROP activity, generating a self-organizing system to control oscillating polar growth. Such a self-organizing design principle might also work in other highly efficient Rho GTPase-mediated cellular processes such as fungal hyphal growth, neuronal growth and cell movement.
RhoGDIs are also conserved and play an important role in the spatial regulation of ROP GTPase in plants.Citation20,Citation99 The Arabidopsis genome encodes three RhoGDI highly similar to mammalian RhoGDIs.Citation101 Loss-of-function of SCN1/AtRhoGDI1 (scn1) causes a phenotype in root hair similar to that induced by ROP2 overexpression since it leads to the mislocalization of ROP2 during root hair initiation and growth.Citation6,Citation7,Citation102 In wild type, ROP2::YFP localization is restricted to the PM at root hair growth sites or the apical PM of growing hairs; however in scn1 mutant, it accumulates at the cell surface in ectopic locations where numerous root hairs are initiated and remains at the cell surface in growing root hairs.Citation102 RNA interference lines of RhoGDI2a (gdi2a-RNAi) cause the depolarization of pollen tube that is also observed in GFP-ROP1 overexpression lines. And the localization of ROPs in pollen tube is depolarized in gdi2a-RNAi mutant that is similar to mislocalization of ROP2 in scn1 mutant root hairs.Citation99 In tabacco pollen tubes, transient expression of NtRhoGDI2 inhibits pollen tube growth while NtRac5 causes depolarization in pollen tube. But co-expression of both proteins produces normal pollen tube, which indicates that NtRhoGDI2 and NtRac5 are together to control the polar growth of pollen tubes. NtRhoGDI2 was localized to cytoplasm, and co-expression of NtRhoGDI2 and YFP:NtRac5 inhibits the accumulation of NtRac5 at PM and translocates NtRac5 to cytoplasm. A point mutation in NtRac5 (R69A) that abolished the specific binding between NtRhoGDI2 and NtRac5 caused the mislocalization of NtRac5 to the flanks of pollen tube apex and enhanced the ability of NtRac5 to depolarize pollen tube when overexpressed.Citation20 These findings demonstrate the role of RhoGDI in control of polar localization of RhoGTPases by depleting them from PM.
Future Perspectives
Plant invention of novel Rho effector proteins to regulate fundamental cellular activities such as cytoskeletal organization and vesicular trafficking raises an intriguing question: what is the necessity for plants to reinvent wheels for old cars? It is likely that these old “cars” have some plant-specific features that would work most efficiently on the new “wheels”, e.g., RIC1 organization of branched MTs into parallel bundles (a plant-specific feature of MT organization). Understanding how these novel ROP effectors regulate these fundamental cellular activities will most likely reveal interesting features of these activities. On the other hand, the studies of conserved ROP regulators in plant cell systems such as pollen tubes have revealed new paradigms regarding the spatial control of Rho GTPases. More new paradigms could be uncovered as ROP signaling mechanisms in multicellular plant systems are being elucidated. Furthermore, future studies of ROP effectors and regulators in mosses may generate insights into the evolution of tip growth mechanisms (reviewed in ref. Citation103). Elucidation of mechanisms activating the plant-specific RopGEFs will generate important new insights into molecular and cellular mechanisms of plant development.
Figures and Tables
Figure 1 Phylogenetic relationships of Rho-family small GTPases. A unrooted phylogenetic tree for representative members of the Rho family of small GTPases from different kingdoms. Representative members of ROP proteins are included, and only some members of Rho, CDC42 and Rac subfamilies are shown.
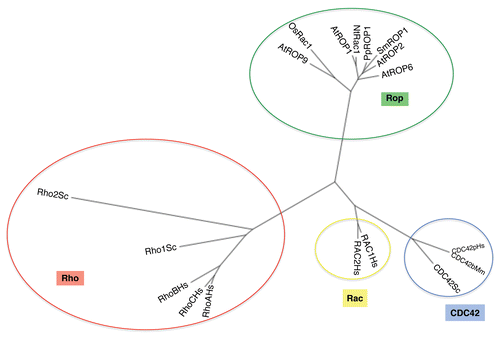
Figure 2 ROP signaling pathways in pollen tube and pavement cells. (A) ROP1 is locally activated at the apex of pollen tube PM. ROP1 activates downstream effector RIC4 that can promote F-actin assembly. ROP1 also activates another effector RIC3 that promotes Actin disassembly through regulating calcium concentration. These two pathways coordinately regulate actin dynamic that is essential for exocytosis at the sub-apical region of growing pollen tubes. REN1, a RhoGAP in plant, is translocated to the sub-apical region by exocytosis that negatively regulate ROP1 activity. (B) Counteraction of RIC3 and RIC4 in pollen tubes. All tubes are expressing pLat52::mTalin that marks actin, with co-expression of indicated RICs, and incubated with indicated amount of Ca concentration. Note that actin cable are protruding at the extreme tip of tubes in RIC3 expressing tube, while meshwork of actin covers the tip of RIC4 expressing tubes. Depolarized growth and abnormal actin organization are suppressed by coexpressing RIC3 and RIC4. Increase of Ca concentration also suppresses abnormal phenotypes induced by RIC4 expression. Images are reproduced from Gu et al.Citation13 (C) In leaf pavement cells that contain interdigitated lobes and indentations, ROP2 is locally activated at the apex of lobes by auxin through ABP1, which leads to the activation of RIC4 at lobe sites that promotes accumulation of F-Acin, which is essential for lobe outgrowth. Local active ROP2 also promotes PIN1 polar localization to apical PM of lobe. And PIN1-exported auxin in turn activates ROP2 at the cell surface to initiate a positive feedback loop which is essential for continuous outgrowth of lobe. PIN1-exported auxin at the lobe sites can diffuse across the cell wall to activate ROP6 pathway at adjacent cell. ROP6 activate RIC1 that can bind with MTs and regulate MT organization to restrict growth in this region. (D) Polar localization of ROP2, ROP6 and PIN1 in pavement cells. GFP-ROP6 signal is stronger in indenting region while GFP-ROP2 is stronger in lobe tip in adjacent lobes and indentations (arrows). Images are reproduced from Fu et al.Citation12 PIN1 localizes to tip of lobes in WT cells (arrows). Polar localization of PIN1 is lost and cytoplsmic signal is increased in rop2 RNAi rop4-1 cells (arrowheads). Images are reproduced from Xu et al.Citation68
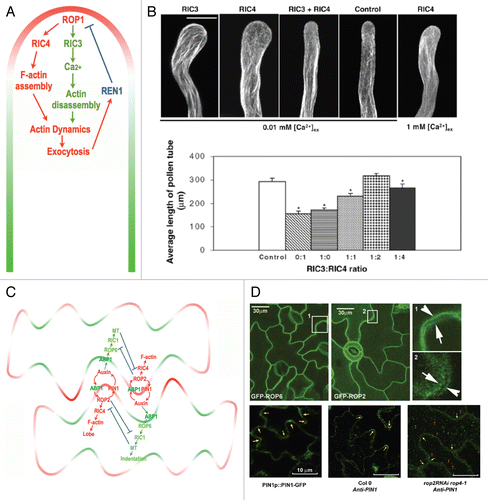
Figure 3 Comparison of CRIB motifs from CRIB-containing proteins found in plants and other kingdoms. Comparison of CRIB motifs of RIC proteins and other CRIB motif containing proteins. ROPGAPs from Arabidopsis as well as CDC42/Rac effector proteins are included. Note the high conservation of RIC proteins.
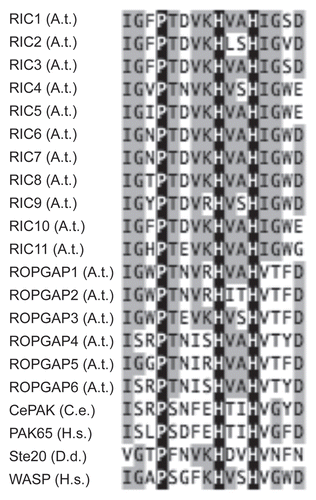
Figure 4 Novel ROP-interactive motif found in the C-terminal region of ICR/RIP proteins from Arabidopsis. Comparison of C-terminal conserved region in ICR/RIP proteins from Arabidopsis. Highly conserved QWRKAA motif required for ROP bining are highlighted.
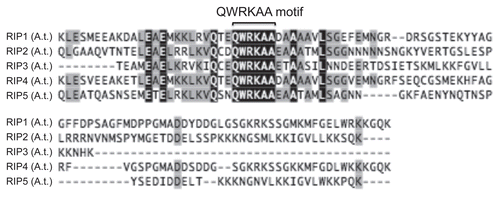
Figure 5 Rho mediated regulation of exocyst complex in different kingdoms. Models describing regulation of exocyst complex by Rho proteins in different kingdoms. Note the difference in subunit or adaptor/scaffolding protein in mediating Rho regulation.
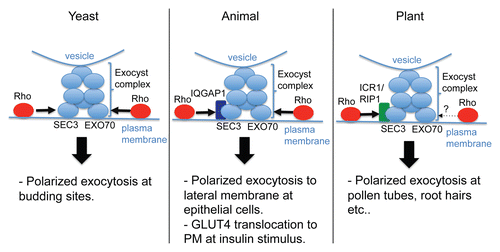
Figure 6 A working model for the polarization of PIN1 by ROP-mediated local suppression of endocytosis and polarized recycling. A working model describing PIN1 polarization achieved by ROP2-mediated regulation of both endocytosis and recycling. ROP2 activated by auxin is speculated to suppress endocytosis of PIN1 by unknown mechanisms. Active ROP2 presumably promotes recycling of PIN1 through the promotion of the ICR1/RIP1-mediated exocyst pathway. As a consequence, PIN1 accumulates at the side where ROP2 is activated, and transport auxin out of cell there. This will further increase auxin levels at the side, and thus a positive feedback loop is formed.
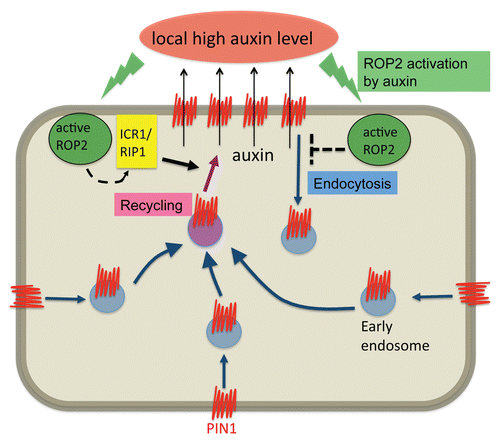
Figure 7 Schematic structures for two types of RhoGAPs in Arabidopsis. The AtRopGAP family that has 6 family members contains a CRIB domain for binding with RhoGTPase and a conserved RhoGAP domain. AtRENs family contains an N-terminal PH domain and a RhoGAP domain, and two coiled-coil (CC) domains at the C-terminus.

Table 1 List of ROP effectors
References
- Winge P, Brembu T, Bones AM. Cloning and characterization of Rac-like cDNAs from Arabidopsis thaliana. Plant Mol Biol 1997; 35:483 - 495
- Kawasaki T, Henmi K, Ono E, Hatakeyama S, Iwano M, Satoh H, et al. The small GTP-binding protein rac is a regulator of cell death in plants. Proc Natl Acad Sci USA 1999; 96:10922 - 10926
- Cheung AY, Chen CYH, Tao LZ, Andreyeva T, Twell D, Wu HM. Regulation of pollen tube growth by Rac-like GTPases. J Exp Bot 2003; 54:73 - 81
- Kost B, Lemichez E, Spielhofer P, Hong Y, Tolias K, Carpenter C, et al. Rac homologues and compartmentalized phosphatidylinositol-4,5-bisphosphate act in a common pathway to regulate polar pollen tube growth. J Cell Biol 1999; 145:317 - 330
- Fu Y, Wu G, Yang Z. Rop GTPase-dependent dynamics of tip-localized F-actin controls tip growth in pollen tubes. J Cell Biol 2001; 152:1019 - 1032
- Molendijk AJ, Bischoff F, Rajendrakumar CS, Friml J, Braun M, Gilroy S, et al. Arabidopsis thaliana Rop GTPases are localized to tips of root hairs and control polar growth. EMBO J 2001; 20:2779 - 2788
- Jones MA, Shen JJ, Fu Y, Li H, Yang Z, Grierson CS. The Arabidopsis Rop2 GTPase is a positive regulator of both root hair initiation and tip growth. Plant Cell 2002; 14:763 - 776
- Arthur KM, Vejlupkova Z, Meeley RB, Fowler JE. Maize ROP2 GTPase provides a competitive advantage to the male gametophyte. Genetics 2003; 165:2137 - 2151
- Chen CYH, Cheung AY, Wu HM. Actin-depolymerizing factor mediates Rac/Rop GTPase-regulated pollen tube growth. Plant Cell 2003; 15:237 - 249
- Basu D, El-Assal SED, Le J, Mallery EL, Szymanski DB. Interchangeable functions of Arabidopsis PIROGI and the human WAVE complex subunit SRA1 during leaf epidermal development. Development 2004; 131:4345 - 4355
- Fu Y, Gu Y, Zheng Z, Wasteneys G, Yang Z. Arabidopsis interdigitating cell growth requires two antagonistic pathways with opposing action on cell morphogenesis. Cell 2005; 120:687 - 700
- Fu Y, Xu T, Zhu L, Wen M, Yang Z. A ROP GTPase signaling pathway controls cortical microtubule ordering and cell expansion in Arabidopsis. Curr Biol 2009; 19:1827 - 1832
- Gu Y, Fu Y, Dowd P, Li S, Vernoud V, Gilroy S, et al. A Rho family GTPase controls actin dynamics and tip growth via two counteracting downstream pathways in pollen tubes. J Cell Biol 2005; 169:127 - 138
- Lee YJ, Szumlanski A, Nielsen E, Yang Z. Rho-GTPase-dependent filamentous actin dynamics coordinate vesicle targeting and exocytosis during tip growth. J Cell Biol 2008; 181:1155 - 1168
- Lavy M, Bloch D, Hazak O, Gutman I, Poraty L, Sorek N, et al. A Novel ROP/RAC effector links cell polarity, root-meristem maintenance and vesicle trafficking. Curr Biol 2007; 17:947 - 952
- Uhrig JF, Mutondo M, Zimmermann I, Deeks MJ, Machesky LM, Thomas P, et al. The role of Arabidopsis SCAR genes in ARP2-ARP3-dependent cell morphogenesis. Development 2007; 134:967 - 977
- Hazak O, Bloch D, Poraty L, Sternberg H, Zhang J, Friml J, et al. A rho scaffold integrates the secretory system with feedback mechanisms in regulation of auxin distribution. PLoS Biol 2010; 8:1000282
- Kawasaki T, Koita H, Nakatsubo T, Hasegawa K, Wakabayashi K, Takahashi H, et al. Cinnamoyl-CoA reductase, a key enzyme in lignin biosynthesis, is an effector of small GTPase Rac in defense signaling in rice. Proc Natl Acad Sci USA 2006; 103:230 - 235
- Gu Y, Li S, Lord EM, Yang Z. Members of a novel class of Arabidopsis Rho guanine nucleotide exchange factors control Rho GTPase-dependent polar growth. Plant Cell 2006; 18:366 - 381
- Klahre U, Becker C, Schmitt AC, Kost B. Nt-RhoGDI2 regulates Rac/Rop signaling and polar cell growth in tobacco pollen tubes. Plant J 2006; 46:1018 - 1031
- Klahre U, Kost B. Tobacco RhoGTPase ACTIVATING PROTEIN1 spatially restricts signaling of RAC/Rop to the apex of pollen tubes. Plant Cell 2006; 18:3033 - 3046
- Zhang Y, McCormick S. A distinct mechanism regulating a pollen-specific guanine nucleotide exchange factor for the small GTPase Rop in Arabidopsis thaliana. Proc Natl Acad Sci USA 2007; 104:18830 - 18835
- Lee YJ, Yang ZB. Tip growth: signaling in the apical dome. Curr Opin Plant Biol 2008; 11:662 - 671
- Yang Z. Cell polarity signaling in Arabidopsis. Annu Rev Cell Dev Biol 2008; 24:551 - 575
- Park J, Choi HT, Lee S, Lee T, Yang ZB, Lee Y. Racrelated GTP-binding protein in elicitor-induced reactive oxygen generation by suspension-cultured soybean cells. Plant Physiol 2000; 124:725 - 732
- Ono E, Wong HL, Kawasaki T, Hasegawa M, Kodama O, Shimamoto K. Essential role of the small GTPase Rac in disease resistance of rice. Proc Natl Acad Sci USA 2001; 98:759 - 764
- Agrawal GK, Iwahashi I, Rakwal R. Small GTPase ‘Rop’: molecular switch for plant defense responses. Febs Lett 2003; 546:173 - 180
- Schultheiss H, Dechert C, Kogel KH, Huckelhoven R. Functional analysis of barley RAC/ROP G-protein family members in susceptibility to the powdery mildew fungus. Plant J 2003; 36:589 - 601
- Moeder W, Yoshioka K, Klessig DF. Involvement of the small GTPase Rac in the defense responses of tobacco to pathogens. Mol Plant Microbe Interact 2005; 18:116 - 124
- Schultheiss H, Hensel G, Imani J, Broeders S, Sonnewald U, Kogel KH, et al. Ectopic expression of constitutively activated RACB in barley enhances susceptibility to powdery mildew and abiotic stress. Plant Physiol 2005; 139:353 - 362
- Fujiwara M, Umemura K, Kawasaki T, Shimamoto K. Proteomics of Rac GTPase signaling reveals its predominant role in elicitor-induced defense response of cultured rice cells. Plant Physiol 2006; 140:734 - 745
- Wong HL, Pinontoan R, Hayashi K, Tabata R, Yaeno T, Hasegawa K, et al. Regulation of rice NADPH oxidase by binding of Rac GTPase to its N-terminal extension. Plant Cell 2007; 19:4022 - 4034
- Thao NP, Chen L, Nakashima A, Hara S, Umemura K, Takahashi A, et al. RAR1 and HSP90 form a complex with Rac/Rop GTPase and function in innate-immune responses in rice. Plant Cell 2007; 19:4035 - 4045
- Nakashima A, Chen L, Thao NP, Fujiwara M, Wong HL, Kuwano M, et al. RACK1 functions in rice innate immunity by interacting with the Rac1 immune complex. Plant Cell 2008; 20:2265 - 2279
- Pathuri IP, Zellerhoff N, Schaffrath U, Hensel G, Kumlehn J, Kogel KH, et al. Constitutively activated barley ROPs modulate epidermal cell size, defense reactions and interactions with fungal leaf pathogens. Plant Cell Rep 2008; 27:1877 - 1887
- Schultheiss H, Preuss J, Pircher T, Eichmann R, Huckelhoven R. Barley RIC171 interacts with RACB in planta and supports entry of the powdery mildew fungus. Cell Microbiol 2008; 10:1815 - 1826
- Baxter-Burrell A, Yang Z, Springer PS, Bailey-Serres J. RopGAP4-dependent Rop GTPase rheostat control of Arabidopsis oxygen deprivation tolerance. Science 2002; 296:2026 - 2028
- Tao LZ, Cheung AY, Wu HM. Plant Rac-like GTPases are activated by auxin and mediate auxin-responsive gene expression. Plant Cell 2002; 14:2745 - 2760
- Zheng ZL, Nafisi M, Tam A, Li H, Crowell DN, Chary SN, et al. Plasma membrane-associated ROP10 small GTPase is a specific negative regulator of abscisic acid responses in Arabidopsis. Plant Cell 2002; 14:2787 - 2797
- Tao LZ, Cheung AY, Nibau C, Wu HM. RAC GTPases in tobacco and Arabidopsis mediate auxin-induced formation of proteolytically active nuclear protein bodies that contain AUX/IAA proteins. Plant Cell 2005; 17:2369 - 2383
- Xin Z, Zhao Y, Zheng ZL. Transcriptome analysis reveals specific modulation of abscisic acid signaling by ROP10 small GTPase in Arabidopsis. Plant Physiol 2005; 139:1350 - 1365
- Jeon BW, Hwang JU, Hwang Y, Song WY, Fu Y, Gu Y, et al. The Arabidopsis small G protein ROP2 is activated by light in guard cells and inhibits light-induced stomatal opening. Plant Cell 2008; 20:75 - 87
- Bishop AL, Hall A. Rho GTPases and their effector proteins. Biochemical Journal 2000; 348:241 - 255
- Perez P, Rincon SA. Rho GTPases: regulation of cell polarity and growth in yeasts. Biochemical Journal 2010; 426:243 - 253
- Wu G, Gu Y, Li S, Yang Z. A genome-wide analysis of Arabidopsis Rop-interactive CRIB motif-containing proteins that act as Rop GTPase targets. Plant Cell 2001; 13:2841 - 2856
- Wu G, Li H, Yang Z. Arabidopsis RopGAPs are a novel family of rho GTPase-activating proteins that require the Cdc42/Rac-interactive binding motif for rop-specific GTPase stimulation. Plant Physiol 2000; 124:1625 - 1636
- Hsu SW, Cheng CL, Tzen TCJ, Wang CS. Rop GTPase and Its Target Cdc42/Rac-Interactive-Binding Motif-Containing Protein Genes Respond to Desiccation during Pollen Maturation. Plant and Cell Physiology 2010; 51:1197 - 1209
- Gu Y, Vernoud V, Fu Y, Yang Z. ROP GTPase regulation of pollen tube growth through the dynamics of tip-localized F-actin. J Exp Bot 2003; 54:93 - 101
- Hong-Geller E, Cerione RA. Cdc42 and Rac stimulate exocytosis of secretory granules by activating the IP3/calcium pathway in RBL-2H3 mast cells. J Cell Biol 2000; 148:481 - 493
- Mehta D, Ahmmed GU, Paria BC, Holinstat M, Voyno-Yasenetskaya T, Tiruppathi C, et al. RhoA interaction with inositol-1,4,5-trisphosphate receptor and transient receptor potential channel-1 regulates Ca2+ entry—Role in signaling increased endothelial permeability. J Biol Chem 2003; 278:33492 - 33500
- Yan A, Xu GS, Yang ZB. Calcium participates in feedback regulation of the oscillating ROP1 Rho GTPase in pollen tubes. P Natl Acad Sci USA 2009; 106:22002 - 22007
- Li S, Gu Y, Yan A, Lord E, Yang ZB. RIP1 (ROP Interactive Partner 1)/ICR1 marks pollen germination sites and may act in the ROP1 pathway in the control of polarized pollen growth. Mol Plant 2008; 1:1021 - 1035
- Zajac A, Sun X, Zhang J, Guo W. Cyclical regulation of the exocyst and cell polarity determinants for polarized cell growth. Mol Biol Cell 2005; 16:1500 - 1512
- Zhang X, Bi E, Novick P, Du L, Kozminski KG, Lipschutz JH, et al. Cdc42 interacts with the exocyst and regulates polarized secretion. J Biol Chem 2001; 276:46745 - 46750
- Sakurai-Yageta M, Recchi C, Le Dez G, Sibarita JB, Daviet L, Camonis J, et al. The interaction of IQGAP1 with the exocyst complex is required for tumor cell invasion downstream of Cdc42 and RhoA. J Cell Biol 2008; 181:985 - 998
- Hala M, Cole R, Synek L, Drdova E, Pecenkova T, Nordheim A, et al. An exocyst complex functions in plant cell growth in Arabidopsis and tobacco. Plant Cell 2008; 20:1330 - 1345
- Synek L, Schlager N, Elias M, Quentin M, Hauser MT, Zarsky V. AtEXO70A1, a member of a family of putative exocyst subunits specifically expanded in land plants, is important for polar growth and plant development. Plant J 2006; 48:54 - 72
- Friml J, Benkova E, Blilou I, Wisniewska J, Hamann T, Ljung K, et al. AtPIN4 mediates sink-driven auxin gradients and root patterning in Arabidopsis. Cell 2002; 108:661 - 673
- Benkova E, Michniewicz M, Sauer M, Teichmann T, Seifertova D, Jurgens G, et al. Local, efflux-dependent auxin gradients as a common module for plant organ formation. Cell 2003; 115:591 - 602
- Friml J, Vieten A, Sauer M, Weijers D, Schwarz H, Hamann T, et al. Efflux-dependent auxin gradients establish the apical-basal axis of Arabidopsis. Nature 2003; 426:147 - 153
- Reinhardt D, Pesce ER, Stieger P, Mandel T, Baltensperger K, Bennett M, et al. Regulation of phyllotaxis by polar auxin transport. Nature 2003; 426:255 - 260
- Blilou I, Xu J, Wildwater M, Willemsen V, Paponov I, Friml J, et al. The PIN auxin efflux facilitator network controls growth and patterning in Arabidopsis roots. Nature 2005; 433:39 - 44
- Abas L, Benjamins R, Malenica N, Paciorek T, Wirniewska J, Moulinier-Anzola JC, et al. Intracellular trafficking and proteolysis of the Arabidopsis auxinefflux facilitator PIN2 are involved in root gravitropism. Nat Cell Biol 2006; 8:249 - 256
- Li L, Xu J, Xu ZH, Xue HW. Brassinosteroids stimulate plant tropisms through modulation of polar auxin transport in Brassica and Arabidopsis. Plant Cell 2005; 17:2738 - 2753
- Bloch D, Lavy M, Efrat Y, Efroni I, Bracha-Drori K, Abu-Abied M, et al. Ectopic expression of an activated RAC in Arabidopsis disrupts membrane cycling. Mol Biol Cell 2005; 16:1913 - 1927
- Dhonukshe P, Tanaka H, Goh T, Ebine K, Mahonen AP, Prasad K, et al. Generation of cell polarity in plants links endocytosis, auxin distribution and cell fate decisions. Nature 2008; 456:962 - 966
- Paciorek T, Zazimalova E, Ruthardt N, Petrasek J, Stierhof YD, Kleine-Vehn J, et al. Auxin inhibits endocytosis and promotes its own efflux from cells. Nature 2005; 435:1251 - 1256
- Robert S, Kleine-Vehn J, Barbez E, Sauer M, Paciorek T, Baster P, et al. ABP1 mediates auxin inhibition of clathrin-dependent endocytosis in Arabidopsis. Cell 2010; 143:111 - 121
- Xu T, Wen M, Nagawa S, Fu Y, Chen JG, Wu MJ, et al. Cell Surface- and Rho GTPase-Based Auxin Signaling Controls Cellular Interdigitation in Arabidopsis. Cell 2010; 143:99 - 110
- Molendijk AJ, Ruperti B, Singh MK, Dovzhenko A, Ditengou FA, Milia M, et al. A cysteine-rich receptorlike kinase NCRK and a pathogen-induced protein kinase RBK1 are Rop GTPase interactors. Plant J 2008; 53:909 - 923
- Dorjgotov D, Jurca ME, Fodor-Dunai C, Szucs A, Otvos K, Klement E, et al. Plant Rho-type (Rop) GTPase-dependent activation of receptor-like cytoplasmic kinases in vitro. Febs Lett 2009; 583:1175 - 1182
- Lieberherr D, Thao NP, Nakashima A, Umemura K, Kawasaki T, Shimamoto K. A sphingolipid elicitorinducible mitogen-activated protein kinase is regulated by the small GTPase OsRac1 and heterotrimeric G-protein in rice 1[w]. Plant Physiol 2005; 138:1644 - 1652
- Hordijk PL. Regulation of NADPH oxidases—The role of Rac proteins. Circulation Research 2006; 98:453 - 462
- Ivanchenko M, Vejlupkova Z, Quatrano RS, Fowler JE. Maize ROP7 GTPase contains a unique, CaaX box-independent plasma membrane targeting signal. Plant J 2000; 24:79 - 90
- Lavy M, Bracha-Drori K, Sternberg H, Yalovsky S. A cell-specific, prenylation-independent mechanism regulates targeting of type II RACs. Plant Cell 2002; 14:2431 - 2450
- Lavy M, Yalovsky S. Association of Arabidopsis type-II ROPs with the plasma membrane requires a conserved C-terminal sequence motif and a proximal polybasic domain. Plant J 2006; 46:934 - 947
- Sorek N, Poraty L, Sternberg H, Bar E, Lewinsohn E, Yalovsky S. Activation status-coupled transient S acylation determines membrane partitioning of a plant Rho-related GTPase. Mol Cell Biol 2007; 27:2144 - 2154
- Sorek N, Segev O, Gutman O, Bar E, Richter S, Poraty L, et al. An S-Acylation Switch of Conserved G Domain Cysteines Is Required for Polarity Signaling by ROP GTPases. Curr Biol 2010; 20:1326
- Yalovsky S, Bloch D, Sorek N, Kost B. Regulation of membrane trafficking, cytoskeleton dynamics and cell polarity by ROP/RAC GTPases. Plant Physiol 2008; 147:1527 - 1543
- Hemsley PA, Grierson CS. Multiple roles for protein palmitoylation in plants. Trends Plant Sci 2008; 13:295 - 302
- Meller N, Merlot S, Guda C. CZH proteins: a new family of Rho-GEFs. J Cell Sci 2005; 118:4937 - 4946
- Fukuhara S, Murga C, Zohar M, Igishi T, Gutkind JS. A novel PDZ domain containing guanine nucleotide exchange factor links heterotrimeric G proteins to Rho. J Biol Chem 1999; 274:5868 - 5879
- Schlessinger J. Cell signaling by receptor tyrosine kinases. Cell 2000; 103:211 - 225
- Sah VP, Seasholtz TM, Sagi SA, Brown JH. The role of Rho in G protein-coupled receptor signal transduction. Annu Rev Pharmacol 2000; 40:459 - 489
- Schiller MR, Chakrabarti K, King GF, Schiller NI, Eipper BA, Maciejewski MW. Regulation of RhoGEF activity by intramolecular and intermolecular SH3 domain interactions. J Biol Chem 2006; 281:18774 - 18786
- Yang Z. Small GTPases: versatile signaling switches in plants. Plant Cell 2002; 14:375 - 388
- Qiu JL, Jilk R, Marks MD, Szymanski DB. The Arabidopsis SPIKE1 gene is required for normal cell shape control and tissue development. Plant Cell 2002; 14:101 - 118
- Fu Y, Li H, Yang Z. The ROP2 GTPase controls the formation of cortical fine F-actin and the early phase of directional cell expansion during Arabidopsis organogenesis. Plant Cell 2002; 14:777 - 794
- Basu D, Le J, Zakharova T, Mallery EL, Szymanski DB. A SPIKE1 signaling complex controls actin-dependent cell morphogenesis through the heteromeric WAVE and ARP2/3 complexes. Proc Natl Acad Sci USA 2008; 105:4044 - 4049
- Berken A, Thomas C, Wittinghofer A. A new family of RhoGEFs activates the Rop molecular switch in plants. Nature 2005; 436:1176 - 1180
- Thomas C, Fricke I, Scrima A, Berken A, Wittinghofer A. Structural evidence for a common intermediate in small G protein-GEF reactions. Mol Cell 2007; 25:141 - 149
- Kaothien P, Ok SH, Shuai B, Wengier D, Cotter R, Kelley D, et al. Kinase partner protein interacts with the LePRK1 and LePRK2 receptor kinases and plays a role in polarized pollen tube growth. Plant J 2005; 42:492 - 503
- Cao Y, Li Z, Chen T, Zhang Z, Zhang J, Chen S. Overexpression of a tobacco small G protein gene NtRop1 causes salt sensitivity and hydrogen peroxide production in transgenic plants. Sci China C Life Sci 2008; 51:383 - 390
- Cartwright HN, Humphries JA, Smith LG. PAN1: a receptor-like protein that promotes polarization of an asymmetric cell division in maize. Science 2009; 323:649 - 651
- Hwang JU, Vernoud V, Szumlanski A, Nielsen E, Yang Z. A tip-localized RhoGAP controls cell polarity by globally inhibiting Rho GTPase at the cell apex. Curr Biol 2008; 18:1907 - 1916
- Jenkins N, Saam JR, Mango SE. CYK-4/GAP provides a localized cue to initiate anteroposterior polarity upon fertilization. Science 2006; 313:1298 - 1301
- Wells CD, Fawcett JP, Traweger A, Yamanaka Y, Goudreault M, Elder K, et al. A Rich1/Amot complex regulates the Cdc42 GTPase and apical-polarity proteins in epithelial cells. Cell 2006; 125:535 - 548
- Knaus M, Pelli-Gulli MP, van Drogen F, Springer S, Jaquenoud M, Peter M. Phosphorylation of Bem2p and Bem3p may contribute to local activation of Cdc42p at bud emergence. EMBO J 2007; 26:4501 - 4513
- Hwang JU, Wu G, Yan A, Lee YJ, Grierson CS, Yang Z. Pollen-tube tip growth requires a balance of lateral propagation and global inhibition of Rho-family GTPase activity. J Cell Sci 2010; 123:340 - 350
- Hwang JU, Gu Y, Lee YJ, Yang Z. Oscillatory ROP GTPase activation leads the oscillatory polarized growth of pollen tubes. Mol Biol Cell 2005; 16:5385 - 5399
- Bischoff F, Vahlkamp L, Molendijk A, Palme K. Localization of AtROP4 and AtROP6 and interaction with the guanine nucleotide dissociation inhibitor AtRhoGDI1 from Arabidopsis. Plant Mol Biol 2000; 42:515 - 530
- Carol RJ, Takeda S, Linstead P, Durrant MC, Kakesova H, Derbyshire P, et al. A RhoGDP dissociation inhibitor spatially regulates growth in root hair cells. Nature 2005; 438:1013 - 1016
- Eklund DM, Svensson EM, Kost B. Physcomitrella patens: a model to investigate the role of RAC/ROP GTPase signalling in tip growth. J Exp Bot 2010; 61:1917 - 1937