Abstract
The Ras family is the largest and most diverse sub-group of Ras-like G proteins. This complexity is further increased by the high number of regulatory Guanine nucleotide Exchange Factors (GEFs) and GTPase activating proteins (GAPs) that target specific members of this subfamily. Di-Ras1 and Di-Ras2 are little characterized members of the Raslike sub-group with still unidentified regulatory and effector proteins. Here we determined the nucleotide binding properties of Di-Ras1/Di-Ras2. The above nanomolar affinity and the inability to react with members of the Cdc25 RasGEF family might suggest that activation does not require a GEF. We identified Rap1GAP1 and Rap1GAP2 as specific GTPase activating proteins of the Di-Ras family. Dual-specificity GAPs of the GAP1m family could not activate Di-Ras proteins, despite the presence of the required catalytic residue. Although DiRas proteins share GAPs with Rap G proteins, no common effectors could be identified in vitro.
Introduction
The superfamily of Ras-like GTP-binding proteins (G proteins) is subdivided in at least 5 families including Ras, Rho, Ran, Arf and Rab proteins. The Ras sub-family consists of 35 membersCitation1 that are grouped according to their sequence homology and lack of specific functional motifs that would classify them as members of the other families. This renders the Ras subgroup the most diverse group with highly heterologous functions.
Common features of most Ras proteins are a high nucleotide affinity and a low GTPase activity. Hence, Ras proteins interact with Guanine nucleotide exchange factors (GEF) that increase nucleotide exchange up to 105 fold and with GTPase activating proteins (GAP) that catalyze the GTPase reaction by a similar magnitude.Citation2
Within the Ras subfamily, three different proteins are designated Di-Ras1/Rig, Di-Ras2 and Di-Ras3/ARHI/Noey2. ARHI is classified as Di-Ras protein, based on a sequence homology of 48% and 46% to Di-Ras1 and Di-Ras2, respectively. However, features like an extended N-terminus and the diversity of important sequence motifs (see below) suggest that this protein should not be sorted into the Di-Ras family. Hence, the term Di-Ras proteins used here refers to Di-Ras1 and Di-Ras2.
Di-Ras proteins are predominantly expressed in brain and heartCitation3,Citation4 with Di-Ras1 being described as potential tumor suppressor protein that is downregulated in human glioblastomas.Citation4,Citation5 It further inhibits cell growth and survival, when overexpressed. In Elk-1-dependent luciferase assays, Di-Ras1 antagonizes H-Ras signaling, leading to the suggestion that Di-Ras1 competes with Ras for binding to effector molecules like the Raf-Kinase.Citation4 However other results exclude an interaction between Di-Ras1/Di-Ras2 and H-/K-/N-Ras effectorsCitation3 and propose the existence of a different set of effector molecules. These might include the ArfGAP/RhoGAP protein Arap1.Citation6 The biological function of Di-Ras1/Di-Ras2 remains unknown. Overexpression of either leads to formation of vacuoles in HEK293T cells suggesting comparable biochemical functions.Citation3
Like other members of the Ras-family, Di-Ras1/Di-Ras2 exhibit a low intrinsic nucleotide hydrolysis activity.Citation3 In contrast, intrinsic nucleotide exchange seems to be faster for Di-Ras1 compared to H-Ras.Citation3
GAPs for G proteins of the Ras subfamily are usually specific for a subset of proteins. NF1, p120GAP and GAP1m are active on H-/N-/K-Ras as well as members of the R-Ras group.Citation7,Citation8 R-Ras also interacts with R-RasGAP that additionally stimulates TC21 but not M-Ras.Citation7,Citation8 Rap1 and Rap2 are activated by proteins of the Rap1GAP family.Citation9 The Ras family member Rheb responds to the Rap1GAP homologue Tuberin/Tsc2.Citation10 GTPase activating proteins for other, less well-known members of the Ras subfamily, such as the Di-Ras group, are not identified yet.
The catalytic function of GAP proteins relies on completion and precise arrangement of the G protein catalytic center.Citation11 Many GAPs use a positively charged residue, the ‘Arg finger’, to counteract developing negative charges of the nucleotide during hydrolysis.Citation12–Citation15 The nucleophilic water is positioned by an essential hydrophilic residue that has the ability to act as hydrogen bond donor and acceptor. In the case of Ras, Ran, Rho and Arf/Sar proteins such a residue, Gln or His, is provided by the G protein itself (e.g., Gln61 in Ras), or it is supplied in trans by the GAP protein as in the Rab/RabGAP system.Citation14
Rap1GAPs are mechanistically exceptional compared to other GAPs, because they provide an invariant Asn residue (the Asn thumb) compensating for Gln61, which Rap proteins lack.Citation10,Citation16 A similar, Asn-based mechanism is anticipated for the RhebGAP Tuberin/Tsc2 due to its homology with Rap1GAPCitation10,Citation16 and despite the fact that Rheb possesses a Gln at the required position.
Interestingly, a family of dual-specificity GAPs was identified that act on both Ras and Rap proteins.Citation17 These GAPs use the intrinsic Gln and the conventional Arg finger mechanism on Ras proteins. On Rap G proteins, they use additional domains extra to the GAP domain that reorient switch II and employ another Gln residue in the switch II motif to take over the function of the catalytic Gln61.Citation18
In order to further characterize the Di-Ras proteins we tested GTPase activating proteins and Guanine nucleotide exchange factors for their ability to regulate Di-Ras1 and Di-Ras2. Our results indicate that the Di-Ras proteins belong to the Rap family of Ras-like proteins as they are substrates of classical Rap1GAPs. However, none of the tested Ras-family GEFs induced a significant increase in nucleotide exchange. The Ras-/Rap-effectors Raf(RBD), RalGDS(RA), Arap1 and Arap3 did not interact with Di-Ras proteins in vitro.
Results
Nucleotide affinity of Di-Ras1/Di-Ras2 is in the nanomolar range.
The family of Ras-like G proteins feature a very high nucleotide affinity in the picomolar to low nanomolar range, basically determined by a low off-rate. In order to calculate affinities for different nucleotides to Di-Ras1/Di-Ras2, both proteins were preloaded with mGDP or mGppNHp and nucleotide off-rates were measured. mGDP and mGppNHp exhibited similar dissociation rates ( and ). Interestingly, the dissociation rates are approximately 100–400x faster than the H-Ras-mGDP off-rate of 1.2 × 10−5 s−1.Citation19
To allow for determination of on-rates, both proteins were freed of nucleotide and brought into the guanosine-bound state following alkaline phosphatase/phosphodiesterase treatment.Citation20 During this procedure, Di-Ras1 precipitated under all conditions tested and could not be used for association rate measurements. Di-Ras2 was reacted with different concentrations of mant-nucleotides under pseudo-first order conditions. Observed rates were plotted against nucleotide concentrations to get a rate constant of 0.357 s−1µM−1 for mGDP and 3x lower for mGppNHp (0.099 s−1µM−1) ( and C). Using the nucleotide off-rates, nucleotide affinities of Di-Ras2 can be calculated to 7.8 nM for mgdp and 12 nM for mGppNHp, respectively (). Given that the off-rates as the major determinants of affinity are similar between Di-Ras1 and 2 we estimate similar affinities for Di-Ras1.
Di-Ras1/Di-Ras2 are independent of canonical Ras- and RapGEFs.
Although intrinsic Di-Ras1/2 nucleotide exchange rates are faster than those of other Ras-family members, they may or may not be sufficient for fast in vivo signaling with half-lifes of 2 min (Di-Ras1, mGDP) and 4 min (Di-Ras2, mGDP) (). We thus tested the Ras-specific GEF Sos, the RapGEFs Epac1, Epac2 and C3G and RasGEF1A and RasGEF1B as Rap2-specifc GEFs. A generally accepted Rheb-GEF has not been identified so far.Citation21,Citation22
The RapGEFs Epac1 (+cAMP), Epac2 (+cAMP), C3G and the RasGEF Sos did not show nucleotide exchange catalysis on Di-Ras1 or Di-Ras2 (). The specific Rap2-GEFs, previously called RasGEF1A/RasGEF1B, were likewise inactive on Di-Ras1/Di-Ras2 (data not shownCitation23). Hence, we were not able to identify Di-Ras GEFs, underlining the general observation that Ras-family GEFs act highly specific. However, this leaves open the question, whether Di-Ras proteins function independently of GEFs or have unexpected and not yet identified exchange factors.
Di-Ras1 and Di-Ras2 are activated by Rap1GAP1 and Rap1GAP2.
In order to identify GTPase activating proteins for Di-Ras1 and Di-Ras2, we tested the activity of known Ras-family GAPs at different concentrations. As expected, Di-Ras1 and Di-Ras2 showed no significant intrinsic nucleotide hydrolysis (Di-Ras1: 0.0002 min−1, Di-Ras2, not detectable). Addition of 0.05 µM Rap1GAP1 increased nucleotide hydrolysis of Di-Ras1 approximately 150-fold (). Rap1GAP1 also showed activity on Di-Ras2 with similar concentration dependent hydrolysis rates (). GAP activity on Rap1 was 30-fold (Di-Ras2: 33-fold) higher compared to Di-Ras proteins, using 0.15 µM Rap1GAP1 ().
The homologous protein Rap1GAP2 also activated nucleotide hydrolysis of Di-Ras1 and Di-Ras2. However, higher concentrations of Rap1GAP2 were necessary to observe comparable rates as with Rap1GAP1. 0.25 µM Rap1GAP2 showed rates of 0.018 min−1 and 0.0065 min−1 for Di-Ras1 and Di-Ras2, respectively, being 14 (Di-Ras2: 37)-fold slower than with Rap1GAP1. Rap1GAP2 activity on Rap1 is also lower, indicating a generally lower activity of this GAP.
Further RapGAP proteins that belong to the group of dual-specificity RasGAPs were tested on Di-Ras1 and Di-Ras2. GAP1IP4BP and Rasal showed no activity on Di-Ras proteins at low concentrations of 0.05 µM ( and ). At very high concentrations of 50 µM both GAPs accelerated nucleotide hydrolysis of Di-Ras1 with rate constants of 0.011 min−1/0.0012 min−1. However, Rap1 was stimulated significantly stronger at concentrations of just 0.05 µM. Catalytic activity of both GAPs on Di-Ras2 was negligible even at 50 µM concentration, showing that GAP1IP4BP and Rasal are no genuine GAPs for Di-Ras2.
As representative for canonical RasGAPs, NF1 was tested for its activity on Di-Ras1/2 and no significant activation of nucleotide hydrolysis could be detected. The RhebGAP Tuberin was likewise inactive on Di-Ras1 and Di-Ras2, whereas the control with Rheb showed hydrolysis activity. This demonstrates that Di-Ras1 and Di-Ras2 are not activated by GAP proteins of other family members.
Di-Ras1 and Di-Ras2 do not show significant affinity to Raf(RBD) or RalGDS (RA).
There are conflicting reports, whether Di-Ras1 and Di-Ras2 bind to Ras/Rap effector proteins. We tested binding of Di-Ras1/Di-Ras2 to the RBD domain of Raf and the RA domain of RalGDS in vitro, using GDI assays and fluorescence polarisation experiments. In GDI assays, high concentrations of 100 µM Raf(RBD) or RalGDS(RA) did not cause a significant reduction in mGppNHp exchange of Di-Ras1/Di-Ras2, showing that neither binds with high affinity to Ras effectors (data not shown). In fluorescence polarisation experiments, an increase of polarisation could be detected after adding either GST-Raf(RBD) or RalGDS(RA) to mGppNHp bound Di-Ras proteins. However, high amounts of Ras-effectors were needed and gave estimated affinities in a high µM to mM range (). Hence, Di-Ras proteins would not be able to compete with Ras or Rap1 in binding to Raf(RBD) or RalGDS(RA) (), respectively.
We further tested the interaction of Arap1(RA) and Arap3(RA) to Di-Ras1 or Di-Ras2, but could not detect binding under various conditions using different methods (data not shown).
Discussion
In the current study, we have identified Rap1GAP proteins as GTPase activating proteins for Di-Ras1 and Di-Ras2. An alignment of Di-Ras1 and Di-Ras2 with other Ras-family members shows that the conserved glutamine (Q61 in Ras) is replaced by serine in Di-Ras proteins (). This feature is shared by Rap proteins, which exhibit a threonine at the corresponding position, suggesting a common hydrolysis activation mechanism for Rap and Di-Ras proteins.
However, the activity of Rap1GAPs on Di-Ras1/Di-Ras2 is lower than on Rap1 and thus more comparable to Rap2.Citation24 Given that the catalytic machinery of the Di-Ras proteins, Rap1 and Rap2 are identical, sequence differences between Rap1 and Di-Ras1/Di-Ras2 that affect affinity are most likely responsible for the differences in activity. The binding site of Rap1GAP1 mainly includes switch I, switch II and adjacent residues (). A general comparison of all interacting residues shows, that the majority is conserved between Di-Ras proteins and Rap1 ( left, red surface). Two important residues (Gln63, Phe64 in Rap1) that were shown to abrogate GAP activity when mutated,Citation10 are conserved in Di-Ras1/2, guaranteeing sufficient affinity. The function of Thr61 of Rap1, which is required for binding rather than catalysisCitation25 might be taken over by Ser of Di-Ras1/Di-Ras2.
The number of residues, which participate in binding but are not conserved between Rap and Di-Ras1/2 is low. His66 (Di-Ras1/Di-Ras2) could be a candidate residue that is responsible for lower affinity and hence lower activity of Rap1GAPs on Di-Ras1/2. Its corresponding residue in Rap1 (Glu62) is part of a negatively charged patch that attracts Arg240 and Arg286 of Rap1GAP1. Replacing it by Histidine might result in a reduction of affinity.
Interestingly, there is only one residue of Di-Ras proteins that is homologous to Rap2 but not Rap1. Gln92 in Di-Ras1 and Gln88 in Rap2 are replaced by Ser88 in Rap1 ( and B). Hence this residue might be the potential candidate that causes the jointly lower activity of Di-Ras proteins and Rap2 with Rap1GAP1 compared to Rap1.
The question remains, why the dual-specificity GAPs GAP1IP4BP and Rasal are inactive on Di-Ras proteins. Gln63 and Phe64 of Rap1, proven to be the most essential residues for activation by these GAPs,Citation18 are conserved in Di-Ras1/Di-Ras2. However, their presence is clearly not sufficient to allow stimulation of GTP hydrolysis (). Hence sequence differences that affect the affinity of Di-Ras1/Di-Ras2 towards dual specificity GAPs may be responsible for inactivity. Major prerequisite for dual-specificity GAP function is the remodelling of Rap's switch II. Mutation of Thr65 to Ala (Rap1) reduces GAP1IP4BP activity by 88%.Citation18 This residue is Pro69 in Di-Ras1/Di-Ras2, which most likely decreases switch II flexibility, thereby preventing reorientation of Gln63 and reducing the affinity between GAP and G protein.
Thus, no GAP-mediated cross-talk exists between the H-/K-/N-Ras signaling pathway and Di-Ras proteins as exists between Rap1 and Ras.
In agreement with previous studies,Citation3 nucleotide off-rate of Di-Ras proteins is considerably fast, compared to other Ras-family G proteins like H-Ras.Citation19 The result is a 100x lower nucleotide affinityCitation26 assuming similar nucleotide on-rates for both Di-Ras proteins.Citation26 Comparison with Ras supports an earlier notion that differences in affinities are mostly due to difference in dissociation rates. Furthermore, Di-Ras proteins are not regulated by RapGEFs, despite sharing GTPase activating proteins. Neither are they regulated by the RasGEF Sos. Comparison of Di-Ras1/2 with Rap1 in complex with Epac2 shows, that the majority of residues facing Epac2 are different in Di-Ras1/2 ( center, dark gray). Two large patches, the hydrophobic area around Leu56 and Tyr71 (Rap1) and the hydrophilic helix α3 including Asp95 (Rap1), have extensive interaction surfaces with Epac2 but show no sequence conservation in Di-Ras1/2 ( center).
Catalytic residues of G proteins, generally involved in GEF reactions are not conserved in Di-Ras proteins: The Ala residue in Walker B (e.g., Ala59 in Ras), normally displacing Mg2+, is a Thr in Di-Ras1/2. Remarkably, the residue absolutely essential for GEF reactions and conserved in many small G proteins, Glu62 in Ras, is mutated to His in Di-Ras1 and Di-Ras2. Thus, Di-Ras proteins are unlikely to be stimulated by Ras-, Rap-, Ranor Rho-GEFs that require the presence of the conserved Glu.Citation27,Citation28 Since the intrinsic GDP off-rate of Di-Ras proteins is several hundred fold higher and the ∼10 nM affinity is higher than that of Ras, it is not obvious whether nucleotide exchange is exclusively intrinsic or does require a GEF. This leaves the question, whether yet unidentified, specific Di-Ras-GEFs exist that function independently of Glu in the switch II region, similar to Arf- or Rab-GEFs. It might even be possible that these GEFs cross-talk with Di-Ras proteins.
Another distinction from the Ras subfamily is the inability of Di-Ras1/Di-Ras2 to bind common Ras-effectors. While Rap1 shows high affinity towards RalGDS(RA) () and moderate affinity to Raf(RBD),Citation29 neither of the Di-Ras proteins binds with significant affinity. Interaction surfaces between Ras-like proteins and RA/RB-domains usually include only switch I and adjacent residues ( and right). Switch I is highly conserved between Rap, Ras and Di-Ras proteins. However, those residues that convey effector affinity and specificity, K31 (Rap1) and D33 (Rap1), are modified in Di-Ras1/2 (), suggesting that RA/RBD-binding is inhibited.
This supports previous findings, in which Di-Ras1 fails to interact with Raf(RBD) in vivo and does not activate the MAPK pathway.Citation3 The suggestion that Di-Ras proteins interact with Arap1,Citation6 could not be confirmed in vitro either. Hence, Di-Ras proteins have a distinct, Ras- and Rap-independent subset of effectors that remain to be identified.
In summary, Di-Ras proteins share the typical properties of high nucleotide affinity and low GTPase activity with other members of the Ras family. The identification of Rap1GAPs as GTPase activating proteins for Di-Ras1/Di-Ras2 opens interesting questions as to the function of Di-Ras proteins, being downregulated by Rap1GAPs without sharing GEFs or effectors. Both Di-Ras1/Di-Ras2 and Rap1GAP were previously identified as tumorsuppressor proteins,Citation4,Citation30 suggesting that they might have joint impact on tumorigenesis.
Material and Methods
Constructs and cloning.
hDi-Ras1(1–170), hDi-Ras2(1–171), hRalGDS(RA)(789–885), hRaf(RBD)(51–131), hRheb(1–170), hTuberin(1538–1729), Rap1GAP1(75–415), Rap1GAP2(142–481), Rasal(full length), hSoscat (564–1049), C3G (830–1078), hEpac1ΔDEP (141–881) and mEpac2ΔDEP (280–993) were cloned into pGEX4T1 or pGEX4T3 vectors, NF1(1197–1528) was cloned into a modified pET-3d vectorCitation31 and GAP1IP4BP into pPROEX-HTa. The Escherichia coli strain BL21 (Codon+RIL) was used for protein expression. hRap1b (1–167) and hH-Ras (1–166) were cloned into ptac expression vectors and transformed into E. coli CK600K cells.
Protein purification.
Protein expression was induced at OD600 0.6 in TB media with 1 mM Isopropyl-β-D-1-thiogalactopyranoside (IPTG) (Sigma, I6758) and incubated over night at 20°C. GST-tagged proteins were purified using Glutathion-FF columns. Di-Ras1, Di-Ras2, RalGDS(RA), Raf(RBD), Rheb and Tuberin were lysed using a French Press and purified in buffer A (50 mM Tris/HCl pH 7.5, 50 mM NaCl (Rheb, Tuberin: 100 mM NaCl), 5 mM MgCl2, 5 mM β-Mercaptoethanol). After on-column proteolysis, using either TEV protease or Thrombin, the proteins were eluted and subjected to size exclusion chromatography (buffer A). Purified proteins were snap-frozen in liquid nitrogen and stored at −80°C. For polarisation experiments, RalGDS(RA) and Raf(RBD) were further purified as GST-fusion proteins. Rap1GAP1 and Rap1GAP2 were purified following the protocol already described for Rap1GAP1.Citation32 Rasal and GAP1IP4BP were purified as previously described in reference Citation18.
Protein nucleotide exchange and deliverance.
Exchange of Di-Ras1, Di-Ras2, Rap1, H-Ras and Rheb to mGDP (Biolog, M041) was done as described previously in reference 28, using 15 mM EDTA, 20–40x molar excess mGDP in buffer A (-MgCl2) and incubating over night at 4°C. Excess nucleotide was removed by size-exclusion chromatography on a S75 10/30 column. The nucleotide content was analysed using reversed-phase HPLC (Beckman Coulter).
For loading of Di-Ras1/Di-Ras2 with mGppNHp (Biolog, M047), purified proteins were incubated with 1 U/mg Calf Intestine Alkaline Phosphatase (Roche, 10108146001) at 4°C over night in the presence of 1.5x molar excess mGppNHp. Buffer was exchanged by size-exclusion chromatography.
Preparation of Guanosine/GMP bound Di-Ras1/Di-Ras2 was performed as described previously in reference 20: G proteins were incubated with bead-coupled alkaline phosphatase (Sigma, P0927) in presence of 1.5x molar excess of GppCH2p (Sigma, M3509). After incubation at 4°C over night, bead-coupled AP was removed by repeated centrifugation. Excess and protein-bound GppCH2p was hydrolysed to Guanosine/GMP, using 2 U/ml snake venom Phosphodiesterase (Sigma, P3134) that was subsequently inactivated by repeated freezing in liquid N2.
Hydrolysis assays.
50 µM GTP-prebound G protein and different concentrations of GAP were incubated at 25°C in buffer A (incl. 100 mM NaCl). At indicated time points 10 µl were removed, added to 15 µl H2O and snap-frozen in liquid N2. The nucleotide content was determined by reversed-phase HPLC after flash-thawing and protein denaturing at 95°C for 1 min. Relative GTP content, normalized to relative GTP content at time point 0 was plotted against time and rates determined using the program GraFit Ver. 5.
Nucleotide exchange and GEF assays.
For nucleotide exchange assays, 1 µM mGDP- or mGppNHp-bound Di-Ras1/Di-Ras2 was incubated with either 2 µM GEF or buffer in buffer A and the reaction was started with excess unlabelled GDP (Pharma Waldhof, 2252) or GppNHp (Sigma, G0635) at 25°C, respectively. Reactions of Di-Ras proteins with Epac1 or Epac2 contained 1 mM cAMP (Biolog, A001), additionally. Time-dependent fluorescence change was recorded using a Fluoromax 4 (Horiba Jobin Yvon) and analysed with GraFit Ver. 5.
Nucleotide affinity measurements.
For determination of nucleotide on-rates, 100 nM mGDP (mGppNHp) were mixed with different concentrations of Guanosine/GMP-bound Di-Ras2 in a Stopped flow (Applied Photophysics) at 25°C in buffer A. Pseudo first-order rates were determined and plotted against Di-Ras2 concentration (GraFit 5 Ver. 5). The on-rate was determined from the slope of linear regression.
Fluorescence polarisation assays.
1 µM mGppNHp-bound Di-Ras1/Di-Ras2 were incubated in buffer A at 25°C. Polarisation changes after step-wise addition of GST-Raf(RBD) or GST-RalGDS(RA) were monitored using a Fluoromax 4 (Horiba Jobin Yvon) spectrometer. Plotting of polarisation against effector concentration allowed determination of affinity constants (GraFit Ver. 5).
Figures and Tables
Figure 1 Intrinsic nucleotide exchange and determination of nucleotide affinity. (A) Nucleotide exchange reaction of 1 µM Di-Ras proteins, prebound to mGDP or mGppNHp. The protein-bound nucleotide is reacted with excess unlabelled nucleotide. (B) Plot of pseudo-first-order rate constants for the association of 100 nM mGDP with Guanosin/GMP-bound Di-Ras2. (C) Pseudo-first-order rate constants for the association of 100 nM mGppNHp with Guanosin/GMP-bound Di-Ras2.
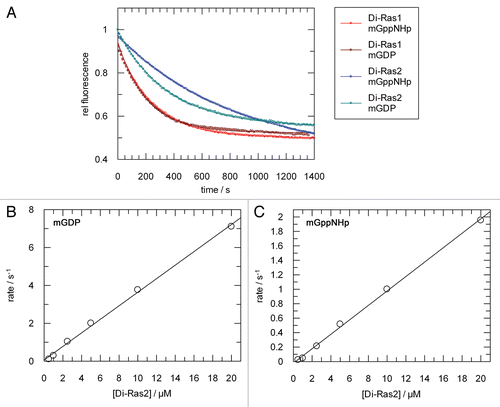
Figure 2 Ras- and Rap-GEFs are inactive on Di-Ras proteins. Nucleotide exchange activity of Di-Ras1 (intrinsic: red, GEF-catalysed: green), Di-Ras2 (intrinsic: magenta, GEF-catalysed: blue) and control G proteins (intrinsic: gray, GEF-catalysed: black). 1 µM of mGDP preloaded G protein were incubated with 2 µM of Sos (A), C3G (B), Epac1 (C) or Epac2 (D) and the reaction started by adding excess unlabelled nucleotide at time zero. Epac1 and Epac2 reactions were measured in the presence of 1 mM cAMP. The amount of mGDP loaded protein was set to 1.
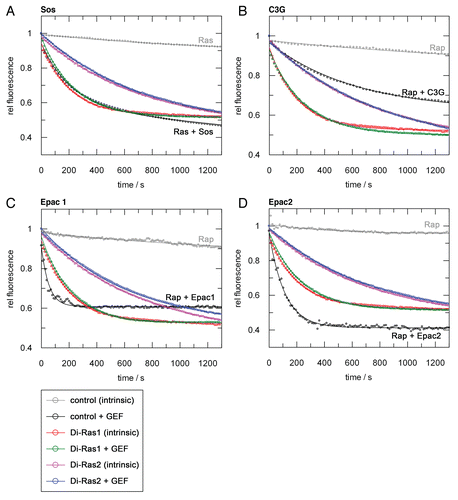
Figure 3 Nucleotide hydrolysis of Di-Ras proteins is stimulated by Rap1GAPs. Different concentrations of GAPs were tested for activity on GTP-bound Di-Ras1 (left, red) and Di-Ras2 (right, blue). Only Rap1GAP1 and Rap1GAP2 stimulated GTPase activity of Di-Ras proteins. Control reactions of GAPs with their cognate G proteins are shown in gray. 50 µM G protein were incubated with varying GAP concentrations and the decrease in relative GTP concentration was followed by HPLC. Concentration of G protein•GTP was set to 1 at time zero for all experiments.
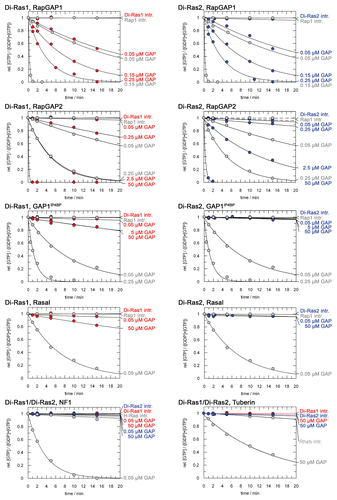
Figure 4 Low affinity interaction between Di-Ras1/Di-Ras2 and Ras-effectors. 1 µM mGppNHp-bound Di-Ras1 and Di-Ras2 were titrated with GST-Raf(RBD) (A) or GST-RalGDS(RA) (B). Complex formation was followed by determining fluorescence polarisation. GST-Raf(RBD) and GST-RalGDS(RA) showed very low affinity binding to Di-Ras1 or Di-Ras2. Only an approximate KD of 120 µM could be calculated for the Raf+Di-Ras1 interaction. The KDs for (RalGDS+Di-Ras1), (Raf+Di-Ras2) and (RalGDS+Di-Ras2) are above 200 µM. mGppNHp-bound H-Ras and mGppNHp-bound Rap1 were used as positive controls for GST-Raf(RBD) and GST-RalGDS(RA), respectively.
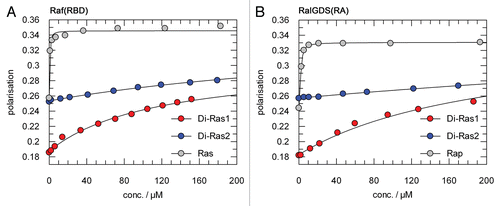
Figure 5 Sequence conservation of Ras-family G proteins. (A) Sequence alignment of selected Ras-family G proteins showing 100% conservation (red), 80% conservation (orange) and 60% conservation (yellow). The non-conserved catalytic residue (Rap: T61) is shown in green, the dual-specificity GAP catalytic residue (Rap: Q63) is highlighted in blue. The catalytic residue for GEF reactions (Rap: E62) is marked in purple. Numbers are according to Di-Ras1 sequence. Residues that are labelled in (B) are further indicated with cyan (Rap(s)-Raf), green (Rap1-Rap1GAP) and magenta (Rap1-Epac) lines. (B) Surface representations of Rap1 in complex with regulatory proteins. Between Rap1 and Di-Ras1/2 conserved residues that interact with the binding partners are shown in red. Not-conserved, but interacting residues are depicted in dark gray. Conserved, not-interacting residues are shown in lightorange. Left (pdb: 3BRW): Rap1 in complex with Rap1GAP1 (not shown). Middle (pdb: 3CF6): Rap1 in complex with Epac2 (not shown). Right (pdb: 1GUA): Rap(s) (Rap E30D, K31E) in complex with Raf(RBD) (not shown).
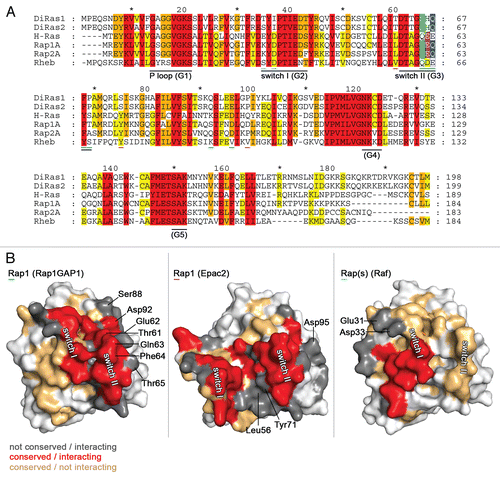
Table 1 Nucleotide on- and off-rates
Table 2 Observed rate constants for nucleotide hydrolysis of Di-Ras proteins
Acknowledgements
We thank Dorothee Vogt, Patricia Stege and Carolin Koerner for help in cloning, protein purification and general lab assistance. We thank Andrea Scrima for Rheb and Tuberin constructs and Shehab Ismail for fruitful discussions. R.G. was supported by the Fonds der Chemischen Industrie and B.S. by an Alexander v. Humboldt fellowship.
References
- Colicelli J. Human RAS superfamily proteins and related GTPases. Science's STKE 2004; 250:1 - 31
- Vetter IR, Wittinghofer A. Signal transduction—The guanine nucleotide-binding switch in three dimensions. Science 2001; 294:1299 - 1304
- Kontani K, Tada M, Ogawa T, Okai T, Saito K, Araki Y, et al. Di-Ras, a distinct subgroup of Ras family GTPases with unique biochemical properties. J Biol Chem 2002; 277:41070 - 41078
- Ellis CA, Vos MD, Howell H, Vallecorsa T, Fults DW, Clark GJ. Rig is a novel Ras-related protein and potential neural tumor suppressor. Proc Natl Acad Sci USA 2002; 99:9876 - 9881
- Ligon AH, Pershouse MA, Jasser SA, Yung WK, Steck PA. Identification of a novel gene product, RIG, that is downregulated in human glioblastoma. Oncogene 1997; 14:1075 - 1081
- Kiel C, Foglierini M, Kuemmerer N, Beltrao P, Serrano L. A genome-wide Ras-effector interaction network. J Mol Biol 2007; 370:1020 - 1032
- Bollag G, McCormick F. Differential regulation of rasGAP and neurofibromatosis gene product activities. Nature 1991; 351:576 - 579
- Ohba Y, Mochizuki N, Yamashita S, Chan AM, Schrader JW, Hattori S, et al. Regulatory proteins of R-Ras, TC21/R-Ras2 and M-Ras/R-Ras3. J Biol Chem 2000; 275:20020 - 20026
- Kurachi H, Wada Y, Tsukamoto N, Maeda M, Kubota H, Hattori M, et al. Human Spa-1 gene product selectively expressed in lymphoid tissues is a specific GTPase-activating protein for Rap1 and Rap2—segregate expression profiles from a Rap1GAP gene product. J Biol Chem 1997; 272:28081 - 28088
- Scrima A, Thomas C, Deaconescu D, Wittinghofer A. The Rap-RapGAP complex: GTP hydrolysis without catalytic glutamine and arginine residues. EMBO J 2008; 27:1145 - 1153
- Bos JL, Rehmann H, Wittinghofer A. GEFs and GAPs: Critical elements in the control of small G proteins [Review]. Cell 2007; 129:865 - 877
- Scheffzek K, Ahmadian MR, Kabsch W, Wiesmuller L, Lautwein A, Schmitz F, et al. The Ras-RasGAP complex: structural basis for GTPase activation and its loss in oncogenic Ras mutants. Science 1997; 277:333 - 338
- Rittinger K, Walker PA, Eccleston JF, Nurmahomed K, Owen D, Laue E, et al. Crystal structure of a small G protein in complex with the GTPase-activating protein RhoGAP. Nature 1997; 388:693 - 697
- Pan XJ, Eathiraj S, Munson M, Lambright DG. TBC-domain GAPs for Rab GTPases accelerate GTP hydrolysis by a dual-finger mechanism. Nature 2006; 442:303 - 306
- Ismail SA, Vetter IR, Sot B, Wittinghofer A. The structure of an Arf-ArfGAP complex reveals a Ca2+ regulatory mechanism. Cell 2010; 141:812 - 821
- Daumke O, Weyand M, Chakrabarti PP, Vetter IR, Wittinghofer A. The GTPase-activating protein Rap1GAP uses a catalytic asparagine. Nature 2004; 429:197 - 201
- Cullen PJ, Hsuan JJ, Truong O, Letcher AJ, Jackson TR, Dawson AP, et al. Identification of a specific Ins(1,3,4,5)P4-binding protein as a member of the GAP1 family. Nature 1995; 376:527 - 530
- Sot B, Kotting C, Deaconescu D, Suveyzdis Y, Gerwert K, Wittinghofer A. Unravelling the mechanism of duals-pecificity GAPs. EMBO J 2010; 29:1205 - 1214
- Lenzen C, Cool RH, Prinz H, Kuhlmann J, Wittinghofer A. Kinetic analysis by fluorescence of the interaction between Ras and the catalytic domain of the guanine nucleotide exchange factor Cdc25Mm. Biochemistry 1998; 19:7420 - 7430
- Eberth A, Ahmadian MR. In vitro GEF and GAP assays. Curr Protoc Cell Biol 2009; 14.9:14.9.1 - 14.9.25
- Hsu YC, Chern JJ, Cai Y, Liu MY, Choi KW. Drosophila TCTP is essential for growth and proliferation through regulation of dRheb GTPase. Nature 2007; 445:785 - 788
- Rehmann H, Bruning M, Berghaus C, Schwarten M, Kohler K, Stocker H, et al. Biochemical characterisation of TCTP questions its function as a guanine nucleotide exchange factor for Rheb. FEBS Lett 2008; 582:3005 - 3010
- Yaman E, Gasper R, Koerner C, Wittinghofer A, Tazebay UH. RasGEF1A and RasGEF1B are guanine nucleotide exchange factors that discriminate between Rap GTP-binding proteins and mediate Rap2-specific nucleotide exchange. FEBS J 2009; 276:4607 - 4616
- Ohba Y, Mochizuki N, Matsuo K, Yamashita S, Nakaya M, Hashimoto Y, et al. Rap2 as a slowly responding molecular switch in the Rap1 signaling cascade. Mol Cell Biol 2000; 20:6074 - 6083
- Chakrabarti PP, Daumke O, Suveyzdis Y, Kotting C, Gerwert K, Wittinghofer A. Insight into catalysis of a unique GTPase reaction by a combined biochemical and FTIR approach. J Mol Biol 2007; 367:983 - 995
- John J, Sohmen R, Feuerstein J, Linke R, Wittinghofer A, Goody RS. Kinetics of interaction of nucleotides with nucleotide-free H-ras p21. Biochemistry 1990; 29:6058 - 6065
- Boriack-Sjodin PA, Margarit SM, Barsagi D, Kuriyan J. The structural basis of the activation of ras by sos. Nature 1998; 394:337 - 343
- Gasper R, Thomas C, Ahmadian MR, Wittinghofer A. The role of the conserved switch II glutamate in guanine nucleotide exchange factor-mediated nucleotide exchange of GTP-binding proteins. J Mol Biol 2008; 379:51 - 63
- Wohlgemuth S, Kiel C, Kramer A, Serrano L, Wittinghofer F, Herrmann C. Recognizing and defining true Ras binding domains I: biochemical analysis. J Mol Biol 2005; 348:741 - 758
- Zhang LZ, Li CW, Mahmood R, van Golen K, Greenson J, Li GY, et al. Identification of a putative tumor suppressor gene Rap1GAP in pancreatic cancer. Cancer Res 2006; 66:898 - 906
- Ahmadian MR, Wiesmuller L, Lautwein A, Bischoff FR, Wittinghofer A. Structural differences in the minimal catalytic domains of the GTPase-activating proteins p120GAP and neurofibromin. J Biol Chem 1996; 271:16409 - 16415
- Daumke O, Wittinghofer A, Weyand M. Purification, crystallization and preliminary structural characterization of human Rap1GAP. Acta Crystallographica Section D-Biological Crystallography 2004; 60:752 - 754