Abstract
Cell migration is fundamental to development and many cell types have a migratory phase during embryonic development when tissues and body structures are forming. Cancer metastasis is in many ways thought to be analogous to embryonic development. Some of the mechanisms that tumor cells use to hijack the adult body are thought to derive from their abilities to reactivate embryonic signaling and motility pathways and thus enhance their growth and motility. Melanomas are notorious for their ability to become highly invasive and metastatic if not removed early. While adult melanin producing cells, melanocytes, have limited mobility, melanoblasts are highly motile cells that move through the dermis and epidermis during embryogenesis and could serve as a useful paradigm for some aspects of melanoma invasion and metastasis. Recent findings from our laboratory using ex-vivo imaging of mouse melanoblast migration in the epidermis provide the first insights into the role of Rac1 in developing mouse melanoblasts in vivo. Melanoblasts do not move as a collective group, or use an invasive or blebbing mode of migration as revealed by other in vivo systems, but rather they extend short and long dynamic pseudopodia and squeeze between epidermal keratinocytes using myosin motors. Melanoblasts can initiate short actin-based protrusions independently of Rac1. Rac1 is required to control the rate of formation of long actin-based protrusions for effective translocation in skin. Our results reveal a novel mode of in vivo migration controlled by Rac1 that is important for normal development and likely in melanoma.
Keywords: :
Introduction
Murine embryo melanoblasts are precursors of melanin producing melanocytes, which derived from neural crest cells during closure of the neural tube and arise around embryo day E8.5-E9.5. From E10.5, the melanoblasts begin to spread from the area adjacent to the neural tube, through the developing dermis and migrate along a dorsolateral pathway toward the face, ventral abdomen and the developing limbs. They emerge upward through the developing dermis and into the epidermis at around E13.5. By E16.5, melanoblasts home to developing hair follicles and reside within the hair bulb throughout the adult life.Citation1 This is different from the human, where melanocytes remain in both the skin and hair follicles. Developmental studies in mouse, chick and zebrafish have revealed much about the regulation of melanoblast growth, positioning and differentiation during embryogenesis.Citation2 Many murine mutants are available that have characteristic coat color patterns suggesting particular defectsCitation3,Citation4 (see also the useful website by the International Federation of Pigment Cell Societies http://www.espcr.org/micemut/). But there is still much to learn about how melanoblasts interact with their varying environments during development. Owing to improvements in imaging technology and careful development of ex-vivo systemsCitation5,Citation6 we have begun to gain a deeper molecular understanding of how these fascinating cells migrate and interact with the changing stromal environments that they encounter along the way. We predict that this analysis will lead to new insights into how melanoma cells can be so aggressively metastatic and hopefully pinpoint key targets against melanoma metastasis in the future.
Rac Drives Cell Migration in Different in Vivo Systems
Rac1, a Rho-family small GTPase, is key regulator of the actin cytoskeleton and cell signaling which controls the formation of lamellipodia in 2D cell culture.Citation7 Cells in culture can still migrate without Rac1, but their cytoskeleton is severely affected and they show growth defects.Citation8,Citation9 We used a melanoblast-specific Rac1 knockout mouse combined with expression of a GFP marker in melanoblasts and confocal imagining of skin explants to determine how melanoblasts use Rac1 when they migrate in embryo epidermis.Citation10 Rac1 loss in the melanocyte lineage resulted in severe coat color defects in mice (), suggesting both migration and proliferation defects (). Normal melanoblasts in embryo epidermis exhibit an elongated morphology with long protrusions traversing the spaces between adjacent keratinocytes (). These protrusions contain a microtubule core and several actin rich tips. In contrast, Rac1 depleted melanoblasts rarely have long protrusions and are more rounded. Despite these differences, most Rac1 depleted melanoblasts in skin explants could still migrate, but only slowly and they appear to utilize short stubby actin-dependent protrusions that form independently of the Rac-Scar/WAVE-Arp2/3 pathway ().
Figure 1. Rac1 is required for mouse melanoblast migration. (A) Melanoblast specific deletion of Rac1 results a hypopigmentation in C57BL/6 mice. (B) Ventral surface of β-galactosidase stained whole-mount DCT::LacZ control (left) and Rac1 f/f Tyr::Cre+/o (Right) embryos at E15.5. Yellow dotted line circles the area devoid of melanoblasts (C) Ex-vivo live cell imaging of F-actin dynamics in control (Ctr) or Rac1 depleted (Rac1 f/f) melanoblasts in embryo epidermis explant. Yellow arrows indicate protrusions. (D) Combined Z-stack images (1 μm depth interval) of control or Rac1 depleted melanoblasts in embryo epidermis explant treated with ROCK inhibitor Y27632.
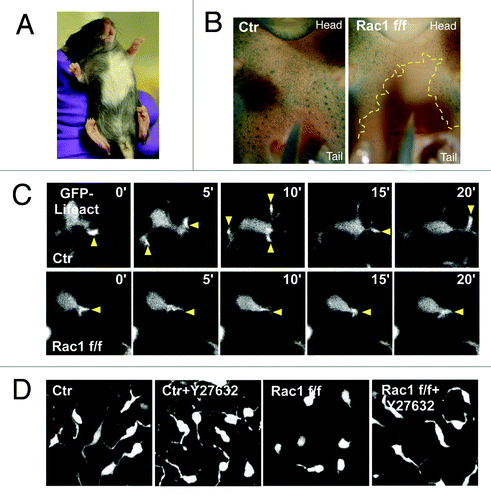
Unlike melanoblasts, when Rac is inhibited in Drosophila border cells,Citation11,Citation12 they display a total failure to migrate between the nurse cells. Similar to melanoblasts, Rac is required for extension of long protrusions in border cells, but border cells undergo polarized collective migration as a small cluster and this is absolutely dependent on Rac1. Mouse anterior visceral endoderm (AVE) cells are some of the earliest migrating cells in the embryo. During E5.5-E6.0 and they retain their tight junctions and adherence junctions and migrate as a sheet in the visceral endoderm to the developing anterior side of the embryo during body axis specification. Movement of these cells requires Rac1, similar to Drosophila border cells, but unlike melanoblasts. AVE cells extend filopodia and long cellular protrusions in the direction of migration but Rac1 depleted AVE cells lack protrusions and do not move.Citation13 Partial disruption of Nap1, a subunit of the Scar/WAVE complex,Citation14 in AVE cells revealed strong motility defects, but still some motility, indicating that at least part of the effects of Rac1 on motility in AVE cells is via Scar/WAVE complex and Arp2/3 complex.Citation15 A major difference between melanoblasts and the above systems is that melanoblasts migrate individually, rather than collectively and so might be more similar to cells in culture such as fibroblasts or macrophages, which can migrate without Rac in vitro.Citation8,Citation9,Citation16,Citation17 In addition, photoactivation of a Rac analog in one cell of the border cell cluster caused a dramatic response in the other cells.Citation18 This suggests that cells migrating collectively may sense direction as a group and inhibition of Rac may cause cell cluster to lose its ability to integrate their directional signaling. Thus, Rac inactivation could result in more severe migration defects in cells migrating collectively than in single cells. This also raises the possibility that during tumor cell invasion, Rac may be a more crucial regulator for certain types of tumors that mainly use collective invasionCitation19 (such as squamous-cell carcinoma) compared with tumors that mainly use single cell invasion (such as melanomaCitation20 (discussed below).
Like murine melanoblasts, Zebrafish germ cells also migrate as individual cells. During the two-somite stage, germ cells migrate close to somites 1–3 in response to chemokine CXCL12a/SDF-1a, which act as intermediate targets. From these intermediate targets, germ cells start migrating toward the final targets when embryos reach 8–10 somites. By 24 h post-fertilisation, germ cells coalesce with their somatic counterparts to form the gonads.Citation21 Unlike melanoblasts, germ cells extend spherical cellular protrusions termed blebs, which are driven by actomyosin cortex contraction and hydrostatic pressure. Inhibition of Rac activity in these cells resulted in a strong inhibition of actin-based protrusion (brush) formation at the cell leading edge, which lead to a loss of cell polarity resulting in random bleb formation, and failed migration to the developing gonad.Citation22 In contrast, our study indicated that Rac1 depleted melanoblasts could still home to hair follicles in the majority of the areas of the skin and fur during development, suggesting that Rac1 might not be essential for polarized migration. However, it is still not fully understood whether melanoblast migration is a signal-sensing process or driven by contact inhibition like neural crest cellsCitation23 or both.Citation24 Injection of blocking antibodies against KIT receptor tyrosine kinase into pregnant mice has shown that KIT signaling is necessary for melanoblast migration during movement from the dermis to the epidermis and subsequent localization to the follicle.Citation25 However, a more recent study suggests that KIT acts more like a chemokinetic rather than a chemotactic factor.Citation6 Thus, another mechanism is required to promote melanoblast migration to the correct location during early embryogenesis and our results demonstrate that Rac1 is not required for this process.
Migration is a complex phenomenon, involving many parameters, such as adhesion, protrusion and contractility, so whether or not a cell needs a particular pathway or set of pathways to migrate is likely to be highly dependent on the local environment. Interestingly, melanoma cells in vitro can exhibit a spectrum of morphologies during individual cell movement, ranging from elongated and mesenchymal to rounded and bleb-based.Citation26 Mesenchymal-type movement is characterized by an elongated cellular morphology, driven by activation of Rac and often is accompanied by extracellular proteolysis. In bleb-based movement, cells have a rounded morphology and often display high levels of Rho-kinase signaling to drive elevated levels of actomyosin contractility. Blebs result when the contractility in the cell exceeds the force required to attach the cytoskeleton to the plasma membrane. Tumor cells can apparently interconvert between these various invasion strategies and suppression of Rac activity often accompanies conversion to bleb-based motility.Citation26 In contrast to melanoma cells in culture, the short stubby protrusions in Rac1 null melanoblasts in vivo are distinct from blebs as they generally are thin and spiky and are rich in dynamic filamentous actin,Citation10 suggesting that loss of Rac1 in melanoblasts is not sufficient to convert them toward genuine bleb-based motility in vivo. Perhaps in normal melanoblasts Rac and Rho activity is tightly regulated at a steady activation state. However, in cancer cells, mutation of oncogenes or deletion of tumor suppressors may cause abnormal elevation of Rho GTPase signaling, which allows cells to adopt different modes of migration. For example, it has been shown that RasV12 and loss of p53 synergistically induce RhoA activity.Citation27 In the presence of p53, the RhoA negative regulator, p190 RhoGAP, is activated, resulting in a steady activation state of RhoA. Upon p53 loss, the p190 RhoGAP is less phosphorylated, leading to its inactivation and thus to increased activation of RhoA. This in turn results in increased cell motility and invasiveness.
Rac1 Controls the Formation of Long Protrusions in Mouse Melanoblast
Not only was Rac1 is not required for melanoblast migration in vivo, but it also wasn’t absolutely required for long pseudopod generation, as around 20% of Rac1 depleted melanoblasts still extended long actin-rich protrusions in embryo skin explants even when we inhibited myosin activity (). This raises a very interesting question: how are long protrusions generated in absence of Rac1? Our hypothesis is that these long protrusions may initiate from short stubby protrusions, which are driven by other small Rho GTPases, such as RhoA and Cdc42 (), which both localize to the cell leading edge.Citation28-Citation30 Downstream targets may be diaphanous related formins (DRF), which coordinate actin and microtubule assembly and dynamics.Citation31 Mena/VASP, is another potential target downstream of Cdc42, which can support extension of actin-based unbranched networks.Citation32,Citation33 Another possibility is that Scar/WAVE and Arp2/3 complex could generate pseudopodia in a Rac independent manner. This may depend on other signaling, such as via phosphorylationCitation34 or phospholipid or adaptor protein interactions.Citation14 There are likely to be multiple controls of Scar/WAVE activity and although in mammalian cells, Rac emerges as the major one, it is even possible that other Rho family GTPases could regulate the Scar/WAVE complex. However, neither of these mechanisms apparently induces long protrusions in normal conditions, where myosin-mediated contractility generates the force to retract protrusions. Our data therefore suggest that Rac1 efficiently promotes high frequency of long protrusion generation balanced by the force of myosin-II contractile activity. However, whether Rac1 dependent long protrusions derive directly from a distinct sort of short stubby precursor protrusion remains to be determined.
Figure 2. Molecular regulation of formation of long and short protrusions in melanoblasts migration in epidermis during mouse embryogenesis. (A) Rac1 positively regulates the frequency of initiation of long pseudopods, which promote migration speed and allow cells to change their direction of migration. Scar/WAVE and Arp2/3 complex drive actin assembly (red line) for long pseudopod extension, which also depends on microtubule dynamics (green line). Myosin contractility balances the extension of long pseudopods by effecting retraction and allowing force generation for movement through the complex 3D epidermal environment. (B) Short stubs are initiated in a Rac1 independent manner, which is also independent of Arp2/3 complex and microtubules. Short stubs may be initiated by another Rho GTPases such as RhoA or Cdc42 via DRFs or Mena/VASP family proteins. However, none of these alternative mechanisms can produce enough power to oppose myosin contractile activity to drive elongation of short protrusions into long protrusions. melanoblasts are painted with orange and surrounding keratinocytes are colored with gray.
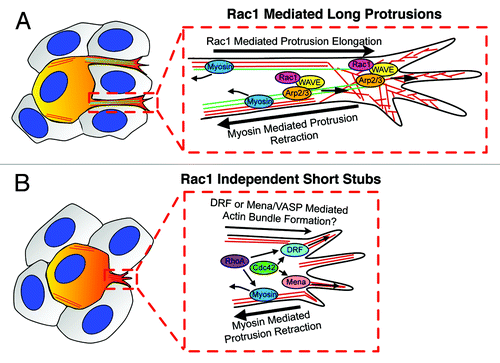
Summary and Future Perspective
Embryo skin explant cultures offer a unique opportunity to study mammalian cell motility in a physiological setting. Melanoblasts in explants are accessible to high resolution imaging, pharmacological and genetic manipulations. As this is a relatively new model system, many interesting questions remain to be answered. Our data demonstrated how melanoblasts migrate in epidermis while surrounded by keratinocytes. But it remains to be examined how melanoblasts migrate in the dermis before crossing into the epidermis. We found no requirement for metalloprotease activity for migration in epidermis, but perhaps protease activity will be more important in the dermis. The nature of the cell-cell and cell-matrix adhesions in dermis and epidermis are also only rudimentarily defined and need to be examined in greater molecular detail. By combining this melanoblast system with melanoma tumor models, there is a potential to study melanoblast motility in the presence of mutated oncogenes. These will help us gain more insights into the function of these mutations in cell motility in physiological setting and how melanoma cells might move during metastatic progression when they acquire certain gene mutations. Many challenges remain, such as how to image earlier stages of development to catch melanoblasts in the dermis and how to perform genetic manipulations on the melanoblasts and/or the keratinocytes yet maintain a physiological environment. Overall, this system offers promise for gaining insights into basic mechanisms of migration in vivo as well as mechanisms of migration that could be hijacked by invasive cancer cells and offers an interesting contrast to in vitro studies of melanoma cells.
Acknowledgments
A.L. and L.M.M. are funded by a CRUK core grant to L.M.M.. We also thank Lionel Larue and Sansom for many helpful discussions during the course of this work.
References
- Mayer TC. The migratory pathway of neural crest cells into the skin of mouse embryos. Dev Biol 1973; 34:39 - 46; http://dx.doi.org/10.1016/0012-1606(73)90337-0; PMID: 4595498
- Kelsh RN, Harris ML, Colanesi S, Erickson CA. Stripes and belly-spots -- a review of pigment cell morphogenesis in vertebrates. Semin Cell Dev Biol 2009; 20:90 - 104; http://dx.doi.org/10.1016/j.semcdb.2008.10.001; PMID: 18977309
- Baxter LL, Hou L, Loftus SK, Pavan WJ. Spotlight on spotted mice: a review of white spotting mouse mutants and associated human pigmentation disorders. Pigment Cell Res 2004; 17:215 - 24; http://dx.doi.org/10.1111/j.1600-0749.2004.00147.x; PMID: 15140066
- Bennett DC, Lamoreux ML. The color loci of mice--a genetic century. Pigment Cell Res 2003; 16:333 - 44; http://dx.doi.org/10.1034/j.1600-0749.2003.00067.x; PMID: 12859616
- Mort RL, Hay L, Jackson IJ. Ex vivo live imaging of melanoblast migration in embryonic mouse skin. Pigment Cell Melanoma Res 2010; 23:299 - 301; http://dx.doi.org/10.1111/j.1755-148X.2010.00669.x; PMID: 20067551
- Jordan SA, Jackson IJ. MGF (KIT ligand) is a chemokinetic factor for melanoblast migration into hair follicles. Dev Biol 2000; 225:424 - 36; http://dx.doi.org/10.1006/dbio.2000.9856; PMID: 10985860
- Heasman SJ, Ridley AJ. Mammalian Rho GTPases: new insights into their functions from in vivo studies. Nat Rev Mol Cell Biol 2008; 9:690 - 701; http://dx.doi.org/10.1038/nrm2476; PMID: 18719708
- Vidali L, Chen F, Cicchetti G, Ohta Y, Kwiatkowski DJ. Rac1-null mouse embryonic fibroblasts are motile and respond to platelet-derived growth factor. Mol Biol Cell 2006; 17:2377 - 90; http://dx.doi.org/10.1091/mbc.E05-10-0955; PMID: 16525021
- Guo F, Debidda M, Yang L, Williams DA, Zheng Y. Genetic deletion of Rac1 GTPase reveals its critical role in actin stress fiber formation and focal adhesion complex assembly. J Biol Chem 2006; 281:18652 - 9; http://dx.doi.org/10.1074/jbc.M603508200; PMID: 16698790
- Li A, Ma Y, Yu X, Mort RL, Lindsay CR, Stevenson D, et al. Rac1 drives melanoblast organization during mouse development by orchestrating pseudopod- driven motility and cell-cycle progression. Dev Cell 2011; 21:722 - 34; http://dx.doi.org/10.1016/j.devcel.2011.07.008; PMID: 21924960
- Murphy AM, Montell DJ. Cell type-specific roles for Cdc42, Rac, and RhoL in Drosophila oogenesis. J Cell Biol 1996; 133:617 - 30; http://dx.doi.org/10.1083/jcb.133.3.617; PMID: 8636236
- Duchek P, Rørth P. Guidance of cell migration by EGF receptor signaling during Drosophila oogenesis. Science 2001; 291:131 - 3; http://dx.doi.org/10.1126/science.291.5501.131; PMID: 11141565
- Migeotte I, Omelchenko T, Hall A, Anderson KV. Rac1-dependent collective cell migration is required for specification of the anterior-posterior body axis of the mouse. PLoS Biol 2010; 8:e1000442; http://dx.doi.org/10.1371/journal.pbio.1000442; PMID: 20689803
- Chen Z, Borek D, Padrick SB, Gomez TS, Metlagel Z, Ismail AM, et al. Structure and control of the actin regulatory WAVE complex. Nature 2010; 468:533 - 8; http://dx.doi.org/10.1038/nature09623; PMID: 21107423
- Rakeman AS, Anderson KV. Axis specification and morphogenesis in the mouse embryo require Nap1, a regulator of WAVE-mediated actin branching. Development 2006; 133:3075 - 83; http://dx.doi.org/10.1242/dev.02473; PMID: 16831833
- Wells CM, Walmsley M, Ooi S, Tybulewicz V, Ridley AJ. Rac1-deficient macrophages exhibit defects in cell spreading and membrane ruffling but not migration. J Cell Sci 2004; 117:1259 - 68; http://dx.doi.org/10.1242/jcs.00997; PMID: 14996945
- Wheeler AP, Wells CM, Smith SD, Vega FM, Henderson RB, Tybulewicz VL, et al. Rac1 and Rac2 regulate macrophage morphology but are not essential for migration. J Cell Sci 2006; 119:2749 - 57; http://dx.doi.org/10.1242/jcs.03024; PMID: 16772332
- Wang X, He L, Wu YI, Hahn KM, Montell DJ. Light-mediated activation reveals a key role for Rac in collective guidance of cell movement in vivo. Nat Cell Biol 2010; 12:591 - 597 http://www.nature.com/ncb/journal/v12/n6/suppinfo/ncb2061_S1.html
- Gaggioli C, et al. Fibroblast-led collective invasion of carcinoma cells with differing roles for RhoGTPases in leading and following cells. Nat Cell Biol 2007; 9:1392 - 1400 http://www.nature.com/ncb/journal/v9/n12/suppinfo/ncb1658_S1.html
- Sanz-Moreno V, Gadea G, Ahn J, Paterson H, Marra P, Pinner S, et al. Rac activation and inactivation control plasticity of tumor cell movement. Cell 2008; 135:510 - 23; http://dx.doi.org/10.1016/j.cell.2008.09.043; PMID: 18984162
- Kunwar PS, Siekhaus DE, Lehmann R. In vivo migration: a germ cell perspective. Annu Rev Cell Dev Biol 2006; 22:237 - 65; http://dx.doi.org/10.1146/annurev.cellbio.22.010305.103337; PMID: 16774460
- Kardash E, Reichman-Fried M, Maitre JL, Boldajipour B, Papusheva E, Messerschmidt EM, et al. A role for Rho GTPases and cell-cell adhesion in single-cell motility in vivo. Nat Cell Biol 2010; 12:47 - 53 http://www.nature.com/ncb/journal/v12/n1/suppinfo/ncb2003_S1.html
- Theveneau E, Marchant L, Kuriyama S, Gull M, Moepps B, Parsons M, et al. Collective chemotaxis requires contact-dependent cell polarity. Dev Cell 2010; 19:39 - 53; http://dx.doi.org/10.1016/j.devcel.2010.06.012; PMID: 20643349
- Luciani F, Champeval D, Herbette A, Denat L, Aylaj B, Martinozzi S, et al. Biological and mathematical modeling of melanocyte development. Development 2011; 138:3943 - 54; http://dx.doi.org/10.1242/dev.067447; PMID: 21862558
- Nishikawa S, Kusakabe M, Yoshinaga K, Ogawa M, Hayashi S, Kunisada T, et al. In utero manipulation of coat color formation by a monoclonal anti-c-kit antibody: two distinct waves of c-kit-dependency during melanocyte development. EMBO J 1991; 10:2111 - 8; PMID: 1712289
- Sanz-Moreno V, Marshall CJ. The plasticity of cytoskeletal dynamics underlying neoplastic cell migration. Curr Opin Cell Biol 2010; 22:690 - 6; http://dx.doi.org/10.1016/j.ceb.2010.08.020; PMID: 20829016
- Xia M, Land H. Tumor suppressor p53 restricts Ras stimulation of RhoA and cancer cell motility. Nat Struct Mol Biol 2007; 14:215 - 223
- Machacek M, et al. Coordination of Rho GTPase activities during cell protrusion. Nature 2009; 461:99 - 103
- Nalbant P, Hodgson L, Kraynov V, Toutchkine A, Hahn KM. Activation of endogenous Cdc42 visualized in living cells. Science 2004; 305:1615 - 9; http://dx.doi.org/10.1126/science.1100367; PMID: 15361624
- Pertz O, Hodgson L, Klemke RL, Hahn KM. Spatiotemporal dynamics of RhoA activity in migrating cells. Nature 2006; 440:1069 - 1072
- Olson M. F.. GTPase Signalling: New Functions for Diaphanous-Related Formins. Curr Biol 2003; 13:R360 - R362
- Krugmann S, Jordens I, Gevaert K, Driessens M, Vandekerckhove J, Hall A. Cdc42 induces filopodia by promoting the formation of an IRSp53:Mena complex. Curr Biol 2001; 11:1645 - 55; http://dx.doi.org/10.1016/S0960-9822(01)00506-1; PMID: 11696321
- Urban E, Jacob S, Nemethova M, Resch GP, Small JV. Electron tomography reveals unbranched networks of actin filaments in lamellipodia. Nat Cell Biol 2010; 12:429 - 35; http://dx.doi.org/10.1038/ncb2044; PMID: 20418872
- Lebensohn AM, Kirschner MW. Activation of the WAVE complex by coincident signals controls actin assembly. Mol Cell 2009; 36:512 - 24; http://dx.doi.org/10.1016/j.molcel.2009.10.024; PMID: 19917258