Abstract
Abstract The regulation of the small GTPases leading to their membrane localization has long been attributed to processing of their C-terminal CAAX box. As deregulation of many of these GTPases have been implicated in cancer and other disorders, prenylation and methylation of this CAAX box has been studied in depth as a possibility for drug targeting, but unfortunately, to date no drug has proved clinically beneficial. However, these GTPases also undergo other modifications that may be important for their regulation. Ubiquitination has long been demonstrated to regulate the fate of numerous cellular proteins and recently it has become apparent that many GTPases, along with their GAPs, GEFs and GDIs, undergo ubiquitination leading to a variety of fates such as re-localization or degradation. In this review we focus on the recent literature demonstrating that the regulation of small GTPases by ubiquitination, either directly or indirectly, plays a considerable role in controlling their function and that targeting these modifications could be important for disease treatment.
Introduction
Post-translational modifications, such as ubiquitination, phosphorylation and methylation have long been known to play crucial roles in regulating the function of proteins by altering activity, localization or stability. The Ras superfamily of GTPases undergo numerous modifications in their C-terminal region, and more specifically through their CAAX motif (where C is a Cysteine, A an aliphatic amino acid, and X any amino acid) before they can be appropriately localized and activated.Citation1,Citation2 Briefly, these modifications occur in sequential order and include (1) prenylation of the cysteine by a prenyltransferase, (2) cleavage of the tripeptide AAX motif by Ras converting enzyme 1 (RCE1) and (3) methylation of the now C-terminal cysteine by isoprenylcysteine carboxyl methyltransferase (ICMT). In addition, some Ras family members undergo an additional lipid modification termed either palmitoylation or S-acylation. These modifications result in a hydrophobic C-terminus, which anchors the GTPase at the membrane, awaiting activation by a growth factor. Some small GTPases, instead of possessing a CAAX motif, have a homologous C-terminal sequence (CC, CXC or CXXC) that can be similarly modified by some of these enzymes, for instance prenyltransferase.Citation3
The highly regulated processing of GTPases suggests that these numerous modifications are crucial for proper cellular function. Indeed, mutations can affect their localization or activation state leading to a variety of disorders.Citation4–Citation6 For example, constitutive activation of Ras and Rho family members has been implicated in cancer and so targeting the enzymes involved in their processing has become a potential target for therapeutic intervention. Unfortunately, to date, inhibitors targeting the initial steps such as prenylation have not been as successful as anticipated in the clinicCitation7,Citation8 and so additional targets are now under investigation.
Perhaps not surprisingly, these proteins also undergo ubiquitination, as discussed in detail below. Briefly, ubiquitin conjugation plays numerous intracellular roles such as localization, protein interactions, signaling and degradation. Therefore, targeting this process may represent an alternative approach.Citation4,Citation5 Additionally, deubiquitinating enzymes (DUBs), which remove ubiquitin from substrate proteins, may also be possible targets for therapeutic interventionCitation5 and, although not yet at the clinical stage, the development of DUB inhibitors is indeed underway.Citation4 Directly targeting the proteasome in order to prevent protein degradation has shown some promise,Citation9 although due to the broad specificity ‘off-targets’ effects are likely. However manipulating GTPase function by targeting ubiquitination specifically may be a way around this and produce a source of new therapeutic targets. Therefore, this review will focus on the roles of ubiquitination on GTPases and their modifiers and the possible implications in drug targeting. It must be recognized however, that many of the studies quoted have used biochemical and overexpression approaches and therefore any conclusions drawn regarding a role for ubiquitin in controlling GTPase function needs to be interpreted with caution.
Small GTPases
The activation and regulation of small GTPases have been extensively reviewed elsewhere (for example see ref. Citation10) and alterations in these GTPases often lead to disease. For instance, activating mutations of the Ras isoforms are found in approximately 30% of cancers making them among the most prevalent mutations, though the isoform incidence associated with disease varies.Citation11,Citation12 For example, K-Ras is mutated in 90% of pancreatic cancers, while N-Ras mutations are found in 20% of melanomas.Citation11,Citation12 H-Ras mutations are less commonly observed in cancer, but have been discovered in bladder cancer along with N-Ras mutations.Citation13,Citation14 However, germline mutations of H-Ras are associated with inherited disorders such as Costello Syndrome and Noonan Syndrome, and with mental retardation, cardiac problems and musculoskeletal defects, and can increase cancer susceptibility in these patients.Citation15 Although Rho mutations have not been detected in cancer, enhanced expression has frequently been observed and is often associated with metastatic disease.Citation6 The activity of these GTPase is highly regulated through their interaction with their numerous GAPs and so it is not surprising that dysregulated RhoGAP expression of these has also been observed in tumors.Citation16 Therefore, detailed study of these GTPases and mechanisms that govern their activation is critical to ascertain useful modalities for therapeutic intervention.
Ubiquitination and Deubiquitination
Ubiquitination is a reversible multistep process catalyzed by a number of enzymes, that entails the addition of a 79 amino acid tag to a substrate and while frequently this labels for destruction, it also may direct trafficking or alter function. Briefly, ubiquitin is initially activated by an E1 ubiquitin-activating enzyme in an energy dependent process requiring ATP and is subsequently transferred to an E2 ubiquitin conjugating enzyme. Finally, the ubiquitin is transferred to a lysine residue on the target protein with the assistance of a third enzyme termed the E3 ubiquitin ligase (). In some cases longer ubiquitin chains can be assembled by the recruitment of additional ligases known as E4s.Citation16 E3 ligases can be subclassified into two main families: HECT (homologous to E6-Ap C-terminal domain) and RING (really interesting new gene) E3s. HECT family E3 ligases, which include the Nedd4 (neuronal precursor cell expressed developmentally downregulated) ligases, interact directly with the ubiquitin attached to the E2.Citation16 RING E3s, on the other hand, are complexes that work by positioning the substrate and E2 enzyme in near proximity so that the E2 transfers the ubiquitin directly to the substrate.Citation17 There are seven lysines on ubiquitin (lysine 6, 11, 27, 29, 33, 48, 63) capable of forming polyubiquitin chains, with Lys48 and Lys63 being the best studied. Interestingly, lysine 48-linked polyubiquitination often targets a protein to be degraded by the proteasome, as with the NFκB regulating protein IκB.Citation18 Lys63 ubiquitination, on the other hand, has multiple roles such as relocalization of proteins like Akt,Citation19 receptor internalization of EGFRCitation20 and the regulation of DNA repair through PCNA or histone H2AX modification.Citation21
Interestingly, nature has evolved many ubiquitin-like proteins, such as Nedd8 and SUMO1, also capable of modifying and altering protein function. For example, neddylation is a process whereby the ubiquitin homolog Nedd8 is attached to a protein, specifically the Cullin family (Cul1, Cul2, Cul3, Cul4 and Cul5 in mammalian cells).Citation22,Citation23 Cullins interact with either ring finger proteins Rbx1/Roc1 or Rbx2/Roc2 forming an E3 ligase complex and targeting the substrate for proteasomal degradation. Additionally, sumoylation adds the 11 kda SUMO (small ubiquitin-like modifier) protein to a substrate also utilizing E3 ligases.Citation24 Additionally, a similar protein in prokaryotes termed pup has also been identified, which is specifically conjugated to proteasomal substrates in mycobacteria.Citation25 Thus ubiquitin and similar molecules are evolutionarily critical regulating protein half-life and function.
The removal of ubiquitin by DUBs alters the fate of the protein not only by rescuing it from degradation but also by altering its function, trafficking or activity.Citation26,Citation27 Although almost 100 human DUBs have been identified, this is a limited number compared with both the number of E3 ligases (>500) and ubiquitinated substrates suggesting that many of these enzymes must have multiple substrates. These DUBs can be divided into five main families based on their similarity within the catalytic domain.Citation28 Four of these classes are cysteine proteases, which represent most of the DUBs identified: ubiquitin-C-terminal hydrolases (UCHs), ubiquitin-specific processing proteases (USPs), ovarian tumor domain ubiquitin-aldehyde binding protein (Otubain/OTU), and the Machado-Joseph disease (MJD) families. The fifth class contains a JAMM (Jab/Rad1/MPN domain) motif and these are metalloproteases.
As previously mentioned ubiquitination and deubiquitination play significant roles in regulating cellular processes, such as proteasomal degradation, receptor internalization, cell cycle regulation, transcriptional control, signaling and trafficking (). One well studied example is the regulation of NFκB-pathway. Interestingly, many of the upstream proteins (such as TRAF2/6, RIP1/2, MALT1, BCL10, NEMO and RIG-IκB) are ubiquitinated leading to I ubiquitination, its proteasomal degradation and NFκB-translocation to the nucleus.Citation29 The deubiquitinating enzymes A20, Cezanne and CYLD block this process by removing ubiquitin from the upstream intermediates.Citation30,Citation31 As another example, after activation, EGFR undergoes ubiquitination,Citation32 is internalized into early endosomes and trafficked to the lysosome for degradation.Citation33 This process has been shown to be regulated by two DUBs: AMSH [associated molecule with Src homology 3 domain of STAM (signal-transducing adaptor molecule)] and USP8/UBPY. AMSH removes ubiquitin from EGFR redirecting it to the multivesicular body (MVB) and onwards for lysosomal degradation, while USP8 has been proposed to deubiquitinate the receptor at the latter stages of degradation.Citation34 In addition, many DUBs are essential for cell cycle progression: USP28 is involved in the DNA damage checkpoint,Citation35 CYLD (cyclindromatosis) and USP17 regulate G1/S progression.Citation36–Citation38 Interestingly, USP17 is also required for cell migration and its depletion disrupts Rho GTPase activation.Citation39 Thus, ubiquitination and deubiquitination regulate numerous cellular processes, and not surprisingly, as recent literature suggests may play a critical role in GTPase function.
Ubiquitination of the Ras and Rho Families
Several members of the Ras family have recently been reported to be modified by ubiquitin resulting in a change in their cellular localization and altered activity state(summarized in ). It is well documented that the Ras isoforms are localized to the plasma membrane where they undergo activation via GDP/GTP exchange leading to downstream signaling and proliferative gene expression (reviewed in ref. Citation40). Recently Jura et al. demonstrated that plasma membrane localized H-Ras and N-Ras underwent lysine-63 linked ubiquitination independent of their activation state and that this promoted their internalization into endosomesCitation41 (). Additionally, the Rab5 GEF Rabex-5 was shown to promote Ras ubiquitination in Drosophila and the resultant E3 ligase activity of Rabex-5 decreased the activation of downstream effectors and thus suppressed oncogenic Ras transformation.Citation42,Citation43 More recently, Rabex-5 has been identified in mammalian cells and has a similar role: Rabex-5 ubiquitinates H-Ras leading to its internalization into EEA1 positive early endosomes resulting in the quenching of downstream signaling.Citation44 As Rabex-5 activity would promote Rab5 mediated endocytosis at the plasma membrane,Citation45 the internalization of H-Ras was perhaps not surprising. They further demonstrated that the Rab5 GEF Rin1 was required for this modification. This built upon previous observations suggesting that H-Ras stimulated Rin1 activity and that this was required for EGFR internalization.Citation46 Thus the ubiquitination of H-Ras may be required for its internalization in situations where it is constitutively expressed. However, it is interesting to note that although the activation of ubiquitinated Ras was shown to be decreased when internalized into endosomes, activation of Ras has been observed previously in vesicles.Citation47 As many of the GEFs and effectors are location specific, this may account for the decrease in activity in endosomes. Additionally, there are numerous internalization pathways, and recently it was demonstrated that H-Ras can be internalized via a clathrin-independent route, whereas its main effector Raf-1 was localized to EEA1 positive early endosomes.Citation48 Interestingly, ubiquitinated H-Ras was observed in early endosomes, where the effector Raf1 also resides, without downstream activation, suggesting that the modification may prevent interaction with effectors.
Additionally, H-Ras was also shown to be polyubiquitinated in the presence of the SCF-β-TrCP E3 ligase complex with resultant H-Ras proteasomal degradation and reduced proliferation.Citation49 In a similar mechanism to H-Ras ubiquitination, β-catenin, important for adheren junctions (epithelial cell-cell interactions) and proliferation, also undergoes ubiquitination and proteasomal degraded by SCF-β-TrCP.Citation50 Thus, this E3 ligase appears to regulate cell growth by lowering both H-Ras and β-catenin levels. Taken together, the data suggests that ubiquitination of H-Ras can reduce its activity either by facilitating proteasomal degradation or endosomal sequestration. An interesting difference is that the degradation is permanent while the endosomal track may result in recycling back to the plasma membrane.
Although the other reports did not observe K-Ras ubiquitination in their experimental setups, recently, K-Ras was also demonstrated to be ubiquitinated, but in this case, the monoubiquitination enhanced GDP to GTP conversion (from 4 to 24% compared with non-ubiquitinated K-Ras) and downstream signaling.Citation51 One major difference between the studies was the cell types used and since K-Ras ubiquitination was not observed, it is possible that CHOK1 cells may lack the required E3 components. Interestingly, although each Ras isoform can be ubiquitinated at multiple lysine residues, in the case of both H-Ras and K-Ras lysine 147 seems to be the preferred site for ubiquitin conjugation.Citation52 Furthermore mutation of this lysine in a xenograph model that harbors oncogenic K-Ras resulted in reduced tumor volume suggesting that blocking K-Ras ubiquitination, on this specific residue, could be an important target to debilitate K-Ras activity in tumors.Citation53 Since constitutive ubiquitination would be detrimental to the cell, these studies also imply that there is most likely an endogenous DUB that would remove ubiquitin from these proteins blocking downstream signaling responses and thus providing a useful therapeutic target. Interestingly, the deubiquitinating enzyme USP17 was demonstrated to removed ubiquitin from RCE1 (Ras converting enzyme 1), an enzyme involved in Ras post-translational processing, blunting its activity and specifically decreasing H-Ras and N-Ras but not K-Ras membrane localization.Citation37,Citation52 Although this was not direct removal of ubiquitin from the GTPase, it does demonstrate that DUBs may play a key role in regulating Ras activity.
Not surprisingly, other members of the Ras GTPase subfamily are also modified by ubiquitination. Ras family members Rap1 and Rap2 play important roles in the maintenance of neurite extension and their disregulation has been associated with pathologies such as Parkinson, Alzheimer and autism.Citation53,Citation54 In the unpolarized neuron, Rap1 is localized to the tips of extending neurites, where it can regulate their ability to extend. Following a stimulus, the neuron becomes polarized, and in most of the neurites, Rap1 is ubiquitinated by the E3 ligase Smurf2 and the subsequent degradation inhibits neurite extension.Citation55,Citation56 However, in one of the neurites, Rap1 remains activated and can facilitate microtubule extension and so this neurite becomes the axon.Citation56,Citation57 In a similar manner, Rap2 is neddylated by the ubiquitin-like protein Nedd8, resulting in decreased Rap2 activity, blunted downstream signaling and dendrite extension.Citation58 Hence, in neurons regulation of these GTPases by ubiquitination and ubiquitin-like modifications are critical for maintenance of axonogenesis. Therefore targeting Rap ubiquitination could be an additional strategy for stimulating axon extension in disease such as Parkinson where neurite retraction is common.Citation59
Interestingly, the Smurf family of HECT E3 ligases have been demonstrated to ubiquitinate many of the GTPases. As previously mentioned, Smurf2 can ubiquitinate Rap1 in neuronsCitation56 and the related E3 ligase Smurf1 has been shown to be localized to cellular protrusions (filopodia and lamellipodia) along with the PAR6 effector PKCzeta in migrating cells following Rac1 and Cdc42 activation.Citation60 Inactive RhoA is ubiquitinated by this complex at the leading edge resulting in RhoA degradation and decreased motility, but Rac1 and Cdc42 ubiquitination by Smurf1 was not observed.Citation60,Citation61 This suggests that these cells would have increased motility and may then contribute to diseases where migration takes precedence such as metastasic disease or autoimmune conditions like Rheumatoid Arthritis. However, in MDA-MB-231 breast cancer cells, TGFβ induces Smurf1 ubiquitination by Smurf2 leading to Smurf1 degradation and reduced migration suggesting cell line specific roles.Citation62
Smurf1 also ubiquitinates and degrades regulators of GTPase function such as the Cdc42 GEF hPEM-2.Citation63 Additionally, treatment with the CNF1 toxin and proteasomal inhibition was demonstrated to increase RhoA, Rac and Cdc42 ubiquitination in bladder cells.Citation64,Citation65 These differences may be cell line specific since different GTPase ubiquitination levels displayed have been reported since overexpressing Smurf1 in cells with low endogenous levels of this E3 resulted in RhoA ubiquitination.Citation66 Thus, differential ubiquitination of these GTPase may be due to deficits of the required E3 ligase.
Interestingly, the Smurf E3 ligases appear to play a role primarily on those GTPases (RhoA and Rap1) that are involved in integrin adhesion. This may be in part through actin binding to the talin head domain (which is essential for integrin activation) resulting in a talin/Smurf1 complex, degradation of the talin head and consequently integrin stability.Citation67 Therefore, the Smurf family of E3 ligases play critical roles in the regulation of GTPases by inhibiting their activation and facilitating their degradation and further understanding regarding the specificity of these Smurfs could pose as potential drug discovery targets. Indeed, a number of groups have begun to explore this avenue and are using high throughput assays to uncover other substrates of Smurf1.Citation68
RhoA can also be ubiquitinated and degraded by a number of other E3 ligases, besides Smurf1, that also drive its degradation. The Cullin-RING ubiquitin E3 ligase (CRLs) complex (BACURD1/2, the Cul-3 scaffold protein, Rbx1, E2 ligase) can target inactive RhoA to the proteasome and inhibiting this can blunt cell migration.Citation69,Citation70 In addition another enzyme, the RING finger E3 ligase LNX (ligand of Numb protein X), initially identified as the E3 ligase for Numb, also associates with RhoC.Citation71 RhoC is normally found in the cytosol but can co-localize with LNX in the nucleus resulting in decreased AP-1 transcriptional activity.Citation71 Although in this case RhoC ubiquitination was not reported, the implication is that a number of E3 ligases can target a single GTPase, perhaps each with distinct functional outcomes. Additionally, as observed with the Ras GTPases, there is likely also location dependent ubiquitination for RhoA.
Understanding the influence of ubiquitin and ubiquitin-like modifications of certain GTPases has revealed unexpected intricacy. For instance, Rac1 can also be poly-ubiquitinated leading to its degradation by the proteasome, but a splice variant of Rac1 termed Rac1b, is not ubiquitinated and therefore is more stable.Citation72 As Rac1b is constitutively active and is often highly expressed in colorectal and breast tumors, this increased stability may facilitate increased tumorigenicity. However, the ubiquitination seems to specifically target active GTP-bound Rac1,Citation72,Citation73 suggesting that in polar regions of the cell, containing pools of active and inactive Rac1, active Rac1 would be preferentially ubiquitin-conjugated and thus reduced motility would ensue. Interestingly, although ubiquitination of Rac1 results in its degradation and would therefore decrease migration, sumoylation of Rac1 may be necessary for maintenance of Rac1 activation following HGF stimulation and the subsequent formation of membrane ruffles.Citation74 Hence, different modifications on the same protein can have opposing effects adding to the emerging complexity.
Not surprisingly then, Rac1 was also demonstrated to colocalize with the E3 ligase component cul-1 at the plasma membrane where it complexes with RelB and p100 regulating NFκB signaling.Citation75 Interestingly, cul-1 also associates with RhoA, Rac2, Rac3 and Cdc42 in the cell, and this interaction likely depends on the subcellular localization, since cul-1 and active Rac3 associate at the perinuclear regionCitation76 while the Rac1/cul-1 complex translocates to membrane ruffles.Citation77 Therefore targeting cul-1, and thereby inhibiting the activity of the E3 ligase complex, could regulate a number of GTPases.
Rab GTPase Ubiquitination
The Rab family of small GTPases consists of over 60 members that are predominantly involved in vesicular trafficking.Citation76 They are localized throughout the cell to distinct membrane compartments where they regulate the transport of various cargo proteins to and from the endoplasmic reticulum (ER), golgi apparatus, early and late endosomes, lysosome and plasma membrane, as well as many other cell type specific transport vesicles.Citation78 They bind to the membrane of their allotted compartment upon GTP binding and are involved in the formation and transport of a diverse range of vesicles through their interactions with downstream effector proteins.Citation78 Ubiquitination can act as an important signal to trigger vesicular trafficking, particularly in regards to receptor endocytosis, where ubiquitination can act as a signal for receptor internalization and transport to the lysosome for degradation.Citation79,Citation80 Indeed, the deubiquitinating enzymes USP8 and AMSH are thought to be important regulators of receptor fate, in particular whether the receptor is sent to the lysosome for degradation or recycled back to the plasma membrane.Citation81 However, to date there is no evidence that any of the Rabs are targeted for ubiquitination, although, in Xenopus, the homolog of mammalian Rab40 (XRab40) has been shown to act as part of an E3 ligase when complexed with XElonginC and Xcul5 at the golgi.Citation82 This ligase regulates the ubiquitination and localization of the small GTPase Rap2, which subsequently regulates the recruitment of disheveled (Dsh) to the plasma membrane and the activation of the non-canonical Wnt signaling pathway, all of which regulates Xenopus gastrulation.Citation82
Rab GTPase function, like that of other small GTPases, can be regulated by many different proteins including GEF, GAP and effector proteins and while the Rabs have not been shown to be ubiquitinated, a number of these regulatory and effector proteins have been shown to be regulated by ubiquitin (summarized in ). Rab5 is important for the formation and regulation of early endosomes and can be recruited by a number of different GEFs.Citation83 One of these, Rabex-5, has been shown to regulate the recruitment of Rab5 to the membrane either directly or indirectly, through an interaction with the Rab5 effector Rabaptin-5.Citation84 Interestingly, Rabex-5 possesses an A20-like zinc finger (ZnF) domain that binds ubiquitin and recently it has been demonstrated that the binding of this domain to ubiquitin is important for the recruitment of Rabex-5 to endosomes, and thus the activation and recruitment of Rab5.Citation85 In addition, this A-20 like zinc finger domain also exhibits E3 ligase activity and this has been shown to result in the self mono-ubiquitination of Rabex-5.Citation86 This mono-ubiquitination has been shown to be associated with a cytosolic distribution of Rabex-5 and it has been proposed that the mono-ubiquitination of Rabex-5 may sequester it to the cytosol and that its removal may be required to allow recruitment to the membrane through its binding to ubiquitinated cargo. In addition, as mentioned above, Rabex-5 can promote H-Ras ubiquitination and blunt its activity through endosomal sequestering.Citation42–Citation44 The role Rab5 plays in this is as yet unclear, but it is interesting to note that another Rab5 GEF, Rin1, which can interact with activated Ras, is required for the endosomal localization of H-Ras suggesting this in some way involves Rab5.Citation44 The yeast GEF Vps9p acts upon the yeast homolog of Rab5 (Ypt21p) and has also been demonstrated to be mono-ubiquitinated.Citation87 Vps9p possesses a CUE domain, which is capable of interacting with ubiquitin and is important for the endosomal transport of at least a subset of proteins, suggesting that Vps9p and Rabex-5 may be regulated in a similar fashion.
The Rab7 effector Rabring7 (BCA-2) has also been shown to act as an E3 ligase through its RING domain, resulting in self-ubiquitination, but not ubiquitination of Rab7. Rabring7 is also required for the ubiquitination of the EGF receptor and its subsequent lysosomal degradation as an inactive mutant of Rabring7 inhibits this process.Citation88 Rabring7 has also been shown to be involved in the internalization and trafficking of HIV-1 virions to the lysosome for degradationCitation89 suggesting this effector is important for transport to the lysosome.
GAP, GEF and GDI Ubiquitination
Due to intrinsic slowness of GDP/GTP conversion, GTPases requires the assistance of GEFs (guanine nucleotide exchange factors) and GAPs (GTPase activating proteins) while some also are regulated by GDIs (guanine nucleotide dissociation inhibitors). Although some of the GTPases have not been demonstrated to be ubiquitinated, they associate with ubiquitinated proteins and/or complex with E3 ligases that influence downstream signaling. In fact, GAPs, GEFs and RhoGDI are frequently targets of the ubiquitination machinery (). The RasGAPs, Ira2 (yeast) and neurofibromin gene NF1 (human) have been recently shown to be ubiquitinated.Citation90 Interestingly, the authors demonstrated that the GAP domain of NF1 is ubiquitinated by ETEA/UBXD8 leading to its degradation and blunting of the ability of NF1 to regulate Ras. In neurons, the Rap GAP spine-associated Rap GTPase (SPAR) is ubiquitinated by the E3 ligase complex SCF (β-TrCP) leading to its degradation and regulation of actin polymerization and neuron growth.Citation91,Citation92 Additionally, the Rac GAP α1-Chimaerin also undergoes polyubiquitination in neurons resulting in its degradation with resultant enhanced Rac activation. Although the precise roles of these pathways are unknown, the ubiquitination of these proteins may well play a key role in axon generation and neural signaling.
As the abundance of GEFs far outweighs the number of GAPs, it is then not surprisingly that the number of identified ubiquitinated GEFs exceeds that of GAPs. Analogous with the GTPases, ubiquitination can alter GEF function in numerous ways. For instance, the Cyclic nucleotide Ras GEF (NCrasGEF) can interact with both Ras and Rap1, is ubiquitinated and proteasomally degraded thus enhancing cell proliferation.Citation93,Citation94 NCrasGEF, also known as PDZ-GEF1/RA-GEF/nRapGEP, has also been demonstrated to regulate integrin activation and therefore cell migration,Citation95 and its murine knockout results in reduced angiogenesis due to inappropriate activation of Rap1.Citation96,Citation97 Additionally, as discussed previously, the E3 ligase Smurf1 can ubiquitinate the Cdc42 GEF hPEM-2.Citation63 The Cdc42 GEFs FGD1 and FGD3 also undergo ubiquitination by the SCF ligase complex (FWD1/β-TrCP), but with different functions since FGD1 stimulates, while FGD3 inhibits cell migration.Citation98,Citation99 Moreover, EGF stimulation can translocate FGD1 but not FGD3 to the plasma membrane, further suggesting that the ubiquitin modification plays a substrate specific role in the EGF signaling pathway.Citation100 Hence, the ubiquitination of Ras and Rho family GEFs and GAPs play a critical role in regulating the activation of GTPases, and since many of these GEFs and GAPs can regulate multiple GTPases, targeting one of these proteins may have additional effects. For example, by inhibiting Smurf1, Cdc42, Rac1 and RhoA are all affected, and therefore multiple downstream pathways are controlled. In fact an informative review on the importance of targeting these proteins has recently been published in reference Citation103.
Even though the number of RhoGDIs in the cell are few (only three have yet been identified in mammalian cells) (reviewed in ref. Citation104), to date only one has been demonstrated to be ubiquitinated. In a high throughput screen mining targets of the E3 ligase GRAIL in T-cells, RhoGDI was ubiquitinated.Citation101 This resulted specifically in reduced RhoA activity but did not affect Rac1 or Cdc42, despite the fact that RhoGDI is reported to interact with these GTPases as well.Citation102 These observations would be consistent with the hypothesis that targeting E3 ligases to manipulate GTPase function could provide a degree of specificity that has been lacking hitherto.
Targeting Ubiquitination/deubiquitination
Although Ras and other GTPases are mutated in many common cancers, GTPases are by no means the only proteins modified by ubiquitin that are deregulated in cancer or other pathologies. But development of novel drugs to control their ubiquitin-modified activity has the potential to prove clinically beneficial. A previous example, Bortezomib/Velcade that inhibits proteasome activity, has been approved for the treatment of multiple myeloma and is in clinical trials for other diseases such as non-small cell lung cancer, androgen-independent prostate carcinoma and non-Hodgkin's lymphomaCitation105,Citation106 and may also be useful in Ras or Rho mutant cancers. One concern with this broad spectrum approach is the potential side effects, and so more specific targeting of upstream proteins may prove a better option. E3 ligases are one possibility. Indeed, inhibitors for the HECT E3 ligase HDM2, key in p53 stability, have been identified.Citation107 Additionally, small molecule inhibitors targeting DUBs are also under investigation.Citation4 However, directly targeting the GTPases and their regulators, may prevent the side effects that have been observed with less specific drugs, like Velcade, and recent studies have unearthed a number of potential targets ().
Conclusions
Post-translational modifications of proteins such as GTPases have been under investigation for drug targeting since these proteins were first shown to play an important role in cancer and to undergo processing of their C-terminal region. More recently many functions of these proteins have been reported to be regulated by ubiquitination. In addition to prenylation, methlyation and palmitoylation, many members of the Ras superfamily as well as proteins involved in their regulation, also undergo ubiquitination (). The role of this modification can be quite distinct depending on the protein, E3 ligase involved, and the activation status of the target. Elucidating the ubiquitin types (mono vs. poly) and chains (K48 vs. K63, etc.) as well as all the players involved, will help to determine the role this modification has on GTPases. Additionally, little is known about which DUBs are involved. As disregulation of these GTPases are often times associated with disease and current modes of drug targeting have been unsuccessful, finding an alternate avenue would be opportunistic. Targeting the ubiquitination/deubiquitination may be an option. This is an exciting area with many potential drug targets, but clearly much further understanding is needed to address the likely specificity of targeting GTPase activity in this way.
Figures and Tables
Figure 1 Ubiquitination and its roles in protein function. Free ubiquitin is conjugated onto a substrate as described in the text. A monoubiquitinated protein can regulate receptor internalization, protein trafficking, DNA repair and transcriptional control. Polyubiquitinated proteins can undergo proteasomal degradation, kinase activation, trafficking and cell cycle regulation.
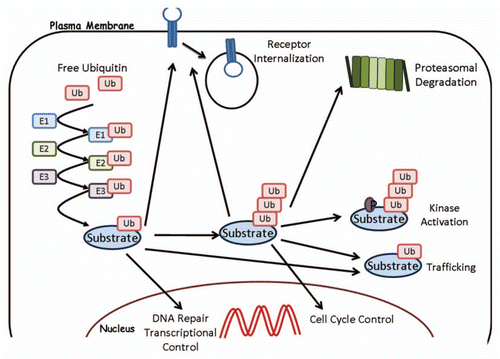
Figure 2 Ubiquitination of Ras GTPase leads to endosomal internalization. Following activation of H-Ras, Rabex-5 is ubiquitinated and Rab5 activated. These proteins, along with Rin1, are involved in Ras ubiquitination. This results in the formation of clathrin coated pits and the endocytosis of Ras into early endosomes, sequestering the protein away from the plasma membrane.
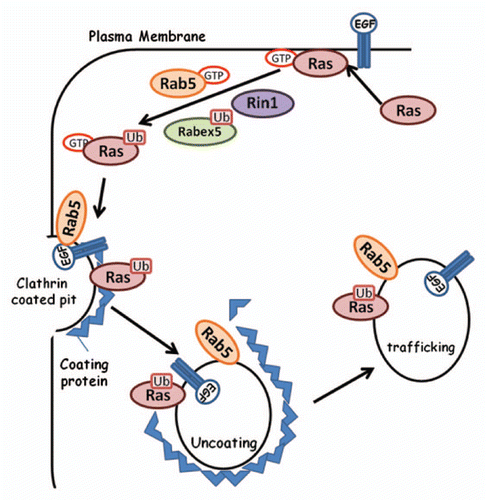
Figure 3 Possible Drug Targets of Ubiquitination/Deubiquitination. Regulation of GDP/GTP conversion is controlled by numerous proteins as described in the text. Many of these proteins (GAPs, GEFs and GDIs) undergo ubiquitination to direct their activity and that of the GTPases. Targeting these processes at critical stages (indicated by Rx) could therefore regulate GTPase activation and multiple disease states.
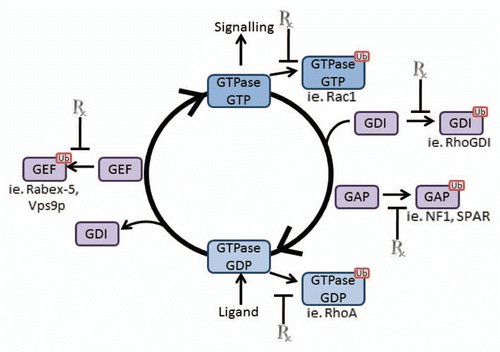
Table 1 Role of GTPase ubiquitination and ubiquitin-like modifications
Table 2 Ubiquitin and ubiquitin-like modifications of Rab GTPases and associated proteins
Table 3 Role of GAP, GEF and GDI Ubiquitination
References
- Lane KT, Beese LS. Thematic review series: Lipid post-translational modifications, structural biology of protein farnesyltransferase and geranylgeranyltransferase type I. J Lipid Res 2006; 47:681 - 699; PMID: 16477080; PMID: 10.1194/jlr.R600002-JLR200
- Roberts PJ, Mitin N, Keller PJ, Chenette EJ, Madigan JP, Currin RO, et al. Rho family GTPase modification and dependence on CAAX motif-signaled posttranslational modification. J Biol Chem 2008; 283:25150 - 25163; PMID: 18614539; http://dx.doi.org/10.1074/jbc.M800882200
- Pechlivanis M, Kuhlmann J. Hydrophobic modifications of ras proteins by isoprenoid groups and fatty acids—more than just membrane anchoring. Biochim Biophys Acta 2006; 1764:1914 - 1931
- Colland F. The therapeutic potential of deubiquitinating enzyme inhibitors. Biochem Soc Trans 2010; 38:137 - 143; PMID: 20074048; http://dx.doi.org/10.1042/BST0380137
- Goldenberg SJ, Marblestone JG, Mattern MR, Nicholson B. Strategies for the identification of ubiquitin ligase inhibitors. Biochem Soc Trans 2010; 38:132 - 136; PMID: 20074047; http://dx.doi.org/10.1042/BST0380132
- Narumiya S, Tanji M, Ishizaki T. Rho signaling, ROCK and mDia1, in transformation, metastasis and invasion. Cancer Metastasis Rev 2009; 28:65 - 76; PMID: 19160018; http://dx.doi.org/10.1007/s10555008-9170-7
- Sousa SF, Fernandes PA, Ramos MJ. Farnesyltransferase inhibitors: A detailed chemical view on an elusive biological problem. Curr Med Chem 2008; 15:1478 - 1492; PMID: 18537624; http://dx.doi.org/10.2174/092986708784638825
- Saxena N, Lahiri SS, Hambarde S, Tripathi RP. RAS: Target for cancer therapy. Cancer Invest 2008; 26:948 - 955; PMID: 18798058; http://dx.doi.org/10.1080/07357900802087275
- Genin E, Reboud-Ravaux M, Vidal J. Proteasome inhibitors: Recent advances and new perspectives in medicinal chemistry. Curr Top Med Chem 2010; 10:232 - 256; PMID: 20166955; http://dx.doi.org/10.2174/156802610790725515
- Cox AD, Der CJ. Ras history: The saga continues. Small GTPases 2010; 1:2 - 27; PMID: 21686117; http://dx.doi.org/10.4161/sgtp.1.1.12178
- Schubbert S, Shannon K, Bollag G. Hyperactive ras in developmental disorders and cancer. Nat Rev Cancer 2007; 7:295 - 308; PMID: 17384584; http://dx.doi.org/10.1038/nrc2109
- Whitwam T, Vanbrocklin MW, Russo ME, Haak PT, Bilgili D, Resau JH, et al. Differential oncogenic potential of activated RAS isoforms in melanocytes. Oncogene 2007; 26:4563 - 4570; PMID: 17297468; http://dx.doi.org/10.1038/sj.onc.1210239
- Kompier LC, Lurkin I, van der Aa MN, van Rhijn BW, van der Kwast TH, Zwarthoff EC. FGFR3, HRAS, KRAS, NRAS and PIK3CA mutations in bladder cancer and their potential as biomarkers for surveillance and therapy. PLoS ONE 2010; 5:13821; PMID: 21072204; http://dx.doi.org/10.1371/journal.pone.0013821
- Jebar AH, Hurst CD, Tomlinson DC, Johnston C, Taylor CF, Knowles MA. FGFR3 and ras gene mutations are mutually exclusive genetic events in urothelial cell carcinoma. Oncogene 2005; 24:5218 - 5225; PMID: 15897885; http://dx.doi.org/10.1038/sj.onc.1208705
- Tidyman WE, Rauen KA. Noonan, costello and cardio-facio-cutaneous syndromes: Dysregulation of the ras-MAPK pathway. Expert Rev Mol Med 2008; 10:37; PMID: 19063751; http://dx.doi.org/10.1017/S1462399408000902
- Heckman-Stoddard BM, Vargo-Gogola T, McHenry PR, Jiang V, Herrick MP, Hilsenbeck SG, et al. Haploinsufficiency for p190B RhoGAP inhibits MMTV-neu tumor progression. Breast Cancer Res 2009; 11:61; PMID: 19703301; http://dx.doi.org/10.1186/bcr2352
- Deshaies RJ, Joazeiro CA. RING domain E3 ubiquitin ligases. Annu Rev Biochem 2009; 78:399 - 434; PMID: 19489725; http://dx.doi.org/10.1146/annurev.biochem.78.101807.093809
- Wu CJ, Conze DB, Li T, Srinivasula SM, Ashwell JD. Sensing of lys 63-linked polyubiquitination by NEMO is a key event in NFkappaB activation [corrected]. Nat Cell Biol 2006; 8:398 - 406; PMID: 16547522; http://dx.doi.org/10.1038/ncb1384
- Yang WL, Wang J, Chan CH, Lee SW, Campos AD, Lamothe B, et al. The E3 ligase TRAF6 regulates akt ubiquitination and activation. Science 2009; 325:1134 - 1138; PMID: 19713527; http://dx.doi.org/10.1126/science.1175065
- Huang F, Kirkpatrick D, Jiang X, Gygi S, Sorkin A. Differential regulation of EGF receptor internalization and degradation by multiubiquitination within the kinase domain. Mol Cell 2006; 21:737 - 748; PMID: 16543144; http://dx.doi.org/10.1016/j.molcel.2006.02.018
- Hofmann K. Ubiquitin-binding domains and their role in the DNA damage response. DNA Repair (Amst) 2009; 8:544 - 556; PMID: 19213613; http://dx.doi.org/10.1016/j.dnarep.2009.01.003
- Rabut G, Peter M. Function and regulation of protein neddylation. 'protein modifications: Beyond the usual suspects' review series. EMBO Rep 2008; 9:969 - 976; PMID: 18802447; http://dx.doi.org/10.1038/embor.2008.183
- Xirodimas DP. Novel substrates and functions for the ubiquitin-like molecule NEDD8. Biochem Soc Trans 2008; 36:802 - 806; PMID: 18793140; http://dx.doi.org/10.1042/BST0360802
- Wilkinson KA, Henley JM. Mechanisms, regulation and consequences of protein SUMOylation. Biochem J 2010; 428:133 - 145; PMID: 20462400; http://dx.doi.org/10.1042/BJ20100158
- Burns KE, Darwin KH. Pupylation versus ubiquitylation: Tagging for proteasome-dependent degradation. Cell Microbiol 2010; 12:424 - 431; PMID: 20109157; http://dx.doi.org/10.1111/j.1462-5822.2010.01447.x
- Katz EJ, Isasa M, Crosas B. A new map to understand deubiquitination. Biochem Soc Trans 2010; 38:21 - 28
- Ventii KH, Devi NS, Friedrich KL, Chernova TA, Tighiouart M, Van Meir EG, et al. BRCA1-associated protein-1 is a tumor suppressor that requires deubiquitinating activity and nuclear localization. Cancer Res 2008; 68:6953 - 6962; PMID: 18757409; http://dx.doi.org/10.1158/0008-5472.CAN-08-0365
- Reyes-Turcu FE, Ventii KH, Wilkinson KD. Regulation and cellular roles of ubiquitin-specific deubiquitinating enzymes. Annu Rev Biochem 2009; 78:363 - 397; PMID: 19489724; http://dx.doi.org/10.1146/annurev.biochem.78.082307.091526
- Skaug B, Jiang X, Chen ZJ. The role of ubiquitin in NFkappaB regulatory pathways. Annu Rev Biochem 2009; 78:769 - 796; PMID: 19489733; http://dx.doi.org/10.1146/annurev.biochem.78.070907.102750
- Enesa K, Zakkar M, Chaudhury H, Luong le A, Rawlinson L, Mason JC, et al. NFkappaB suppression by the deubiquitinating enzyme cezanne: A novel negative feedback loop in pro-inflammatory signaling. J Biol Chem 2008; 283:7036 - 7045; PMID: 18178551; http://dx.doi.org/10.1074/jbc.M708690200
- Harhaj EW, Dixit VM. Deubiquitinases in the regulation of NFkappaB signaling. Cell Res 2011; 21:22 - 39; PMID: 21119682; http://dx.doi.org/10.1038/cr.2010.166
- Levkowitz G, Waterman H, Zamir E, Kam Z, Oved S, Langdon WY, et al. c-Cbl/Sli-1 regulates endocytic sorting and ubiquitination of the epidermal growth factor receptor. Genes Dev 1998; 12:3663 - 3674; PMID: 9851973; http://dx.doi.org/10.1101/gad.12.23.3663
- Urbé S, Sachse M, Row PE, Preisinger C, Barr FA, Strous G, et al. The UIM domain of hrs couples receptor sorting to vesicle formation. J Cell Sci 2003; 116:4169 - 4179; PMID: 12953068; http://dx.doi.org/10.1242/jcs.00723
- Williams RL, Urbe S. The emerging shape of the ESCRT machinery. Nat Rev Mol Cell Biol 2007; 8:355 - 368; PMID: 17450176; http://dx.doi.org/10.1038/nrm2162
- Popov N, Herold S, Llamazares M, Schulein C, Eilers M. Fbw7 and Usp28 regulate myc protein stability in response to DNA damage. Cell Cycle 2007; 6:2327 - 2331; PMID: 17873522; http://dx.doi.org/10.4161/cc.6.19.4804
- Massoumi R, Chmielarska K, Hennecke K, Pfeifer A, Fassler R. Cyld inhibits tumor cell proliferation by blocking bcl-3-dependent NFkappaB signaling. Cell 2006; 125:665 - 677; PMID: 16713561; http://dx.doi.org/10.1016/j.cell.2006.03.041
- Burrows JF, Kelvin AA, McFarlane C, Burden RE, McGrattan MJ, De la Vega M, et al. USP17 regulates ras activation and cell proliferation by blocking RCE1 activity. J Biol Chem 2009; 284:9587 - 9595; PMID: 19188362; http://dx.doi.org/10.1074/jbc.M807216200
- McFarlane C, Kelvin AA, de la Vega M, Govender U, Scott CJ, Burrows JF, et al. The deubiquitinating enzyme USP17 is highly expressed in tumor biopsies, is cell cycle regulated, and is required for G1-S progression. Cancer Res 2010; 70:3329 - 3339; PMID: 20388806; http://dx.doi.org/10.1158/0008-5472.CAN-09-4152
- de la Vega M, Kelvin AA, Dunican DJ, McFarlane C, Burrows JF, Jaworski J, et al. The deubiquitinating enzyme USP17 is essential for GTPase subcellular localization and cell motility. Nat Commun 2011; 2:259; PMID: 21448158; http://dx.doi.org/10.1038/ncomms1243
- Omerovic J, Prior IA. Compartmentalized signalling: Ras proteins and signalling nanoclusters. FEBS J 2009; 276:1817 - 1825; PMID: 19243428; http://dx.doi.org/10.1111/j.1742-4658.2009.06928.x
- Jura N, Scotto-Lavino E, Sobczyk A, Bar-Sagi D. Differential modification of ras proteins by ubiquitination. Mol Cell 2006; 21:679 - 687; PMID: 16507365; http://dx.doi.org/10.1016/j.molcel.2006.02.011
- Yan H, Jahanshahi M, Horvath EA, Liu HY, Pfleger CM. Rabex-5 ubiquitin ligase activity restricts ras signaling to establish pathway homeostasis in drosophila. Curr Biol 2010; 20:1378 - 1382; PMID: 20655224; http://dx.doi.org/10.1016/j.cub.2010.06.058
- Tam SY, Kalesnikoff J, Nakae S, Tsai M, Galli SJ. RabGEF1, a negative regulator of ras signalling, mast cell activation and skin inflammation. Novartis Found Symp 2005; 271:115 - 124
- Xu L, Lubkov V, Taylor LJ, Bar-Sagi D. Feedback regulation of ras signaling by rabex-5-mediated ubiquitination. Curr Biol 2010; 20:1372 - 1377; PMID: 20655225; http://dx.doi.org/10.1016/j.cub.2010.06.051
- Horiuchi H, Lippe R, McBride HM, Rubino M, Woodman P, Stenmark H, et al. A novel Rab5 GDP/ GTP exchange factor complexed to rabaptin-5 links nucleotide exchange to effector recruitment and function. Cell 1997; 90:1149 - 1159; PMID: 9323142; http://dx.doi.org/10.1016/S0092-8674(00)80380-3
- Tall GG, Barbieri M, Stahl P, Horazdovsky. Ras-activate endocytosis is mediated by the Rab5 guanine nucleotide exchange activity of RIN1. Dev Cell 2001; 1:73 - 82; PMID: 11703925; http://dx.doi.org/10.1016/S1534-5807(01)00008-9
- Fehrenbacher N, Bar-Sagi D, Philips M. Ras/MAPK signaling from endomembranes. Mol Oncol 2009; 3:297 - 307; PMID: 19615955; http://dx.doi.org/10.1016/j.molonc.2009.06.004
- McKay J, Wang X, Ding J, Buss JE, Ambrosio L. H-ras resides on clathrin-independent ARF6 vesicles that harbor little RAF-1, but not on clathrin-dependent endosomes. Biochim Biophys Acta 2011; 1813:298 - 307; PMID: 21145357; http://dx.doi.org/10.1016/j.bbamcr.2010.11.019
- Kim SE, Yoon JY, Jeong WJ, Jeon SH, Park Y, Yoon JB, et al. H-ras is degraded by Wnt/beta-catenin signaling via beta-TrCP-mediated polyubiquitylation. J Cell Sci 2009; 122:842 - 848; PMID: 19240121; http://dx.doi.org/10.1242/jcs.040493
- Su Y, Fu C, Ishikawa S, Stella A, Kojima M, Shitoh K, et al. APC is essential for targeting phosphorylated beta-catenin to the SCFbeta-TrCP ubiquitin ligase. Mol Cell 2008; 32:652 - 661; PMID: 19061640; http://dx.doi.org/10.1016/j.molcel.2008.10.023
- Sasaki AT, Carracedo A, Locasale JW, Anastasiou D, Takeuchi K, Kahoud ER, et al. Ubiquitination of k-ras enhances activation and facilitates binding to select downstream effectors. Sci Signal 2011; 4:13; PMID: 21386094; http://dx.doi.org/10.1126/scisignal.2001518.
- de la Vega M, Burrows JF, McFarlane C, Govender U, Scott CJ, Johnston JA. The deubiquitinating enzyme USP17 blocks N-ras membrane trafficking and activation but leaves K-ras unaffected. J Biol Chem 2010; 285:12028 - 12036; PMID: 20147298; http://dx.doi.org/10.1074/jbc.M109.081448
- Woolfrey KM, Srivastava DP, Photowala H, Yamashita M, Barbolina MV, Cahill ME, et al. Epac2 induces synapse remodeling and depression and its disease-associated forms alter spines. Nat Neurosci 2009; 12:1275 - 1284; PMID: 19734897; http://dx.doi.org/10.1038/nn.2386
- Hall A, Lalli G. Rho and ras GTPases in axon growth, guidance and branching. Cold Spring Harb Perspect Biol 2010; 2:1818; PMID: 20182621; http://dx.doi.org/10.1101/cshperspect.a001818
- Schwamborn JC, Puschel AW. The sequential activity of the GTPases Rap1B and Cdc42 determines neuronal polarity. Nat Neurosci 2004; 7:923 - 929; PMID: 15286792; http://dx.doi.org/10.1038/nn1295
- Schwamborn JC, Muller M, Becker AH, Puschel AW. Ubiquitination of the GTPase Rap1B by the ubiquitin ligase Smurf2 is required for the establishment of neuronal polarity. EMBO J 2007; 26:1410 - 1422; PMID: 17318188; http://dx.doi.org/10.1038/sj.emboj.7601580
- Schwamborn JC, Khazaei MR, Puschel AW. The interaction of mPar3 with the ubiquitin ligase Smurf2 is required for the establishment of neuronal polarity. J Biol Chem 2007; 282:35259 - 35268; PMID: 17906294; http://dx.doi.org/10.1074/jbc.M703438200
- Kawabe H, Neeb A, Dimova K, Young S Jr, Takeda M, Katsurabayashi S, et al. Regulation of Rap2A by the ubiquitin ligase Nedd4-1 controls neurite development. Neuron 2010; 65:358 - 372; PMID: 20159449; http://dx.doi.org/10.1016/j.neuron.2010.01.007
- Chan D, Citro A, Cordy JM, Shen GC, Wolozin B. Rac1 protein rescues neurite retraction caused by G2019S leucine-rich repeat kinase 2 (LRRK2). J Biol Chem 2011; 286:16140 - 16149; PMID: 21454543; http://dx.doi.org/10.1074/jbc.M111.234005
- Wang HR, Zhang Y, Ozdamar B, Ogunjimi AA, Alexandrova E, Thomsen GH, et al. Regulation of cell polarity and protrusion formation by targeting RhoA for degradation. Science 2003; 302:1775 - 1779; PMID: 14657501; http://dx.doi.org/10.1126/science.1090772
- Crose LE, Hilder TL, Sciaky N, Johnson GL. Cerebral cavernous malformation 2 protein promotes smad ubiquitin regulatory factor 1-mediated RhoA degradation in endothelial cells. J Biol Chem 2009; 284:13301 - 13305; PMID: 19318350; http://dx.doi.org/10.1074/jbc.C900009200
- Fukunaga E, Inoue Y, Komiya S, Horiguchi K, Goto K, Saitoh M, et al. Smurf2 induces ubiquitin-dependent degradation of Smurf1 to prevent migration of breast cancer cells. J Biol Chem 2008; 283:35660 - 35667; PMID: 18927080; http://dx.doi.org/10.1074/jbc.M710496200
- Yamaguchi K, Ohara O, Ando A, Nagase T. Smurf1 directly targets hPEM-2, a GEF for Cdc42, via a novel combination of protein interaction modules in the ubiquitin-proteasome pathway. Biol Chem 2008; 389:405 - 413; PMID: 18208356; http://dx.doi.org/10.1515/BC.2008.036
- Doye A, Mettouchi A, Bossis G, Clement R, Buisson-Touati C, Flatau G, et al. CNF1 exploits the ubiquitin-proteasome machinery to restrict rho GTPase activation for bacterial host cell invasion. Cell 2002; 111:553 - 564; PMID: 12437928; http://dx.doi.org/10.1016/S00928674(02)01132-7
- Doye A, Boyer L, Mettouchi A, Lemichez E. Ubiquitinmediated proteasomal degradation of rho proteins by the CNF1 toxin. Methods Enzymol 2006; 406:447 - 456; PMID: 16472677; http://dx.doi.org/10.1016/S00766879(06)06033-2
- Boyer L, Turchi L, Desnues B, Doye A, Ponzio G, Mege JL, et al. CNF1-induced ubiquitylation and proteasome destruction of activated RhoA is impaired in Smurf1-/- cells. Mol Biol Cell 2006; 17:2489 - 2497; PMID: 16540523; http://dx.doi.org/10.1091/mbc.E05-09-0876
- Huang CY, Lee CY, Chen MY, Yang WH, Chen YH, Chang CH, et al. Stromal cell-derived factor-1/CXCR4 enhanced motility of human osteosarcoma cells involves MEK1/2, ERK and NFkappaB-dependent pathways. J Cell Physiol 2009; 221:204 - 212; PMID: 19496172; http://dx.doi.org/10.1002/jcp.21846
- Andrews PS, Schneider S, Yang E, Michaels M, Chen H, Tang J, et al. Identification of substrates of SMURF1 ubiquitin ligase activity utilizing protein microarrays. Assay Drug Dev Technol 2010; 8:471 - 487; PMID: 20804422; http://dx.doi.org/10.1089/adt.2009.0264
- Chen Y, Yang Z, Meng M, Zhao Y, Dong N, Yan H, et al. Cullin mediates degradation of RhoA through evolutionarily conserved BTB adaptors to control actin cytoskeleton structure and cell movement. Mol Cell 2009; 35:841 - 855; PMID: 19782033; http://dx.doi.org/10.1016/j.molcel.2009.09.004
- Wu S, Wolf DA. Destruction of RhoA CULtivates actin. Mol Cell 2009; 35:735 - 736; PMID: 19782022; http://dx.doi.org/10.1016/j.molcel.2009.09.012
- Zheng D, Sun Y, Gu S, Ji C, Zhao W, Xie Y, et al. LNX (ligand of numb-protein X) interacts with RhoC, both of which regulate AP-1-mediated transcriptional activation. Mol Biol Rep 2010; 37:2431 - 2437; PMID: 19701800; http://dx.doi.org/10.1007/s11033009-9754-5
- Visvikis O, Lores P, Boyer L, Chardin P, Lemichez E, Gacon G. Activated Rac1, but not the tumorigenic variant Rac1b, is ubiquitinated on lys 147 through a JNK-regulated process. FEBS J 2008; 275:386 - 396; PMID: 18093184; http://dx.doi.org/10.1111/j.17424658.2007.06209.x
- Kärkkäinen S, van der Linden M, Renkema GH. POSH2 is a RING finger E3 ligase with Rac1 binding activity through a partial CRIB domain. FEBS Lett 2010; 584:3867 - 3872; PMID: 20696164; http://dx.doi.org/10.1016/j.febslet.2010.07.060
- Castillo-Lluva S, Tatham MH, Jones RC, Jaffray EG, Edmondson RD, Hay RT, et al. SUMOylation of the GTPase Rac1 is required for optimal cell migration. Nat Cell Biol 2010; 12:1078 - 1085; PMID: 20935639; http://dx.doi.org/10.1038/ncb2112
- Matos P, Jordan P. Rac1, but not Rac1B, stimulates RelB-mediated gene transcription in colorectal cancer cells. J Biol Chem 2006; 281:13724 - 13732; PMID: 16551621; http://dx.doi.org/10.1074/jbc.M513243200
- Senadheera D, Haataja L, Groffen J, Heisterkamp N. The small GTPase rac interacts with ubiquitination complex proteins cullin-1 and CDC23. Int J Mol Med 2001; 8:127 - 133; PMID: 11445862
- Boyer L, Travaglione S, Falzano L, Gauthier NC, Popoff MR, Lemichez E, et al. Rac GTPase instructs nuclear factor-kappaB activation by conveying the SCF complex and IkBalpha to the ruffling membranes. Mol Biol Cell 2004; 15:1124 - 1133; PMID: 14668491; http://dx.doi.org/10.1091/mbc.E03-05-0301
- Stenmark H. Rab GTPases as coordinators of vesicle traffic. Nat Rev Mol Cell Biol 2009; 10:513 - 525; PMID: 19603039; http://dx.doi.org/10.1038/nrm2728
- Mills IG. The interplay between clathrin-coated vesicles and cell signalling. Semin Cell Dev Biol 2007; 18:459 - 470; PMID: 17692542; http://dx.doi.org/10.1016/j.semcdb.2007.07.001
- Luzio JP, Parkinson MD, Gray SR, Bright NA. The delivery of endocytosed cargo to lysosomes. Biochem Soc Trans 2009; 37:1019 - 1021; PMID: 19754443; http://dx.doi.org/10.1042/BST0371019
- Clague MJ, Urbe S. Endocytosis: the DUB version. Trends Cell Biol 2006; 16:551 - 559; PMID: 16996268; http://dx.doi.org/10.1016/j.tcb.2006.09.002
- Lee RHK, Iioka H, Ohashi M, Iemura S, Natsume T, Kinoshita N. XRab40 and XCullin5 form a ubiquitin ligase complex essential for the noncanonical wnt pathway. EMBO J 2007; 26:3592 - 3606; PMID: 17627283; http://dx.doi.org/10.1038/sj.emboj.7601781
- Carney DS, Davies BA, Horazdovsky BF. Vps9 domain-containing proteins: activators of Rab5 GTPases from yeast to neurons. Trends Cell Biol 2006; 16:27 - 35; PMID: 16330212; http://dx.doi.org/10.1016/j.tcb.2005.11.001
- Zhu H, Qian H, Li G. Delayed Onset of Positive Feedback Activation of Rab5 by Rabex-5 and Rabaptin-5 in Endocytosis. PLoS ONE 2010; 5:9226; PMID: 20169068; http://dx.doi.org/10.1371/journal.pone.0009226
- Mattera R, Tsai YC, Weissman AM, Bonifacino JS. The Rab5 guanine nucleotide exchange factor rabex-5 binds ubiquitin (ub) and functions as a ub ligase through an atypical ub-interacting motif and a zinc finger domain. J Biol Chem 2006; 281:6874 - 6883; PMID: 16407276; http://dx.doi.org/10.1074/jbc.M509939200
- Mattera R, Bonifacino JS. Ubiquitin binding and conjugation regulate the recruitment of rabex-5 to early endosomes. EMBO J 2008; 27:2484 - 2494; PMID: 18772883; http://dx.doi.org/10.1038/emboj.2008.177
- Davies BA, Carney DS, Horazdovsky BF. Ubiquitin regulation of the Rab5 family GEF Vps9p. Methods Enzymol 2005; 403:561 - 583; PMID: 16473620; http://dx.doi.org/10.1016/S0076-6879(05)03049-1
- Sakane A, Hatakeyama S, Sasaki T. Involvement of Rabring7 in EGF receptor degradation as an E3 ligase. Biochem Biophys Res Commun 2007; 357:1058 - 1064; PMID: 17462600; http://dx.doi.org/10.1016/j.bbrc.2007.04.052
- Miyakawa K, Ryo A, Murakami T, Ohba K, Yamaoka S, Fukuda M, et al. BCA2/Rabring7 promotes tetherin-dependent HIV-1 restriction. PLoS Pathog 2009; 5:1000700; PMID: 20019814; http://dx.doi.org/10.1371/journal.ppat.1000700
- Phan VT, Ding VW, Li F, Chalkley RJ, Burlingame A, McCormick F. The RasGAP proteins Ira2 and neurofibromin are negatively regulated by Gpb1 in yeast and ETEA in humans. Mol Cell Biol 2010; 30:2264 - 2279; PMID: 20160012; http://dx.doi.org/10.1128/MCB.01450-08
- Ang XL, Seeburg DP, Sheng M, Harper JW. Regulation of postsynaptic RapGAP SPAR by polo-like kinase 2 and the SCFbeta-TRCP ubiquitin ligase in hippocampal neurons. J Biol Chem 2008; 283:29424 - 29432; PMID: 18723513; http://dx.doi.org/10.1074/jbc.M802475200
- Spilker C, Acuna Sanhuez GA, Bockers TM, Kreutz MR, Gundelfinger ED. SPAR2, a novel SPAR-related protein with GAP activity for Rap1 and Rap2. J Neurochem 2008; 104:187 - 201; PMID: 17961154
- Pham N, Rotin D. Nedd4 regulates ubiquitination and stability of the guanine-nucleotide exchange factor CNrasGEF. J Biol Chem 2001; 276:46995 - 47003; PMID: 11598133; http://dx.doi.org/10.1074/jbc.M108373200
- Amsen EM, Pham N, Pak Y, Rotin D. The guanine nucleotide exchange factor CNrasGEF regulates melanogenesis and cell survival in melanoma cells. J Biol Chem 2006; 281:121 - 128; PMID: 16272156; http://dx.doi.org/10.1074/jbc.M507595200
- Yoshikawa Y, Satoh T, Tamura T, Wei P, Bilasy SE, Edamatsu H, et al. The M-ras-RA-GEF-2-Rap1 pathway mediates tumor necrosis factor-alpha dependent regulation of integrin activation in splenocytes. Mol Biol Cell 2007; 18:2949 - 2959; PMID: 17538012; http://dx.doi.org/10.1091/mbc.E07-03-0250
- Kanemura H, Satoh T, Bilasy SE, Ueda S, Hirashima M, Kataoka T. Impaired vascular development in the yolk sac and allantois in mice lacking RA-GEF-1. Biochem Biophys Res Commun 2009; 387:754 - 759; PMID: 19635461; http://dx.doi.org/10.1016/j.bbrc.2009.07.108
- Wei P, Satoh T, Edamatsu H, Aiba A, Setsu T, Terashima T, et al. Defective vascular morphogenesis and mid-gestation embryonic death in mice lacking RA-GEF-1. Biochem Biophys Res Commun 2007; 363:106 - 112; PMID: 17826737; http://dx.doi.org/10.1016/j.bbrc.2007.08.149
- Hayakawa M, Kitagawa H, Miyazawa K, Kitagawa M, Kikugawa K. The FWD1/beta-TrCP-mediated degradation pathway establishes a 'turning off switch' of a Cdc42 guanine nucleotide exchange factor, FGD1. Genes Cells 2005; 10:241 - 251; PMID: 15743413; http://dx.doi.org/10.1111/j.1365-2443.2005.00834.x
- Hayakawa M, Matsushima M, Hagiwara H, Oshima T, Fujino T, Ando K, et al. Novel insights into FGD3, a putative GEF for Cdc42, that undergoes SCF(FWD1/ beta-TrCP)-mediated proteasomal degradation analogous to that of its homologue FGD1 but regulates cell morphology and motility differently from FGD1. Genes Cells 2008; 13:329 - 342; PMID: 18363964; http://dx.doi.org/10.1111/j.1365-2443.2008.01168.x
- Oshima T, Fujino T, Ando K, Hayakawa M. Proline-rich domain plays a crucial role in extracellular stimuli-responsive translocation of a Cdc42 guanine nucleotide exchange factor, FGD1. Biol Pharm Bull 2010; 33:35 - 39; PMID: 20045932; http://dx.doi.org/10.1248/bpb.33.35
- Su L, Lineberry N, Huh Y, Soares L, Fathman CG. A novel E3 ubiquitin ligase substrate screen identifies rho guanine dissociation inhibitor as a substrate of gene related to anergy in lymphocytes. J Immunol 2006; 177:7559 - 7566; PMID: 17114425
- Dovas A, Couchman JR. RhoGDI: Multiple functions in the regulation of rho family GTPase activities. Biochem J 2005; 390:1 - 9; PMID: 16083425; http://dx.doi.org/10.1042/BJ20050104
- Sjogren B, Blazer LL, Neubig RR. Regulators of G protein signaling proteins as targets for drug discovery. Prog Mol Biol Transl Sci 2010; 91:81 - 119
- DerMardirossian C, Bokoch GM. GDIs: Central regulatory molecules in rho GTPase activation. Trends Cell Biol 2005; 15:356 - 363; PMID: 15921909; http://dx.doi.org/10.1016/j.tcb.2005.05.001
- Kumar A, Hozo I, Wheatley K, Djulbegovic B. Thalidomide versus bortezomib based regimens as first-line therapy for patients with multiple myeloma: A systematic review. Am J Hematol 2011; 86:18 - 24; PMID: 21120867; http://dx.doi.org/10.1002/ajh.21904
- Einsele H. Bortezomib. Recent Results Cancer Res 2010; 184:173 - 187; PMID: 20072838; http://dx.doi.org/10.1007/978-3-642-01222-8_12
- Yang Y, Ludwig RL, Jensen JP, Pierre SA, Medaglia MV, Davydov IV, et al. Small molecule inhibitors of HDM2 ubiquitin ligase activity stabilize and activate p53 in cells. Cancer Cell 2005; 7:547 - 559; PMID: 15950904; http://dx.doi.org/10.1016/j.ccr.2005.04.029