Abstract
Actin cytoskeleton is one of the main targets of Rho GTPases, which act as molecular switches on many signaling pathways. During the past decade, actin has emerged as an important regulator of gene expression. Nuclear actin plays a key role in transcription, chromatin remodeling, and pre-mRNA processing. In addition, the “status” of the actin cytoskeleton is used as a signaling intermediate by at least the MKL1-SRF and Hippo-pathways, which culminate in the transcriptional regulation of cytoskeletal and growth-promoting genes, respectively. Rho GTPases may therefore regulate gene expression by controlling either cytoplasmic or nuclear actin dynamics. Although the regulation of nuclear actin polymerization is still poorly understood, many actin-binding proteins, which are downstream effectors of Rho, are found in the nuclear compartment. In this review, we discuss the possible mechanisms and key proteins that may mediate the transcriptional regulation by Rho GTPases through actin.
Introduction
The Rho family of small GTP-binding proteins (Rho GTPases) contains well-known regulators of actin cytoskeleton rearrangements. Rho GTPases are activated when they are bound to GTP. This enhances their interaction with downstream effectors, including proteins kinases and actin-binding proteins (ABPs). Once GTP is hydrolyzed to GDP, Rho GTPase activity and signal transduction decreases. These molecular switches are regulated through proteins that can be grouped to GTPase-activating proteins (GAPs), guanine nucleotide dissociation inhibitors (GDIs), and guanine nucleotide exchange factors (GEFs). Briefly, GAPs and GDIs are negative regulators of GTPase activity, while GEFs are positive regulators (reviewed in ref. Citation1). Many of these components, as well as Rho GTPases themselves, are found in the nucleus, suggesting that Rho-related signaling processes may also take place in this cellular compartment.
Actin cytoskeleton is one of the main targets for Rho GTPases. Many ABPs that regulate different aspects of actin polymerization, such filament nucleation, severing and depolymerization, are downstream targets of Rho GTPases. In the cytoplasm, ABPs have the crucial role of modulating actin cytoskeletal organization in response to intra- and extracellular signaling, which enables for example cell motility (reviewed in ref. Citation1). Also actin itself, as well as many ABPs, has been localized to the cell nucleus, where they have been linked to gene expression processes. Nuclear actin can regulate the access of the transcription machinery to the genes. It is part of chromatin modifying complexes and as such can regulate chromatin remodeling and histone epigenetic marks. Also, actin can have a general effect on transcription as a potential component of the polymerase complexes (reviewed in refs. Citation2 and Citation3). In addition to affecting general transcriptional activity, nuclear actin has been linked to the regulation of specific genes. Nuclear actin plays a key role regulating the activity of ABP megakaryoblastic leukemia 1 (MKL1), a co-activator of transcription factor serum response factor (SRF), which regulates the expression of many cytoskeletal genes in response to changes in the actin monomer pool.Citation4-Citation6 The actin cytoskeleton and Rho GTPases have also been linked to transcriptional regulation by the growth-regulating Hippo-pathway,Citation7-Citation9 further expanding the potential impact of the Rho-actin axis on gene expression. Nuclear ABPs may influence gene expression by different mechanisms. They can regulate nuclear actin dynamics and as such regulate chromatin remodeling and transcription initiation. Some ABPs may function as direct co-regulators of transcription factors, and some as part of the general transcription machinery (reviewed in refs. Citation10 and Citation11).
In this review article, we summarize the current knowledge on how the classical actin-regulating Rho GTPases RhoA, Rac1, and Cdc42, may regulate gene expression. As well-established regulators of actin dynamics, these molecular switches can affect transcription either by modulating nuclear actin polymerization properties or by impinging on actin-responsive transcription factors. At the heart of these effects are the numerous ABPs, which are both downstream targets of Rho GTPases and also present in the nuclear compartment. As Rho GTPases themselves have also been localized to the nucleus, they could therefore regulate the nuclearly localized ABPs directly in this cellular compartment. Alternatively, the Rho GTPases could regulate the ABPs in the cytoplasm, followed by subsequent nuclear translocation of the ABP (). An essential open question is therefore: where do the Rho GTPases act, in the cytoplasm or in the nucleus?
Figure 1. ABPs in the nucleus. Many ABPs that are downstream targets of Rho GTPases are also present in the cell nucleus, where they have been linked to gene expression processes and can regulate nuclear actin dynamics. Two alternatives exist on how Rho GTPases can regulate nuclear ABPs. (1) Rho GTPases can activate ABPs in the cytoplasm and the activated ABPs (ABP*) then shuttle into the nucleus. (2) Upstream signaling could activate the nuclear localized Rho GTPases, which then signals to ABPs in the nucleus. Nuclear ABPs may influence gene expression by regulating nuclear actin dynamics (upper part of the figure). Importin-9 (Ipo9) together with ADF/cofilin (cof) mediates the nuclear import of actin (a). Exportin-6 (Exp6) together with profilin mediates the nuclear export of actin (b). Profilin and mDia formins promote actin polymerization by mediating the addition of ATP-actin monomers to the growing end (+ end) of the actin filament (c). In the filament, ATP is hydrolyzed to ADP and ADP-actin monomers are then released from the minus end (- end). ADF/cofilins (cof) mediate the disassembly of actin filaments (d). Arp2/3 complex and its activators WASp/N-WASp and WAVEs may contribute to nuclear actin nucleation (e). Several ABPs themselves have also been linked to gene expression processes, and also actin (red arrow head) is a component of many nuclear complexes (lower part of the figure). Arp2/3 complex, WASp/N-WASp, WAVEs, cofilin-1 (cof), Nuclear myosin I (NMI), Myosin VI (MyoVI), Myosin Vb (MyoVb) and filamin (Fln) have been linked to RNA polymerase function (pol) (f). In this context N-WASp can associate with PSF-NonO complex that binds the polymerase. NMI also participates to chromatin remodeling together with actin (g). Profilin, as well as Myosin Va (MyoVa) are both linked to pre-mRNA splicing. hnRNPs are also involved at this level together with actin (h).
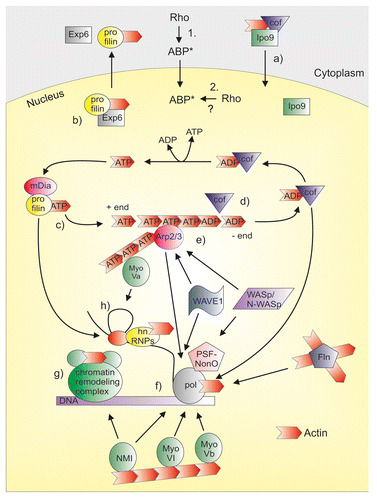
Both Monomeric and Polymeric Actin Have Functional Roles in the Cell Nucleus
In the cytoplasm, Rho GTPases are essential regulators of actin dynamics, regulating the balance between actin monomer (G-actin) and filament (F-actin) pools. This same regulation may also be important in the cell nucleus, as both monomeric and polymeric actin has been linked to gene expression processes. Rho GTPases may therefore modulate nuclear actin dynamics, and thereby control transcription.
In an average cell in culture, about half of actin is monomeric and half polymerized to an elaborate network of cross-linked filaments, which can be beautifully visualized by phalloidin staining. However, phalloidin does not usually stain the nucleus. The polymerization status of nuclear actin has indeed been one of the most enigmatic questions in this research field, due to the fact that the visualization of nuclear actin filaments has been problematic. In the past years however, there has been accumulating evidence advocating for the presence of nuclear actin polymers. Fluorescence recovery of photobleaching experiments demonstrated that in addition to the monomeric pool, there are additional pools of actin with slower mobilities, some of which could correspond to actin polymers.Citation12,Citation13 Very recent data using new probes has made it possible, for the first time, to actually visualize nuclear actin polymers. For example, a recent study demonstrated the transient formation of actin filaments in the nucleus within 20 seconds after serum stimulation by the use of F-actin probe Lifeact-GFP fused with nuclear localization signal (NLS), and these same structures could also be stained with phalloidin.Citation14 Also a new fluorescent actin probe Utr230-EN has been reported to detect specifically nuclear actin polymers. In this probe, EGFP-NLS is fused with part of the Calponin Homology domain of utrophin (Utr230) that binds actin filaments without perturbing the nuclear actin architecture. This probe recognizes nuclear actin punctae of submicron lengths that are excluded from chromatin-rich areas.Citation15 Although the relationships between the actin polymers detected with these probes remains to be investigated, these studies have finally provided the first visual “proof” of nuclear actin polymers. Additionally, these results suggest that also nucleus must contain mechanisms to regulate actin polymerization.
This idea is further supported by the notion that both monomeric and polymeric actin has been linked to gene expression processes. Already 15 years ago, monomeric actin was reported to bind brahma-related gene 1 (BRG1), a subunit of the BRG1-associated factor (BAF) chromatin remodeling complex from the SWI/SNF family. Actin is then required for both the optimal ATPase activity of BRG1 and the association of the BAF complex with chromatin.Citation16 Also INO80 complex in yeast contains monomeric actin as a subunit together with actin related protein 4 (Arp4) and Arp8, which influence actin polymerization and help to keep actin monomeric within the complex.Citation17 Moreover, the barbed end of actin, from where the polymerization usually occurs, is blocked in the INO80 complex.Citation18 Therefore, at least in these chromatin remodeling complexes, actin seems to operate as a monomer, but whether this applies to other actin-containing remodelers needs to be confirmed. Actin is also part of the basal transcription machinery in association with all three RNA polymerases (pol I, II, III)Citation19-Citation23 and antibodies against actin inhibit pol I and pol II mediated transcription.Citation20,Citation23 However, the functional form of actin in the polymerase complexes has not been investigated biochemically. Some evidence favors the monomeric form. For instance, monomeric actin has been reported to associate with cyclin-dependent kinase 9, the catalytic subunit of positive elongation factor P-TEFb and therefore suggested to participate in pol II-dependent transcription elongation.Citation24 Actin, as monomers, also interacts with nascent transcripts through heterogeneous nuclear ribonucleoproteins (hnRNPs). Indeed, actin was coprecipitated with hnRNP U and with the histone acetyl transferase (HAT) p300/CBP-associated factor (PCAF) using DNase I affinity chromatography, which has a high affinity for monomeric actin. Actin in this complex is suggested to promote the recruitment of HATs along active genes. This would result in the unfolding of chromatin and therefore facilitation of the transcription machinery progression.Citation25
There is also evidence in the literature that some type of polymeric actin could play a role in gene expression, including the experiments showing that transcription is impaired by chemical agents that inhibit actin polymerization.Citation13,Citation26 Nuclear actin polymerization is also required for ongoing transcription and transcriptional reactivation of the pluripotency gene Oct4 in transplanted ES cell nuclei.Citation27 Also the presence of numerous myosin isoforms in the nucleus (reviewed in ref. Citation28) argues in favor of functional nuclear actin polymers. In the cytoplasm, these actin-dependent motors walk along actin filaments and either create contractile forces or carry cargo along actin tracks (reviewed in ref. Citation29). Concordantly, actomyosin motor activity provided by nuclear myosin I (NMI) and polymeric actin has been linked to pol I and pol II transcriptionCitation19,Citation23,Citation26 and to other nuclear tasks, including intranuclear movements of interphase chromosomal sitesCitation30 and even whole chromosomes.Citation31 In addition, the nuclear presence of several proteins that regulates actin polymerization or bind filamentous actin in the cytoplasm, and especially their link to transcription further support the requirement of nuclear actin polymers in these processes (see below).
The functional requirement for both monomeric and polymeric nuclear actin strongly suggests that nuclear actin dynamics must be tightly regulated, which opens the doors potentially for Rho signaling and the nuclear ABPs downstream (see below).
Rho-Regulated ABPs in the Nucleus
As mentioned above, many actin-binding proteins (ABPs) are downstream effectors for Rho GTPases. Interestingly, many of these proteins are also found in the cell nucleus, and linked to gene expression processes (; ). Rho GTPases could therefore influence transcription by regulating nuclear activities of these ABPs, and thus affect nuclear actin dynamics, which is potentially a prerequisite for efficient gene expression. However, in many cases, it is not clear if the ABPs actually regulate actin also in the nuclear space, and whether their effect on transcription is linked with actin.
Table 1. Direct Rho effectors in the nucleus
In the cytoplasm, ADF/cofilins mediate the disassembly of actin filaments to monomers by promoting their severing and by increasing actin depolymerization. As such, ADF/cofilins maintain the cellular G-actin pool (reviewed in ref. Citation32). Rho GTPases regulate ADF/cofilin activity through several pathways. Rho kinases (ROCK I and II) and p21 activated kinase 1 (PAK1) are effectors of RhoA and Rac1, respectively, which can phosphorylate and therefore activate LIM kinases (LIMK). Phosphorylation of serine-3 residue of cofilin by LIMKs inhibits the actin-binding activity of cofilin and therefore stabilizes the actin cytoskeleton.Citation33 ADF/Cofilins are also present in the nucleus. Early studies demonstrated the nuclear accumulation of cofilin upon for example heat shock,Citation34 dimethylsulfoxide treatmentCitation35 or costimulatory signals in human T cells.Citation36 Also nuclear accumulation of actin has been observed upon various cellular stresses, and already early studies suggested a role for cofilin in the nuclear import of actin in mast cells upon Latrunculin treatment or heat shock.Citation37 An increase in nuclear amounts of actin and dephosphorylated cofilin has also been observed during senescence of mammalian cells in culture.Citation38 Nuclear accumulation of cofilin during stress conditions seems to be important for the formation of nuclear actin rods, which have been suggested to reduce actin treadmilling and release the ATP for other uses,Citation39 helping cell survival in stress conditions. Indeed, actin rod formation in the nucleus was abolished and cells had an impaired ability to respond to stress, when nuclear shuttling of cofilin was disrupted, highlighting the importance of nuclear cofilin activity in stress conditions.Citation40 It was subsequently demonstrated that cofilin also plays a role in the steady-state nuclear import of actin, where cofilin seems to serve as an adaptor for actin and its nuclear import receptor Importin-9. Because nuclear import of actin supports transcription, cofilin plays a crucial role regulating this process.Citation12 Interestingly, cofilin itself has also been linked to transcription, and it has been suggested that local depolymerization of F-actin by cofilin-1 is necessary for transcription elongation.Citation41 Rho GTPases therefore have the potential to influence transcription by 2 different mechanisms through cofilin regulation. First, they can regulate cofilin activity in the cytoplasm, and thereby influence nuclear import of actin, which is required for maximal transcription.Citation12 Second, the Rho GTPases may influence nuclear activity of cofilin, which may have a more direct impact on gene expression.
Another small actin-binding protein that plays a role in regulating nucleo-cytoplasmic shuttling of actin is profilin. Profilin promotes actin polymerization by mediating the addition of ATP-actin monomers to the growing end of the filament, but also inhibits spontaneous nucleation of the filaments (reviewed in ref. Citation32). In this process, profilins act in concert with Rho GTPase effector proteins formins (reviewed in ref. Citation42), which are also found in the nucleus (see below). Rho GTPases may also influence profilin activity through ROCK1-mediated phosphorylation of profilin,Citation43 and through modulation of cellular phosphoinositide (PI) synthesis (reviewed in ref. Citation44), because for example PtdIns(4,5)P2 binding inhibits the actin-binding activity of profilin.Citation45 In the nucleus profilin serves as an adaptor for nuclear export complex of actin by enhancing the interaction between actin and its export receptor Exportin-6.Citation46 In Chironomus tentans profilin associates with transcriptionally active genes on polytene chromosomes.Citation47 Profilin has also been linked to pre-mRNA splicingCitation48 and regulation of Myb-related transcription factor p42POP.Citation49 Profilin II, a brain isoform of profilin, has been demonstrated to localize in neuron nuclei. This nuclear pool of Profilin II responds to N-methyl-D-aspartate receptor signaling and may influence the transcription of specific genes via p42POP.Citation50 Whether these latter processes depend on profilin to promote nuclear actin filament assembly or inhibit spontaneous actin nucleation remains to be investigated.
Arp2/3 complex is a 7 subunit complex containing 2 actin related proteins, Arp2 and Arp3. It nucleates the formation of new actin filaments from the sides of existing filaments, thereby creating a branched actin filament network found, for example, at the leading edge of motile cells. The nucleation activity of Arp2/3 complex is activated, for instance, by Wiscott-Aldrich syndrome proteins (WASp and N-WASp) and WASp family verprolin homologous (WAVE) family proteins. WASp and the more ubiquitously expressed form N-WASp are both direct targets of Rho GTPase Cdc42, whereas WAVEs are activated by Rac1 (reviewed in ref. Citation51). Interestingly, both Arp2/3 complex itself, as well as its activators N-WASp, WASp, and WAVE proteins, have been observed in the nucleus. How Arp2/3 complex enters the nucleus remains unclear, but Src family kinases have been shown to control the nuclear localization of N-WASp through phosphorylation. The phosphorylation status seems to be important, because the phosphorylated N-WASp was excluded from the nucleus, whereas the unphosphorylated N-WASp was nuclear and could participate in transcriptional regulation of HSP90.Citation52 Also formin-binding protein 11 can regulate N-WASp localization by trapping it in the nucleus.Citation53 Arp2/3 complex has been demonstrated to associate with pol II, and has been linked to its transcriptional activity.Citation54 Together with its activator N-WASp, Arp2/3 complex seems to be required for nuclear actin polymerization at least in some assays,Citation54,Citation55 whereas another study found evidence for only formin-nucleated actin polymerization in nuclear extracts.Citation14 Nuclear N-WASp is found in a large protein complex with polypyrimidine-tract-binding-protein-associated splicing factor-non-Pou-domain octamer-binding protein/p54 (PSF-NonO), and this complex also contains actin and pol II.Citation55 Almost the same complex is also recruited to the gene enhancer upon retinoic acid-induced transcription of the HoxB gene, and actin polymerization seems to be required for this.Citation56 Nuclear WASp, on the other hand, associates with RBB5 methyltransferase, and is required for the targeting of this enzyme to proximal promoter locus of the TBX21 gene. At the same time, mutations causing Wiscott Aldrich Syndrome or depletion of WASp result in decreased enrichment of filamentous actin on this promoter, and results in impaired T helper 1 immunity.Citation57 It has therefore been hypothesized that N-WASp/WASp and Arp2/3 collaborate during RNA pol II-dependent transcription. As mentioned above, nuclear actin polymerization is required for transcriptional reprogramming in the Xenopus oocyte.Citation27 A recent study identified WAVE1 as a candidate for regulating actin polymerization downstream of Toca1 and Rac1 in this context. Nuclear WAVE1 was shown to be required for transcriptional reprogramming, normal embryonic development and hox gene expression. The N-terminal Wave homology domain was responsible for binding to the transcription apparatus, which was required for pol II function. However, the association with the transcription apparatus was not dependent on actin polymerization, and a WAVE1 construct lacking the actin-regulatory part rescued most effects of WAVE1 antisense mediated depletion, leaving the role of actin open.Citation58 The actin nucleator Arp2/3 complex and its activators have therefore many roles in the cell nucleus. In the future, it will be interesting to study if/how the Rho GTPases may impinge on these essential processes, and investigate how these actin branch nucleators function together with, for example formins (see below), in controlling nuclear actin dynamics.
Also formins, which are the largest family of Rho GTPase effectors, have been shown to nucleate and elongate new actin filaments. Formins, in contrast to Arp2/3 complex, form linear unbranched filaments and accelerate actin polymerization (reviewed in ref. Citation42). Some formin family members are autoinhibited and activated by Rho GTPases, which release this inhibition.Citation59 Mouse diaphanous homolog 2 (mDia2) formin has been shown to shuttle between nucleus and cytoplasm in Importin-α/β- and Crm1-dependent manner.Citation60 The nuclear import and export mechanisms of the other mDia isoforms have not yet been clarified, but the nuclear localization seems to be a common feature for all mDia isoforms.Citation14,Citation60 mDia1 and mDia2 has recently been shown to regulate nuclear actin polymerization in response to serum stimulation. As discussed above, these transient filaments were positive for the F-actin probes Lifeact and phalloidin, and promoted SRF transcriptional activity through cofactor MKL1 (see also below).Citation14 How mDia1/2 are activated in the nucleus remains unclear. Also formin homology 2 domain containing protein 1 (FHOD1), another formin, has been demonstrated to have a nuclear function. Caspase-3-mediated cleavage of FHOD1 generates a C-terminal fragment that localizes to the nucleolus. Overexpression of this cleavage fragment seems to inhibit pol I mediated transcription.Citation61 However, whether these functions are dependent on the ability of FHOD1 to nucleate actin polymers is unclear.
Adenomatous polyposis coli (APC) is a tumor suppressor protein that promotes generation of new actin filaments. In this process, APC has been thought to cooperate with another actin nucleation factor mDia1.Citation62 In the nucleus, APC can antagonize β-catenin mediated transcription by sequestering β-catenin from the transcription complex and by enhancing its nuclear export. This prevents β-catenin from activating the Wnt signaling pathway, and as such prevents cell proliferation.Citation63 Thus, APC has an important role in regulating transcription, but whether this regulation is dependent on the ability of APC to promote actin filament assembly has not been studied. In the cytoplasm, APC interacts with Cdc42. This interaction promotes the localization of APC at the leading edge of the cell, where F-actin is enriched.Citation64 Since both of these proteins are present in the nucleus (see below), it is possible, that similar regulation also exists in the nucleoplasm.
The actin-binding IQ motif containing GTPase activating protein 1 (IQGAP1) binds also Rac1 and Cdc42, and acts as a scaffold for many proteins that regulate actin dynamics in the cytoplasm (reviewed in ref. Citation65). Recently, nuclear localization of IQGAP1 in different cell types has been demonstrated. The nuclear entry is cell-cycle-dependent and possibly required for cell-cycle progression.Citation66 Interestingly, also DNA replication stress induces nuclear accumulation of IQGAP1 together with actin and Rac1, suggesting coordinated nuclear transport of actin and its regulators in stress conditions.Citation67
Filamin-A promotes cross-linking of actin filaments and is a downstream substrate of PAK1, an effector protein of Rac1 and Cdc42 (reviewed in refs. Citation68 and Citation69). Both full-length and C-terminal cleavage fragment of Filamin-A have been shown to interact with nuclear proteins. The C-terminal fragment of filamin-A translocates to the nucleus, where it seems to downregulate androgen receptor (AR) function by competing with AR co-activator intermediary factor 2.Citation70 Filamin-A has been also shown to contribute to DNA damage response by interacting with breast cancer type 2 susceptibility protein, a protein that mediates the recombinational repair of DNA double strand breaks.Citation71 Interestingly, filamin-A seems to also localize in the nucleoli, the site of ribosome biogenesis, and associate with pol I machinery to suppress rRNA transcription. Filamin-A mutant lacking the actin-binding domain is unable to repress the promoter activity, suggesting that filamin-A mediates its suppressive function to rRNA transcription together with actin.Citation72 Also ABPs such as Thymosin-β4Citation73 and α-actinin,Citation74 which are both targets of Rho GTPase signaling,Citation75,Citation76 have been found in the nucleus. However their nuclear function has not been determined.
Rho GTPases have also the potential to regulate ABPs through their effects on other signaling molecules, such as Ca2+ and phosphoinositides, which are also present in the cell nucleus and that regulate actin dynamics in the cytoplasm (reviewed in refs. Citation44 and Citation77). PtdIns(4,5)P2 is an important signaling lipid and its metabolism is controlled by many enzymes that are also found in the nucleus (reviewed in ref. Citation77). PtdIns(4,5)P2 has been linked to many nuclear processes, such as chromatin remodeling. For example, PtdIns(4,5)P2-binding has been suggested to mediate actin-binding of the SWI/SNF-like BAF chromatin remodeling complex,Citation78 and it also seems to be critical for targeting this complex to chromatin during lymphocyte activation.Citation16 PtdIns(4,5)P2 modulates the activity of many ABPs found in nucleus, such as N-WASp, cofilin, profilin, CapZ, ezrin, and gelsolin family proteins (reviewed in ref. Citation79). Nevertheless, it is not known if PtdIns(4,5)P2actually regulates the activities of these proteins in the nuclear compartment. The gelsolin-family proteins modulate actin dynamics by capping, uncapping, severing, and bundling of actin filaments. Severing is activated by Ca2+, while capping is blocked by PtdIns(4,5)P2 (reviewed in ref. Citation11). Some of the gelsolin-family members, including gelsolin, flightless I, and supervillin, function as transcriptional co-activators of nuclear hormone receptors.Citation80-Citation82 How actin fits to this picture is yet unclear.
How Does Rho GTPase Signaling Reach the Nucleus?
As we have now established that the nucleus contains a plethora of actin-binding proteins, which are well-established downstream targets of Rho GTPases, and affect gene expression, the next obvious question is how might the Rho signaling actually reach these proteins. As the nuclear actin field is still relatively young, we still do not understand how the activities of ABPs are regulated within the nuclear compartment. Two, not mutually exclusive, alternatives exist on how Rho signaling could regulate nuclear actin dynamics. First, upstream signaling could activate the nuclearly localized Rho GTPases, which then signal to nuclear ABPs or second, ABP activation by Rho takes place in the cytoplasm, and the activated ABPs then shuttle into the nucleus (). Indeed the activity status of certain ABPs has been linked to their subcellular localization. For instance the subcellular localization of cofilin seems to depend, at least partly, on its phosphorylation status, which is heavily influenced by Rho GTPases. It has been shown by many reports that non-phosphorylated and therefore active cofilin accumulates into the nucleus.Citation34-Citation36,Citation83 Also other ABPs, such as myosin II regulatory MLC20Citation84 and N-WASp,Citation85 are found in the nucleus in their active forms. However it is not clear, whether their activation takes place in the nucleus or in the cytoplasm.
Several studies have reported the existence of nuclear Rho GTPases as well as their regulators and downstream effectors. RhoA and Rac1 have been detected in the nucleus by biochemical fractionationCitation86,Citation87 and GFP-tagged Cdc42 and Rac1 by immunofluorescence.Citation87-Citation89 In addition, RhoA and Rac1 are found in the nucleus in their active GTP-bound form.Citation86,Citation87 Rac1 has been shown to accumulate in the nucleus during G2 phase of the cell cycle and accelerates cell division. In early G1, Rac1 is excluded from the nucleus and has the opposite effect on cell division. This suggests that Rac1 shuttles in and out of the nucleus in a cell-cycle dependent manner, and plays a positive role in the regulation of cell division.Citation87 In addition, Rac1 is found in the Xenopus oocyte nucleus, as well as in transplanted somatic cell nuclei.Citation58 The data on Rho GTPase nuclear localization is thus very fragmented, and more experimental evidence is clearly needed to establish their nuclear roles in different cells and organisms.
Rho GTPases are relatively small proteins (~21 kDa), and could therefore, in principle, diffuse passively through the nuclear pore complexes. However, many of them—such as RhoC, RhoG, Rac1, and Cdc42—have a NLS in their C-terminal polybasic region.Citation89,Citation90 The NLS is usually recognized by specialized import receptors, such as Importin-α/β, which docks the complexes through nuclear pores by active transport mechanism.Citation91 It is believed that the NLS of Rho GTPases is required when they are in protein complexes that are too large to passively diffuse through nuclear pores. For instance Rac1, together with its regulator MgcRacGAP, has been shown to promote the nuclear import of Stat3 and Stat5 by mediating the complex formation with Importin-α.Citation92,Citation93 Also nuclear import of another Rho regulatory protein, SmgGDS, is dependent on Rac1 NLS.Citation89 Regardless of the import mechanism, it must be concluded that Rho GTPases are not very easy to detect in the nucleus, and this has clearly hindered the development of the field.
In addition to Rho GTPases, some of their regulators, GAPs, GDIs, and GEFs, have been shown to exhibit a nuclear localization, indicating that Rho GTPase activity could be controlled within the nucleus. The RhoA-specific GEFs Net1 and Ect2 seem even to localize predominantly to the nucleus. The N-terminus of Net1 contains 2 functional NLS sequences, which mediate its nuclear import.Citation94-Citation96 Also Rho GAP ArhGAP11A localizes in the nucleus upon DNA damage stress and associates with p53 affecting its transcriptional activity.Citation97 However, it is not known how Rho GTPases, or the signaling pathways downstream of them, contribute to this. GDIα, another regulator of Rho GTPases, has been identified in a large nuclear multiprotein complex that associates with DNA-bound estrogen receptor α (ERα). GDIα increases ERα transcriptional activity and differentially influences the expression of estrogen responsive genes in MCF-7 breast cancer cells.Citation98 Again here the role of Rho GTPases themselves remains unclear. Interestingly, β-actin and several actin-binding proteins, such as myosin IC and gelsolin, have also been found in the protein complex with ERα in the nucleus after hormonal stimulation, suggesting that regulation of ERα transcriptional activity in breast cancer cells requires a dynamic, regulated nuclear actin network.Citation99
Finally, several downstream effectors of Rho GTPases, which can mediate the signals to ABPs, have been found in the nucleus (). ROCK-I and II represent the best characterized downstream effectors of RhoA and they seem to have nuclear functions as well. For instance, during transforming growth factor-β (TGF-β)-induced epithelial-mesenchymal transition (EMT), ROCK is reported to translocate to the nucleus to regulate cell cycle progression.Citation100 In addition, nuclear ROCK II was identified as an interacting partner for histone acetyltransferase p300, and this interaction promotes p300 acetyltransferase activity.Citation101 Also the effector proteins of Rac1 and Cdc42, such as PAKs have been shown to function in the nucleus. PAK1 has 3 NLSsCitation102 and apparently interaction of dynein light chain 8 is required for nuclear import of PAK1.Citation103 It is also linked to transcription by its association with active chromatin,Citation102 and to regulation of ERα activity.Citation104
MKL1-SRF Pathway Monitors Actin Monomer Levels Downstream of Rho Signaling
In addition to the potential regulation of “general” transcriptional activity through modulation of nuclear actin dynamics, Rho GTPases have also been linked to gene-specific transcription. These pathways seem to utilize both cytoplasmic and perhaps also nuclear signaling events, and many of the above-mentioned ABPs also play a critical role.
Undoubtedly, the best-characterized transcription factor downstream of Rho signaling is the SRF. It is highly conserved during evolution and directs the signal-induced expression of its target genes by binding with high affinity to CC(A/T)6GG sequence also referred as CArG-box or serum response element (SRE).Citation105 SRF target genes consists of growth-promoting, muscle-specific, and contractility-related genesCitation106; therefore, it is not surprising that many biological processes depend on SRF, and SRF null embryos have severe gastrulation defects.Citation107 Importantly, because many target genes are components of the cytoskeleton, SRF has been referred to as “master regulator of actin homeostasis in cells”Citation108 and plays a role in many actin-dependent processes, such as cell motility.Citation109
Two main signaling pathways regulate SRF activity in cells, and they utilize different sets of transcriptional coactivators to transmit the signal. For example, at the c-fos gene, SRF interacts with the members of Ets domain protein family, the ternary complex factors, in response to growth factors and mitogen activated protein (MAP) kinase pathway.Citation110 The other pathway involves Rho GTPases and is required for transcription of the SRF targets such as srf and vinculin, but not c-fos and egr1.Citation6,Citation111 Therefore, at least 2 types of SRF target genes can be distinguished based on their sensitivity to Rho or MAP kinase signaling.Citation112 Downstream of RhoA, 2 pathways leading to actin polymerization have been shown to play a role in SRF activation: activation of kinase cascade consisting of ROCK and LIMK, and leading to phosphorylation and thus inactivation of the actin disassembly factor cofilin, and activation of the formin family member mDia1 cooperatively with its associating protein vasodilator-stimulated phosphoprotein (VASP).Citation6,Citation113,Citation114 The end result of both pathways is increased actin filament formation; however, the activating signal for SRF transcription was shown to be the concomitant decrease in actin monomers.Citation6 Soon MKL1, also known as MAL and Myocardin related transcription factor-A (MRTF-A), was identified as the cofactor that mediates the signals from cellular G-actin levels to SRF.Citation5,Citation115,Citation116 In unstimulated cells, MKL1 is mainly localized to the cytoplasm, but upon stimulations that activate actin polymerization, MKL1 accumulates in the nucleus.Citation5 The RPEL domain of MKL1 is an actin monomer-binding domain and responsible for the actin-mediated regulation of the protein,Citation4,Citation5,Citation117 which occurs at multiple levels. In unstimulated cells (low F-actin, high G-actin), the cytoplasmic localization of MKL1 is held by very efficient Crm1-mediated nuclear export, where interaction with G-actin is required.Citation4 Depletion of the cellular G-actin pool upon RhoA-induced actin polymerization (high F-actin, low G-actin) reduces the interaction between G-actin and MKL1, which inhibits actin-dependent nuclear export of MKL1 and consequently results in its nuclear accumulation.Citation4 In addition, actin-binding inhibits nuclear import of MKL1 by Importin α/β heterodimer.Citation118
MKL1 RPEL domain contains a long bipartite NLS composed of 2 basic boxes (B2 and B3) that are separated by 30 residues. Both basic boxes are required for MKL1 nuclear import, and G-actin competes with Importin α/β import complex for these sites.Citation118 Structural studies have shown that the RPEL domain of MKL1 can bind either 3 (trimeric complex) or 5 actin monomers (pentameric complex). In the pentameric complex three actins are bound to the RPEL motifs and 2 actins to the intervening spacer elements. Pentameric complex retains MKL1 in the cytoplasm in unstimulated cells, because actin binding sterically occludes both basic elements, preventing their recognition by Importin α/β heterodimer. Instead, pentameric complex could serve as a structure that allows Crm1 binding, therefore enhancing MKL1 nuclear export. Pentameric complex dissociates into a trimeric complex relatively easily, because the actin-binding affinity to the spacer elements is weaker than to the RPEL motifs. However, because MKL1 shuttles between the cytoplasm and the nucleus in unstimulated cells, it is proposed that the cellular G-actin levels are not sufficient to saturate the RPEL domain. Rather, both export-competent pentameric complexes and import-competent trimeric complexes would be in equilibrium, resulting in the constant shuttling.Citation119
Actin-MKL1 complexes are also present in the nucleus, which inhibits MKL1 from activating SRF-mediated transcription. Therefore nuclear actin plays a key role in regulating SRF activation by influencing both the subcellular localization and nuclear activity of MKL1. Interestingly, the MKL1-actin complex responds similarly to cellular signals regardless of whether they are confined to cytoplasm or nucleus.Citation4 This suggests that similar signaling pathways that regulate actin polymerization must exist both in the nucleus and in the cytoplasm. This notion is supported by a recent study that demonstrated mDia-dependent actin polymerization in the nucleus that activated MKL1/SRF-dependent gene expression.Citation14 However, it is not known how nuclear mDia is activated or how actin-free MKL1 can activate transcription and whether binding of actin to MKL1 affects its interaction with other binding partners in the nucleus.
Taken together, G-actin regulates MKL1 by three mechanisms; it inhibits nuclear import of MKL1, enhances nuclear export and represses transcriptional activation of SRF target genes ().Citation4 This complex regulatory circuit therefore utilizes both cytoplasmic and nuclear signaling events, both perhaps mediated by appropriately localized Rho GTPases. By regulating MKL1-SRF activity, actin actually controls its own expression, alongside many other proteins that are components of actin cytoskeleton,Citation106 creating a feedback loop that maintains actin homeostasis in cells.
Figure 2. Rho GTPases as regulators of gene-specific transcription factors. Different plasma membrane receptors (a) and mechanical signals mediated by either cell-extracellular matrix adhesions (b) or cell-cell contacts (c) regulate Rho GTPase activity, which promotes actin polymerization (d). These same signals also act upstream of the Hippo-pathway (e), where activation of Mst1/2 and Lats1/2 kinases exclude YAP/TAZ from the nucleus, and prevent it from activating TEAD-mediated transcription. MKL1 is a sensor for actin monomer levels, and actin-binding inhibits nuclear import of MKL1 (f), and enhances its nuclear export (g). Upon actin polymerization, the actin monomer levels decrease and actin-free MKL1 can enter the nucleus (h). In the nucleus, actin-free MKL1 can activate SRF-mediated transcription (i). Actin filaments (or their subpopulation) also regulate the Hippo-pathway, and the regulation can occur either through a Lats-dependent mechanism (j) or directly at YAP/TAZ (k), via an unknown mechanism. Membrane molecules may therefore influence YAP/TAZ activity directly through the regulation of Hippo pathway or through Rho GTPase-dependent actin regulation. The contribution of nuclear actin in YAP/TAZ regulation has not been studied, as indicated by a question mark.
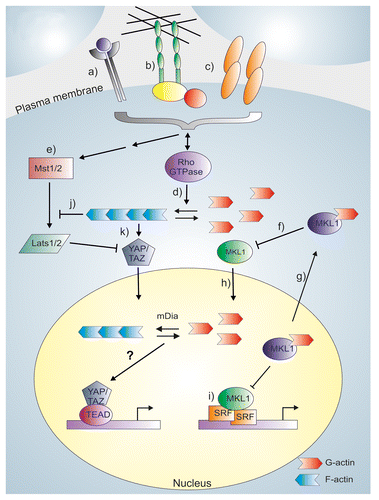
In principle all signals that modulate actin polymerization could also regulate MKL1-SRF circuit. Indeed, activated forms of all three Rho GTPases, RhoA, Cdc42, and Rac1, have been shown to activate SRF-mediated transcription.Citation111 Numerous plasma membrane receptors that respond to extracellular stimulus are upstream regulators of Rho GTPases, and some of them have been clearly demonstrated to activate MKL1-SRF pathway. For example, ligands such as lysophosphatidic acidCitation111 and sphingosine-1-phosphateCitation120 can activate the pathway through G-protein coupled receptors. One such receptor is leucine-rich repeat-containing G-protein coupled receptor 5, whose overexpression leads to activation of RhoA-MKL1-SRF pathway through G-protein G12/13.Citation121 Also signaling through receptor tyrosine kinases with ligands such as vascular endothelial growth factorCitation122 and neurotrophinCitation123 activating the pathway. Recent study has linked also androgens to the stimulation of the MKL1-SRF pathway through RhoA. Only a subset of androgen responsive genes is under control of SRF, but they seem to be overrepresented in prostate cancer cells.Citation124 SRF pathway has also been shown to be responsive to hormone-like signals that align peripheral and central clock cycling. In this process, yet unidentified hormone(s) and receptor(s) via Rho GTPases trigger the rhythmic activation of MRTF co-activators and as such, diurnal transcription of SRF target genes.Citation125
Not all extracellular stimuli are governed by chemical signals. Also mechanical signals have input on cell behavior. The main cellular structures that are involved in mechanosensing are cell-cell and cell-extracellular matrix (ECM) adhesions, which have both been shown to activate Rho GTPasesCitation126 and linked to MKL1-SRF activation. Disassembly of E-cadherin-dependent cell-cell contacts activates SRF via actin dynamics and MKL1. This pathway seems to be mediated by Rac1, but not RhoA, induced actin remodeling.Citation127 Interestingly, since disassembly of epithelial junctions is a key event in EMT, these results also suggest a role for MKL1-SRF in this process. Certainly, MKL1 has been implicated as an important mediator of TGF-β1-induced EMT.Citation128
Integrins mediate the attachment of cells to ECM and bridge ECM to actin cytoskeleton. Integrin-mediated actin dynamics are achieved through Src family of tyrosine kinases, which control GEFs and GAPs that act on Rho GTPases (reviewed in ref. Citation129). Given that MKL1-SRF pathway is regulated by changes in actin dynamics and many MKL1-SRF targets are structural components of focal adhesions, one could speculate that integrin-mediated mechanical signals are important upstream regulators of MKL1. Indeed, force application via integrins has been shown to activate RhoA, resulting in MKL1 nuclear translocation, SRF activation and increased expression of target genes, especially smooth muscle α-actin.Citation130 Interestingly, in addition to being regulated by integrins, the MKL1-SRF pathway has also been implicated in regulating expression of integrins, suggesting a feedback loop. Suppressor of cancer cell invasion (SCAI) has been connected to cancer cell invasion by its ability to control the expression of β1-integrin. It localizes to the nucleus and forms a complex with SRF, which prevents MKL1 from activating the expression of β1-integrin and as such, invasive cell migration.Citation131 Also Cdc42 has been linked to regulation of β1-integrins levels via SRF and this regulation is required for transendothelial migration of cancer cells.Citation132 Mechanical induction of MKL1-SRF pathway has been demonstrated even in isolated cells, where cell shape seems to be the triggering signal. Shape-induced differentiation in keratinocytes is greater in small and rounded cells than in spread and large cells, because in spread cells increased G-actin inhibits MKL1 nuclear translocation.Citation133 Rho GTPases undoubtedly participate in regulating actin cytoskeleton organization upon cell shape changes.
Actin Cytoskeleton is an Important Signaling Platform for Growth-Regulating Hippo-Pathway
Another signaling pathway that responds to Rho-mediated changes in actin dynamics is the Hippo pathway ().Citation7-Citation9 This pathway plays a crucial role in regulating growth by modulating cell proliferation, death, and differentiation and has therefore been linked to many cancers. The main players in the pathway are protein kinases mammalian STE20-like protein kinase 1 (Mst1) and Mst2, and Large tumor-suppressor homolog 1 (Lats1) and Lats2, and the transcription cofactors Yes-associated protein (YAP) and its homolog transcriptional co-activator with PDZ-binding motif (TAZ). Hippo pathway is activated when Mst1/2 phosphorylates Lats1/2, which in turn phosphorylates YAP/TAZ. Phosphorylated form of YAP/TAZ is excluded from the nucleus, which prevents them from activating transcription factor TEA domain family member (TEAD)-mediated gene expression and consequently prevents cell proliferation (reviewed in ref. Citation134).
Mechanical signals are considered as one of the main regulators of the Hippo pathway, and the actin cytoskeleton has emerged as the central platform for the different signals that modulate the Hippo pathway.Citation7-Citation9 Many adhesion molecules, such as FatCitation135 and junction-related proteins such as merlin/NF2,Citation136 angiomotin,Citation137 and α-cateninCitation138 have been shown to act upstream of the Hippo-pathway. However, it is interesting to note that the mechanically-induced activation of YAP/TAZ can occur also in isolated cells, suggesting that Hippo pathway is regulated also independently from cell-cell contacts.Citation7 Indeed, a recent report shows that cell shape or rigidity of the surrounding ECM induced YAP/TAZ-mediated proliferative capability.Citation9
Current view implies that cell density regulates Hippo pathway. In sparse cells that are both flat and spread, Hippo signals are rather weak, which allows nuclear YAP. This promotes cell proliferation by activation of TEAD-mediated transcription. At high cell densities, when cells are round and compact, Hippo signals are strong, which inhibits nuclear YAP and therefore suppresses cell proliferation.Citation8 The actin cytoskeleton is of course very different in round and flat cells, with the latter exhibiting strong stress fibers. Indeed, three recent studies have shown that inhibition of Rho or disruption of F-actin itself excluded YAP from the nucleus and suppressed its transcriptional activation.Citation7-Citation9 In addition, stabilization of the actin cytoskeleton with jasplakinolide results in decreased phosphorylation and activation of YAP.Citation139 Also studies in Drosophila have shown YAP/TAZ homolog Yorkie to be regulated by F-actin.Citation140 Despite some similarities to MKL1-SRF regulation, the mechanism by which Rho-actin signaling regulates the Hippo-pathway appears different. Expression of an actin mutant that cannot polymerize inhibits MKL1 activity,Citation5,Citation141 but no effect on YAP activation was observed, suggesting that actin monomers do not play a role in the Hippo-pathway.Citation7 Rather, it is more likely that contractile F-actin structure, such as a stress fiber subpopulation, and not actin polymerization per se, regulate YAP localization and this can occur either through Lats1/2-dependent or independent mechanism.Citation7-Citation9 However, it is interesting to note that F-actin-capping and –severing proteins such as CapZ, Gelsolin, and Cofilin inhibit YAP and TAZ in cells with low mechanical forces,Citation9 since especially Cofilins can increase the cellular G-actin levels (reviewed in ref. Citation32). The possible involvement of nuclear actin in Hippo-pathway regulation has not yet been examined.
Considering the very different cellular responses elicited by the MKL1-SRF and Hippo-pathways, it is very intriguing that both are activated by signals that modulate actin polymerization through Rho. This might be especially relevant in cancer cells, which exhibit both unrestricted growth and abnormal motile properties, potentially regulated by Hippo- and MKL1-SRF pathways, respectively, at different times of disease progression. Another similarity between the pathways is that the regulatory interactions with actin operates in both directions, because SRF regulates the expression of many cytoskeletal genes,Citation106 and the Hippo-pathway has been suggested to regulate actin organization at least in the fruit fly.Citation142 However, some critical pieces from the picture are still missing. First, if YAP regulation does not require G-actin signaling as MKL1-SRF does, what could be the link between YAP and actin? Core components of the Hippo pathway including Mst1/2Citation143 and Lats1Citation144 have been reported to colocalize with F-actin, but the functional significance of this is still unclear. However, since actin seems to control YAP/TAZ also independently of Lats, it has therefore been suggested that a specific F-actin structure in cells could inhibit an unidentified YAP antagonist or alternatively promote a positive cofactor.Citation7,Citation9 Elucidation of the precise mechanism on how this occurs is undoubtedly one key question in the Hippo research field.
Concluding Remarks
Recent years have elevated nuclear actin from skepticism to a crucial component in the nuclear compartment, with links to processes spanning the whole gene expression cascade, from transcription factor regulation to pre-mRNA processing. Very recent advances have finally provided the first tools to visualize actin polymers also in the nucleus,Citation14,Citation15 partly alleviating one of the main concerns in the field: the polymerizations status of nuclear actin. These developments also raise new questions, with one of the most pressing ones being: how is nuclear actin regulated to create the different structures recognized by the LifeAct and Utr230-EN probes? The nuclear presence of numerous ABPs certainly offers a plethora of options, and it seems that all major classes of actin regulators are also present in the nucleus, suggesting that in principle, actin dynamics could be controlled very similarly in both cellular compartments. However, very few ABPs have actually been shown to regulate actin polymerization in the nucleus, and in most cases it is just assumed that they do so. Therefore, in the future it will be important to find ways to specifically manipulate nuclear activities of key ABPs to dissect the main players in nuclear actin dynamics. The optogenic tools to manipulate nuclear mDia activity developed by the Grosse-lab serve as an excellent example of such approach.Citation14 After identifying the key ABPs, the next step will be the dissection of the upstream signaling pathways, and in this context, the Rho GTPases are certainly an attractive option. It is interesting to note that RhoA deficient cells are multinucleated or have fragmented nuclei. This might arise from defects in chromosomal segregation and cytokinesis in these cells,Citation145 but the potential contribution of the nucleoskeleton, and there the possible role of RhoA in regulating nuclear actin polymerization through formins, should not be neglected. It is clear that also the tools for detection of nuclear Rho GTPases must be improved to aid these functional studies on nuclear signaling pathways.
An interesting avenue of research is also the presence of an acto-myosin motor in the nucleus, and the possible role of Rho GTPases in controlling this interaction. Many isoforms of motor protein myosins, including myosins I, II, V, VI, X, XVI, and XVIII have been localized to the nucleus and linked to gene expression processes (reviewed in ref. Citation28). Rho GTPases may regulate myosins by controlling their phosphorylation status, which has an important role in regulating acto-myosin contractility. ROCK II, which is an effector protein of RhoA, can regulate myosin activity by phosphorylating myosin light chain phosphatase (MLCP). This inhibits MLCP activity and enhances phosphorylation status of regulatory myosin light chain (MLC), which in turn enhances actin binding and actin-dependent ATPase activity of myosin II (reviewed in ref. Citation146). Also PAKs, downstream targets of Rac1 and Cdc42 regulate myosin phosphorylation.Citation147 NMI is the best-studied nuclear myosin, and as stated above has been linked with actin to transcriptionCitation19,Citation23,Citation26 and DNA movement tasks.Citation30,Citation31 Nuclear myosin II has been linked to specific gene transcription by its association with the ICAM-1 gene promoter.Citation84 Myosin Va has been detected in nuclear speckles, which suggests a role in RNA splicing.Citation148 Myosins Vb, in contrast, localizes to the nucleolus together with actin and pol I, proposing a role in rRNA transcription.Citation149 Finally, myosin VI, together with NMI, seems to participate in transcription by pol II.Citation150 Collectively, these findings emphasize the importance of nuclear myosins in gene expression processes, and highlight the need to understand their nuclear regulation, perhaps by Rho GTPases.
However, it must be remembered that, at the moment, there is very little experimental evidence available that would actually link any of the Rho GTPases directly to nuclear actin. Their role in regulating transcription through MKL1-SRF and Hippo-pathways is obvious, but new approaches must be developed to link them via ABPs to nuclear actin-dependent transcription. It is nevertheless interesting to note that more and more cytoplasmic components are not only found in the nucleus, but also functionally linked to essential genome-related tasks. Actin is of course the great example of this. This notion forces us to conclude that many of the signaling pathways and processes that operate in the cytoplasm may also take place in the nucleus, and that many traditional cytoplasmic components might have totally different, and novel, functions in the nuclear compartment.
Abbreviations: | ||
ABP | = | Actin Binding Protein |
APC | = | Adenomatous Polyposis Coli |
AR | = | Androgen Receptor |
Arp | = | Actin related protein |
BAF | = | BRG1-Associated Factor |
BRG1 | = | Brahma-Related Gene 1 |
ECM | = | Extra Cellular Matrix |
EMT | = | Epithelial Mesenchymal Transition |
ERα | = | Estrogen Receptor alpha |
F-actin | = | Filamentous actin |
FHOD1 | = | Formin Homology 2 Domain containing protein 1 |
G-actin | = | Globular actin |
GAP | = | GTPase-Activating Protein |
GDI | = | Guanine nucleotide Dissociation Inhibitor |
GEF | = | Guanine nucleotide Exchange Factor |
HAT | = | Histone Acetyl Transferase |
hnRNP | = | heterogeneous nuclear Ribonucleoprotein |
IQGAP | = | IQ motif containing GTPase Activating Protein |
Lats1 | = | Large tumour suppressor homologue 1 |
LIMK | = | LIM Kinase |
MAP | = | Mitogen Activated Protein |
mDia | = | mouse Diaphanous homolog |
MKL1 | = | Megakaryoblastic Leukemia 1 |
MLC | = | Myosin Light Chain |
MLCP | = | Myosin Light Chain Phosphatase |
Mst1 | = | Mammalian STE20-like protein kinase 1 |
MRTF-A | = | Myocardin Related Transcription Factor-A |
NLS | = | Nuclear Localization Signal |
NMI | = | Nuclear Myosin I |
N-WASp | = | Neural-Wiscott-Aldrich Syndrome protein |
PAK1 | = | p21-Activated Kinase 1 |
PI | = | Phosphoinositide |
pol | = | RNA polymerase |
PSF-NonO | = | Polypyrimidine-tract-binding-protein-associated Splicing Factor-Non-Pou-domain octamer-binding protein/p54 |
ROCK | = | Rho Kinase |
SRF | = | Serum Response Factor |
TAZ | = | Transcriptional co-activator with PDZ-binding motif |
TEAD | = | TEA Domain family member |
TGF-β | = | Ttransforming Growth Factor-beta |
Utr | = | Utrophin |
WASp | = | Wiscott-Aldrich Syndrome protein |
WAVE | = | WASp family verprolin homologous protein |
YAP | = | Yes-associated protein |
Disclosure of Potential Conflicts of Interest
No potential conflicts of interest were closed.
Acknowledgments
We apologize to those authors whose data we have not been able to cite due to space limitations. We thank members of the Vartiainen-lab for critical comments on the manuscript. The work in the lab of M.K.V. is funded by Academy of Finland, ERC Starting grant, and Sigrid Juselius foundation. E.K.R. is funded by the Viikki Doctoral Program in Molecular Biosciences.
References
- Ridley AJ. Life at the leading edge. Cell 2011; 145:1012 - 22; http://dx.doi.org/10.1016/j.cell.2011.06.010; PMID: 21703446
- Huet G, Skarp KP, Vartiainen MK. Nuclear actin levels as an important transcriptional switch. Transcription 2012; 3:226 - 30; http://dx.doi.org/10.4161/trns.21062; PMID: 22771994
- Visa N, Percipalle P. Nuclear functions of actin. Cold Spring Harb Perspect Biol 2010; 2:a000620; http://dx.doi.org/10.1101/cshperspect.a000620; PMID: 20452941
- Vartiainen MK, Guettler S, Larijani B, Treisman R. Nuclear actin regulates dynamic subcellular localization and activity of the SRF cofactor MAL. Science 2007; 316:1749 - 52; http://dx.doi.org/10.1126/science.1141084; PMID: 17588931
- Miralles F, Posern G, Zaromytidou AI, Treisman R. Actin dynamics control SRF activity by regulation of its coactivator MAL. Cell 2003; 113:329 - 42; http://dx.doi.org/10.1016/S0092-8674(03)00278-2; PMID: 12732141
- Sotiropoulos A, Gineitis D, Copeland J, Treisman R. Signal-regulated activation of serum response factor is mediated by changes in actin dynamics. Cell 1999; 98:159 - 69; http://dx.doi.org/10.1016/S0092-8674(00)81011-9; PMID: 10428028
- Dupont S, Morsut L, Aragona M, Enzo E, Giulitti S, Cordenonsi M, Zanconato F, Le Digabel J, Forcato M, Bicciato S, et al. Role of YAP/TAZ in mechanotransduction. Nature 2011; 474:179 - 83; http://dx.doi.org/10.1038/nature10137; PMID: 21654799
- Wada K, Itoga K, Okano T, Yonemura S, Sasaki H. Hippo pathway regulation by cell morphology and stress fibers. Development 2011; 138:3907 - 14; http://dx.doi.org/10.1242/dev.070987; PMID: 21831922
- Aragona M, Panciera T, Manfrin A, Giulitti S, Michielin F, Elvassore N, Dupont S, Piccolo S. A mechanical checkpoint controls multicellular growth through YAP/TAZ regulation by actin-processing factors. Cell 2013; 154:1047 - 59; http://dx.doi.org/10.1016/j.cell.2013.07.042; PMID: 23954413
- Vartiainen MK. Nuclear actin dynamics--from form to function. FEBS Lett 2008; 582:2033 - 40; http://dx.doi.org/10.1016/j.febslet.2008.04.010; PMID: 18423404
- Archer SK, Claudianos C, Campbell HD. Evolution of the gelsolin family of actin-binding proteins as novel transcriptional coactivators. Bioessays 2005; 27:388 - 96; http://dx.doi.org/10.1002/bies.20200; PMID: 15770676
- Dopie J, Skarp KP, Rajakylä EK, Tanhuanpää K, Vartiainen MK. Active maintenance of nuclear actin by importin 9 supports transcription. Proc Natl Acad Sci U S A 2012; 109:E544 - 52; http://dx.doi.org/10.1073/pnas.1118880109; PMID: 22323606
- McDonald D, Carrero G, Andrin C, de Vries G, Hendzel MJ. Nucleoplasmic beta-actin exists in a dynamic equilibrium between low-mobility polymeric species and rapidly diffusing populations. J Cell Biol 2006; 172:541 - 52; http://dx.doi.org/10.1083/jcb.200507101; PMID: 16476775
- Baarlink C, Wang H, Grosse R. Nuclear actin network assembly by formins regulates the SRF coactivator MAL. Science 2013; 340:864 - 7; http://dx.doi.org/10.1126/science.1235038; PMID: 23558171
- Belin BJ, Cimini BA, Blackburn EH, Mullins RD. Visualization of actin filaments and monomers in somatic cell nuclei. Mol Biol Cell 2013; 24:982 - 94; http://dx.doi.org/10.1091/mbc.E12-09-0685; PMID: 23447706
- Zhao K, Wang W, Rando OJ, Xue Y, Swiderek K, Kuo A, Crabtree GR. Rapid and phosphoinositol-dependent binding of the SWI/SNF-like BAF complex to chromatin after T lymphocyte receptor signaling. Cell 1998; 95:625 - 36; http://dx.doi.org/10.1016/S0092-8674(00)81633-5; PMID: 9845365
- Fenn S, Breitsprecher D, Gerhold CB, Witte G, Faix J, Hopfner KP. Structural biochemistry of nuclear actin-related proteins 4 and 8 reveals their interaction with actin. EMBO J 2011; 30:2153 - 66; http://dx.doi.org/10.1038/emboj.2011.118; PMID: 21499228
- Kapoor P, Chen M, Winkler DD, Luger K, Shen X. Evidence for monomeric actin function in INO80 chromatin remodeling. Nat Struct Mol Biol 2013; 20:426 - 32; http://dx.doi.org/10.1038/nsmb.2529; PMID: 23524535
- Fomproix N, Percipalle P. An actin-myosin complex on actively transcribing genes. Exp Cell Res 2004; 294:140 - 8; http://dx.doi.org/10.1016/j.yexcr.2003.10.028; PMID: 14980509
- Hofmann WA, Stojiljkovic L, Fuchsova B, Vargas GM, Mavrommatis E, Philimonenko V, Kysela K, Goodrich JA, Lessard JL, Hope TJ, et al. Actin is part of pre-initiation complexes and is necessary for transcription by RNA polymerase II. Nat Cell Biol 2004; 6:1094 - 101; http://dx.doi.org/10.1038/ncb1182; PMID: 15502823
- Hu P, Wu S, Hernandez N. A role for beta-actin in RNA polymerase III transcription. Genes Dev 2004; 18:3010 - 5; http://dx.doi.org/10.1101/gad.1250804; PMID: 15574586
- Kukalev A, Nord Y, Palmberg C, Bergman T, Percipalle P. Actin and hnRNP U cooperate for productive transcription by RNA polymerase II. Nat Struct Mol Biol 2005; 12:238 - 44; http://dx.doi.org/10.1038/nsmb904; PMID: 15711563
- Philimonenko VV, Zhao J, Iben S, Dingová H, Kyselá K, Kahle M, Zentgraf H, Hofmann WA, de Lanerolle P, Hozák P, et al. Nuclear actin and myosin I are required for RNA polymerase I transcription. Nat Cell Biol 2004; 6:1165 - 72; http://dx.doi.org/10.1038/ncb1190; PMID: 15558034
- Qi T, Tang W, Wang L, Zhai L, Guo L, Zeng X. G-actin participates in RNA polymerase II-dependent transcription elongation by recruiting positive transcription elongation factor b (P-TEFb). J Biol Chem 2011; 286:15171 - 81; http://dx.doi.org/10.1074/jbc.M110.184374; PMID: 21378166
- Obrdlik A, Kukalev A, Louvet E, Farrants AK, Caputo L, Percipalle P. The histone acetyltransferase PCAF associates with actin and hnRNP U for RNA polymerase II transcription. Mol Cell Biol 2008; 28:6342 - 57; http://dx.doi.org/10.1128/MCB.00766-08; PMID: 18710935
- Ye J, Zhao J, Hoffmann-Rohrer U, Grummt I. Nuclear myosin I acts in concert with polymeric actin to drive RNA polymerase I transcription. Genes Dev 2008; 22:322 - 30; http://dx.doi.org/10.1101/gad.455908; PMID: 18230700
- Miyamoto K, Pasque V, Jullien J, Gurdon JB. Nuclear actin polymerization is required for transcriptional reprogramming of Oct4 by oocytes. Genes Dev 2011; 25:946 - 58; http://dx.doi.org/10.1101/gad.615211; PMID: 21536734
- de Lanerolle P, Serebryannyy L. Nuclear actin and myosins: life without filaments. Nat Cell Biol 2011; 13:1282 - 8; http://dx.doi.org/10.1038/ncb2364; PMID: 22048410
- Krendel M, Mooseker MS. Myosins: tails (and heads) of functional diversity. Physiology (Bethesda) 2005; 20:239 - 51; http://dx.doi.org/10.1152/physiol.00014.2005; PMID: 16024512
- Chuang CH, Carpenter AE, Fuchsova B, Johnson T, de Lanerolle P, Belmont AS. Long-range directional movement of an interphase chromosome site. Curr Biol 2006; 16:825 - 31; http://dx.doi.org/10.1016/j.cub.2006.03.059; PMID: 16631592
- Mehta IS, Amira M, Harvey AJ, Bridger JM. Rapid chromosome territory relocation by nuclear motor activity in response to serum removal in primary human fibroblasts. Genome Biol 2010; 11:R5; http://dx.doi.org/10.1186/gb-2010-11-1-r5; PMID: 20070886
- Pollard TD, Borisy GG. Cellular motility driven by assembly and disassembly of actin filaments. Cell 2003; 112:453 - 65; http://dx.doi.org/10.1016/S0092-8674(03)00120-X; PMID: 12600310
- Arber S, Barbayannis FA, Hanser H, Schneider C, Stanyon CA, Bernard O, Caroni P. Regulation of actin dynamics through phosphorylation of cofilin by LIM-kinase. Nature 1998; 393:805 - 9; http://dx.doi.org/10.1038/31729; PMID: 9655397
- Ohta Y, Nishida E, Sakai H, Miyamoto E. Dephosphorylation of cofilin accompanies heat shock-induced nuclear accumulation of cofilin. J Biol Chem 1989; 264:16143 - 8; PMID: 2777782
- Ono S, Abe H, Nagaoka R, Obinata T. Colocalization of ADF and cofilin in intranuclear actin rods of cultured muscle cells. J Muscle Res Cell Motil 1993; 14:195 - 204; http://dx.doi.org/10.1007/BF00115454; PMID: 8315023
- Samstag Y, Eckerskorn C, Wesselborg S, Henning S, Wallich R, Meuer SC. Costimulatory signals for human T-cell activation induce nuclear translocation of pp19/cofilin. Proc Natl Acad Sci U S A 1994; 91:4494 - 8; http://dx.doi.org/10.1073/pnas.91.10.4494; PMID: 8183936
- Pendleton A, Pope B, Weeds A, Koffer A. Latrunculin B or ATP depletion induces cofilin-dependent translocation of actin into nuclei of mast cells. J Biol Chem 2003; 278:14394 - 400; http://dx.doi.org/10.1074/jbc.M206393200; PMID: 12566455
- Kwak IH, Kim HS, Choi OR, Ryu MS, Lim IK. Nuclear accumulation of globular actin as a cellular senescence marker. Cancer Res 2004; 64:572 - 80; http://dx.doi.org/10.1158/0008-5472.CAN-03-1856; PMID: 14744771
- Bernstein BW, Chen H, Boyle JA, Bamburg JR. Formation of actin-ADF/cofilin rods transiently retards decline of mitochondrial potential and ATP in stressed neurons. Am J Physiol Cell Physiol 2006; 291:C828 - 39; http://dx.doi.org/10.1152/ajpcell.00066.2006; PMID: 16738008
- Munsie LN, Desmond CR, Truant R. Cofilin nuclear-cytoplasmic shuttling affects cofilin-actin rod formation during stress. J Cell Sci 2012; 125:3977 - 88; http://dx.doi.org/10.1242/jcs.097667; PMID: 22623727
- Obrdlik A, Percipalle P. The F-actin severing protein cofilin-1 is required for RNA polymerase II transcription elongation. Nucleus 2011; 2:72 - 9; http://dx.doi.org/10.4161/nucl.2.1.14508; PMID: 21647301
- Faix J, Grosse R. Staying in shape with formins. Dev Cell 2006; 10:693 - 706; http://dx.doi.org/10.1016/j.devcel.2006.05.001; PMID: 16740473
- Shao J, Welch WJ, Diprospero NA, Diamond MI. Phosphorylation of profilin by ROCK1 regulates polyglutamine aggregation. Mol Cell Biol 2008; 28:5196 - 208; http://dx.doi.org/10.1128/MCB.00079-08; PMID: 18573880
- Di Paolo G, De Camilli P. Phosphoinositides in cell regulation and membrane dynamics. Nature 2006; 443:651 - 7; http://dx.doi.org/10.1038/nature05185; PMID: 17035995
- Lassing I, Lindberg U. Specific interaction between phosphatidylinositol 4,5-bisphosphate and profilactin. Nature 1985; 314:472 - 4; http://dx.doi.org/10.1038/314472a0; PMID: 2984579
- Stüven T, Hartmann E, Görlich D. Exportin 6: a novel nuclear export receptor that is specific for profilin.actin complexes. EMBO J 2003; 22:5928 - 40; http://dx.doi.org/10.1093/emboj/cdg565; PMID: 14592989
- Söderberg E, Hessle V, von Euler A, Visa N. Profilin is associated with transcriptionally active genes. Nucleus 2012; 3:290 - 9; http://dx.doi.org/10.4161/nucl.20327; PMID: 22572953
- Skare P, Kreivi JP, Bergström A, Karlsson R. Profilin I colocalizes with speckles and Cajal bodies: a possible role in pre-mRNA splicing. Exp Cell Res 2003; 286:12 - 21; http://dx.doi.org/10.1016/S0014-4827(03)00102-2; PMID: 12729790
- Lederer M, Jockusch BM, Rothkegel M. Profilin regulates the activity of p42POP, a novel Myb-related transcription factor. J Cell Sci 2005; 118:331 - 41; http://dx.doi.org/10.1242/jcs.01618; PMID: 15615774
- Birbach A, Verkuyl JM, Matus A. Reversible, activity-dependent targeting of profilin to neuronal nuclei. Exp Cell Res 2006; 312:2279 - 87; http://dx.doi.org/10.1016/j.yexcr.2006.03.026; PMID: 16716297
- Rotty JD, Wu C, Bear JE. New insights into the regulation and cellular functions of the ARP2/3 complex. Nat Rev Mol Cell Biol 2013; 14:7 - 12; http://dx.doi.org/10.1038/nrm3492; PMID: 23212475
- Suetsugu S, Takenawa T. Translocation of N-WASP by nuclear localization and export signals into the nucleus modulates expression of HSP90. J Biol Chem 2003; 278:42515 - 23; http://dx.doi.org/10.1074/jbc.M302177200; PMID: 12871950
- Mizutani K, Suetsugu S, Takenawa T. FBP11 regulates nuclear localization of N-WASP and inhibits N-WASP-dependent microspike formation. Biochem Biophys Res Commun 2004; 313:468 - 74; http://dx.doi.org/10.1016/j.bbrc.2003.11.139; PMID: 14697212
- Yoo Y, Wu X, Guan JL. A novel role of the actin-nucleating Arp2/3 complex in the regulation of RNA polymerase II-dependent transcription. J Biol Chem 2007; 282:7616 - 23; http://dx.doi.org/10.1074/jbc.M607596200; PMID: 17220302
- Wu X, Yoo Y, Okuhama NN, Tucker PW, Liu G, Guan JL. Regulation of RNA-polymerase-II-dependent transcription by N-WASP and its nuclear-binding partners. Nat Cell Biol 2006; 8:756 - 63; http://dx.doi.org/10.1038/ncb1433; PMID: 16767080
- Ferrai C, Naum-Onganía G, Longobardi E, Palazzolo M, Disanza A, Diaz VM, Crippa MP, Scita G, Blasi F. Induction of HoxB transcription by retinoic acid requires actin polymerization. Mol Biol Cell 2009; 20:3543 - 51; http://dx.doi.org/10.1091/mbc.E09-02-0114; PMID: 19477923
- Taylor MD, Sadhukhan S, Kottangada P, Ramgopal A, Sarkar K, D’Silva S, Selvakumar A, Candotti F, Vyas YM. Nuclear role of WASp in the pathogenesis of dysregulated TH1 immunity in human Wiskott-Aldrich syndrome. Sci Transl Med 2010; 2:37ra44; http://dx.doi.org/10.1126/scitranslmed.3000813; PMID: 20574068
- Miyamoto K, Teperek M, Yusa K, Allen GE, Bradshaw CR, Gurdon JB. Nuclear Wave1 is required for reprogramming transcription in oocytes and for normal development. Science 2013; 341:1002 - 5; http://dx.doi.org/10.1126/science.1240376; PMID: 23990560
- Lammers M, Rose R, Scrima A, Wittinghofer A. The regulation of mDia1 by autoinhibition and its release by Rho*GTP. EMBO J 2005; 24:4176 - 87; http://dx.doi.org/10.1038/sj.emboj.7600879; PMID: 16292343
- Miki T, Okawa K, Sekimoto T, Yoneda Y, Watanabe S, Ishizaki T, Narumiya S. mDia2 shuttles between the nucleus and the cytoplasm through the importin-alpha/beta- and CRM1-mediated nuclear transport mechanism. J Biol Chem 2009; 284:5753 - 62; http://dx.doi.org/10.1074/jbc.M806191200; PMID: 19117945
- Ménard I, Gervais FG, Nicholson DW, Roy S. Caspase-3 cleaves the formin-homology-domain-containing protein FHOD1 during apoptosis to generate a C-terminal fragment that is targeted to the nucleolus. Apoptosis 2006; 11:1863 - 76; http://dx.doi.org/10.1007/s10495-006-0087-8; PMID: 17013756
- Okada K, Bartolini F, Deaconescu AM, Moseley JB, Dogic Z, Grigorieff N, Gundersen GG, Goode BL. Adenomatous polyposis coli protein nucleates actin assembly and synergizes with the formin mDia1. J Cell Biol 2010; 189:1087 - 96; http://dx.doi.org/10.1083/jcb.201001016; PMID: 20566685
- Neufeld KL, Zhang F, Cullen BR, White RL. APC-mediated downregulation of beta-catenin activity involves nuclear sequestration and nuclear export. EMBO Rep 2000; 1:519 - 23; http://dx.doi.org/10.1093/embo-reports/kvd117; PMID: 11263497
- Sudhaharan T, Goh WI, Sem KP, Lim KB, Bu W, Ahmed S. Rho GTPase Cdc42 is a direct interacting partner of Adenomatous Polyposis Coli protein and can alter its cellular localization. PLoS One 2011; 6:e16603; http://dx.doi.org/10.1371/journal.pone.0016603; PMID: 21311754
- Brandt DT, Grosse R. Get to grips: steering local actin dynamics with IQGAPs. EMBO Rep 2007; 8:1019 - 23; http://dx.doi.org/10.1038/sj.embor.7401089; PMID: 17972901
- Johnson M, Sharma M, Brocardo MG, Henderson BR. IQGAP1 translocates to the nucleus in early S-phase and contributes to cell cycle progression after DNA replication arrest. Int J Biochem Cell Biol 2011; 43:65 - 73; http://dx.doi.org/10.1016/j.biocel.2010.09.014; PMID: 20883816
- Johnson MA, Sharma M, Mok MT, Henderson BR. Stimulation of in vivo nuclear transport dynamics of actin and its co-factors IQGAP1 and Rac1 in response to DNA replication stress. Biochim Biophys Acta 2013; 1833:2334 - 47; http://dx.doi.org/10.1016/j.bbamcr.2013.06.002; PMID: 23770048
- Feng Y, Walsh CA. The many faces of filamin: a versatile molecular scaffold for cell motility and signalling. Nat Cell Biol 2004; 6:1034 - 8; http://dx.doi.org/10.1038/ncb1104-1034; PMID: 15516996
- Bokoch GM. Biology of the p21-activated kinases. Annu Rev Biochem 2003; 72:743 - 81; http://dx.doi.org/10.1146/annurev.biochem.72.121801.161742; PMID: 12676796
- Loy CJ, Sim KS, Yong EL. Filamin-A fragment localizes to the nucleus to regulate androgen receptor and coactivator functions. Proc Natl Acad Sci U S A 2003; 100:4562 - 7; http://dx.doi.org/10.1073/pnas.0736237100; PMID: 12682292
- Yue J, Wang Q, Lu H, Brenneman M, Fan F, Shen Z. The cytoskeleton protein filamin-A is required for an efficient recombinational DNA double strand break repair. Cancer Res 2009; 69:7978 - 85; http://dx.doi.org/10.1158/0008-5472.CAN-09-2177; PMID: 19808958
- Deng W, Lopez-Camacho C, Tang JY, Mendoza-Villanueva D, Maya-Mendoza A, Jackson DA, Shore P. Cytoskeletal protein filamin A is a nucleolar protein that suppresses ribosomal RNA gene transcription. Proc Natl Acad Sci U S A 2012; 109:1524 - 9; http://dx.doi.org/10.1073/pnas.1107879109; PMID: 22307607
- Huff T, Rosorius O, Otto AM, Müller CS, Ballweber E, Hannappel E, Mannherz HG. Nuclear localisation of the G-actin sequestering peptide thymosin beta4. J Cell Sci 2004; 117:5333 - 41; http://dx.doi.org/10.1242/jcs.01404; PMID: 15466884
- Babakov VN, Petukhova OA, Turoverova LV, Kropacheva IV, Tentler DG, Bolshakova AV, Podolskaya EP, Magnusson KE, Pinaev GP. RelA/NF-kappaB transcription factor associates with alpha-actinin-4. Exp Cell Res 2008; 314:1030 - 8; http://dx.doi.org/10.1016/j.yexcr.2007.12.001; PMID: 18215660
- Ito M, Iguchi K, Usui S, Hirano K. Overexpression of thymosin beta4 increases pseudopodia formation in LNCaP prostate cancer cells. Biol Pharm Bull 2009; 32:1101 - 4; http://dx.doi.org/10.1248/bpb.32.1101; PMID: 19483323
- Martinez JJ, Hultgren SJ. Requirement of Rho-family GTPases in the invasion of Type 1-piliated uropathogenic Escherichia coli. Cell Microbiol 2002; 4:19 - 28; http://dx.doi.org/10.1046/j.1462-5822.2002.00166.x; PMID: 11856170
- Irvine RF. Nuclear lipid signalling. Nat Rev Mol Cell Biol 2003; 4:349 - 60; http://dx.doi.org/10.1038/nrm1100; PMID: 12728269
- Rando OJ, Zhao K, Janmey P, Crabtree GR. Phosphatidylinositol-dependent actin filament binding by the SWI/SNF-like BAF chromatin remodeling complex. Proc Natl Acad Sci U S A 2002; 99:2824 - 9; http://dx.doi.org/10.1073/pnas.032662899; PMID: 11880634
- Barlow CA, Laishram RS, Anderson RA. Nuclear phosphoinositides: a signaling enigma wrapped in a compartmental conundrum. Trends Cell Biol 2010; 20:25 - 35; http://dx.doi.org/10.1016/j.tcb.2009.09.009; PMID: 19846310
- Nishimura K, Ting HJ, Harada Y, Tokizane T, Nonomura N, Kang HY, Chang HC, Yeh S, Miyamoto H, Shin M, et al. Modulation of androgen receptor transactivation by gelsolin: a newly identified androgen receptor coregulator. Cancer Res 2003; 63:4888 - 94; PMID: 12941811
- Ting HJ, Yeh S, Nishimura K, Chang C. Supervillin associates with androgen receptor and modulates its transcriptional activity. Proc Natl Acad Sci U S A 2002; 99:661 - 6; http://dx.doi.org/10.1073/pnas.022469899; PMID: 11792840
- Lee YH, Campbell HD, Stallcup MR. Developmentally essential protein flightless I is a nuclear receptor coactivator with actin binding activity. Mol Cell Biol 2004; 24:2103 - 17; http://dx.doi.org/10.1128/MCB.24.5.2103-2117.2004; PMID: 14966289
- Nebl G, Meuer SC, Samstag Y. Dephosphorylation of serine 3 regulates nuclear translocation of cofilin. J Biol Chem 1996; 271:26276 - 80; http://dx.doi.org/10.1074/jbc.271.42.26276; PMID: 8824278
- Li Q, Sarna SK. Nuclear myosin II regulates the assembly of preinitiation complex for ICAM-1 gene transcription. Gastroenterology 2009; 137:1051 - 60, e1-3; http://dx.doi.org/10.1053/j.gastro.2009.03.040; PMID: 19328794
- Ward ME, Wu JY, Rao Y. Visualization of spatially and temporally regulated N-WASP activity during cytoskeletal reorganization in living cells. Proc Natl Acad Sci U S A 2004; 101:970 - 4; http://dx.doi.org/10.1073/pnas.0306258101; PMID: 14732696
- Dubash AD, Guilluy C, Srougi MC, Boulter E, Burridge K, García-Mata R. The small GTPase RhoA localizes to the nucleus and is activated by Net1 and DNA damage signals. PLoS One 2011; 6:e17380; http://dx.doi.org/10.1371/journal.pone.0017380; PMID: 21390328
- Michaelson D, Abidi W, Guardavaccaro D, Zhou M, Ahearn I, Pagano M, Philips MR. Rac1 accumulates in the nucleus during the G2 phase of the cell cycle and promotes cell division. J Cell Biol 2008; 181:485 - 96; http://dx.doi.org/10.1083/jcb.200801047; PMID: 18443222
- Michaelson D, Silletti J, Murphy G, D’Eustachio P, Rush M, Philips MR. Differential localization of Rho GTPases in live cells: regulation by hypervariable regions and RhoGDI binding. J Cell Biol 2001; 152:111 - 26; http://dx.doi.org/10.1083/jcb.152.1.111; PMID: 11149925
- Lanning CC, Ruiz-Velasco R, Williams CL. Novel mechanism of the co-regulation of nuclear transport of SmgGDS and Rac1. J Biol Chem 2003; 278:12495 - 506; http://dx.doi.org/10.1074/jbc.M211286200; PMID: 12551911
- Williams CL. The polybasic region of Ras and Rho family small GTPases: a regulator of protein interactions and membrane association and a site of nuclear localization signal sequences. Cell Signal 2003; 15:1071 - 80; http://dx.doi.org/10.1016/S0898-6568(03)00098-6; PMID: 14575862
- Görlich D, Kutay U. Transport between the cell nucleus and the cytoplasm. Annu Rev Cell Dev Biol 1999; 15:607 - 60; http://dx.doi.org/10.1146/annurev.cellbio.15.1.607; PMID: 10611974
- Kawashima T, Bao YC, Nomura Y, Moon Y, Tonozuka Y, Minoshima Y, Hatori T, Tsuchiya A, Kiyono M, Nosaka T, et al. Rac1 and a GTPase-activating protein, MgcRacGAP, are required for nuclear translocation of STAT transcription factors. J Cell Biol 2006; 175:937 - 46; http://dx.doi.org/10.1083/jcb.200604073; PMID: 17178910
- Kawashima T, Bao YC, Minoshima Y, Nomura Y, Hatori T, Hori T, Fukagawa T, Fukada T, Takahashi N, Nosaka T, et al. A Rac GTPase-activating protein, MgcRacGAP, is a nuclear localizing signal-containing nuclear chaperone in the activation of STAT transcription factors. Mol Cell Biol 2009; 29:1796 - 813; http://dx.doi.org/10.1128/MCB.01423-08; PMID: 19158271
- Schmidt A, Hall A. The Rho exchange factor Net1 is regulated by nuclear sequestration. J Biol Chem 2002; 277:14581 - 8; http://dx.doi.org/10.1074/jbc.M111108200; PMID: 11839749
- Srougi MC, Burridge K. The nuclear guanine nucleotide exchange factors Ect2 and Net1 regulate RhoB-mediated cell death after DNA damage. PLoS One 2011; 6:e17108; http://dx.doi.org/10.1371/journal.pone.0017108; PMID: 21373644
- Tatsumoto T, Xie X, Blumenthal R, Okamoto I, Miki T. Human ECT2 is an exchange factor for Rho GTPases, phosphorylated in G2/M phases, and involved in cytokinesis. J Cell Biol 1999; 147:921 - 8; http://dx.doi.org/10.1083/jcb.147.5.921; PMID: 10579713
- Xu J, Zhou X, Wang J, Li Z, Kong X, Qian J, Hu Y, Fang JY. RhoGAPs attenuate cell proliferation by direct interaction with p53 tetramerization domain. Cell Rep 2013; 3:1526 - 38; http://dx.doi.org/10.1016/j.celrep.2013.04.017; PMID: 23684608
- El Marzouk S, Schultz-Norton JR, Likhite VS, McLeod IX, Yates JR, Nardulli AM. Rho GDP dissociation inhibitor alpha interacts with estrogen receptor alpha and influences estrogen responsiveness. J Mol Endocrinol 2007; 39:249 - 59; http://dx.doi.org/10.1677/JME-07-0055; PMID: 17909265
- Ambrosino C, Tarallo R, Bamundo A, Cuomo D, Franci G, Nassa G, Paris O, Ravo M, Giovane A, Zambrano N, et al. Identification of a hormone-regulated dynamic nuclear actin network associated with estrogen receptor alpha in human breast cancer cell nuclei. Mol Cell Proteomics 2010; 9:1352 - 67; http://dx.doi.org/10.1074/mcp.M900519-MCP200; PMID: 20308691
- Bhowmick NA, Ghiassi M, Aakre M, Brown K, Singh V, Moses HL. TGF-beta-induced RhoA and p160ROCK activation is involved in the inhibition of Cdc25A with resultant cell-cycle arrest. Proc Natl Acad Sci U S A 2003; 100:15548 - 53; http://dx.doi.org/10.1073/pnas.2536483100; PMID: 14657354
- Tanaka T, Nishimura D, Wu RC, Amano M, Iso T, Kedes L, Nishida H, Kaibuchi K, Hamamori Y. Nuclear Rho kinase, ROCK2, targets p300 acetyltransferase. J Biol Chem 2006; 281:15320 - 9; http://dx.doi.org/10.1074/jbc.M510954200; PMID: 16574662
- Singh RR, Song C, Yang Z, Kumar R. Nuclear localization and chromatin targets of p21-activated kinase 1. J Biol Chem 2005; 280:18130 - 7; http://dx.doi.org/10.1074/jbc.M412607200; PMID: 15749698
- Lightcap CM, Kari G, Arias-Romero LE, Chernoff J, Rodeck U, Williams JC. Interaction with LC8 is required for Pak1 nuclear import and is indispensable for zebrafish development. PLoS One 2009; 4:e6025; http://dx.doi.org/10.1371/journal.pone.0006025; PMID: 19557173
- Holm C, Rayala S, Jirström K, Stål O, Kumar R, Landberg G. Association between Pak1 expression and subcellular localization and tamoxifen resistance in breast cancer patients. J Natl Cancer Inst 2006; 98:671 - 80; http://dx.doi.org/10.1093/jnci/djj185; PMID: 16705121
- Treisman R. Identification of a protein-binding site that mediates transcriptional response of the c-fos gene to serum factors. Cell 1986; 46:567 - 74; http://dx.doi.org/10.1016/0092-8674(86)90882-2; PMID: 3524858
- Sun Q, Chen G, Streb JW, Long X, Yang Y, Stoeckert CJ Jr., Miano JM. Defining the mammalian CArGome. Genome Res 2006; 16:197 - 207; http://dx.doi.org/10.1101/gr.4108706; PMID: 16365378
- Arsenian S, Weinhold B, Oelgeschläger M, Rüther U, Nordheim A. Serum response factor is essential for mesoderm formation during mouse embryogenesis. EMBO J 1998; 17:6289 - 99; http://dx.doi.org/10.1093/emboj/17.21.6289; PMID: 9799237
- Miano JM, Long X, Fujiwara K. Serum response factor: master regulator of the actin cytoskeleton and contractile apparatus. Am J Physiol Cell Physiol 2007; 292:C70 - 81; http://dx.doi.org/10.1152/ajpcell.00386.2006; PMID: 16928770
- Olson EN, Nordheim A. Linking actin dynamics and gene transcription to drive cellular motile functions. Nat Rev Mol Cell Biol 2010; 11:353 - 65; http://dx.doi.org/10.1038/nrm2890; PMID: 20414257
- Shaw PE, Schröter H, Nordheim A. The ability of a ternary complex to form over the serum response element correlates with serum inducibility of the human c-fos promoter. Cell 1989; 56:563 - 72; http://dx.doi.org/10.1016/0092-8674(89)90579-5; PMID: 2492906
- Hill CS, Treisman R. Differential activation of c-fos promoter elements by serum, lysophosphatidic acid, G proteins and polypeptide growth factors. EMBO J 1995; 14:5037 - 47; PMID: 7588632
- Gineitis D, Treisman R. Differential usage of signal transduction pathways defines two types of serum response factor target gene. J Biol Chem 2001; 276:24531 - 9; http://dx.doi.org/10.1074/jbc.M102678200; PMID: 11342553
- Geneste O, Copeland JW, Treisman R. LIM kinase and Diaphanous cooperate to regulate serum response factor and actin dynamics. J Cell Biol 2002; 157:831 - 8; http://dx.doi.org/10.1083/jcb.200203126; PMID: 12034774
- Grosse R, Copeland JW, Newsome TP, Way M, Treisman R. A role for VASP in RhoA-Diaphanous signalling to actin dynamics and SRF activity. EMBO J 2003; 22:3050 - 61; http://dx.doi.org/10.1093/emboj/cdg287; PMID: 12805219
- Wang DZ, Li S, Hockemeyer D, Sutherland L, Wang Z, Schratt G, Richardson JA, Nordheim A, Olson EN. Potentiation of serum response factor activity by a family of myocardin-related transcription factors. Proc Natl Acad Sci U S A 2002; 99:14855 - 60; http://dx.doi.org/10.1073/pnas.222561499; PMID: 12397177
- Selvaraj A, Prywes R. Megakaryoblastic leukemia-1/2, a transcriptional co-activator of serum response factor, is required for skeletal myogenic differentiation. J Biol Chem 2003; 278:41977 - 87; http://dx.doi.org/10.1074/jbc.M305679200; PMID: 14565952
- Guettler S, Vartiainen MK, Miralles F, Larijani B, Treisman R. RPEL motifs link the serum response factor cofactor MAL but not myocardin to Rho signaling via actin binding. Mol Cell Biol 2008; 28:732 - 42; http://dx.doi.org/10.1128/MCB.01623-07; PMID: 18025109
- Pawłowski R, Rajakylä EK, Vartiainen MK, Treisman R. An actin-regulated importin α/β-dependent extended bipartite NLS directs nuclear import of MRTF-A. EMBO J 2010; 29:3448 - 58; http://dx.doi.org/10.1038/emboj.2010.216; PMID: 20818336
- Mouilleron S, Langer CA, Guettler S, McDonald NQ, Treisman R. Structure of a pentavalent G-actin*MRTF-A complex reveals how G-actin controls nucleocytoplasmic shuttling of a transcriptional coactivator. Sci Signal 2011; 4:ra40; http://dx.doi.org/10.1126/scisignal.2001750; PMID: 21673315
- Lockman K, Hinson JS, Medlin MD, Morris D, Taylor JM, Mack CP. Sphingosine 1-phosphate stimulates smooth muscle cell differentiation and proliferation by activating separate serum response factor co-factors. J Biol Chem 2004; 279:42422 - 30; http://dx.doi.org/10.1074/jbc.M405432200; PMID: 15292266
- Kwon MS, Park BO, Kim HM, Kim S. Leucine-rich repeat-containing G-protein coupled receptor 5/GPR49 activates G12/13-Rho GTPase pathway. Mol Cells 2013; 36:267 - 72; http://dx.doi.org/10.1007/s10059-013-0173-z; PMID: 23912594
- Chai J, Jones MK, Tarnawski AS. Serum response factor is a critical requirement for VEGF signaling in endothelial cells and VEGF-induced angiogenesis. FASEB J 2004; 18:1264 - 6; PMID: 15180964
- Wickramasinghe SR, Alvania RS, Ramanan N, Wood JN, Mandai K, Ginty DD. Serum response factor mediates NGF-dependent target innervation by embryonic DRG sensory neurons. Neuron 2008; 58:532 - 45; http://dx.doi.org/10.1016/j.neuron.2008.03.006; PMID: 18498735
- Schmidt LJ, Duncan K, Yadav N, Regan KM, Verone AR, Lohse CM, Pop EA, Attwood K, Wilding G, Mohler JL, et al. RhoA as a mediator of clinically relevant androgen action in prostate cancer cells. Mol Endocrinol 2012; 26:716 - 35; http://dx.doi.org/10.1210/me.2011-1130; PMID: 22456196
- Gerber A, Esnault C, Aubert G, Treisman R, Pralong F, Schibler U. Blood-borne circadian signal stimulates daily oscillations in actin dynamics and SRF activity. Cell 2013; 152:492 - 503; http://dx.doi.org/10.1016/j.cell.2012.12.027; PMID: 23374345
- Parsons JT, Horwitz AR, Schwartz MA. Cell adhesion: integrating cytoskeletal dynamics and cellular tension. Nat Rev Mol Cell Biol 2010; 11:633 - 43; http://dx.doi.org/10.1038/nrm2957; PMID: 20729930
- Busche S, Descot A, Julien S, Genth H, Posern G. Epithelial cell-cell contacts regulate SRF-mediated transcription via Rac-actin-MAL signalling. J Cell Sci 2008; 121:1025 - 35; http://dx.doi.org/10.1242/jcs.014456; PMID: 18334560
- Morita T, Mayanagi T, Sobue K. Dual roles of myocardin-related transcription factors in epithelial mesenchymal transition via slug induction and actin remodeling. J Cell Biol 2007; 179:1027 - 42; http://dx.doi.org/10.1083/jcb.200708174; PMID: 18056415
- Huveneers S, Danen EH. Adhesion signaling - crosstalk between integrins, Src and Rho. J Cell Sci 2009; 122:1059 - 69; http://dx.doi.org/10.1242/jcs.039446; PMID: 19339545
- Zhao XH, Laschinger C, Arora P, Szászi K, Kapus A, McCulloch CA. Force activates smooth muscle alpha-actin promoter activity through the Rho signaling pathway. J Cell Sci 2007; 120:1801 - 9; http://dx.doi.org/10.1242/jcs.001586; PMID: 17456553
- Brandt DT, Baarlink C, Kitzing TM, Kremmer E, Ivaska J, Nollau P, Grosse R. SCAI acts as a suppressor of cancer cell invasion through the transcriptional control of beta1-integrin. Nat Cell Biol 2009; 11:557 - 68; http://dx.doi.org/10.1038/ncb1862; PMID: 19350017
- Reymond N, Im JH, Garg R, Vega FM, Borda d’Agua B, Riou P, Cox S, Valderrama F, Muschel RJ, Ridley AJ. Cdc42 promotes transendothelial migration of cancer cells through β1 integrin. J Cell Biol 2012; 199:653 - 68; http://dx.doi.org/10.1083/jcb.201205169; PMID: 23148235
- Connelly JT, Gautrot JE, Trappmann B, Tan DW, Donati G, Huck WT, Watt FM. Actin and serum response factor transduce physical cues from the microenvironment to regulate epidermal stem cell fate decisions. Nat Cell Biol 2010; 12:711 - 8; http://dx.doi.org/10.1038/ncb2074; PMID: 20581838
- Halder G, Dupont S, Piccolo S. Transduction of mechanical and cytoskeletal cues by YAP and TAZ. Nat Rev Mol Cell Biol 2012; 13:591 - 600; http://dx.doi.org/10.1038/nrm3416; PMID: 22895435
- Bennett FC, Harvey KF. Fat cadherin modulates organ size in Drosophila via the Salvador/Warts/Hippo signaling pathway. Curr Biol 2006; 16:2101 - 10; http://dx.doi.org/10.1016/j.cub.2006.09.045; PMID: 17045801
- Hamaratoglu F, Willecke M, Kango-Singh M, Nolo R, Hyun E, Tao C, Jafar-Nejad H, Halder G. The tumour-suppressor genes NF2/Merlin and Expanded act through Hippo signalling to regulate cell proliferation and apoptosis. Nat Cell Biol 2006; 8:27 - 36; http://dx.doi.org/10.1038/ncb1339; PMID: 16341207
- Paramasivam M, Sarkeshik A, Yates JR 3rd, Fernandes MJ, McCollum D. Angiomotin family proteins are novel activators of the LATS2 kinase tumor suppressor. Mol Biol Cell 2011; 22:3725 - 33; http://dx.doi.org/10.1091/mbc.E11-04-0300; PMID: 21832154
- Schlegelmilch K, Mohseni M, Kirak O, Pruszak J, Rodriguez JR, Zhou D, Kreger BT, Vasioukhin V, Avruch J, Brummelkamp TR, et al. Yap1 acts downstream of α-catenin to control epidermal proliferation. Cell 2011; 144:782 - 95; http://dx.doi.org/10.1016/j.cell.2011.02.031; PMID: 21376238
- Reddy P, Deguchi M, Cheng Y, Hsueh AJ. Actin cytoskeleton regulates Hippo signaling. PLoS One 2013; 8:e73763; http://dx.doi.org/10.1371/journal.pone.0073763; PMID: 24040060
- Sansores-Garcia L, Bossuyt W, Wada K, Yonemura S, Tao C, Sasaki H, Halder G. Modulating F-actin organization induces organ growth by affecting the Hippo pathway. EMBO J 2011; 30:2325 - 35; http://dx.doi.org/10.1038/emboj.2011.157; PMID: 21556047
- Posern G, Sotiropoulos A, Treisman R. Mutant actins demonstrate a role for unpolymerized actin in control of transcription by serum response factor. Mol Biol Cell 2002; 13:4167 - 78; http://dx.doi.org/10.1091/mbc.02-05-0068; PMID: 12475943
- Lucas EP, Khanal I, Gaspar P, Fletcher GC, Polesello C, Tapon N, Thompson BJ. The Hippo pathway polarizes the actin cytoskeleton during collective migration of Drosophila border cells. J Cell Biol 2013; 201:875 - 85; http://dx.doi.org/10.1083/jcb.201210073; PMID: 23733343
- Densham RM, O’Neill E, Munro J, König I, Anderson K, Kolch W, Olson MF. MST kinases monitor actin cytoskeletal integrity and signal via c-Jun N-terminal kinase stress-activated kinase to regulate p21Waf1/Cip1 stability. Mol Cell Biol 2009; 29:6380 - 90; http://dx.doi.org/10.1128/MCB.00116-09; PMID: 19822666
- Visser-Grieve S, Zhou Z, She YM, Huang H, Cyr TD, Xu T, Yang X. LATS1 tumor suppressor is a novel actin-binding protein and negative regulator of actin polymerization. Cell Res 2011; 21:1513 - 6; http://dx.doi.org/10.1038/cr.2011.122; PMID: 21808298
- Melendez J, Stengel K, Zhou X, Chauhan BK, Debidda M, Andreassen P, Lang RA, Zheng Y. RhoA GTPase is dispensable for actomyosin regulation but is essential for mitosis in primary mouse embryonic fibroblasts. J Biol Chem 2011; 286:15132 - 7; http://dx.doi.org/10.1074/jbc.C111.229336; PMID: 21454503
- Riento K, Ridley AJ. Rocks: multifunctional kinases in cell behaviour. Nat Rev Mol Cell Biol 2003; 4:446 - 56; http://dx.doi.org/10.1038/nrm1128; PMID: 12778124
- Sanders LC, Matsumura F, Bokoch GM, de Lanerolle P. Inhibition of myosin light chain kinase by p21-activated kinase. Science 1999; 283:2083 - 5; http://dx.doi.org/10.1126/science.283.5410.2083; PMID: 10092231
- Pranchevicius MC, Baqui MM, Ishikawa-Ankerhold HC, Lourenço EV, Leão RM, Banzi SR, dos Santos CT, Roque-Barreira MC, Espreafico EM, Larson RE. Myosin Va phosphorylated on Ser1650 is found in nuclear speckles and redistributes to nucleoli upon inhibition of transcription. Cell Motil Cytoskeleton 2008; 65:441 - 56; http://dx.doi.org/10.1002/cm.20269; PMID: 18330901
- Lindsay AJ, McCaffrey MW. Myosin Vb localises to nucleoli and associates with the RNA polymerase I transcription complex. Cell Motil Cytoskeleton 2009; 66:1057 - 72; http://dx.doi.org/10.1002/cm.20408; PMID: 19610025
- Vreugde S, Ferrai C, Miluzio A, Hauben E, Marchisio PC, Crippa MP, Bussi M, Biffo S. Nuclear myosin VI enhances RNA polymerase II-dependent transcription. Mol Cell 2006; 23:749 - 55; http://dx.doi.org/10.1016/j.molcel.2006.07.005; PMID: 16949370