Abstract
The superoxide-generating NADPH oxidase of phagocytes consists of the membrane-associated cytochrome b558 (a heterodimer of Nox2 and p22phox) and 4 cytosolic components: p47phox, p67phox, p40phox, and the small GTPase, Rac, in complex with RhoGDI. Superoxide is produced by the NADPH-driven reduction of molecular oxygen, via a redox gradient located in Nox2. Electron flow in Nox2 is initiated by interaction with cytosolic components, which translocate to the membrane, p67phox playing the central role. The participation of Rac is expressed in the following sequence: (1) Translocation of the RacGDP-RhoGDI complex to the membrane; (2) Dissociation of RacGDP from RhoGDI; (3) GDP to GTP exchange on Rac, mediated by a guanine nucleotide exchange factor; (4) Binding of RacGTP to p67phox; (5) Induction of a conformational change in p67phox, promoting interaction with Nox2. The particular involvement of Rac in NADPH oxidase assembly serves as a paradigm for signaling by Rho GTPases, in general.
Introduction
“Those who cannot remember the past are condemned to repeat it” George Santayana, Life of Reason, Reason in Common Sense
Phagocytic cells (principally, neutrophils and macrophages) destroy engulfed bacterial, fungal, and protozoan pathogens by multiple effector mechanisms. Among these, reactive oxygen species (ROS) play a paramount role, being rapidly generated in response to stimulation of a variety of membrane receptors either directly by the microorganisms or by opsonizing components. This process was coined in the older literature as the “oxidative burst.” ROS are derived from the primordial oxygen radical, superoxide (O2.-), which is produced in response to appropriate engagement of membrane receptors by a strictly regulated enzyme complex, known as the O2.--generating phagocyte NADPH oxidase (briefly, “oxidase”) (for a review, see ref. Citation1). The oxidase catalyzes the formation of O2.- by the NADPH-driven 1-electron reduction of molecular oxygen (O2). The oxidase complex is composed of a membrane-associated flavocytochrome b558, which is a heterodimer of 2 subunits, Nox2 (also known as gp91phox) and p22phox, and 5 cytosolic components, p47phox, p67phox, p40phox, the small GTPase Rac1 or Rac2, and Rho GDP dissociation inhibitor (RhoGDI) (for a review, see ref. Citation2).
The oxidase complex contains only one catalytic component, Nox2, which consists of a membrane-embedded part and a cytosolic segment, also known as the dehydrogenase region. Nox2 is harboring all redox stations responsible for the flow of electrons from NADPH to O2, namely an NADPH-binding site and non-covalently bound FAD, both present in the dehydrogenase region, and 2 hemes, bound to 2 pairs of histidines located in membrane helices. In the resting phagocyte, the membrane-bound and cytosolic entities are kept separate but part of the cytosolic components p47phox, p67phox, and p40phox exist as a large molecular weight tripartite complex, and Rac is found as a bipartite complex with RhoGDI. The role and importance of the Rac-RhoGDI complex in oxidase function will be discussed in detail in this review.
Activation of the oxidase, to yield O2.-, is the consequence of the interaction of flavocytochrome b558 with cytosolic components, a process requiring translocation of the cytosolic components to the membrane environment of the cytochrome. This process involves a complex set of novel protein—protein and protein—lipid interactions and is defined as oxidase assembly (for reviews, see refs. Citation3–Citation5). The canonical hypothesis is that the key protein - protein interaction takes place between the dehydrogenase region of Nox2 and one or more cytosolic components, resulting in a conformational change in Nox2 that initiates the electron flow. In one model of oxidase assembly, p67phox is seen as the only cytosolic component responsible for the “activating” interaction with Nox2. Because p67phox does not possess a membrane attachment signal of its own, it requires the assistance of p47phox and Rac, to be brought in contact with Nox2.Citation6,Citation7 However, the roles of p47phox and Rac in helping the association of p67phox with Nox2 are not interchangeable, as shown by the fact that under certain in vitro conditions, oxidase activation can take place in the absence of p47phox but not that of Rac.Citation8-Citation10 This review is focused on the role the small GTPase Rac in oxidase activation in phagocytic cells, the catalytic element of which is Nox2. With the discovery of non-phagocytic Noxes, a family of membrane-associated proteins with an electron carrier function and comprising the conserved structural and functional modules also present in Nox2 (Nox1, 3, 4, and 5; Duox1 and 2), the involvement of Rac in the activity of some of its members became apparentCitation5,Citation11 but will not be discussed in the present text.
Departing from the customary format, and in the spirit of Santayana’s aphorism, accent will be placed on the history of the discoveries leading to our present understanding of the involvement of Rac in oxidase assembly. Thus, false directions, wrong hypotheses, controversial issues and still unresolved questions, will be discussed, with the purpose of offering the reader a truthful rendering of the tortuous path to the presently accepted paradigms.
NADPH Oxidase Meets Reductionism
The elucidation of the mechanism of oxidase activation (assembly) was helped enormously by the introduction of an in vitro system in which components of the oxidase complex present in the membrane and cytosol of resting phagocytes, as isolated entities, could be induced to assembly into an active O2.--generating complex in the presence of critical concentrations of arachidonic acid and other long-chain unsaturated fatty acidsCitation12 or linear anionic amphiphiles, such as sodium or lithium dodecyl sulfates (SDS, LiDS)Citation13 (for a review, see ref. Citation14). An additional step forward in what became known as the “cell-free system” was the ability to activate the oxidase in a semi-recombinant assay, consisting of phagocyte membranes (or phagocyte membrane liposomes or relipidated purified cytochrome b558) and purified recombinant p47phox, p67phox and Rac (1 or 2).Citation15 The cell-free system allowed a truly quantitative assessment of the requirement for each component, the relative amounts of the components, and the effect of modifying the components (addition of ligands, mutagenesis, or posttranslational modification) for optimal oxidase assembly to occur. The semi-recombinant cell-free system revealed that only 3 cytosolic components were required for oxidase assembly in vitro (p47phox, p67phox and Rac), with RhoGDI acting as a negative modulator. A different type of cell-free system, in which oxidase assembly was achieved in the absence of an anionic amphiphilic activator, was developed later and proved to be of special value in revealing the role of Rac in oxidase function.Citation10
Prehistory: Early Indications for the Involvement of a G Protein in Oxidase Activation
The idea that some form of a GTPase (G protein) participates in the activation of the O2.--generating oxidase has multiple origins and the contemporary picture, pointing clearly at the obligatory involvement of either Rac2 or Rac1, was preceded by the pursuit of many false directions. Additional elements of confusion were introduced by the involvement of heterotrimeric G proteins in numerous membrane receptor-initiated signal transduction cascades in many cells, including phagocytes, and by the participation of Rho GTPases in cytoskeleton-dependent events in phagocytes, most of which are taking place in a close temporal relationship to the activation of the oxidase. None of these important processes are discussed in this review, which is focused on the role of the small GTPase Rac in the caudal event in the “oxidative burst,” namely the assembly of the oxidase complex.
A search of the literature revealed that the first published report clearly linking O2.- production to a G protein is that of Seifert et al.Citation16 The authors described enhancement of arachidonate-stimulated O2.- production in the cell-free system by nonhydrolysable GTP analogs, guanosine 5′-O-(3-thiotriphosphate) (GTPγS) and guanylyl imidodiphosphate (GppNHp), and inhibition by guanosine 5′-O-(2-thiodiphosphate) (GDPβS). Pretreatment of membranes with pertussis toxin did not prevent enhancement by GTP, indicating that the hypothetical G protein involved did not belong to the heterotrimeric category. The enhancing role of GTP was also found to occur with fatty acids other than arachidonic, as activators,Citation17 and with membranes of the myeloid leukemic cell line HL60.Citation18 In what appears to have been an independent study, Gabig et al.Citation19 also described enhancement of amphiphile-dependent cell-free oxidase activation by GTP and nonhydrolysable GTP analogs and its inhibition by GDP and nonhydrolysable GDP analogs; the GTP effect was unaffected by pertussis and cholera toxins, again indicating that the G protein involved was not a member of the heterotrimeric family. The authors also noted that GTP exhibited no enhancing effect on the already activated oxidase, suggesting that it played a role in the process of activation itself. In a follow-up study, a cytosolic localization for the oxidase-related G protein was proposed.Citation20 Studies confirming the likelihood of the need for a G protein for activation of the oxidase, at least as evident in cell-free studies, were also published by Ligeti et al.,Citation21,Citation22 but its molecular identity remained obscure. It is, nevertheless, of interest that a 23 kDa GTP-binding protein, present in the cytosol, was contemplated as a possible candidate.Citation22 In 2 reports, evidence was provided for the G protein having a stabilizing effect on the oxidase complex, when present during assembly, as shown by much higher recoveries of oxidase activity in the membrane fraction, after separation from cytosol, following cell-free activation in the presence of GTP.Citation23,Citation24 As in the work cited before,Citation20 a cytosolic localization was seen as likelyCitation23 and the absolute resistance to pertussis and cholera toxins was confirmed.Citation24 Thus, up to the early nineties of the 20th century, the oxidase-linked G protein remained the “Elusive Pimpernel.”Citation25
Down the Wrong Alley
The first small GTPase that was proposed to be part of the oxidase complex was Rap1A (also known as Krev-1), based on its co-purification with cytochrome b558.Citation26 It was reported that the GTP-bound form of Rap1A had a higher affinity for the cytochrome and that binding was negatively regulated by phosphorylation of Rap1A.Citation27 Attempts to enhance cell-free oxidase activation by exogenous Rap1A were not successful.Citation27 On the other hand, functional evidence for a requirement for Rap1A in an arachidonate-activated cell-free system was brought forward.Citation28 In these studies, depletion of neutrophil cytosol of certain small GTPases by a poorly-specific antibody resulted in the loss of the ability of the cytosol to support cell-free activation and this capacity was reconstituted by recombinant Rap1A. However, this seemingly convincing study suffered from a number of methodological problems. An analysis of Rap1A content in membranes of neutrophils from patients with chronic granulomatous disease (CGD), lacking cytochrome b558, revealed that expression of Rap1A was not coordinated with that of the cytochrome.Citation29 In a rare in vivo study, it was found that neutrophils of Rap1A−/− mice have diminished O2.- production in response to chemotactic and phorbol ester stimuli but the site of action of Rap1A was likely to be upstream from oxidase assembly.Citation30 Finally, an examination of O2.- production by differentiated HL60 cells attempted to provide support for the involvement of both Rap1A and Rac2 in oxidase activation, a conclusion based on the inhibition of O2.- production by both dominant negative mutants, Rac2 N17 and Rap1A N17, implying distinct roles for the 2 GTPases in oxidase activation.Citation31 The introduction of the semi-recombinant cell-free system, in which the membrane is replaced by purified cytochrome b558,Citation15 made it possible to reexamine the implication of Rap1A in oxidase assembly. It was found that there was a progressive and ultimately complete dissociation of Rap1A from cytochrome b558 in the course of the purification of the cytochromeCitation32 but such highly purified material was fully effective in cell-free oxidase activation.Citation15,Citation33 In conclusion, there is no solid evidence for the implication of Rap1A in oxidase assembly proper but a role in a signal transduction event leading to the oxidase cannot be excluded.
Rac Conquers the Center Stage
Overture
There is overwhelming evidence that Rac (1 or 2) is a sine qua non participant in the assembly and consequent activation of the O2.--generating oxidase in phagocytes. Rac1 and Rac2 were first identified by Didsbury et al.Citation34 by the isolation of cDNA clones from a differentiated human promyelocytic leukemia HL60 cells library, sharing 58% homology with Rho GTPases. The name Rac was derived from “Ras-related-C3 botulinum toxin substrate,” although Rac turned out not to be ADP-ribosylated by the toxin. Rac proteins were predicted to consist of 192 residues, and to comprise a Cys-X-X-X-COOH consensus C-terminal sequence, found in Ras GTPases. It was noted that although Rac1 and Rac2 are 92% identical, Rac1 is quite ubiquitously distributed whereas Rac2 exhibited myeloid tissue selectivity and was highly expressed in neutrophils and in differentiated HL60 cells. Basic information on Rac GTPases can be found in references Citation35 and Citation36 and data on the expression and purification of recombinant Rac, in reference Citation37. Human recombinant Rac1 protein, truncated at residue184, and binding the nonhydrolysable GTP analog, guanylyl imidodiphosphate (GMPNP), was crystallized and its structure determined.Citation38
The contribution of small GTPases to oxidase function has been the subject of numerous reviews.Citation39-Citation44 The contemporary concept of small GTPase involvement in oxidase function has the following origins: (1) The evidence presented above on the regulatory function of a GTPase (note the term “regulation” because there was no proof of an “all or none” effect); (2) The work, by many groups, on the characterization of the “cytosolic components” required for oxidase activation in the cell-free system; (3) The focused effort toward the purification and characterization of what became known as the “third cytosolic component”; and (4) Finally, the identification of Rac1 or Rac2, as the oxidase-related GTPase and of its native state as a cytosolic complex with RhoGDI.
There is more than one cytosolic component
The first indication of the molecular identity of what was later found to be the Rac-RhoGDI complex came from work having taken place in many laboratories, showing that phagocyte cytosol required for oxidase activation in the cell-free system contained more than one entity and that cooperation between these was necessary for activity. Fujita et al.Citation45 found a 50 kDa protein in guinea pig neutrophil cytosol that cooperated with a 300 kDa complex, later identified as consisting of p47phox and p67phox; the 50 kDa protein was almost certainly a Rac-RhoGDI complex. Volpp et al.Citation46 and Nunoi et al.Citation47 identified p47phox and p67phox in human neutrophil cytosol and were the first to show that these were lacking in 2 autosomal recessive forms of CGD. In the course of purification studies, Nunoi et al. noticed that cell-free activity could not be reconstituted by a mixture of p47phox and p67phox-containing fractions but the addition of a third fraction, coined neutrophil cytosolic fraction 3 (NCF-3), resulted in significant activation. The authors also noted that no form of CGD could be identified caused by a lack of NCF-3. NCF-3 was not suspected to be a G protein because of the misleading fact, originating in the work of Volpp et al.,Citation46 that the p47phox/p67phox complex was bound by a GTP-agarose matrix (an affinity found later not to be GTP-specific). A material, named SOC I, was isolated by Bolscher et al.Citation48 from human neutrophil cytosol, and found to be distinct from p47phox and p67phox and most likely identical to NCF-3. This report represented a significant advance because it was noted that full cytosolic activity could be reconstituted by a mixture of p47phox, p67phox and SOC I only in the presence of guanosine 5′-O-(3-thiotriphosphate) (GTPγS) and there was evidence for GTP acting by the intermediary of SOC I. In the course of similar attempts to identify the cytosolic components, two fractions were isolated (σ2 and σ1) which, when combined, fully reconstituted the activity of native cytosol.Citation49,Citation50 σ2 was a large molecular weight complex, binding to a variety of nucleotide affinity gels, later found to contain p47phox and p67phox, whereas σ1 was found to be a protein of close to 50 kDa in size that possessed the remarkable property of also being present in the cytosol of nonphagocytic cells, such as thymus, lymph nodes, a myeloma cell line, and brain. Two lessons were learned from these studies: that the 50 kDa cytosolic component was or comprised an ubiquitous protein and that, paradoxically, though to be identified in the future as a GTPase, it did not bind to GTP affinity gels.
The “third cytosolic component” is purified
The turning point in these studies, which were, mostly, performed in isolation from those looking specifically for the elusive G protein, was the purification to near homogeneity of σ1 from the cytosol of guinea pig macrophages and of HL60 cells (independently of their state of differentiation).Citation51 Originally thought to be a single protein of 46 kDa, it was shown to be a heterodimer composed of proteins 22 and 24 kDa in size and it was suggested that one of the 2 proteins was a small GTPase. The 22 kDa and 24 kDa proteins of the dimer could be separated by 1% sodium cholate; upon testing of individual fractions, cell-free activity was supported only by the 22 kDa protein.Citation52 A dimer fully functional in the cell-free assay could be reconstituted by removal of the detergent.Citation52
Identification of the 21–22 kDa protein as Rac
The 21–22 kDa protein was identified as Rac1, when purified from macrophage cytosolCitation32 and as Rac2, when derived from neutrophil cytosol.Citation53,Citation54 The definitive identification of Rac1 was based on amino acid sequencing of peptides derived from the σ1 dimerCitation32 and from the 2 components of the dimer separated by reverse-phase chromatography.Citation52 This comprised also the identification of RhoGDI as the 24 kDa component of σ1.Citation52 The final identification of Rac2 was based on the sequencing of peptides derived from the 22 kDa protein purified from neutrophil cytosol.Citation53 These important findings were confirmed by other investigators by the isolation of a 21–22 kDa protein, participating in cell-free oxidase activation, from the cytosol of differentiated HL60 cellsCitation55 and neutrophils,Citation56 and its identification as Rac2 by the sequencing of peptides derived from the protein. The identification of the 24 kDa protein as RhoGDI was also confirmed, based on sequencing of peptides from the respective band blotted from SDS-PAGE.Citation56 The sequencing data were complemented by immunologic studies demonstrating that the 21–22 kDa band reacted only with anti-Rac antibodies and not with antibodies specific for Ras, Rap1, CDC42Hs, and RhoA.Citation32,Citation52,Citation53,Citation55 The RhoGDI nature of the 24 kDa band was also confirmed by its recognition by an anti-RhoGDI antibody.Citation52,Citation54 Finally, E. coli-derived recombinant Rac1Citation32 and Rac2Citation56 were capable of supporting cell-free oxidase activation in spite of the fact that these proteins were not subject to post-translational processing, which consists of prenylation (geranylgeranylation), carboxyl methylation, and removal of residues 190–192. Post-translationally processed recombinant Rac1 and Rac2, produced in insect cells, were active in cell-free oxidase activation.Citation57 The use of not post-translationally processed (nonprenylated) vs. post-translationally processed (prenylated) Rac in oxidase studies is an important issue in the light of the key role of prenylation in the binding of Rac to the plasma membrane.Citation58 Both Rac1 and Rac2 are the subject of numerous phagocyte oxidase-related studies; it is commonly accepted that oxidase activation in circulating neutrophilsCitation52 implicates Rac2 whereas oxidase activation in macrophagesCitation32 and circulating monocytesCitation59 involves Rac1 but subtle species differences do occur, which became evident in knockout models. In a broader context, it should be noted that the ability of purified or recombinant Rac to support oxidase activation represented the first rigorously quantifiable enzymatic assay for a small GTPase.
p67phox is the Binding Partner of Rac in Oxidase Assembly
If there is one certainty about the oxidase component serving as target for Rac, it is that it is p67phox. This was first established in a groundbreaking paper by Diekmann et al.,Citation60 demonstrating the binding of recombinant Rac1 to recombinant p67phox (but not to p47phox) in a pull-down assay. The binding site for Rac on p67phox was located somewhere upstream from residue 199; thus, excluding the participation of the 2 Src homology 3 (SH3) domains and of the canonical “activation domain” (for a review of functional domains in p67phox, see refs. Citation2–Citation5). Binding was optimal when Rac was in the GTP-bound form and involved residues in the N-terminmal effector switch I region, as shown by the lack of binding of Rac mutants T35A, D38A, and Y40K; the same Rac mutants were unable to support oxidase activity in the cell-free system. In a follow-up paper, the importance of an N-terminal domain (residues 22–45) was confirmed and a second downstream domain (residues 143–175) was found to participate in binding to p67phox, maximal binding requiring cooperation between the N- and C-terminal domains.Citation61 The claim that p67phox also binds CDC42HsCitation62 was not confirmed by later studies. Another uncorroborated claim is that Rac2 interacts with p67phox with much higher affinity than Rac1, a result which might have been due to the use of the constitutively active Rac G12V mutant for comparison.Citation63 A Q61L mutation in Rac2, also leading to a constitutively active state, was shown to enhance its ability to support oxidase activation and to compensate for the loss of this ability, caused by simultaneous T35A and D38A mutations, known to prevent binding to p67phox.Citation64 By the ingenious use of Rac-CDC42Hs chimeras, it was shown that residues A27 and G30, in the pre-switch I region of Rac (1 and 2) are critical for oxidase activation; replacing residues 1–40 of CDC42Hs (a GTPase incapable of supporting oxidase activation) with the corresponding region of Rac1 resulted in a chimera with Rac-like oxidase activating ability.Citation65 It was assumed that residues A27 and G30, just like residues T35, D38, and Y40, are directly involved in binding of Rac to p67phox, 2 assumptions which had to be modified when the Rac1-p67phox complex was crystallized.
A different approach to the identification of functional domains in Rac1 was the use of overlapping 15-mer peptides, covering the full-length of Rac1, as inhibitors of cell-free oxidase activation (“peptide walking”).Citation66 Four domains “lighted up”: a region corresponding to residues 103–107; the insert region (residues 124–135); a region overlapping the C-terminal p67phox-binding domain (residues 163–169), and the polybasic C-terminal region (residues 183–188). Surprisingly, the switch I region was not detected. It should be noted that this method does not reveal the identity of the interaction partner, among oxidase components, of the detected Rac sequence. A systematic mutational analysis of domains identified by “peptide walking,” revealed the importance of Y40 (in switch I), H103, and K166 (in the C-terminal p67phox-binding domain 143–175) but did not support the involvement of the insert region.Citation67 It was also found that Clostridium difficile-induced glucosylation of Rac1 at T35 eliminates its ability to support oxidase activation in a cell-free system, reproducing the effect of a T35A mutation.Citation68
In parallel to the identification of p67phox-binding domains in Rac, work proceeded on the characterization of the Rac-binding domains in p67phox. This pointed to 4 tetratricopeptide repeat (TPR) motifs, in the N-terminal half of p67phox, with emphasis on the first 3 TPRs and on the β hairpin insertion between the 3rd and 4th TPR.Citation69 Of special significance seems to be residue R102 in the 3rd TPR. A clinical correlation is represented by a CGD patient, with a deletion of K58 in the 2nd TPR in p67phox, resulting in the inability to interact with Rac1 and, consequently, to generate O2.-.Citation70 The claim that Rac binds to residues 170–199 of p67phox and that binding of Rac is enhanced by opening of intramolecular bonds in p67phox,Citation71 was not confirmed and might have had a confusion-generating influence on the development of some models of p67phox-Rac interaction (see ref. Citation72).
A complete picture of the Rac1-p67phox binding interface was based on the crystallization of a complex of Rac1 Q61L(1–184) with p67phox(1–203).Citation73 In this study, the high affinity binding of Rac1 Q61L and Rac2 Q61L to p67phox(1–203) and the lack of binding of CDC42Hs were first confirmed by isothermal titration calorimetry. Rac1 residues directly participating in interaction with p67phox comprised S22, T25, N26, F28, G30, E31, at the N-terminus, and A159, L160, and Q162, at the C-terminus (). All the residues involved in binding to p67phox are conserved in both Rac1 and Rac2, explaining the identical affinity for p67phox of the two Rac isoforms. These data exhibit good correlation with results derived from binding to p67phox and oxidase activation in vitro experiments utilizing Rac mutants,Citation60 Rac-Rho chimeras,Citation61 and Rac-CDC42Hs chimeras,Citation65 with the reservation that they do not support the direct participation in the Rac – p67phox interface of Rac residues T35, D38 and Y40 (ref. Citation60), A27 (ref. Citation65), and H103 and K166 (ref. Citation67). It is likely that mutations in these residues cause a conformational change in Rac which indirectly negatively affects its ability to bind to p67phox.
Figure 1. The interface between Rac and p67TPR. (A) Schematic representation of the hydrogen bond interactions at the interface between Rac and p67phox TPRs. Residues from Rac are labeled in red and residues from p67phox TPRs, in blue. Hydrogen bonds are depicted as dotted lines with the bond distances indicated in Å. The positions of switch I and the β hairpin insertion are indicated in red and blue, respectively. (B) Ribbons representation of the RacGTP (red)/p67phox TPR (blue) complex. The effector loop of Rac is colored in yellow, and amino acids 103–107 and the helical insert region (120–135) are indicated in green. The position of Gly30 at the N terminus of the effector loop is indicated to show the orientation of switch I. The positions of mutations in p67phox occurring in CGD are shown as red spheres (Reprinted with permission from ref. Citation73. Copyright 2000, Elsevier).
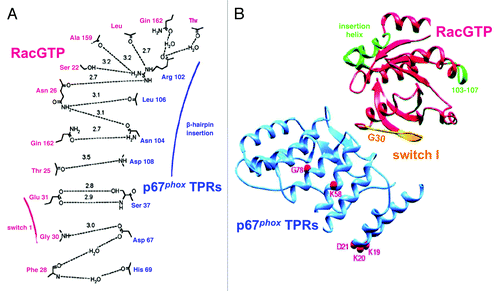
p67phox residues, directly implicated in interaction with Rac comprise residues S37 and D67, in the 2nd TPR, R102, in the 3rd TPR, and N104, L106, and D108, in the β hairpin insertion, between the 3rd and 4th TPRs (). The importance of R102 is in good agreement with the mutational study of Koga et al.,Citation69 describing lack of cell-free oxidase activation by a p67phox R102Q mutant but, as in the case of Rac, other mutations in the first 3 TPRs disturb binding of Rac even though the particular residues might not be directly involved in interactions with residues in Rac. The binding surface on p67phox for Rac1, comprising p67phox residues R28 and R102, was used, in an in silico approach, to screen a library of 700,000 small-molecule compounds for selecting one which competed with Rac1 for binding to p67phox and acted as an inhibitor of Nox2-dependent O2.- production in intact phagocytes and in a cell-free system.Citation74
Is Rac Bigamous?
In parallel to the overwhelming evidence in support of p67phox being the par excellence partner of Rac in oxidase assembly, an interaction of Rac with cytochrome b558 and, more specifically with Nox2, was championed by Bokoch and coworkers. The hypothesis that Rac2 binds to cytochrome b558 originated in work showing that translocation of Rac2 to neutrophil membranes in patients with the X91° form of CGD (lacking cytochrome b558), was markedly reduced.Citation75 Diebold and BokochCitation72 subsequently proposed that Rac2 interacts directly with cytochrome b558, with the insert region of Rac serving as binding site. However, the method used to quantify binding rested on a very modest increment in fluorescence upon addition of cytochrome b558 to Rac, and there was no certainty that binding was not to the lipids present in the cytochrome preparation. Additional doubts are raised by the inability of most researchers to confirm a requirement for the Rac insert region in oxidase function (see section “The Controversial Insert Region”). In more recent work, the same group offered additional support for Rac2 - cytochrome b558 interaction, by using a pull-down assay containing prenylated Rac and purified relipidated cytochrome b558; the binding was not Rac-specific as shown by the fact that prenylated CDC42Hs competed for binding to cytochrome b558, and was also not GTP-dependent.Citation76 Finally, a region in Nox2, comprising residues 419–430, was identified as a specific binding site for Rac2.Citation77 The author of this review believes that confirmation of these significant claims by other investigators is required.
How Does Rac Assist p67phox?
The commonly accepted model of oxidase assembly confers to p67phox the role of inducing a conformational change in Nox2, leading to the initiation of electron flow along the redox stations gradient represented by NADPH → FAD → 2 hemes → 2 O2 → 2 O2.-. Rac is a sine qua non participant in oxidase assembly, both in in vivo and in vitro situations and it is widely accepted that its main, if not only, partner, is p67phox. Initially, the main function of Rac was seen as that of a “carrier” protein, assisting p67phox to be directed toward the membrane environment of cytochrome b558. This seemed logical because there are no known motifs in p67phox to interact with either Nox2 or p22phox, similar to those identified in p47phox, nor does p67phox express electrostatic or hydrophobic elements with affinity for the negatively charged inner aspect of the plasma membrane (on the contrary, the low pI of p67phox promotes repulsion by the membrane) . The “carrier” role of Rac is poignantly illustrated by the ability of prenylated Rac to recruit p67phox to the membrane, in the absence of p47phox and of an amphiphilic activatorCitation10 but recruitment also results in oxidase activation, suggesting that the mere cooperation of Rac and p67phox is sufficient for the initiation in Nox2 of the catalytic stage of O2.- generation. In order to clearly separate the carrier and “activating” roles of Rac, extensive use was made of chimeric constructs, consisting of an N-terminal segment of p67phox (residues 1–210 or 1–212) and full-length Rac, the C-terminus of the p67phox moiety being fused to the N-terminus of the Rac moiety. The first such chimera was designed by Miyano et al.Citation78 and was found to support oxidase activation in vitro with higher activity and stability than the individual components, and, when applied at high concentrations, also in the absence of p47phox. Our group generated a large number of chimeric constructs consisting of either parts of p67phox fused to Rac or parts of RacCitation7,Citation79,Citation80 () or of parts of p47phox, in a tripartite complex with parts of p67phox and Rac.Citation81,Citation82 For a review of the use of such chimeric constructs for the elucidation of the mechanism of oxidase assembly, in general, and of Rac function, in particular, see ref. Citation83. We found that a [p67phox(1–212)-Rac1(1–192)] chimera (prototype chimera), in the GTPγS-bound state, was a potent activator of the oxidase in vitro and bound GTP in equimolar amounts, just as monomeric Rac1.Citation79 If Rac serves only as a passive carrier for p67phox, a covalent bond between segments of Rac1, responsible for membrane attachment (residues 178–192; see section ” The Name of the Game is Translocation”), and parts of p67phox, containing the “activation domain” or the “activation domain” supplemented with a region extending from the “activation domain” to the TPRs, should be capable of oxidase activation. As described in ref. Citation79 and shown in , this was not the case. We also showed that the prototype chimera was most active in the GTP-bound form, a result which was not expected should the Rac moiety serve only as an instrument for the attachment of p67phox to the membrane. All this suggested that an intrachimeric interaction between regions, in both Rac and p67phox, responsible for the canonical interaction between the monomeric components under physiological conditions, is an absolute requirement for the chimera to be active. This proposal was supported by work involving mutagenesis of the prototype chimera.Citation7 Thus, mutation V204ACitation84 in the “activation domain,” and R102ECitation68 in the 3rd TPR of the p67phox moiety, known to eliminate the ability of p67phox to support oxidase activation, and mutations A27K and G30SCitation65 in the Rac moiety, known to eliminate the ability of Rac to support oxidase activation, markedly reduced the activity of the chimera (). These findings led us to a model of Rac - p67phox interaction, illustrated in , in the forms of the “closed” (active) and “open” (inactive) chimeras. The model is discussed in detail in references Citation79 and Citation83 and its essence is that Rac is much more than a carrier for p67phox, its additional function being the causation of a conformational change in p67phox, required for productive interaction with Nox2. This is based on 3 sets of experimental evidenceCitation7,Citation83: (1) The prototype chimera, in the GMPPNP-bound (“closed”) form, has a more compact shape than the GDP-bound (“open”) form, as evident by gel filtration; (2) An inactive chimera (due to it being in the GDP-bound form or as a consequence of A27K or G30S mutations in the Rac moiety) can be reactivated by the addition of exogenous Rac-GTP, binding to the p67phox moiety, and (3) Fluorescence resonance energy transfer (FRET) studies, to assess the proximity of the Rac and p67phox moieties in chimeras in the GMPPNP- and GDP-bound forms. In these latter studies, chimeras were labeled with the fluorescent GTP or GDP analogs, 2'-(or 3′)-O-(N-methylanthraniloyl)-GMPPNP (mant-GMPPNP) or mant-GDP, and the FRET from the 4 tryptophans present in p67phox moiety to the fluorescent GMPPNP or GDP bound to the Rac moiety, was measured.Citation83 As apparent in , “closed” GMPPNP chimeras exhibit lower tryptophan fluorescence and higher mant-derived FRET-based fluorescence, than the “open” GDP chimeras. A requirement for an intrachimeric bond between TPRs in the p67phox moiety and the pre-switch I region in the Rac moiety was also evident in tripartite [p47phox-p67phox-Rac] chimeras.Citation81 Surprisingly, there is no crystallographic evidence for a structural change in p67phox as a direct consequence of binding of Rac. Thus, the structure of p67phox(1–203), with electron density visible up to residues 186Citation73 in complex with Rac(1–184) was indistinguishable from that of p67phox(1–213), crystallized independently, with electron density visible up to residue 193.Citation85 One has to consider, however, that neither the canonical (199–212),Citation84 nor the extended (190–208)Citation5 p67phox “activation domain” were visible in the crystals of the complexCitation73 and it is also possible that membrane attachment of Rac is required for the induction of a conformational change in p67phox.
Figure 2. Schematic representation of [p67phox – Rac1] chimeras and a [p67phox – CDC42Hs] chimera. The numbering of chimeras are according to Alloul et al.Citation79 The characteristic feature of each construct is briefly indicated at the right of the scheme of the chimera, with the color of the font corresponding to the color of respective moiety of the chimera. The presence (+) or absence (-) of NADPH oxidase supporting activity was determined in an amphiphile-activated cell-free system, consisting of phagocyte membrane, chimera in the GTPγS-bound form, and p47phox (modified from ref. Citation83).
![Figure 2. Schematic representation of [p67phox – Rac1] chimeras and a [p67phox – CDC42Hs] chimera. The numbering of chimeras are according to Alloul et al.Citation79 The characteristic feature of each construct is briefly indicated at the right of the scheme of the chimera, with the color of the font corresponding to the color of respective moiety of the chimera. The presence (+) or absence (-) of NADPH oxidase supporting activity was determined in an amphiphile-activated cell-free system, consisting of phagocyte membrane, chimera in the GTPγS-bound form, and p47phox (modified from ref. Citation83).](/cms/asset/aca56430-c085-4861-b359-5578d162a1f7/ksgt_a_10927952_f0002.gif)
Figure 3. Intrachimeric bonds between residues in the switch I of the Rac1 moiety and in the TPR domains of the p67phox moiety and an intact activation domain in the p67phox moiety of chimera [(p67phox(1–212)-Rac1(1–192)) (prototype chimera 3) are essential for enabling the chimera to support NADPH oxidase activity. The numbering of chimeras are according to Alloul et al.Citation79 Four mutants of the prototype chimera 3 were constructed in which residues in the activation domain and one of the TPR domains of the p67phox moiety and 2 residues in the switch I of the Rac1 moiety were mutated. Graphs A and B describe the ability of mutant chimeras to support NADPH oxidase activation in vitro. (A) NADPH oxidase supporting activity of the mutant chimeras, in non-prenylated form, was assessed in an amphiphile-dependent cell-free assay consisting of phagocyte membrane, chimera in the GTPγS-bound form, and p47phox. (B) The activity of the equivalent prenylated chimeras was measured in an amphiphile-independent cell-free system, consisting of membrane and chimera in the GTPγS-bound form, in the absence of p47phox (modified from ref. Citation83).
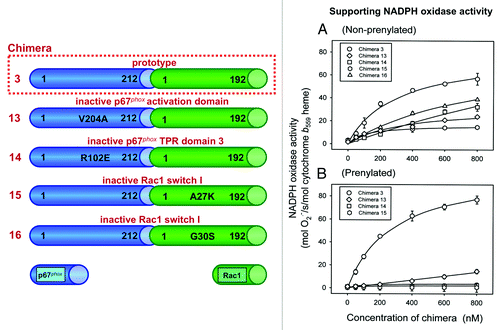
Figure 4. Hypothetical models of the “closed” (active) and “open” (inactive) conformations of the prototype chimera 3 and Rac1 moiety mutants. Nucleotide exchange to GMPPNP or mutation Q61L, in the Rac1 moiety, assuring that the chimera is permanently in the GTP-bound form, lead to a closed conformation. A GDP-bound form of the chimera or mutations A27K and G30S in the Rac1 moiety of the chimera, in the GMPPNP-bound form, prevent protein – protein interaction with the p67phox moiety and lead to an open conformation (modified from ref. Citation83).
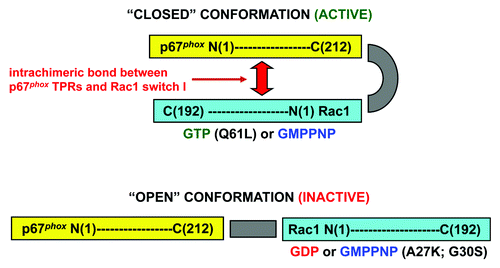
Figure 5. Structure of mutants used in intramolecular FRET studies on the [p67phox – Rac1] chimera. The prototype chimera [p67phox(1–212)-Rac1(1–192)] was subjected to either one (W56F) or 2 mutations (W56F; W97F) in the Rac1 moiety, resulting in the generation of chimeras with 4 tryptophans in the p67phox moiety and 1 or none in the Rac1 moiety. The W56F mutation was confirmed by the lack of response of the mutant Rac1 to the Rac-specific guanine nucleotide exchange factor (GEF), TrioN.Citation129 The principle of intramolecular FRET is summarized in the bottom part of the figure. In the upper left panel of the figure is a ribbon diagram of Rac1 in the GMPPNP-bound form. Residues W56 and W97 are displayed in dark and light green, respectively. The GMPPNP is displayed as a stick model with atoms colored by atom type (oxygen, red; carbon, white; nitrogen, blue; phosphorous, yellow). The orange sphere represents the Mg2+. The binding surface of Rac1 with p67phox (based on ref. Citation72) is colored in pink. The upper right side panel illustrates an overlay of characteristic emission spectra (305–485 nm) of the [p67phox – Rac1] chimera containing W56F and W97F mutations, in the mant-GDP and mant-GMPPNP-bound forms, excited at 295 nm. The GMPPNP-bound form exhibits enhanced FRET, in comparison to the GDP-bound form of the same chimera. This is expressed in an increase in mant-dependent fluorescence emission, at 440 nm, and a reduction in tryptophan-dependent fluorescence emission, at 340 nm (modified from ref. Citation83).
![Figure 5. Structure of mutants used in intramolecular FRET studies on the [p67phox – Rac1] chimera. The prototype chimera [p67phox(1–212)-Rac1(1–192)] was subjected to either one (W56F) or 2 mutations (W56F; W97F) in the Rac1 moiety, resulting in the generation of chimeras with 4 tryptophans in the p67phox moiety and 1 or none in the Rac1 moiety. The W56F mutation was confirmed by the lack of response of the mutant Rac1 to the Rac-specific guanine nucleotide exchange factor (GEF), TrioN.Citation129 The principle of intramolecular FRET is summarized in the bottom part of the figure. In the upper left panel of the figure is a ribbon diagram of Rac1 in the GMPPNP-bound form. Residues W56 and W97 are displayed in dark and light green, respectively. The GMPPNP is displayed as a stick model with atoms colored by atom type (oxygen, red; carbon, white; nitrogen, blue; phosphorous, yellow). The orange sphere represents the Mg2+. The binding surface of Rac1 with p67phox (based on ref. Citation72) is colored in pink. The upper right side panel illustrates an overlay of characteristic emission spectra (305–485 nm) of the [p67phox – Rac1] chimera containing W56F and W97F mutations, in the mant-GDP and mant-GMPPNP-bound forms, excited at 295 nm. The GMPPNP-bound form exhibits enhanced FRET, in comparison to the GDP-bound form of the same chimera. This is expressed in an increase in mant-dependent fluorescence emission, at 440 nm, and a reduction in tryptophan-dependent fluorescence emission, at 340 nm (modified from ref. Citation83).](/cms/asset/14f6e746-89ee-47bc-aa9a-bf9ee04a6e21/ksgt_a_10927952_f0005.gif)
The Controversial Insert Region
A characteristic of Rho proteins is a 12-amino acids helical insert, corresponding, in Rac, to residues 124–135.Citation38,Citation86,Citation87 Its participation in oxidase activation is highly controversial and was the theme of intense debate. The first indication that the insert region is important for oxidase activation originated in Rac1 “peptide walking” studies, with peptides corresponding to residues 123–133 exhibiting modest inhibitory activity.Citation66 Freeman et al.Citation86 originally described decreased oxidase activation in vitro by Rac in which the whole insert region was deleted or certain residues were mutated but, although EC50 values were much increased, the effect on Vmax was rather modest. In a subsequent study, it was proposed, though not proven, that the target of the insert region might be cytochrome b558.Citation88 Our studies, performed at about the time of publication of these reports, did not support a significant role for the insert region, as shown by the finding of only a minor increase in the EC50 and no effect on the Vmax of an insert region deletion mutant.Citation67 The most prominent support for the requirement for the insert region in oxidase assembly came from Bokoch and coworkers. They proposed that Rac2 interacts directly with cytochrome b558, to support electron transfer from NADPH to FAD, and that this interaction depends on the insert region.Citation71 Binding of Rac2 to cytochrome b558, mediated by the insert region, was confirmed in yet another report by the same group, in which it was also shown that CDC42Hs competes with Rac2 for binding to the cytochrome, the competition involving the insert region of CDC42Hs.Citation76 It is of interest that binding of Rac2 to cytochrome b558 was largely independent of whether Rac was in the GTP- or GDP-bound form.Citation76,Citation77
As opposed to the view expounded above, a very large body of evidence exists demonstrating the lack of involvement of the Rac insert region in oxidase activation. A [p67phox(1–212)-Rac1(1–192)] chimera, with the insert region deleted, was as active an oxidase activator as the native chimera, in both nonprenylatedCitation79 and prenylatedCitation80 forms. The lack of requirement for the Rac insert region was also evidenced by assessing the oxidase-activating ability of tripartite [p47phox-p-67phox-Rac1] chimeras with a deleted insert region in the Rac moiety; in both nonprenylatedCitation81and prenylatedCitation82 forms, the deletion mutants were fully competent activators in the cell-free system. In a most extensive study, Miyano et al.Citation89 conclude that the insert region of Rac1 and Rac2 is dispensable for oxidase activation under both cell-free and whole cell conditions. It is also of interest that, in this report, the ability of Rac3 to activate the oxidase, at least in a transfected whole cells situation, is demonstrated for the first time.
The Name of the Game is Translocation
It seems incredible at present that the fact that Rac has to translocate to the membrane in order to contribute to oxidase assembly, was subject to controversy. It is clearly established now that Rac1 and Rac2 translocate from the cytosol to the membrane following dissociation from the regulator protein RhoGDI. When in complex with RhoGDI, Rac is in the GDP-bound form; dissociation from RhoGDI is an obligatory first step in the way to the membrane, the mechanism of dissociation being only partially understood (see section “RhoGDI - The Embrace of the Jailer”).Citation52,Citation90,Citation91 Dissociation from RhoGDI is associated with but, more likely, followed by nucleotide exchange to GTP, a process mediated by a member of the guanine nucleotide exchange factor (GEF) family. The timing of the encounter with a GEF and its cellular location are not fully understood, though it is likely to occur at the level of the membrane. Unknown is also the cellular site of the critical binding to p67phox; this could occur in the cytosol, before translocation of Rac to the membrane, or after anchoring of Rac to the membrane, the latter occurrence being more likely, considering that GEF-mediated exchange to GTP is a precondition for binding to p67phox. A “heretical” model was also proposed, in which an intermediate ternary complex, consisting of RacGDP-RhoGDI-p67phox, is first formed, to be followed by dissociation from RhoGDI, nucleotide exchange to GTP and the formation of the higher affinity RacGTP-p67phox binary complex.Citation92 It should be noted that engagement of Rac with each of the three partners, p67phox, RhoGDI, and GEFs, does not appear to be mutually prohibitive.
Translocation of Rac is entirely dependent on the the C-terminus of the protein and is mediated by 2 mechanisms: (a) An electrostatic attraction between basic residues (6 in Rac1, and 3 in Rac2) and the negatively charged inner aspect of the lipid bilayer of the membrane, and (b) A hydrophobic interaction between the geranylgeranyl “tail” of both Rac1 and Rac2 and the lipid bilayer. It is obvious that the physiological mechanism of Rac translocation should be studied with prenylated Rac, which is the only form found in the cytosol and capable of association with RhoGDI. In vitro membrane translocation experiments are also performed with nonprenylated Rac but these are obviously artifacts because they are resting exclusively on the electrostatic mechanism.
Probably the first in vitro study on this subject is that of Sawai et al.,Citation93 who described Rac translocation to the membrane in mixtures of membrane and cytsosol of differentiated HL60 cells, by the addition of the anionic amphiphile, arachidonic acid, and GTPγS. A first major in vivo study showed clearly that, in stimulated human neutrophils, Rac translocated from the cytosol (where it was found in association with RhoGDI) to the membrane, in temporal correlation and equimolar amounts with p47phox and p67phox, and that translocation of the 3 components coincided with the generation of O2.-.Citation94 In combined in vivo and in vitro studies in human neutrophils, it was found that Rac2 translocation was independent of that of p47phox and p67phox but was reduced when membranes of CGD patients of the X91° form, lacking cytochrome b558, were used in the assay.Citation75
This early clear picture was somewhat clouded by a report suggesting that carboxyl methylation of Rac, following exchange to GTP and dissociation from RhoGDI, conferred membrane tropism,Citation95 and by 2 studies, performed in human neutrophils, claiming that Rac did not translocate to the membrane in response to stimuli activating the oxidase.Citation96,Citation97 Some of these confusing results may have been due to methodological difficulties related to the detection of membrane-bound GTPases and, possibly, to the fact that assessment of membrane attachment of a specific component might depend on its half-life in the new location and on secondary interaction with another component(s). This is illustrated by the finding that recombinant Rac1 did not translocate to membrane liposomes, in response to the anionic amphiphile SDS, in a cell-free system but when Rac was a moiety of a chimera with p67phox, the chimera did show amphiphile-dependent translocation.Citation79 A transient association of Rac2 with the membrane is supported by whole cell studies, demonstrating an uninterrupted translocation of Rac2 ( = high turnover) in a myeloid cell line, indicating continuous exchange of membrane-bound Rac for cytosolic Rac.Citation98 In a more general context, membrane localization of Rac proteins is prominently governed by the acidic phospholipids on the inner aspect of the plasma membrane and by the changes in their nature and quantity in the course of remodeling associated with the process of phagocytosis, normally a precondition for the initiation of oxidase activation (reviewed in ref. Citation99). The monovalent phospholipids phosphatidylserine (PS) and phosphatidylinositol (PI) contribute most of the negative charge but polyvalent phosphoinositides, such as phosphatidylinositol 4-monophosphate (PtdIns(4)P), phosphatidylinositol 4,5-bisphosphate (PtdIns(4,5)P2), and phosphatidylinositol 3,4,5-trisphosphate (PtdIns(3,4,5)P3) also contribute, these being derived by receptor-mediated activation of phosphoinositide metabolism. Rac proteins are targeted to the membrane by a “coincidence detector” mechanism that combines electrostatic attraction of the polybasic region and partitioning of the isoprenyl tail into the lipid bilayer, thus preventing mistargeting to non-membrane anionic structures.Citation99
Cash or Charge
For a brief review of the importance of charge in the cellular localization of Rac, see ref. Citation100. The first description of the role of the polybasic region of Rac1 in oxidase activation was based on the inhibitory effect of Rac1 peptide 178–188 on cell-free oxidase activation.Citation101 The original interpretation of this as a sequence-specific effect had to be modified consequent to the finding that peptides consisting of the corresponding residues in RhoA, RhoB, a Rac1 C-terminus retropeptide, and several unrelated basic polyamino acids were also inhibitory, indicating that the effect depended on the overall charge and not on amino acid sequence specificity.Citation102 The critical importance of the polybasic region in Rac in oxidase activation was beautifully demonstrated in a landmark paper by Kreck et al.,Citation6 who showed: the higher efficiency of Rac1 over Rac2, when assayed in the nonprenylated form; the lack of activity of both Rac1 and Rac2, when the 10 C-terminal residues were eliminated; the loss of activity of Rac1 mutants in which basic residues were replaced by acid or neutral amino acids; the loss of Rac activity at high ionic concentrations, and the enhanced activity when the lipid environment of cytochrome b558 was enriched in the anionic phospholipid, PI. Prenylation led to equal activities of Rac1 and Rac2, compensating for the difference in charge. The paramount role of the polybasic region of Rac1 in membrane attachment and in the ensuing oxidase activation has been proven in numerous additional reports, using a variety of approaches of progressing sophistication. These comprise: the marked inhibitory effect of C-terminal Rac peptides, revealed by “peptide walking”Citation66; the lack of activity of a [p67phox-Rac1] chimera, in which the Rac1 moiety was truncated at residue 178Citation79; the very poor membrane association of a [p67phox-Rac1] chimera which was prenylated but lacked the polybasic regionCitation80, and the finding that a C-terminal Rac1 peptide and the cationic antibiotic neomycin inhibited canonical cell-free oxidase activation supported by nonprenylated Rac1 but not the amphiphile-independent activation,Citation10 supported by prenylated Rac1.Citation14,Citation103
In a detailed study, comparing the effect of incremental mutations to the neutral glutamine, of the basic residues 183–188 (from 1 to 6 residues) in the Rac1 moiety of of a [p67phox-Rac1] chimera, in both nonprenylated and prenylated forms, it was found that a single mutation was sufficient to markedly reduce the activity of the nonprenylated chimera, whereas replacement of all six basic residues by glutamine was required to eliminate the activity of the prenylated chimera ().Citation7 Oxidase activation by the chimera was inhibited by a Rac1 C-terminal peptide, comprising the polybasic domain, only when the chimera was nonprenylated; a prenylated chimera was refractory to inhibition by the same peptide.Citation7
Figure 6. A minimal polybasic stretch at the C-terminus of the [p67phox – Rac1] chimeras is essential for the support of NADPH oxidase activity. Four mutants of the prototype chimera 3 were constructed in which 1, 3, 4, or 6 basic residues were replaced by glutamines. The numbering of chimeras are according to Alloul et al.Citation79 (A) NADPH oxidase supporting activity of the mutant chimeras, in nonprenylated form, was assessed in an amphiphile-dependent cell-free assay consisting of phagocyte membrane, chimera in the GTPγS-bound form, and p47phox. (B) The activity of the equivalent prenylated chimeras was measured in an amphiphile-independent cell-free system, consisting of membrane and chimera in the GTPγS-bound form, in the absence of p47phox (modified from ref. Citation83).
![Figure 6. A minimal polybasic stretch at the C-terminus of the [p67phox – Rac1] chimeras is essential for the support of NADPH oxidase activity. Four mutants of the prototype chimera 3 were constructed in which 1, 3, 4, or 6 basic residues were replaced by glutamines. The numbering of chimeras are according to Alloul et al.Citation79 (A) NADPH oxidase supporting activity of the mutant chimeras, in nonprenylated form, was assessed in an amphiphile-dependent cell-free assay consisting of phagocyte membrane, chimera in the GTPγS-bound form, and p47phox. (B) The activity of the equivalent prenylated chimeras was measured in an amphiphile-independent cell-free system, consisting of membrane and chimera in the GTPγS-bound form, in the absence of p47phox (modified from ref. Citation83).](/cms/asset/94bd5762-f7a4-4226-a6cb-f6bfc1948062/ksgt_a_10927952_f0006.gif)
The seminal role of charge-based interactions was most emphatically demonstrated in work on oxidase activation by [p47phox-p67phox-Rac1] tripartite chimeras.Citation81 One expression of this was the finding that enrichment of phagocyte membrane liposomes, used in the cell-free oxidase activation system, with anionic phospholipids, such as phosphatidic acid (PA), phosphatidylglycerol (PG), PI, or PS, but not with a neutral phospholipid, such as phosphatidylcholine (PC), made oxidase activation amphiphile-independent. The removal of the need for an activating amphiphile in a cell-free system by the enrichment of the membrane with anionic phospholipids also applied to canonical activation by a mixture of p47phox, p67phox, and Rac1, not linked by covalent bondsCitation81 and was first alluded to by the findings of Kreck et al.,Citation6 describing the decrease in EC50 for Rac1 (but not Rac2) by the presence of the anionic phospholipid, PI, in cytochrome b558 liposomes. Removing the positive charge of the tripartite chimera by one of three procedures, performed on the Rac1 moiety (removing the C-terminal residues 179–192; replacing the 6 basic residues 182–188 with glutamines, or exchanging residues 183–188 of Rac1 by the corresponding residues in Rac2 [only 3 basic residues]), resulted in a the total elimination of oxidase activation (procedures 1 and 2) or a marked increase in the EC50 of the chimera (procedure 3).Citation81 When the tripartite chimera was prenylated, it supported oxidase activation independently of amphiphile in a cell-free system with native membranes, and binding of the chimera to the membranes was not enhanced by enrichment of the latter with anionic phospholipids.Citation82 Replacing the basic residues 183–188 in the Rac1 moiety with glutamines or exchanging residues 183–188 of Rac1 by the corresponding residues in Rac2, in the prenylated chimera, resulted in only a partial reduction in binding to native membrane liposomes and did not diminish oxidase activating ability, proving that, on some occasions, prenylation can supersede the charge-based mechanism (see also , for the same phenomenon with prenylated [p67phox-Rac1] bipartite chimeras).
Although the accepted view of charge-based interactions, involving Rac, is that these are nonspecific, recognition by Rac of specific phospholipids was occasionally described. Thus, it has been claimed that Rac1 binds selectively to the phosphoinositide 3-kinase (PI3K) product, PtdIns(3,4,5)P3 by a mixed electrostatic (phosphate groups) and hydrophobic (fatty acyl chains) interaction, requiring the participation of the Rac1 insert region.Citation104 In a different report, specificity of Rac1 for the anionic phospholipid PS, involving the polybasic region, was decribed.Citation105 The significance of charge in the membrane localization of various Rac isoforms was also examined in whole cells.Citation106 The study shows that the accumulation of Rac isoforms at the phagosome membrane, in the course of phagocytosis in a macrophage cell line, is proportional to the number of basic residues (Rac1 > Rac3 > Rac2) and is dependent on the production of PA and specific mono- and poly-phosphoinositides in the phagosome membrane; the study also shows that Rac2, unlike Rac1, is localized at endomembranes by a charge-independent process.Citation106 In a study in primary murine neutrophils, it was also shown that phagocytosing cells exhibit a negative charge at the phagosome membrane generated by PS and poly-phosphoinositides, leading to the preferential localization of Rac1; the ensuing decrease in anionic phospholipids creates a lesser negative charge that results in the recruitment of Rac2.Citation107
The Importance of Being Earnestly Prenylated
All Rac proteins isolated from the cytosol of phagocytes and found to participate in oxidase activation were recovered in the prenylated (geranylgeranylated) form.Citation32,Citation51-Citation56 To the best of our knowledge, no nonprenylated Rac is present in the cytosol, a fact that did not prevent a considerable part of oxidase-related research on Rac being performed using the nonphysiologic nonprenylated protein. The 20-carbon lipophilic geranylgeranyl isoprene confers to both Rac1 and Rac2 a major part of its tropism for membranes in conjunction with the positively-charged residues present the polybasic region of Rho proteins.
The enzyme responsible for prenylation of Rho proteins is geranylgeranyltransferase type I (GGTase-I), which recognizes a CAAX sequence at the C-terminus of Rho proteins and attaches the prenyl group to the cysteine; specificity for Rho is conferred by the C-terminal residue (X) being leucine (for a review, see ref. Citation108). In the mature Rho protein, prenylation is followed by the removal of residues AAX and the attachment of a carboxymethyl group to the prenyl-cysteine.
The first report that the prenylated forms of Rac1 and Rac2 support oxidase activation in vitro was that of Ando et al.,Citation57 who used recombinant post-translationally modified proteins produced in Sf9 insect cells. In a precocious finding, made before the identification of Rac as the oxidase-related GTPase, prenylation inhibitors were shown to interfere with the oxidative burst of differentiated HL60 cells.Citation109 The role of prenylation in membrane localization of Rac proteins was proposed soon after their discovery.Citation110 In the first in depth study on the role of prenylation in oxidase activation, Heyworth et al.Citation111 confirmed the finding of Ando et al. concerning the ability of prenylated Rac1 and Rac2 to support oxidase activation in vitro and make the important observation that exogenous GTP has to be present in the cell-free reaction buffer unless Rac was preloaded with GTP. Nonprenylated Rac was inactive, even upon the addition of exogenous GTP, but was active if preloaded with GTP. Since binding of GTP under high Mg2+ conditions is slow,Citation54 the authors explained the rapid GDP to GTP exchange on prenylated Rac by the existence of a GEF in the membrane, a prediction proven right in the future and supported by findings of Fuchs et al.Citation112 The fact that, under the same conditions, nonprenylated Rac was not converted to the GTP-bound form was explained by the hypothesis that prenylation-dependent localization to the membrane was required for GEF-mediated nucleotide exchange to GTP (another fulfilled prediction). A good example for the importance of methodology in reaching conclusions is provided by the finding that the requirement for prenylated Rac in cell-free oxidase systems depended on the method of membrane preparation; thus, when membranes were used in the native form, no requirement for added Rac was demonstrated, due to the presence of Rac attached to the membrane, but washing the membranes with high molarity KCl removed Rac and made the system dependent on added Rac.Citation112 As mentioned above, the use of prenylated Rac in in vitro experiments has the advantage of being closer to the in vivo reality, as shown by the finding that, although Rac2 is the physiological activator of the oxidase in human neutrophils,Citation53,Citation54 Rac2 is a poor activator in vitro when tested in the nonprenylated form (due to exclusive charge-based attachment to the membrane) but a good activator, when tested in the prenylated form.Citation6,Citation57
A wealth of information on the relevance of Rac prenylation for oxidase function was obtained by making use of recombinant prenylated RacCitation10,Citation103 and of bipartiteCitation80 or tripartiteCitation82 chimeric constructs, with the Rac moiety prenylated. This raises again a methodological issue, namely the way by which prenylated Rac is prepared. In earlier work, prenylated Rac was expressed in Sf9 insect cells and extracted from the membrane fraction by detergent.Citation10,Citation57,Citation103 Our group introduced the routine production of prenylated Rac for cell-free oxidase studies by enzymatic geranylgeranylation of recombinant Rac produced in E. coli, by GGTase-I.Citation7,Citation80,Citation82 The latter Rac is, thus, not truncated at residue 189 and not carboxyl methylated. Using prenylated Rac1 and chimeras with a prenylated Rac1 moiety, its key importance in carrying p67phox to the membrane, even in the absence of p47phox, was demonstrated.Citation7,Citation80 A prenylated [p47phox-p67phox-Rac1] chimera was found capable of binding to native membranes and elicit NADPH-dependent O2.- production in the absence of an activator.Citation82
Prenylated Rac was used successfully for the elucidation of the chemical basis of binding to the membrane, studies which proved to be invaluable for deciphering the mechanism of dissociation from RhoGDI. For this purpose, binding of prenylated Rac1 to artificial phospholipid vesicles (not containing any membrane components), enriched or not in anionic phospholipids, was assessed. It was found that prenylated, but not nonprenylated Rac1, was bound to vesicles made of the neutral phospholipid, PC, but binding was markedly enhanced by enrichment with 33% of the anionic phospholipids PA, PG, PI, or PS.Citation103,Citation113 In accordance with these results, it was found that PC vesicles and free RhoGDI interfere, in a dose-dependent manner, with cell-free oxidase activation supported by prenylated Rac1 or a prenylated [p67phox-Rac1] chimera, in an amphiphile-independent system, but not with activation supported by nonprenylated Rac, in a system containing amphiphile and p47phox.Citation7,Citation10
Finally, an intriguing study describes the state of Rac in mice in which GGTase-I was specifically deleted in macrophages.Citation114 Surprisingly, a high amount of GTP-bound Rac was found associated with the membrane, also leading to the production of proinflammatory cytokines. Paradoxically, these findings support the dominant role of charge in the membrane attachment of Rac1; in the absence of prenylation, Rac1 (the predominant form of Rac in macrophagesCitation59) will still translocate to the membrane, promoted by the fact that, in the absence of prenylation, it will not be bound to RhoGDI.
The Directors
RhoGDI: The embrace of the jailer
Rac1 and Rac2 are found in the cytosol of phagocytes exclusively as heterodimers with RhoGDI, Rac being in the GDP-bound form. Three major forms exist in mammals: RhoGDI1 (also known as RhoGDIα), RhoGDI2 (also known as RhoGDIβ, LyGDI, or D4-GDI), and RhoGDI3 (also known as RhoGDIγ) (for reviews, see refs. Citation115–Citation117). RhoGDI1 is ubiquitously expressed and best characterized and binds with high affinity to both Rac1 and Rac2. RhoGDI2 is expressed predominantly in hematopoietic cells and associates with Rac1 and Rac2 with lesser affinity. The commonly referred to Rac-RhoGDI dimer, present in phagocytes, represents Rac in complex with RhoGDI1.
The crystal structures of RhoGDI1 in complex with RhoA,Citation118 CDC42Hs,Citation119 and Rac1Citation120 were solved and provided a wealth of information on the structure of these complexes and on the residues in RhoGDI and in the partner Rho GTPases, involved. RhoGDIs interact with Rho GTPases by means of 2 regions: an N-terminal “regulatory arm” (residues 5–55), which inhibits nucleotide exchange by contacting the switch I and II regions in the GTPase, and a C-terminal region (residues 70–200), adopting an immunoglobulin-like fold, which forms a “pocket,” lined with hydrophobic residues, responsible for the binding of the geranylgeranyl group of the GTPase. In the Rac1-RhoGDI1 complex, residue T35 in switch I of Rac (a key residue for coordination of Mg2+ and nucleotide binding) forms a hydrogen bond with D45 in RhoGDI, and R66, in switch II, makes hydrogen bonds with P30, A31, and P185, in RhoGDI. H103 and H104 in Rac interact with residues D184 and Y27, respectively, in RhoGDI. It is of interest that Rac1 peptides, comprising residues H103 and H104, were found to prevent oxidase activation in vitro in “peptide walking” experiments in which the Rac1 component was represented by the Rac1-RhoGDI complex.Citation66 No evidence was found for the postulated involvementCitation119 of the insert region of Rac in interaction with RhoGDI. The N-terminal region of RhoGDI, disordered when in solution, folds into two anti-parallel α-helices when bound to Rac; the geranylgeranyl-binding pocket comprises nine β-strands, forming two antiparallel sheets (see for a schematic representation of the structure of the Rac1-RhoGDI1 complex). In summary, the N-terminal part of RhoGDI is responsible for GDP/GTP cycling whereas the C-terminal pocket determines cytosol/membrane partitioning of Rac.
Figure 7. Ribbon representation of the Rac1−RhoGDI complex. Rac1 is depicted in blue and RhoGDI in green. The switch I and II regions in Rac1 are highlighted in yellow and red, respectively. The GDP molecule and geranylgeranyl group are represented in ball and sticks. Mg2+ is shown in gray. Loop (58−66) in GDI (dashed line) is not visible in the crystallographic structure (reprinted with permission from ref. Citation120. Copyright 2001, American Chemical Society).
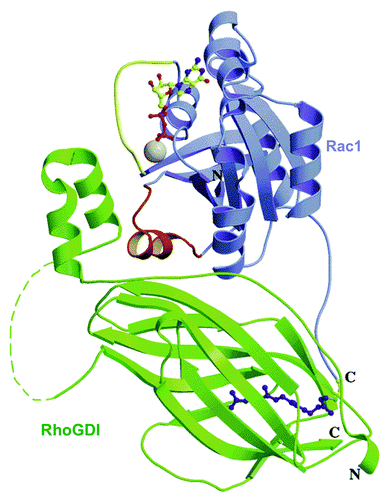
In the context of oxidase activation, RhoGDI has three functions: (1) Maintains Rac in the GDP-bound (inactive) form, thus, preventing GDP dissociation by GEF; (2) Conserves Rac in soluble form in the cytosol by forming a high affinity complex with the isoprenyl tail of Rac; release from RhoGDI enables binding of Rac to the membrane, interaction with GEF, conversion to the GTP-bound form, and interaction with down-stream effectors, as typified by binding to p67phox, and (3) Interacts with lower affinity with the GTP-bound form of Rac, blocking both spontaneous and GTPase activating protein (GAP)-mediated GTP hydrolysis.Citation121
It should be recalled that, on all occasions, Rac1 and Rac2 were isolated from the cytosol of phagocytes as Rac-RhoGDI complexes,Citation32,Citation51-Citation56,Citation90,Citation91,Citation122 although, in the absence of complete molecular characterization, this was not always realized at the time.Citation53,Citation55
It is firmly established that dissociation of Rac-RhoGDI complexes is an obligatory step in oxidase activation. The precise mechanism of this process has yet to be fully understood. Bokoch and coworkersCitation122 have first proposed that certain fatty acids and anionic phospholipids, in soluble form, might be responsible for dissociation, a proposal that seemed reasonable in light of the oxidase activating ability of anionic amphiphiles in the cell-free systemCitation14 and the reports on amphiphile-induced Rac translocation to the membraneCitation79,Citation93 but the equal or superior activity of neutral lipids was difficult to explain. More recent models were based on a modifying effect on RhoGDI itself, such as phosphorylation of specific serines in the immunoglobulin-like region, by a p21-activated kinase (PAK),Citation123 or on a coordinated set of processes affecting the membrane and Rac.Citation113,Citation124 Thus, it was shown that RacGDP- and RacGTP-RhoGDI complexes generated from recombinant Rac, prenylated enzymatically, and recombinant RhoGDI were dissociated on encountering liposomes containing anionic phospholipids.Citation113 The complexes were able to elicit cell-free oxidase activation, when added to native membrane liposomes together with p67phox and p47phox, the extent of activation being enhanced by enriching the membrane liposomes with anionic phospholipids.Citation113 Next, an in vitro model was designed meant to mimic membrane phospholipid remodeling during phagocyte stimulation in vivo.Citation124 Liposomes of “resting cell membrane” composition (less than 20 mol % monovalent anionic phospholipids), supplemented with 1 mol % of the polyvalent anionic phosphoinositide, PtdIns(3,4,5)P3, in conjunction with constitutively active forms of the Rac GEFs Trio or Tiam1, and a non-hydrolysable GTP analog, were shown to cause dissociation of Rac1GDP-RhoGDI complexes, GDP to GTP exchange on Rac1, and binding of Rac1GTP to the liposomes. Dissociation of Rac1GDP-RhoGDI complexes was found to be correlated with the affinity of particular GEF constructs for PtdIns(3,4,5)P3, and involved GEF-mediated GDP to GTP exchange on Rac1. This model is illustrated in . The prevalent hypothesis is that the Rac-RhoGDI complex translocates to the membrane as such and the process of dissociation occurs fully at the level of the membrane. The attraction to the membrane appears to be essentially electrostatic, the polybasic region of Rac interacting with the anionic inner aspect of the membrane enriched with de novo generated phosphoinositides. A second competitive event for the isoprenyl tail of Rac is that between the hydrophobic pocket of RhoGDI and the lipid bilayer of the membrane. A recent report introduces yet another component, represented by the electrostatic repulsion between the negatively charged residues of the N-terminal segment of RhoGDI and the anionic inner aspect of the membrane, favoring dissociation of RhoGDI from the membrane-attached Rac.Citation125
Figure 8. Extrapolation of the in vitro mechanism of Rac-RhoGDI dissociation by the cooperative action of PtdIns(3,4,5)P3-containing liposomes, GTP and GEF, to events hypothesized to occur in the course of oxidase activation in the intact phagocyte. The proposed sequence of events is: (1) In the plasma membrane of the resting phagocyte, represented by a phospholipid composition of less than 20% anionic lipids, the Rac-RhoGDI complexes are in the cytosol. (2 and 3) Upon phagocyte stimulation, PtdIns(3,4,5)P3 is generated on the cytosolic aspect of the plasma membrane by PI3K, resulting in a marked increase in negative charge. A small proportion of the Rac-RhoGDI complexes dissociates spontaneously and RacGDP translocates to the PtdIns(3,4,5)P3-enriched plasma membrane. (4) A marked enhancement of the dissociation of Rac-RhoGDI complexes takes place upon the translocation of a Rac-specific GEF to the plasma membrane, by virtue of the affinity of the PH domain of GEF for PtdIns(3,4,5)P3. This leads to GDP to GTP exchange on Rac, also bound to the plasma membrane, preventing reassociation with RhoGDI due to the lower affinity of the latter for RacGTP. (5) Membrane-associated RacGTP interacts with down-stream effectors, exemplified by p67phox. Intrinsic or GAP-enhanced GTPase activity leads to the conversion of RacGTP to RacGDP, which reassociates with RhoGDI and is returned to the cytosol. “Minus” symbols represent the negative charge of the phospholipids on the cytosolic aspect of the plasma membrane; “plus” symbols represent the positive charge of the polybasic C-terminus of Rac, and “ip” stands for isoprenyl (reproduced from ref. Citation124).
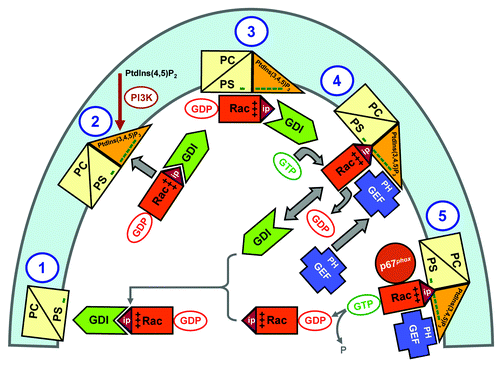
An appropriate illustration of the potential of RhoGDI to also act as an inhibitor of oxidase activation is offered by experiments in an amphiphile-independent cell-free system, consisting of membrane, p67phox and prenylated Rac; the presence of RhoGDI in molar excess to Rac prevents oxidase activation, when added before assembly, but not when added after the completion of assembly.Citation7,Citation10
“Beefing up” Rac
The fact that Rac1 and Rac2 support oxidase activation in vivo, when in the GTP-bound form is established with the strength of a dogma. The occasions when Rac1, in the GDP-bound form, was found capable of a certain degree of activation were all described in cell-free systems, in which artifactual elements cannot be totally excluded, one of which being the use of high concentrations of Rac and of its p67phox partner.Citation10,Citation14,Citation79,Citation80,Citation91,Citation92 In the physiological situation, Rac in complex with RhoGDI is in the GDP-bound form but interaction with p67phox is conditional on nucleotide exchange to GTP.
GDP to GTP exchange on Rho GTPases, such as Rac, is an enzymatic process performed by GEFs belonging to the Dbl homology (DH) domain family (for reviews, see refs. Citation126 and127). A brief description of the mechanism of nucleotide exchange is that the process is initiated by recognition by GEF of the GDP-bound form of Rac, which is followed by the dissociation of GDP and the formation of a transient binary complex of [GEF - nucleotide-free Rac]; this is, then, dissociated by GTP (found in cells in a 10-fold excess over GDP), which binds to Rac. DH family GEFs, thus, act by destabilizing the Rac-GDP bond and stabilizing the nucleotide-depleted Rac in a transient complex with GEF. The DH domain is essential for the catalytic action but Rho GEFs also possess a pleckstrin homology (PH) domain, adjacent and C-terminal to the DH domain, the DH-PH module representing the minimal structure catalyzing nucleotide exchange. PH domains regulate the activity of DH domains, bind polyphosphoinositides, assist in targeting GEFs to specific intracellular locations, and can also act as autoinhibitory elements by “covering” DH domains, an interaction which is blocked by the binding to PH domains of PI3K-derived PtdIns(3,4,5)P3 (reviewed in ref. Citation127).
Our group investigated the effect of GEFs on cell-free oxidase activation by making use of the amphiphile-independent system, which, in addition to the membrane, contains only two variables: prenylated Rac and p67phox.Citation10,Citation14 In these experiments, we utilized the Rac1-specifc DH domain family GEFs, Trio and Tiam1.Citation128 It was shown that Trio and Tiam1 act as GEFs for Rac1 but not for the closely related Rho GTPase CDC42Hs. Residues 53–72 on Rac1 are required for specific recognition and nucleotide exchange on Rac1, with W56 being the critical residue; mutating F56, in CDC42Hs, to W rendered CDC42Hs responsive to Rac1-specific GEFs.Citation128 We generated two recombinant Rac1-specific GEF segments; these were TrioN, consisting of the N-terminal DH and PH domains of Trio, and Tiam1(C374), consisting of the only DH domain and the adjacent C-terminal PH domain of Tiam1. It was found that both GEFs enabled oxidase activation in a cell-free system consisting of membrane, p67phox, prenylated native Rac1 (shown to be exclusively in the GDP-bound form), GTP, and GEF.Citation129,Citation130 Rac1 mutants unable to either bind or respond to GEFs (most notably, a W56F mutant) failed to support oxidase activation; also, a TrioN double mutant in the N-terminal DH domain, which does not stimulate nucleotide exchange on Rac1, was incapable of supporting oxidase activation in vitro.Citation129 Rac1-specific GEFs were also shown to be essential for oxidase activation supported by Rac1GDP-RhoGDI complexes in vitro, in a system containing membranes, p67phox, and GTP; enrichment of the membranes with PtdIns(3,4,5)P3 further enhanced activation, provided that the GEF construct contained a PH domain with affinity for PtdIns(3,4,5)P3.Citation124
Although GEFs are capable of binding to and acting on nonprenylated Rac,Citation128,Citation129 prenylated RacGDP, bound to membrane-mimicking liposomes, is exchanged to RacGTP by Tiam1 much more efficiently than when not present in a membrane-like environment.Citation131 The membrane may act as a template to concentrate and reorient both Rac and GEF. This finding argues in favor of the GDP to GTP exchange on Rac, in the course of oxidase activation, taking place at the level of the membrane, following its translocation from the cytosol. An interesting situation developed in GGTase-I-deficient mouse macrophages,Citation114 in which Rac1 was not prenylated but, nevertheless, translocated to the membrane and was exchanged to GTP, a strong argument in favor of membrane localization, and not prenylation, being important for the action of GEFs on Rac.
A considerable effort was made in order to identify Rac-specific GEFs acting in human and animal neutrophils. A leading candidate is the P-Rex family of GEFs, consisting of several members (P-Rex1, P-Rex2, P-Rex2B).Citation132-Citation134 P-Rex family GEFs are synergistically activated by PtdIns(3,4,5)P3 and by the Gβγ subunit of the heterotrimeric G protein complex, linked to G protein-coupled receptors, and it was suggested that P-Rex acts as a “coincidence detector” for the 2 signals.Citation133 PtdIns(3,4,5)P3 was shown to bind to the PH domain whereas the Gβγ subunit binds to the DH domain; thus, the DH/PH domain tandem is sufficient for both PtdIns(3,4,5)P3- and Gβγ subunit-initiated GEF activity.Citation132 P-Rex-1 was first isolated from pig neutrophil cytosol and was shown to be activated by PtdIns(3,4,5)P3 in vitro; also, in the whole cell, membrane receptors can activate the GEF activity of P-Rex1 in a PI3K (PtdIns(3,4,5)P3)-dependent manner.Citation133 A differentiated human promyeloytic cell line treated with P-Rex1 antisense oligonucleotide showed a marked fall in P-Rex1 content and the C5a-stimulated ROS production fell by 40%.Citation133 In a study based on the oxidase-related functions in neutrophils of P-Rex1 knockout mice, it was found that disruption of the P-Rex1 gene had no effect on Rac1 activation but diminished Rac2 activation ( = level of Rac2GTP) in response to the chemoattractant N-formyl-methionyl-leucyl-phenylalanine (fMLF); O2.- production was reduced in response to fMLF but not to lipopolysaccharide (LPS) and phorbol myristate acetate (PMA).Citation134 In a similar study, it was found that in mouse neutrophils, fMLF-stimulated O2.- generation is regulated not only by the P-Rex1 but also by the GEF, Vav1, in a synergistic manner; mice lacking both P-Rex1 and Vav1 showed the most severely impaired response and the most marked reduction in GDP to GTP exchange in both Rac1 and Rac2.Citation135 The multiplicity of GEFs acting on Rac in phagocytes and other cells and the central place of PI3K and of its product, PtdIns(3,4,5)P3, in activation of Rac GEFs is reviewed in reference Citation136. Studies done in cell lines transfected with oxidase components and GEFs and in murine neutrophils deficient in Vav1 revealed the multiplicity and complexity of the in vivo action of GEFs (Vav1, Vav2, Tiam1), a central physiologically relevant role being assigned to Vav1.Citation137,Citation138 The number and methodological intricacy of whole cells studies dealing with the role of Rac GEFs in oxidase activation makes a full rendering of these beyond the scope of this review.
Mind the GAP
GAPs augment the intrinsic GTPase activity of GTPases and are thus potentially important regulators of oxidase activation, via downregulation of RacGTP-dependent processes. The multifaceted role of GAPs in the suppression or termination of GTPase dependent processes, in general, is reviewed in reference Citation139. GAPs acting on Rho GTPases share a conserved RhoGAP domain of about 150 amino acids and their number exceeds that of their target Rho GTPases (reviewed in ref. Citation140). One of the first indications that RhoGAPs can modulate oxidase activation was the finding that neutrophils from mice deficient in the breakpoint cluster region protein (bcr), previously identified as a GAP acting on Rac, exhibited markedly increased O2.- production in response to stimulation by both PMA and fMLF.Citation141 Heyworth et al.Citation142 were the first to present in vitro evidence for downregulation of oxidase activity by 2 Rho GAPs (p190RhoGAP and p50RhoGAP) by describing the inhibition of cell-free oxidase activation by the 2 Rho GAPs; GAPs were inactive when added after the completion of oxidase assembly and were also ineffective on Rac1 Q61L (a mutant incapable of hydrolysing GTP) and on Rac preloaded with the nonhydrolysable analog, GTPγS. Human neutrophils were found to contain several Rho GAPs; p190RhoGAP and bcr were present in the cytosol whereas p50RhoGAP was found in both membrane and cytosol. Cell-free oxidase activity was found to be diminished by both endogenous membrane GAP and by exogenous (added) bcr and p50RhoGAP; as expected, RhoGAPs were ineffective when Rac was exchanged to the nonhydrolysable GTPγS and less effective when Rac was nonprenylated.Citation143 It was proposed that, in the course of oxidase assembly in the intact cell, RacGTP is accessible and, thus, potentially, modulated by both cytosolic and membrane-localized GAPs; in the assembled oxidase complex in the membrane, RacGTP is no longer accessible to cytosolic GAP but membrane-associated GAP maintained its ability to downregulate post-assembly oxidase activity.Citation144
A unique group of RhoGAPs is represented by bacterial GAPs, which share neither sequence similarity nor structural resemblance with eukaryotic GAPs (reviewed in ref. Citation145). Bacterial GAPs are used by a variety of pathogens to enhance their survival by preventing the cytoskeleton-related, Rho GTPases-dependent events, required for internalization by phagocytes. Another potential advantage for bacterial virulence would be the neutralization of ROS production by phagocytes by depleting Rac of GTP. Our group investigated the effect of a recombinant protein, corresponding to the GAP domain of AexT toxin of Aeromonas salmonicida, on oxidase activation in vitro (Y. Litvak, S. Molshanski-Mor, Z. Selinger, and E. Pick, unpublished data). We found that addition of AexT GAP inhibited oxidase activation in both amphiphile- and p47phox-dependent, and amphiphile- and p47phox-independent cell-free systems, supported by nonprenylated and prenylated Rac1GTP, respectively, but not by Rac1 exchanged to the nonhydrolysable analog, GMPPNP. In resemblance to the results described in reference Citation144, GAP added after assembly was capable of reverting activation supported by prenylated Rac1GTP but not that by nonprenylated Rac1GTP, probably due to the complex stabilizing effect of p47phox in the latter situation.
The poor man's homemade GTP: NDPK and the oxidase
Nucleoside diphosphate kinase (NDPK) transfers a phosphate group from a nucleoside triphosphate to a nucleoside diphosphate. Evidence was presented, as early as 1988, that cell-free oxidase activation with membranes and cytosol of differentiated HL60 cells was enhanced not only by GTP but also by ATP and it was proposed that NDPK, present in the membrane, phosphorylates GDP to generate GTP.Citation146 It is of interest that this proposal was made before the identification of Rac as the oxidase-related GTPase and that the location of NDPK and the fact that it was, probably, acting on GDP bound to a component present in the reaction, were predicted correctly. Support for the involvement of a NDPK in cell-free oxidase activation in a human membrane/cytosol system was provided in two more publications.Citation147,Citation148 The first was based on results obtained at a time when the participation of a GTPase was assumed but not proven.Citation147 In the second report, the already existing evidence for the roles of Rac1 and Rac2 in oxidase activation was interpreted as these acting as mere enhancers of activation, and the focus was on the role of NDPK as a converter of free cytosolic GDP to GTP.Citation148
Our group confirmed the involvement of a membrane associated NDPK in oxidase activation by using a semi-recombinant cell-free system to dissect the complex mechanism by which exogenous ATP can replace GTP in supporting O2.- production.Citation130 This consisted of membranes, p67phox, prenylated Rac1GDP and the Rac GEFs Trio or Tiam1; oxidase activation was induced in the absence of an amphiphilic activator and p47phox. We found that oxidase activation involving Rac GEFs was supported not only by GTP but also by ATP. Nonhydrolysable GTP analogs were active whereas ATP analogs, incapable of γ-phosphoryl transfer, were inactive. The ability of ATP to support GEF-induced oxidase activation was explained by ATP serving as γ-phosphoryl donor for a membrane-associated NDPK (B isoform), converting GDP to GTP. NDPK acted on GDP derived by dissociation from prenylated Rac1GDP, mediated by GEF, and the newly formed GTP was bound to Rac. NDPK and GEF appeared to be functionally linked in the sense that the availability of GDP, serving as substrate for NDPK, was dependent on the level of activity of GEF. This model is illustrated in .
Figure 9. Schematic representation of the involvement of a membrane localized NDPK in NADPH oxidase activation. The scheme proposes the existence of a linkage between GEF-induced dissociation of GDP from RacGDP and γ-phosphoryl transfer from ATP to free GDP, by NDPK, resulting in the formation of GTP, which is then bound to Rac, to generate RacGTP. It is also suggested that 3 components participating in GDP dissociation and GDP phosphorylation (prenylated Rac, GEF, and NDPK) are attached to the membrane and are, possibly, co-localized in micro-domains. A hypothetical integration of GEF and NDPK with the subunits of the NADPH oxidase complex is also proposed (modified from ref. Citation130).
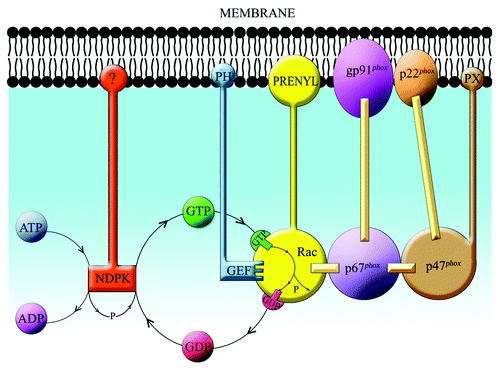
Rac CGD: More Authors than Patients
To the best of my knowledge, only one patient with a Rac-focused form of CGD was described, so far.Citation149-Citation151 The male infant patient had severe bacterial infections and poor wound healing and his neutrophils exhibited decreased chemotaxis in response to zymosan-activated serum; O2.- production, in response to fMLF, was severely reduced. The cells contained half the amount of Rac2 present in normal controls and Rac had a D57N mutation. D57 is conserved in all GTPases and is important for binding of Mg2+ and for GTP hydrolysis. Cytosol from the patient’s neutrophils did not support cell-free oxidase activation, a defect that could be corrected by the addition of normal Rac2. Addition of Rac2 D57N to a cell free system containing normal cytosol blocked oxidase activation, a behavior seen with dominant negative mutants. The D57N mutant Rac did not bind GTP but did bind GDP normally. The mutant bound Rac GEFs, such as Trio, but did not respond to it by GDP to GTP exchange and it seems likely that its dominant negative behavior in vivo was due to sequestration of endogenous GEFs, reducing their availability to other Rho GTPases.
Life without Rac
Rac2 deficient mice were created and rac2−/− neutrophils were examined for oxidase-related functions.Citation152 O2.- production by bone marrow neutrophils of Rac2-deficient mice in response to PMA was markedly reduced but the response of elicited inflammatory neutrophils recovered from the peritoneal cavity, was normal. The lack of response of Rac2-deficient bone marrow neutrophils could be partially corrected by preincubation with the cytokine, tumor necrosis factor α (TNF α). These results can be explained by the fact that Rac2-deficient neutrophils, “activated” in vivo or in vitro, compensate for the lack of Rac2 by recruiting Rac1. In a follow-up study, it was found that O2.- production by bone marrow and elicited peritoneal neutrophils of rac2−/− mice was normal in response to opsonized zymosan but much reduced in response to IgG-coated red cells and fMLF, indicating that Rac2 is key to the mediation of oxidase activation in neutrophils, downstream of chemoattractant and Fcγ receptors.Citation153 In a study in mice, in which rac1 was deleted specifically in the granulocyte/monocyte lineage (overall Rac1 deletion results in embryonic lethality), O2.- production by bone marrow neutrophils was normal, in response to both PMA and fMLF, as opposed to rac2−/− mice, the cells of which generated diminished amounts of O2.-.Citation154 These studies demonstrate that, at least in mouse neutrophils, both Rac1 and Rac2 are required for functions related to the cytoskeleton but Rac2 is specifically involved in the activation of the oxidase.
Anti-Rac
In the light of the absolute requirement for Rac for oxidase activation in vivo and in vitro, it seems logical to assume that inhibitors focused on Rac will prevent oxidase activation. The accent in the development of such inhibitors was placed on small molecular weight compounds, with synthetic peptides occupying a central position.
Rac inhibitors were designed mainly for 2 purposes: (1) As means to understand the mechanism of Rac action, in particular, and of oxidase assembly, in general, and (2) As potential therapeutic agents, to be used in disease situations in which excessive production of ROS is a pathogenic mechanism.
The use of peptides as inhibitors targeting Rho GTPases is reviewed in reference Citation155 and the strategies followed for the identification of peptides to act as inhibitors of the oxidase, in general, is reviewed in reference Citation156. A brief overview of oxidase inhibitors registered as commercial patents is provided by reference Citation157.
Rac peptides, mapping with the C-terminus of Rac1, were found to inhibit oxidase activation in a cell-free system and the initial belief that inhibition was sequence-specificCitation101 was replaced by the interpretation that it was based on the overall positive charge of the peptides,Citation102 a finding that contributed to the understanding of the importance of the polybasic region of Rac in oxidase assembly. Overlapping peptides, 15 residues-long and with a 13-residues overlap, covering the full-length of Rac1, were tested as inhibitors of cell-free oxidase activation and the detected clusters of inhibitory peptides contributed greatly to the identification of functional domains in Rac.Citation66 Rac peptides are inhibitory only when added at the initiation of oxidase assembly and are less and less inhibitory as the process of assembly progresses.Citation66,Citation102
Following the definition of the 2 pathways of oxidase activation in vitro, reflecting the electrostatic and hydrophobic attachment of Rac to the membrane,Citation103 it became clear that inhibitors are very useful in pointing to one or the other mechanism as being involved. Thus, Rac1 C-terminal peptides and the cationic antibiotic neomycin were effective in inhibiting oxidase activation involving electrostatic binding of Rac to the membrane,Citation7,Citation79,Citation102,Citation103 whereas inhibition by RhoGDI and phospholipid vesicles pointed to a predominantly hydrophobic (Rac prenylation-dependent) mechanism.Citation7,Citation10
A target protein of Rac is PAK,Citation61 and residues 65–137 of PAK represent the Rac-binding domain. This segment of PAK was found to be an effective dose-dependent inhibitor of oxidase activation in vitro, supported by both nonprenylated and prenylated Rac, probably by competing with p67phox for binding to Rac (p67phox and PAK bind to identical regions in RacCitation61).Citation79,Citation103 A remarkable feature of this inhibitor is that it is active also after completion of oxidase assembly, a finding suggesting that it might promote the dissociation of the assembled complex.Citation79,Citation103 Another example of inhibition by a Rac target protein is Nox2, found to bind Rac2 at Nox2 residues 419–430; a Nox2 peptide corresponding to residues 419–430 inhibited cell-free oxidase activation and a cell-permeant version of the peptide inhibited O2.- production by neutrophils stimulated by PMA or fMLF.Citation77 A related type of inhibition of Rac-dependent ROS formation is that by CDC42Hs, which competes with Rac for binding to Nox2; CDC42Hs inhibited O2.- production in a cell-free system and in a transfected cell line and, reciprocally, inhibition of CDC42Hs activity in neutrophils enhanced the fMLF-stimulated O2.- production.Citation76 A related situation, resting on a different mechanism, is the inhibition of Rac-dependent O2.- production by differentiated HL60 cells by the expression of the dominant negative CDC42Hs mutant T17N, probably by sequestration of a Rac GEF.Citation158
A promising approach to interference with the Rac - p67phox interaction is to perform an in silico screen for small molecules targeting the site in p67phox to which Rac binds; this approach yielded a compound (Phox-I1) which inhibited O2.- production by mouse and human neutrophils, with IC50 values in the low micromolar range.Citation74
Finally, a little explored path to inhibition of ROS production is the pretreatment of cells with Rac antisense oligonucleotides; in at least one case, this was found to reduce the Rac content in Epstein-Barr virus transformed B lymphocytes and to cause inhibition of O2.- production in response to cross-linking of surface immunoglobulin or exposure to PMA.Citation159
Brief Conclusion
The most remarkable fact about the involvement of Rac GTPases in oxidase activation in phagocytes is that a highly specialized enzyme complex, composed of cell-specific components, and performing a particular function, has borrowed a “Jack of all trades” ubiquitous protein as an essential member of the complex. This unique situation was predicted, quite some time before the identification of Rac as a member of the oxidase complex, by the following sentence in the Discussion of reference Citation49: “The relative ubiquity of σ1 (later found to be the Rac1-RhoGDI complex) suggests that it is unlikely to represent a specific component of the electron transport chain of the NADPH oxidase but rather a protein related to oxidase activation. The same protein might, in nonphagocytic cells, have a different function, such as signal transduction to or modulation of another enzyme.”
Abbreviations: | ||
ROS | = | reactive oxygen species |
O2.- | = | superoxide |
RhoGDI | = | Rho GDP dissociation inhibitor |
GEF | = | guanine nucleotide exchange factor |
GAP | = | GTPase activating protein |
SDS | = | sodium dodecyl sulfate |
LiDS | = | lithium dodecyl sulfate |
TPR | = | tetratricopeptide repeat |
GTPγS | = | guanosine 5′-O-(3-thiotriphosphate) |
GMPPNP | = | guanylyl imidodiphosphate |
GDPβS | = | guanosine 5′-O-(2-thiodiphosphate) |
FRET | = | fluorescence resonance energy transfer |
SH3 | = | Src homology 3 |
CGD | = | chronic granulomatous disease |
mant-GMPPNP | = | 2'-(or 3′)-O-(N-methylanthraniloyl)-GMPPNP |
mant-GDP | = | 2'-(or 3′)-O-(N-methylanthraniloyl)-GDP |
PC | = | phosphatidylcholine |
PA | = | phosphatidic acid |
PG | = | phosphatidylglycerol |
PS | = | phosphatidylserine |
PI | = | phosphatidylinositol |
PtdIns(4)P | = | phosphatidylinositol 4-monophosphate |
PtdIns(4,5)P2 | = | phosphatidylinositol 4,5-bisphosphate |
PtdIns(3,4,5)P3 | = | phosphatidylinositol 3,4,5-trisphosphate |
PI3K | = | phosphoinositide 3-kinase |
GGTase-I | = | geranylgeranyltransferase type I |
DH | = | Dbl homology |
PH | = | pleckstrin homology |
fMLF | = | N-formyl-methionyl-leucyl-phenylalanine |
LPS | = | lipopolysaccharide |
PMA | = | phorbol myiristate acetate |
bcr | = | breakpoint cluster region |
NDPK | = | nucleoside diphosphate kinase |
TNF α | = | tumor necrosis factor α |
PAK | = | p21-activated kinase |
Disclosure of Potential Conflicts of Interest
No potential conflicts of interest were disclosed.
Acknowledgments
Our own research described in this report was supported by the Julius Friedrich Cohnheim-Minerva Center for Phagocyte Research, the Ela Kodesz Institute of Host Defense against Infectious Diseases, Israel Science Foundation Grants 428/01, 19/05, 49/09, and 300/13, the Roberts-Guthman Chair in Immunopharmacology, the Walter J. Levy Benevolent Trust, the Roberts Fund, the Milken–Lowell Fund, the Wallis Foundation, the Rubanenko Fund, and the Joseph and Shulamit Salomon Fund.
References
- Nauseef WM. How human neutrophils kill and degrade microbes: an integrated view. Immunol Rev 2007; 219:88 - 102; http://dx.doi.org/10.1111/j.1600-065X.2007.00550.x; PMID: 17850484
- Nauseef WM. Assembly of the phagocyte NADPH oxidase. Histochem Cell Biol 2004; 122:277 - 91; http://dx.doi.org/10.1007/s00418-004-0679-8; PMID: 15293055
- Quinn MT, Gauss KA. Structure and regulation of the neutrophil respiratory burst oxidase: comparison with nonphagocyte oxidases. J Leukoc Biol 2004; 76:760 - 81; http://dx.doi.org/10.1189/jlb.0404216; PMID: 15240752
- Groemping Y, Rittinger K. Activation and assembly of the NADPH oxidase: a structural perspective. Biochem J 2005; 386:401 - 16; http://dx.doi.org/10.1042/BJ20041835; PMID: 15588255
- Sumimoto H. Structure, regulation and evolution of Nox-family NADPH oxidases that produce reactive oxygen species. FEBS J 2008; 275:3249 - 77; http://dx.doi.org/10.1111/j.1742-4658.2008.06488.x; PMID: 18513324
- Kreck ML, Freeman JL, Abo A, Lambeth JD. Membrane association of Rac is required for high activity of the respiratory burst oxidase. Biochemistry 1996; 35:15683 - 92; http://dx.doi.org/10.1021/bi962064l; PMID: 8961931
- Sarfstein R, Gorzalczany Y, Mizrahi A, Berdichevsky Y, Molshanski-Mor S, Weinbaum C, Hirshberg M, Dagher M-C, Pick E. Dual role of Rac in the assembly of NADPH oxidase, tethering to the membrane and activation of p67phox: a study based on mutagenesis of p67phox-Rac1 chimeras. J Biol Chem 2004; 279:16007 - 16; http://dx.doi.org/10.1074/jbc.M312394200; PMID: 14761978
- Freeman JL, Lambeth JD. NADPH oxidase activity is independent of p47phox in vitro.. J Biol Chem 1996; 271:22578 - 82; http://dx.doi.org/10.1074/jbc.271.37.22578; PMID: 8798426
- Koshkin V, Lotan O, Pick E. The cytosolic component p47(phox) is not a sine qua non participant in the activation of NADPH oxidase but is required for optimal superoxide production. J Biol Chem 1996; 271:30326 - 9; http://dx.doi.org/10.1074/jbc.271.48.30326; PMID: 8939991
- Gorzalczany Y, Sigal N, Itan M, Lotan O, Pick E. Targeting of Rac1 to the phagocyte membrane is sufficient for the induction of NADPH oxidase assembly. J Biol Chem 2000; 275:40073 - 81; http://dx.doi.org/10.1074/jbc.M006013200; PMID: 11007780
- Bedard K, Krause K-H. The NOX family of ROS-generating NADPH oxidases: physiology and pathophysiology. Physiol Rev 2007; 87:245 - 313; http://dx.doi.org/10.1152/physrev.00044.2005; PMID: 17237347
- Bromberg Y, Pick E. Unsaturated fatty acids stimulate NADPH-dependent superoxide production by cell-free system derived from macrophages. Cell Immunol 1984; 88:213 - 21; http://dx.doi.org/10.1016/0008-8749(84)90066-2; PMID: 6090027
- Bromberg Y, Pick E. Activation of NADPH-dependent superoxide production in a cell-free system by sodium dodecyl sulfate. J Biol Chem 1985; 260:13539 - 45; PMID: 2997168
- Molshanski-Mor S, Mizrahi A, Ugolev Y, Dahan I, Berdichevsky Y, Pick E. Cell-free Assays: The Reductionist Approach to the Study of NADPH Oxidase Assembly, or All You Wanted to Know About Cell-Free Assays but Did Not Dare to Ask. In Quinn MT, DeLeo FR, Bokoch GM, editors. Neutrophil Methods and Protocols.Totowa NJ: Humana Press; 2007. p. 385-428.
- Abo A, Boyhan A, West I, Thrasher AJ, Segal AW. Reconstitution of neutrophil NADPH oxidase activity in the cell-free system by four components: p67-phox, p47-phox, p21rac1, and cytochrome b-245. J Biol Chem 1992; 267:16767 - 70; PMID: 1512217
- Seifert R, Rosenthal W, Schultz G. Guanine nucleotides stimulate NADPH oxidase in membranes of human neutrophils. FEBS Lett 1986; 205:161 - 5; http://dx.doi.org/10.1016/0014-5793(86)80886-9; PMID: 3017756
- Seifert R, Schultz G. Fatty-acid-induced activation of NADPH oxidase in plasma membranes of human neutrophils depends on neutrophil cytosol and is potentiated by stable guanine nucleotides. Eur J Biochem 1987; 162:563 - 9; http://dx.doi.org/10.1111/j.1432-1033.1987.tb10676.x; PMID: 3549290
- Seifert R, Schultz G. Reversible activation of NADPH oxidase in membranes of HL-60 human leukemic cells. Biochem Biophys Res Commun 1987; 146:1296 - 302; http://dx.doi.org/10.1016/0006-291X(87)90790-X; PMID: 3113431
- Gabig TG, English D, Akard LP, Schell MJ. Regulation of neutrophil NADPH oxidase activation in a cell-free system by guanine nucleotides and fluoride. Evidence for participation of a pertussis and cholera toxin-insensitive G protein. J Biol Chem 1987; 262:1685 - 90; PMID: 3027097
- Gabig TG, Eklund EA, Potter GB, Dykes JR 2nd. A neutrophil GTP-binding protein that regulates cell free NADPH oxidase activation is located in the cytosolic fraction. J Immunol 1990; 145:945 - 51; PMID: 2115550
- Ligeti E, Doussiere J, Vignais PV. Activation of the O2(.-)-generating oxidase in plasma membrane from bovine polymorphonuclear neutrophils by arachidonic acid, a cytosolic factor of protein nature, and nonhydrolyzable analogues of GTP. Biochemistry 1988; 27:193 - 200; http://dx.doi.org/10.1021/bi00401a029; PMID: 2831954
- Ligeti E, Tardif M, Vignais PV. Activation of O2.- generating oxidase of bovine neutrophils in a cell-free system. Interaction of a cytosolic factor with the plasma membrane and control by G nucleotides. Biochemistry 1989; 28:7116 - 23; http://dx.doi.org/10.1021/bi00443a050; PMID: 2554964
- Doussiere J, Pilloud MC, Vignais PV. Activation of bovine neutrophil oxidase in a cell free system. GTP-dependent formation of a complex between a cytosolic factor and a membrane protein. Biochem Biophys Res Commun 1988; 152:993 - 1001; http://dx.doi.org/10.1016/S0006-291X(88)80382-6; PMID: 3132160
- Aharoni I, Pick E. Activation of the superoxide-generating NADPH oxidase of macrophages by sodium dodecyl sulfate in a soluble cell-free system: evidence for involvement of a G protein. J Leukoc Biol 1990; 48:107 - 15; PMID: 2164554
- Orczi E. The Elusive Pimpernel. London: Hutchinson and Co; 1908.
- Quinn MT, Parkos CA, Walker L, Orkin SH, Dinauer MC, Jesaitis AJ. Association of a Ras-related protein with cytochrome b of human neutrophils. Nature 1989; 342:198 - 200; http://dx.doi.org/10.1038/342198a0; PMID: 2509942
- Bokoch GM, Quilliam LA, Bohl BP, Jesaitis AJ, Quinn MT. Inhibition of Rap1A binding to cytochrome b558 of NADPH oxidase by phosphorylation of Rap1A. Science 1991; 254:1794 - 6; http://dx.doi.org/10.1126/science.1763330; PMID: 1763330
- Eklund EA, Marshall M, Gibbs JB, Crean CD, Gabig TG. Resolution of a low molecular weight G protein in neutrophil cytosol required for NADPH oxidase activation and reconstitution by recombinant Krev-1 protein. J Biol Chem 1991; 266:13964 - 70; PMID: 1906890
- Quinn MT, Curnutte JT, Parkos CA, Mullen ML, Scott PJ, Erickson RW, Jesaitis AJ. Reconstitution of defective respiratory burst activity with partially purified human neutrophil cytochrome B in two genetic forms of chronic granulomatous disease: possible role of Rap1A. Blood 1992; 79:2438 - 45; PMID: 1315174
- Li Y, Yan J, De P, Chang H-C, Yamauchi A, Christopherson KW 2nd, Paranavitana NC, Peng X, Kim C, Munugalavadla V, et al. Rap1a null mice have altered myeloid cell functions suggesting distinct roles for the closely related Rap1a and 1b proteins. J Immunol 2007; 179:8322 - 31; PMID: 18056377
- Gabig TG, Crean CD, Mantel PL, Rosli R. Function of wild-type or mutant Rac2 and Rap1a GTPases in differentiated HL60 cell NADPH oxidase activation. Blood 1995; 85:804 - 11; PMID: 7833480
- Abo A, Pick E, Hall A, Totty N, Teahan CG, Segal AW. Activation of the NADPH oxidase involves the small GTP-binding protein p21rac1. Nature 1991; 353:668 - 70; http://dx.doi.org/10.1038/353668a0; PMID: 1922386
- Knoller S, Shpungin S, Pick E. The membrane-associated component of the amphiphile-activated, cytosol-dependent superoxide-forming NADPH oxidase of macrophages is identical to cytochrome b559. J Biol Chem 1991; 266:2795 - 804; PMID: 1847135
- Didsbury J, Weber RF, Bokoch GM, Evans T, Snyderman R. rac, a novel ras-related family of proteins that are botulinum toxin substrates. J Biol Chem 1989; 264:16378 - 82; PMID: 2674130
- Jaffe AB, Hall A. Rho GTPases: biochemistry and biology. Annu Rev Cell Dev Biol 2005; 21:247 - 69; http://dx.doi.org/10.1146/annurev.cellbio.21.020604.150721; PMID: 16212495
- Bokoch GM. Rac2. In: Zerial M, Huber LA, editors. Guidebook to the small GTPases. Oxford: Oxford University Press; 1995. p. 225-227.
- Self AJ, Hall A. Purification of recombinant Rho/Rc/G25K from Escherichia coli. In: Balch WE, Der CJ, Hall A, editors. Methods in Enzymology, San Diego: Academic Press; 1995. vol. 256, p. 3-10.
- Hirshberg M, Stockley RW, Dodson G, Webb MR. The crystal structure of human rac1, a member of the rho-family complexed with a GTP analogue. Nat Struct Biol 1997; 4:147 - 52; http://dx.doi.org/10.1038/nsb0297-147; PMID: 9033596
- Dagher MC, Fuchs A, Bourmeyster N, Jouan A, Vignais PV. Small G proteins and the neutrophil NADPH oxidase. Biochimie 1995; 77:651 - 60; http://dx.doi.org/10.1016/0300-9084(96)88180-6; PMID: 8589075
- Bokoch GM, Diebold BA. Current molecular models for NADPH oxidase regulation by Rac GTPase. Blood 2002; 100:2692 - 6; http://dx.doi.org/10.1182/blood-2002-04-1149; PMID: 12351373
- Diebold BA, Bokoch GM. Rho GTPases and the control of the oxidative burst in polymorphonuclear leukocytes. Curr Top Microbiol Immunol 2005; 291:91 - 111; http://dx.doi.org/10.1007/3-540-27511-8_6; PMID: 15981461
- Bokoch GM, Zhao T. Regulation of the phagocyte NADPH oxidase by Rac GTPase. Antioxid Redox Signal 2006; 8:1533 - 48; http://dx.doi.org/10.1089/ars.2006.8.1533; PMID: 16987009
- Hordijk PL. Regulation of NADPH oxidases: the role of Rac proteins. Circ Res 2006; 98:453 - 62; http://dx.doi.org/10.1161/01.RES.0000204727.46710.5e; PMID: 16514078
- Miyano K, Sumimoto H. Assessment of the role for Rho family GTPases in NADPH oxidase activation. In: Rivero F, editor. Rho GTPases; Methods and protocols. Berlin: Springer Science + Business Media; 2012. p. 195-212.
- Fujita I, Takeshige K, Minakami S. Characterization of the NADPH-dependent superoxide production activated by sodium dodecyl sulfate in a cell-free system of pig neutrophils. Biochim Biophys Acta 1987; 931:41 - 8; http://dx.doi.org/10.1016/0167-4889(87)90048-6; PMID: 2820510
- Volpp BD, Nauseef WM, Clark RA. Two cytosolic neutrophil oxidase components absent in autosomal chronic granulomatous disease. Science 1988; 242:1295 - 7; http://dx.doi.org/10.1126/science.2848318; PMID: 2848318
- Nunoi H, Rotrosen D, Gallin JI, Malech HL. Two forms of autosomal chronic granulomatous disease lack distinct neutrophil cytosol factors. Science 1988; 242:1298 - 301; http://dx.doi.org/10.1126/science.2848319; PMID: 2848319
- Bolscher BGJM, Denis SW, Verhoeven AJ, Roos D. The activity of one soluble component of the cell-free NADPH:O2 oxidoreductase of human neutrophils depends on guanosine 5′-O-(3-thio)triphosphate. J Biol Chem 1990; 265:15782 - 7; PMID: 2203787
- Pick E, Kroizman T, Abo A. Activation of the superoxide-forming NADPH oxidase of macrophages requires two cytosolic components--one of them is also present in certain nonphagocytic cells. J Immunol 1989; 143:4180 - 7; PMID: 2556480
- Sha’ag D, Pick E. Nucleotide binding properties of cytosolic components required for expression of activity of the superoxide generating NADPH oxidase. Biochim Biophys Acta 1990; 1037:405 - 12; http://dx.doi.org/10.1016/0167-4838(90)90044-G; PMID: 2155658
- Abo A, Pick E. Purification and characterization of a third cytosolic component of the superoxide-generating NADPH oxidase of macrophages. J Biol Chem 1991; 266:23577 - 85; PMID: 1660877
- Pick E, Gorzalczany Y, Engel S. Role of the rac1 p21-GDP-dissociation inhibitor for rho heterodimer in the activation of the superoxide-forming NADPH oxidase of macrophages. Eur J Biochem 1993; 217:441 - 55; http://dx.doi.org/10.1111/j.1432-1033.1993.tb18264.x; PMID: 8223583
- Knaus UG, Heyworth PG, Evans T, Curnutte JT, Bokoch GM. Regulation of phagocyte oxygen radical production by the GTP-binding protein Rac 2. Science 1991; 254:1512 - 5; http://dx.doi.org/10.1126/science.1660188; PMID: 1660188
- Knaus UG, Heyworth PG, Kinsella BT, Curnutte JT, Bokoch GM. Purification and characterization of Rac 2. A cytosolic GTP-binding protein that regulates human neutrophil NADPH oxidase. J Biol Chem 1992; 267:23575 - 82; PMID: 1331090
- Mizuno T, Kaibuchi K, Ando S, Musha T, Hiraoka K, Takaishi K, Asada M, Nunoi H, Matsuda I, Takai Y. Regulation of the superoxide-generating NADPH oxidase by a small GTP-binding protein and its stimulatory and inhibitory GDP/GTP exchange proteins. J Biol Chem 1992; 267:10215 - 8; PMID: 1316893
- Kwong CH, Malech HL, Rotrosen D, Leto TL. Regulation of the human neutrophil NADPH oxidase by rho-related G-proteins. Biochemistry 1993; 32:5711 - 7; http://dx.doi.org/10.1021/bi00072a029; PMID: 8504089
- Ando S, Kaibuchi K, Sasaki T, Hiraoka K, Nishiyama T, Mizuno T, Asada M, Nunoi H, Matsuda I, Matsuura Y, et al. Post-translational processing of rac p21s is important both for their interaction with the GDP/GTP exchange proteins and for their activation of NADPH oxidase. J Biol Chem 1992; 267:25709 - 13; PMID: 1464587
- Cordle A, Koenigsknecht-Talboo J, Wilkinson B, Limpert A, Landreth G. Mechanisms of statin-mediated inhibition of small G-protein function. J Biol Chem 2005; 280:34202 - 9; http://dx.doi.org/10.1074/jbc.M505268200; PMID: 16085653
- Zhao X, Carnevale KA, Cathcart MK. Human monocytes use Rac1, not Rac2, in the NADPH oxidase complex. J Biol Chem 2003; 278:40788 - 92; http://dx.doi.org/10.1074/jbc.M302208200; PMID: 12912997
- Diekmann D, Abo A, Johnston C, Segal AW, Hall A. Interaction of Rac with p67phox and regulation of phagocytic NADPH oxidase activity. Science 1994; 265:531 - 3; http://dx.doi.org/10.1126/science.8036496; PMID: 8036496
- Diekmann D, Nobes CD, Burbelo PD, Abo A, Hall A. Rac GTPase interacts with GAPs and target proteins through multiple effector sites. EMBO J 1995; 14:5297 - 305; PMID: 7489719
- Prigmore E, Ahmed S, Best A, Kozma R, Manser E, Segal AW, Lim L. A 68-kDa kinase and NADPH oxidase component p67phox are targets for Cdc42Hs and Rac1 in neutrophils. J Biol Chem 1995; 270:10717 - 22; http://dx.doi.org/10.1074/jbc.270.18.10717; PMID: 7738010
- Dorseuil O, Reibel L, Bokoch GM, Camonis J, Gacon G. The Rac target NADPH oxidase p67phox interacts preferentially with Rac2 rather than Rac1. J Biol Chem 1996; 271:83 - 8; http://dx.doi.org/10.1074/jbc.271.1.83; PMID: 8550629
- Xu X, Barry DC, Settleman J, Schwartz MA, Bokoch GM. Differing structural requirements for GTPase-activating protein responsiveness and NADPH oxidase activation by Rac. J Biol Chem 1994; 269:23569 - 74; PMID: 8089125
- Kwong CH, Adams AG, Leto TL. Characterization of the effector-specifying domain of Rac involved in NADPH oxidase activation. J Biol Chem 1995; 270:19868 - 72; http://dx.doi.org/10.1074/jbc.270.34.19868; PMID: 7649999
- Joseph G, Pick E. “Peptide walking” is a novel method for mapping functional domains in proteins. Its application to the Rac1-dependent activation of NADPH oxidase. J Biol Chem 1995; 270:29079 - 82; http://dx.doi.org/10.1074/jbc.270.49.29079; PMID: 7493930
- Toporik A, Gorzalczany Y, Hirshberg M, Pick E, Lotan O. Mutational analysis of novel effector domains in Rac1 involved in the activation of nicotinamide adenine dinucleotide phosphate (reduced) oxidase. Biochemistry 1998; 37:7147 - 56; http://dx.doi.org/10.1021/bi9800404; PMID: 9585526
- Sehr P, Joseph G, Genth H, Just I, Pick E, Aktories K. Glucosylation and ADP ribosylation of rho proteins: effects on nucleotide binding, GTPase activity, and effector coupling. Biochemistry 1998; 37:5296 - 304; http://dx.doi.org/10.1021/bi972592c; PMID: 9548761
- Koga H, Terasawa H, Nunoi H, Takeshige K, Inagaki F, Sumimoto H. Tetratricopeptide repeat (TPR) motifs of p67(phox) participate in interaction with the small GTPase Rac and activation of the phagocyte NADPH oxidase. J Biol Chem 1999; 274:25051 - 60; http://dx.doi.org/10.1074/jbc.274.35.25051; PMID: 10455184
- Leusen JHW, de Klein A, Hilarius PM, Ahlin A, Palmblad J, Smith CIE, Diekmann D, Hall A, Verhoeven AJ, Roos D. Disturbed interaction of p21-rac with mutated p67-phox causes chronic granulomatous disease. J Exp Med 1996; 184:1243 - 9; http://dx.doi.org/10.1084/jem.184.4.1243; PMID: 8879195
- Ahmed S, Prigmore E, Govind S, Veryard C, Kozma R, Wientjes FB, Segal AW, Lim L. Cryptic Rac-binding and p21(Cdc42Hs/Rac)-activated kinase phosphorylation sites of NADPH oxidase component p67(phox).. J Biol Chem 1998; 273:15693 - 701; http://dx.doi.org/10.1074/jbc.273.25.15693; PMID: 9624165
- Diebold BA, Bokoch GM. Molecular basis for Rac2 regulation of phagocyte NADPH oxidase. Nat Immunol 2001; 2:211 - 5; http://dx.doi.org/10.1038/85259; PMID: 11224519
- Lapouge K, Smith SJ, Walker PA, Gamblin SJ, Smerdon SJ, Rittinger K. Structure of the TPR domain of p67phox in complex with Rac.GTP. Mol Cell 2000; 6:899 - 907; http://dx.doi.org/10.1016/S1097-2765(05)00091-2; PMID: 11090627
- Bosco EE, Kumar S, Marchioni F, Biesiada J, Kordos M, Szczur K, Meller J, Seibel W, Mizrahi A, Pick E, et al. Rational design of small molecule inhibitors targeting the Rac GTPase-p67(phox) signaling axis in inflammation. Chem Biol 2012; 19:228 - 42; http://dx.doi.org/10.1016/j.chembiol.2011.12.017; PMID: 22365606
- Heyworth PG, Bohl BP, Bokoch GM, Curnutte JT. Rac translocates independently of the neutrophil NADPH oxidase components p47phox and p67phox. Evidence for its interaction with flavocytochrome b558. J Biol Chem 1994; 269:30749 - 52; PMID: 7982999
- Diebold BA, Fowler B, Lu J, Dinauer MC, Bokoch GM. Antagonistic cross-talk between Rac and Cdc42 GTPases regulates generation of reactive oxygen species. J Biol Chem 2004; 279:28136 - 42; http://dx.doi.org/10.1074/jbc.M313891200; PMID: 15123662
- Kao Y-Y, Gianni D, Bohl B, Taylor RM, Bokoch GM. Identification of a conserved Rac-binding site on NADPH oxidases supports a direct GTPase regulatory mechanism. J Biol Chem 2008; 283:12736 - 46; http://dx.doi.org/10.1074/jbc.M801010200; PMID: 18347018
- Miyano K, Ogasawara S, Han C-H, Fukuda H, Tamura M. A fusion protein between rac and p67phox (1-210) reconstitutes NADPH oxidase with higher activity and stability than the individual components. Biochemistry 2001; 40:14089 - 97; http://dx.doi.org/10.1021/bi010882u; PMID: 11705402
- Alloul N, Gorzalczany Y, Itan M, Sigal N, Pick E. Activation of the superoxide-generating NADPH oxidase by chimeric proteins consisting of segments of the cytosolic component p67(phox) and the small GTPase Rac1. Biochemistry 2001; 40:14557 - 66; http://dx.doi.org/10.1021/bi0117347; PMID: 11724569
- Gorzalczany Y, Alloul N, Sigal N, Weinbaum C, Pick E. A prenylated p67phox-Rac1 chimera elicits NADPH-dependent superoxide production by phagocyte membranes in the absence of an activator and of p47phox: conversion of a pagan NADPH oxidase to monotheism. J Biol Chem 2002; 277:18605 - 10; http://dx.doi.org/10.1074/jbc.M202114200; PMID: 11896062
- Berdichevsky Y, Mizrahi A, Ugolev Y, Molshanski-Mor S, Pick E. Tripartite chimeras comprising functional domains derived from the cytosolic NADPH oxidase components p47phox, p67phox, and Rac1 elicit activator-independent superoxide production by phagocyte membranes: an essential role for anionic membrane phospholipids. J Biol Chem 2007; 282:22122 - 39; http://dx.doi.org/10.1074/jbc.M701497200; PMID: 17548354
- Mizrahi A, Berdichevsky Y, Casey PJ, Pick E. A prenylated p47phox-p67phox-Rac1 chimera is a Quintessential NADPH oxidase activator: membrane association and functional capacity. J Biol Chem 2010; 285:25485 - 99; http://dx.doi.org/10.1074/jbc.M110.113779; PMID: 20529851
- Mizrahi A, Berdichevsky Y, Ugolev Y, Molshanski-Mor S, Nakash Y, Dahan I, Alloul N, Gorzalczany Y, Sarfstein R, Hirshberg M, et al. Assembly of the phagocyte NADPH oxidase complex: chimeric constructs derived from the cytosolic components as tools for exploring structure-function relationships. J Leukoc Biol 2006; 79:881 - 95; http://dx.doi.org/10.1189/jlb.1005553; PMID: 16641134
- Han C-H, Freeman JLR, Lee T, Motalebi SA, Lambeth JD. Regulation of the neutrophil respiratory burst oxidase. Identification of an activation domain in p67(phox).. J Biol Chem 1998; 273:16663 - 8; http://dx.doi.org/10.1074/jbc.273.27.16663; PMID: 9642219
- Grizot S, Fieschi F, Dagher M-C, Pebay-Peyroula E. The active N-terminal region of p67phox. Structure at 1.8 A resolution and biochemical characterizations of the A128V mutant implicated in chronic granulomatous disease. J Biol Chem 2001; 276:21627 - 31; http://dx.doi.org/10.1074/jbc.M100893200; PMID: 11262407
- Freeman JL, Abo A, Lambeth JD. Rac “insert region” is a novel effector region that is implicated in the activation of NADPH oxidase, but not PAK65. J Biol Chem 1996; 271:19794 - 801; http://dx.doi.org/10.1074/jbc.271.33.19794; PMID: 8702687
- Thapar R, Karnoub AE, Campbell SL. Structural and biophysical insights into the role of the insert region in Rac1 function. Biochemistry 2002; 41:3875 - 83; http://dx.doi.org/10.1021/bi0120087; PMID: 11900529
- Nisimoto Y, Freeman JLR, Motalebi SA, Hirshberg M, Lambeth JD. Rac binding to p67(phox). Structural basis for interactions of the Rac1 effector region and insert region with components of the respiratory burst oxidase. J Biol Chem 1997; 272:18834 - 41; http://dx.doi.org/10.1074/jbc.272.30.18834; PMID: 9228059
- Miyano K, Koga H, Minakami R, Sumimoto H. The insert region of the Rac GTPases is dispensable for activation of superoxide-producing NADPH oxidases. Biochem J 2009; 422:373 - 82; http://dx.doi.org/10.1042/BJ20082182; PMID: 19534724
- Abo A, Webb MR, Grogan A, Segal AW. Activation of NADPH oxidase involves the dissociation of p21rac from its inhibitory GDP/GTP exchange protein (rhoGDI) followed by its translocation to the plasma membrane. Biochem J 1994; 298:585 - 91; PMID: 8141770
- Bromberg Y, Shani E, Joseph G, Gorzalczany Y, Sperling O, Pick E. The GDP-bound form of the small G protein Rac1 p21 is a potent activator of the superoxide-forming NADPH oxidase of macrophages. J Biol Chem 1994; 269:7055 - 8; PMID: 8125910
- Di-Poï N, Fauré J, Grizot S, Molnár G, Pick E, Dagher M-C. Mechanism of NADPH oxidase activation by the Rac/Rho-GDI complex. Biochemistry 2001; 40:10014 - 22; http://dx.doi.org/10.1021/bi010289c; PMID: 11513579
- Sawai T, Asada M, Nunoi H, Matsuda I, Ando S, Sasaki T, Kaibuchi K, Takai Y, Katayama K. Combination of arachidonic acid and guanosine 5′-O-(3-thiotriphosphate) induce translocation of rac p21s to membrane and activation of NADPH oxidase in a cell-free system. Biochem Biophys Res Commun 1993; 195:264 - 9; http://dx.doi.org/10.1006/bbrc.1993.2039; PMID: 8395827
- Quinn MT, Evans T, Loetterle LR, Jesaitis AJ, Bokoch GM. Translocation of Rac correlates with NADPH oxidase activation. Evidence for equimolar translocation of oxidase components. J Biol Chem 1993; 268:20983 - 7; PMID: 8407934
- Philips MR, Pillinger MH, Staud R, Volker C, Rosenfeld MG, Weissmann G, Stock JB. Carboxyl methylation of Ras-related proteins during signal transduction in neutrophils. Science 1993; 259:977 - 80; http://dx.doi.org/10.1126/science.8438158; PMID: 8438158
- Le Cabec V, Möhn H, Gacon G, Maridonneau-Parini I. The small GTP-binding protein rac is not recruited to the plasma membrane upon NADPH oxidase activation in human neutrophils. Biochem Biophys Res Commun 1994; 198:1216 - 24; http://dx.doi.org/10.1006/bbrc.1994.1172; PMID: 8117279
- Philips MR, Feoktistov A, Pillinger MH, Abramson SB. Translocation of p21rac2 from cytosol to plasma membrane is neither necessary nor sufficient for neutrophil NADPH oxidase activity. J Biol Chem 1995; 270:11514 - 21; http://dx.doi.org/10.1074/jbc.270.19.11514; PMID: 7744791
- van Bruggen R, Anthony E, Fernandez-Borja M, Roos D. Continuous translocation of Rac2 and the NADPH oxidase component p67(phox) during phagocytosis. J Biol Chem 2004; 279:9097 - 102; http://dx.doi.org/10.1074/jbc.M309284200; PMID: 14623873
- Yeung T, Grinstein S. Lipid signaling and the modulation of surface charge during phagocytosis. Immunol Rev 2007; 219:17 - 36; http://dx.doi.org/10.1111/j.1600-065X.2007.00546.x; PMID: 17850479
- Pick E. Editorial: When charge is in charge--“Millikan” for leukocyte biologists. J Leukoc Biol 2010; 87:537 - 40; http://dx.doi.org/10.1189/jlb.1109710; PMID: 20356903
- Kreck ML, Uhlinger DJ, Tyagi SR, Inge KL, Lambeth JD. Participation of the small molecular weight GTP-binding protein Rac1 in cell-free activation and assembly of the respiratory burst oxidase. Inhibition by a carboxyl-terminal Rac peptide. J Biol Chem 1994; 269:4161 - 8; PMID: 8307977
- Joseph G, Gorzalczany Y, Koshkin V, Pick E. Inhibition of NADPH oxidase activation by synthetic peptides mapping within the carboxyl-terminal domain of small GTP-binding proteins. Lack of amino acid sequence specificity and importance of polybasic motif. J Biol Chem 1994; 269:29024 - 31; PMID: 7961867
- Sigal N, Gorzalczany Y, Pick E. Two pathways of activation of the superoxide-generating NADPH oxidase of phagocytes in vitro--distinctive effects of inhibitors. Inflammation 2003; 27:147 - 59; http://dx.doi.org/10.1023/A:1023869828688; PMID: 12875368
- Missy K, Van Poucke V, Raynal P, Viala C, Mauco G, Plantavid M, Chap H, Payrastre B. Lipid products of phosphoinositide 3-kinase interact with Rac1 GTPase and stimulate GDP dissociation. J Biol Chem 1998; 273:30279 - 86; http://dx.doi.org/10.1074/jbc.273.46.30279; PMID: 9804788
- Finkielstein CV, Overduin M, Capelluto DGS. Cell migration and signaling specificity is determined by the phosphatidylserine recognition motif of Rac1. J Biol Chem 2006; 281:27317 - 26; http://dx.doi.org/10.1074/jbc.M605560200; PMID: 16861229
- Ueyama T, Eto M, Kami K, Tatsuno T, Kobayashi T, Shirai Y, Lennartz MR, Takeya R, Sumimoto H, Saito N. Isoform-specific membrane targeting mechanism of Rac during Fc gamma R-mediated phagocytosis: positive charge-dependent and independent targeting mechanism of Rac to the phagosome. J Immunol 2005; 175:2381 - 90; PMID: 16081809
- Magalhaes MAO, Glogauer M. Pivotal Advance: Phospholipids determine net membrane surface charge resulting in differential localization of active Rac1 and Rac2. J Leukoc Biol 2010; 87:545 - 55; http://dx.doi.org/10.1189/jlb.0609390; PMID: 19955208
- Zhang FL, Casey PJ. Protein prenylation: molecular mechanisms and functional consequences. Annu Rev Biochem 1996; 65:241 - 69; http://dx.doi.org/10.1146/annurev.bi.65.070196.001325; PMID: 8811180
- Bokoch GM, Prossnitz V. Isoprenoid metabolism is required for stimulation of the respiratory burst oxidase of HL-60 cells. J Clin Invest 1992; 89:402 - 8; http://dx.doi.org/10.1172/JCI115599; PMID: 1310693
- Didsbury JR, Uhing RJ, Snyderman R. Isoprenylation of the low molecular mass GTP-binding proteins rac 1 and rac 2: possible role in membrane localization. Biochem Biophys Res Commun 1990; 171:804 - 12; http://dx.doi.org/10.1016/0006-291X(90)91217-G; PMID: 2119580
- Heyworth PG, Knaus UG, Xu X, Uhlinger DJ, Conroy L, Bokoch GM, Curnutte JT. Requirement for posttranslational processing of Rac GTP-binding proteins for activation of human neutrophil NADPH oxidase. Mol Biol Cell 1993; 4:261 - 9; http://dx.doi.org/10.1091/mbc.4.3.261; PMID: 8387355
- Fuchs A, Dagher M-C, Jouan A, Vignais PV. Activation of the O2(-)-generating NADPH oxidase in a semi-recombinant cell-free system. Assessment of the function of Rac in the activation process. Eur J Biochem 1994; 226:587 - 95; http://dx.doi.org/10.1111/j.1432-1033.1994.tb20084.x; PMID: 8001573
- Ugolev Y, Molshanski-Mor S, Weinbaum C, Pick E. Liposomes comprising anionic but not neutral phospholipids cause dissociation of Rac(1 or 2) x RhoGDI complexes and support amphiphile-independent NADPH oxidase activation by such complexes. J Biol Chem 2006; 281:19204 - 19; http://dx.doi.org/10.1074/jbc.M600042200; PMID: 16702219
- Khan OM, Ibrahim MX, Jonsson I-M, Karlsson C, Liu M, Sjogren A-KM, Olofsson FJ, Brisslert M, Andersson S, Ohlsson C, et al. Geranylgeranyltransferase type I (GGTase-I) deficiency hyperactivates macrophages and induces erosive arthritis in mice. J Clin Invest 2011; 121:628 - 39; http://dx.doi.org/10.1172/JCI43758; PMID: 21266780
- DerMardirossian C, Bokoch GM. GDIs: central regulatory molecules in Rho GTPase activation. Trends Cell Biol 2005; 15:356 - 63; http://dx.doi.org/10.1016/j.tcb.2005.05.001; PMID: 15921909
- Dovas A, Couchman JR. RhoGDI: multiple functions in the regulation of Rho family GTPase activities. Biochem J 2005; 390:1 - 9; http://dx.doi.org/10.1042/BJ20050104; PMID: 16083425
- Garcia-Mata R, Boulter E, Burridge K. The ‘invisible hand’: regulation of RHO GTPases by RHOGDIs. Nat Rev Mol Cell Biol 2011; 12:493 - 504; http://dx.doi.org/10.1038/nrm3153; PMID: 21779026
- Longenecker K, Read P, Derewenda U, Dauter Z, Liu X, Garrard S, Walker L, Somlyo AV, Nakamoto RK, Somlyo AP, et al. How RhoGDI binds Rho. Acta Crystallogr D Biol Crystallogr 1999; 55:1503 - 15; http://dx.doi.org/10.1107/S090744499900801X; PMID: 10489445
- Hoffman GR, Nassar N, Cerione RA. Structure of the Rho family GTP-binding protein Cdc42 in complex with the multifunctional regulator RhoGDI. Cell 2000; 100:345 - 56; http://dx.doi.org/10.1016/S0092-8674(00)80670-4; PMID: 10676816
- Grizot S, Fauré J, Fieschi F, Vignais PV, Dagher M-C, Pebay-Peyroula E. Crystal structure of the Rac1-RhoGDI complex involved in nadph oxidase activation. Biochemistry 2001; 40:10007 - 13; http://dx.doi.org/10.1021/bi010288k; PMID: 11513578
- Chuang TH, Xu X, Knaus UG, Hart MJ, Bokoch GM. GDP dissociation inhibitor prevents intrinsic and GTPase activating protein-stimulated GTP hydrolysis by the Rac GTP-binding protein. J Biol Chem 1993; 268:775 - 8; PMID: 8419353
- Chuang T-H, Bohl BP, Bokoch GM. Biologically active lipids are regulators of Rac.GDI complexation. J Biol Chem 1993; 268:26206 - 11; PMID: 8253741
- DerMardirossian C, Schnelzer A, Bokoch GM. Phosphorylation of RhoGDI by Pak1 mediates dissociation of Rac GTPase. Mol Cell 2004; 15:117 - 27; http://dx.doi.org/10.1016/j.molcel.2004.05.019; PMID: 15225553
- Ugolev Y, Berdichevsky Y, Weinbaum C, Pick E. Dissociation of Rac1(GDP).RhoGDI complexes by the cooperative action of anionic liposomes containing phosphatidylinositol 3,4,5-trisphosphate, Rac guanine nucleotide exchange factor, and GTP. J Biol Chem 2008; 283:22257 - 71; http://dx.doi.org/10.1074/jbc.M800734200; PMID: 18505730
- Ueyama T, Son J, Kobayashi T, Hamada T, Nakamura T, Sakaguchi H, Shirafuji T, Saito N. Negative charges in the flexible N-terminal domain of Rho GDP-dissociation inhibitors (RhoGDIs) regulate the targeting of the RhoGDI-Rac1 complex to membranes. J Immunol 2013; 191:2560 - 9; http://dx.doi.org/10.4049/jimmunol.1300209; PMID: 23918979
- Zheng Y. Dbl family guanine nucleotide exchange factors. Trends Biochem Sci 2001; 26:724 - 32; http://dx.doi.org/10.1016/S0968-0004(01)01973-9; PMID: 11738596
- Schmidt A, Hall A. Guanine nucleotide exchange factors for Rho GTPases: turning on the switch. Genes Dev 2002; 16:1587 - 609; http://dx.doi.org/10.1101/gad.1003302; PMID: 12101119
- Gao Y, Xing J, Streuli M, Leto TL, Zheng Y. Trp(56) of rac1 specifies interaction with a subset of guanine nucleotide exchange factors. J Biol Chem 2001; 276:47530 - 41; http://dx.doi.org/10.1074/jbc.M108865200; PMID: 11595749
- Sigal N, Gorzalczany Y, Sarfstein R, Weinbaum C, Zheng Y, Pick E. The guanine nucleotide exchange factor trio activates the phagocyte NADPH oxidase in the absence of GDP to GTP exchange on Rac. “The emperor’s nw clothes”. J Biol Chem 2003; 278:4854 - 61; http://dx.doi.org/10.1074/jbc.M211011200; PMID: 12475976
- Mizrahi A, Molshanski-Mor S, Weinbaum C, Zheng Y, Hirshberg M, Pick E. Activation of the phagocyte NADPH oxidase by Rac Guanine nucleotide exchange factors in conjunction with ATP and nucleoside diphosphate kinase. J Biol Chem 2005; 280:3802 - 11; http://dx.doi.org/10.1074/jbc.M410257200; PMID: 15557278
- Robbe K, Otto-Bruc A, Chardin P, Antonny B. Dissociation of GDP dissociation inhibitor and membrane translocation are required for efficient activation of Rac by the Dbl homology-pleckstrin homology region of Tiam. J Biol Chem 2003; 278:4756 - 62; http://dx.doi.org/10.1074/jbc.M210412200; PMID: 12471028
- Hill K, Krugmann S, Andrews SR, Coadwell WJ, Finan P, Welch HCE, Hawkins PT, Stephens LR. Regulation of P-Rex1 by phosphatidylinositol (3,4,5)-trisphosphate and Gbetagamma subunits. J Biol Chem 2005; 280:4166 - 73; http://dx.doi.org/10.1074/jbc.M411262200; PMID: 15545267
- Welch HCE, Coadwell WJ, Ellson CD, Ferguson GJ, Andrews SR, Erdjument-Bromage H, Tempst P, Hawkins PT, Stephens LR. P-Rex1, a PtdIns(3,4,5)P3- and Gbetagamma-regulated guanine-nucleotide exchange factor for Rac. Cell 2002; 108:809 - 21; http://dx.doi.org/10.1016/S0092-8674(02)00663-3; PMID: 11955434
- Dong X, Mo Z, Bokoch G, Guo C, Li Z, Wu D. P-Rex1 is a primary Rac2 guanine nucleotide exchange factor in mouse neutrophils. Curr Biol 2005; 15:1874 - 9; http://dx.doi.org/10.1016/j.cub.2005.09.014; PMID: 16243036
- Lawson CD, Donald S, Anderson KE, Patton DT, Welch HCE. P-Rex1 and Vav1 cooperate in the regulation of formyl-methionyl-leucyl-phenylalanine-dependent neutrophil responses. J Immunol 2011; 186:1467 - 76; http://dx.doi.org/10.4049/jimmunol.1002738; PMID: 21178006
- Welch HCE, Coadwell WJ, Stephens LR, Hawkins PT. Phosphoinositide 3-kinase-dependent activation of Rac. FEBS Lett 2003; 546:93 - 7; http://dx.doi.org/10.1016/S0014-5793(03)00454-X; PMID: 12829242
- Price MO, Atkinson SJ, Knaus UG, Dinauer MC. Rac activation induces NADPH oxidase activity in transgenic COSphox cells, and the level of superoxide production is exchange factor-dependent. J Biol Chem 2002; 277:19220 - 8; http://dx.doi.org/10.1074/jbc.M200061200; PMID: 11896053
- Kim C, Marchal CC, Penninger J, Dinauer MC. The hemopoietic Rho/Rac guanine nucleotide exchange factor Vav1 regulates N-formyl-methionyl-leucyl-phenylalanine-activated neutrophil functions. J Immunol 2003; 171:4425 - 30; PMID: 14530369
- Ligeti E, Welti S, Scheffzek K. Inhibition and termination of physiological responses by GTPase activating proteins. Physiol Rev 2012; 92:237 - 72; http://dx.doi.org/10.1152/physrev.00045.2010; PMID: 22298657
- Moon SY, Zheng Y. Rho GTPase-activating proteins in cell regulation. Trends Cell Biol 2003; 13:13 - 22; http://dx.doi.org/10.1016/S0962-8924(02)00004-1; PMID: 12480336
- Voncken JW, van Schaick H, Kaartinen V, Deemer K, Coates T, Landing B, Pattengale P, Dorseuil O, Bokoch GM, Groffen J, et al. Increased neutrophil respiratory burst in bcr-null mutants. Cell 1995; 80:719 - 28; http://dx.doi.org/10.1016/0092-8674(95)90350-X; PMID: 7889565
- Heyworth PG, Knaus UG, Settleman J, Curnutte JT, Bokoch GM. Regulation of NADPH oxidase activity by Rac GTPase activating protein(s). Mol Biol Cell 1993; 4:1217 - 23; http://dx.doi.org/10.1091/mbc.4.11.1217; PMID: 8305740
- Geiszt M, Dagher M-C, Molnár G, Havasi A, Faure J, Paclet M-H, Morel F, Ligeti E. Characterization of membrane-localized and cytosolic Rac-GTPase-activating proteins in human neutrophil granulocytes: contribution to the regulation of NADPH oxidase. Biochem J 2001; 355:851 - 8; PMID: 11311150
- Moskwa P, Dagher M-C, Paclet M-H, Morel F, Ligeti E. Participation of Rac GTPase activating proteins in the deactivation of the phagocytic NADPH oxidase. Biochemistry 2002; 41:10710 - 6; http://dx.doi.org/10.1021/bi0257033; PMID: 12186557
- Litvak Y, Selinger Z. Bacterial mimics of eukaryotic GTPase-activating proteins (GAPs). Trends Biochem Sci 2003; 28:628 - 31; http://dx.doi.org/10.1016/j.tibs.2003.10.001; PMID: 14659693
- Seifert R, Rosenthal W, Schultz G, Wieland T, Gierschick P, Jakobs KH. The role of nucleoside-diphosphate kinase reactions in G protein activation of NADPH oxidase by guanine and adenine nucleotides. Eur J Biochem 1988; 175:51 - 5; http://dx.doi.org/10.1111/j.1432-1033.1988.tb14165.x; PMID: 2841126
- Uhlinger DJ, Burnham DN, Lambeth JD. Nucleoside triphosphate requirements for superoxide generation and phosphorylation in a cell-free system from human neutrophils. Sodium dodecyl sulfate and diacylglycerol activate independently of protein kinase C. J Biol Chem 1991; 266:20990 - 7; PMID: 1657941
- Peveri P, Heyworth PG, Curnutte JT. Absolute requirement for GTP in activation of human neutrophil NADPH oxidase in a cell-free system: role of ATP in regenerating GTP. Proc Natl Acad Sci U S A 1992; 89:2494 - 8; http://dx.doi.org/10.1073/pnas.89.6.2494; PMID: 1312725
- Ambruso DR, Knall C, Abell AN, Panepinto J, Kurkchubasche A, Thurman G, Gonzalez-Aller C, Hiester A, deBoer M, Harbeck RJ, et al. Human neutrophil immunodeficiency syndrome is associated with an inhibitory Rac2 mutation. Proc Natl Acad Sci U S A 2000; 97:4654 - 9; http://dx.doi.org/10.1073/pnas.080074897; PMID: 10758162
- Williams DA, Tao W, Yang F, Kim C, Gu Y, Mansfield P, Levine JE, Petryniak B, Derrow CW, Harris C, et al. Dominant negative mutation of the hematopoietic-specific Rho GTPase, Rac2, is associated with a human phagocyte immunodeficiency. Blood 2000; 96:1646 - 54; PMID: 10961859
- Gu Y, Jia B, Yang F-C, D’Souza M, Harris CE, Derrow CW, Zheng Y, Williams DA. Biochemical and biological characterization of a human Rac2 GTPase mutant associated with phagocytic immunodeficiency. J Biol Chem 2001; 276:15929 - 38; http://dx.doi.org/10.1074/jbc.M010445200; PMID: 11278678
- Roberts AW, Kim C, Zhen L, Lowe JB, Kapur R, Petryniak B, Spaetti A, Pollock JD, Borneo JB, Bradford GB, et al. Deficiency of the hematopoietic cell-specific Rho family GTPase Rac2 is characterized by abnormalities in neutrophil function and host defense. Immunity 1999; 10:183 - 96; http://dx.doi.org/10.1016/S1074-7613(00)80019-9; PMID: 10072071
- Kim C, Dinauer MC. Rac2 is an essential regulator of neutrophil nicotinamide adenine dinucleotide phosphate oxidase activation in response to specific signaling pathways. J Immunol 2001; 166:1223 - 32; PMID: 11145705
- Glogauer M, Marchal CC, Zhu F, Worku A, Clausen BE, Foerster I, Marks P, Downey GP, Dinauer M, Kwiatkowski DJ. Rac1 deletion in mouse neutrophils has selective effects on neutrophil functions. J Immunol 2003; 170:5652 - 7; PMID: 12759446
- Marchioni F, Zheng Y. Targeting rho GTPases by peptidic structures. Curr Pharm Des 2009; 15:2481 - 7; http://dx.doi.org/10.2174/138161209788682334; PMID: 19601845
- Dahan I, Pick E. Strategies for identifying synthetic peptides to act as inhibitors of NADPH oxidases, or “all that you did and did not want to know about Nox inhibitory peptides”. Cell Mol Life Sci 2012; 69:2283 - 305; http://dx.doi.org/10.1007/s00018-012-1007-4; PMID: 22562603
- Kim J-A, Neupane GP, Lee ES, Jeong B-S, Park BC, Thapa P. NADPH oxidase inhibitors: A patent review. Expert Opin Ther Patents 2011; 21:1147-58.
- Rabiet M-J, Tardif M, Braun L, Boulay F. Inhibitory effects of a dominant-interfering form of the Rho-GTPase Cdc42 in the chemoattractant-elicited signaling pathways leading to NADPH oxidase activation in differentiated HL-60 cells. Blood 2002; 100:1835 - 44; http://dx.doi.org/10.1182/blood-2001-12-0193; PMID: 12176907
- Dorseuil O, Vazquez A, Lang P, Bertoglio J, Gacon G, Leca G. Inhibition of superoxide production in B lymphocytes by rac antisense oligonucleotides. J Biol Chem 1992; 267:20540 - 2; PMID: 1328203