Abstract
The mammalian blood-testis barrier (BTB) restructures throughout spermatogenesis, thereby allowing developing germ cells to enter the adluminal compartment of the seminiferous epithelium. Previous studies have shown pro-inflammatory cytokines such as tumor necrosis factor α (TNFα) and interleukin-1α to be important regulators of Sertoli cell barrier/BTB function in vitro and in vivo. In this study, the effects of TNFα on Sertoli cell barrier function were assessed, with emphasis on changes in proteases and cell adhesion molecules following treatment. By immunoblotting and immunohistochemistry, MMP9 was found to be present in germ cells, localizing by and large to spermatocytes and spermatids in the adult rat testis. Following treatment of Sertoli cells with physiologically relevant consecutive doses of recombinant human TNFα (25 ng/ml), the steady-state levels of active-matrix metalloprotease 9 (MMP9), membrane-bound intercellular adhesion molecule (mICAM-1) and androgen receptor increased significantly. TNFα also downregulated the steady-state level of occludin, in agreement with earlier results that showed TNFα to disrupt Sertoli cell barrier/BTB function. In addition, TNFα affected the filamentous actin cytoskeleton in Sertoli cells, which appeared to be mediated by cortactin, a regulator of actin dynamics. Taken collectively, these findings imply that germ cells may be involved in BTB restructuring via the localized production of TNFα. These results also illustrate that barrier restructuring correlated with an increase in Sertoli cell mICAM-1, suggesting that it may be critical for adhesion as germ cells traverse the “opened” BTB.
Keywords: :
Introduction
Spermatogenesis is an extremely complex cellular process that produces approximately 150 million sperm daily in an average healthy male, and it takes place within the seminiferous epithelium under the regulation of several factors, including testosterone, follicle stimulating hormone (FSH), luteinizing hormone (LH) and estradiol 17β.Citation1-Citation3 To appreciate the process of spermatogenesis, the biology of possibly the most important ultrastructure within the seminiferous epithelium—the blood-testis barrier (BTB, also known as the Sertoli cell barrier)—has to be first understood.Citation1,Citation4-Citation7 For instance, the BTB is known to be constituted by different types of co-existing junctions (i.e., tight junctions, basal ectoplasmic specializations, desmosomes and gap junctions) that localize basally between adjacent Sertoli cells, and it creates a highly specialized microenvironment that separates developing germ cells into two distinct yet inter-related compartments: (1) the basal compartment where spermatogonia and primary spermatocytes exist and (2) the adluminal compartment where secondary spermatocytes, haploid spermatids and spermatozoa reside. As germ cells transition through different developmental stages, their movement across the seminiferous epithelium is coordinated with restructuring of the BTB, which allows zygotene spermatocytes to gain entry into the adluminal compartment.Citation8-Citation10 Thus, unique mechanisms are believed to be in place to facilitate the timely movement of preleptotene and leptotene spermatocytes across the BTB without compromising the homeostasis of the seminiferous epithelium and without affecting spermatogenesis. Indeed, morphological studies have shown there to be a brief moment during the seminiferous epithelial cycle when a preleptotene or leptotene spermatocyte can be observed as being trapped between Sertoli cell barriers, thereby creating an intermediate compartment.Citation9,Citation11,Citation12 The only imaginable way that spermatocytes can transit upwards without affecting the integrity of the BTB is if disassembly of old junctions is synchronized with assembly of new junctions that are present above and below migrating spermatocytes, respectively. In this way, the integrity of the seminiferous epithelium is maintained.
To get a better idea of which additional molecules and mechanisms might be involved in BTB restructuring, we looked carefully at studies from other in vitro and in vivo systems. From these studies, we identified membrane-bound ICAM-1 (mICAM-1) as an important protein involved in junction dynamics and cell movement. mICAM-1 is best studied with respect to the movement of leukocytes across the endothelium during inflammation,Citation13 and its expression in Sertoli and germ cells has been previously reported.Citation14 Soluble forms of ICAM-1 (sICAM-1) generated from the proteolytic cleavage of mICAM-1 have also been shown to have important biological functions in Sertoli cells as well as in other epithelia.Citation15-Citation17 In a recently published study, we showed mICAM-1 and sICAM-1 to have critical but opposing roles in BTB function,Citation14 which led us to conclude that mICAM-1 participates in the assembly of the new BTB below migrating spermatocytes and conversely that sICAM-1 participates in the disassembly of the old BTB above migrating spermatocytes. Earlier studies by flow cytometry using mouse Sertoli cells showed ICAM-1 to be upregulated by tumor necrosis factor α (TNFα),Citation18-Citation20 a pro-inflammatory cytokine produced by spermatocytes and spermatids.Citation21 Interestingly, TNFα negatively affected Sertoli cell barrier/BTB function by upregulating proteases [e.g., matrix metalloprotease 9 (MMP9)], downregulating transmembrane protein levels [e.g., occludin, junctional adhesion molecule-A (JAM-A), and coxsackie and adenovirus receptor (CAR)] and triggering protein internalization (e.g., occludin and JAM-A).Citation22-Citation25 In this in vitro study, we continue our investigation on the role of mICAM-1 and sICAM-1 in Sertoli cell barrier dynamics by determining if their steady-state levels are indeed affected by TNFα since early studies relied largely on cytometric analysis. Our results support the role of mICAM-1 in BTB dynamics as well as contribute to a new mechanism of BTB regulation by TNFα. Our results illustrate that BTB restructuring involves many proteins. They also suggest that germ cells may trigger BTB restructuring through localized secretion of TNFα, thereby upregulating mICAM-1, which may be critical for adhesion as germ cells traverse the barrier.
Materials and Methods
Animals
Male Sprague-Dawley rats at 90 (~300 g b.w.) and 20 d of age were purchased from Charles River Laboratories. The Institutional Animal Care and Use Committee of The Rockefeller University approved the use of rats (protocol numbers 09016 and 12506). All experiments involving rats were performed by following ethical guidelines outlined in the Guide for the Care and Use of Laboratory Animals.
Sertoli cell cultures and treatment of cells with TNFα
Sertoli cells were isolated from 20-d-old rat testes and cultured in serum-free DMEM/F12 (Sigma-Aldrich) supplemented with growth factors and an antibiotic.Citation26-Citation28 Cells were plated onto Matrigel™- (BD Biosciences) coated 12-well plates at 0.5 × 106 cells/cm2 for lysate preparation or onto Matrigel™-coated micro cover glasses (18 mm, Thomas Scientific) at 0.04 × 106 cells/cm2 for immunofluorescent staining. Cultures were incubated in a humidified atmosphere of 95% air and 5% CO2 (vol/vol) at 35°C. At 48 h, cultures were treated with a hypotonic buffer (20 mM Tris pH 7.4 at 22°C) to lyse contaminating germ cells, yielding Sertoli cells with a relative purity of ~98%.Citation29 Recombinant human TNFα (R&D Systems, Inc.) at 25 ng/ml (~1.4 nM) was added daily into Sertoli cell cultures from day 4 onwards. Thereafter, Sertoli cells were terminated at specific time points for lysate preparation. TNFα was prepared at a stock concentration of 100 μg/ml in PBS (10 mM NaH2PO4 pH 7.4 at 22°C containing 0.15 M NaCl) containing 0.1% BSA (wt/vol). The control consisted of culturing Sertoli cells in DMEM/F12 containing an equivalent amount of BSA (wt/vol).
Immunohistochemistry and immunofluorescent staining
Immunohistochemistry was performed as previously described.Citation30 Testes were fixed in Bouin’s fixative (Polysciences, Inc.) for 24 h, dehydrated using increasing concentrations of ethanol, cleared in xylene, embedded in paraffin and sectioned at 5 μm. For antigen retrieval, sections were submerged in 10 mM citrate buffer pH 6.0 at 22°C and heated in a microwave (750 W) twice for 5 min. Endogenous peroxidase activity was inhibited with 3% H2O2 (vol/vol) in methanol for 10 min, and non-specific binding sites were blocked with 10% non-immune goat serum (vol/vol) for 30 min at R.T. Thereafter, sections were incubated with primary antibody at 4°C overnight in a humidified chamber (). On the next day, biotinylated goat anti-rabbit IgG (1:400; Vector Laboratories) was applied for 60 min, followed by an avidin-biotinylated horseradish peroxidase complex (Vectastain® ABC Reagent, Vector Laboratories). Sections were carefully washed with Tris-buffered saline (TBS; 0.05 M Tris-HCl pH 7.6 at 22°C containing 0.15 M NaCl) after each step. The primary antibody was also diluted in TBS. Bound antibody was visualized by using 0.05% 3,3′-diaminobenzidine tetrahydrochloride (DAB, wt/vol), 0.01% H2O2 (vol/vol) and 0.07% imidazole (wt/vol) in TBS. Sections were counterstained lightly with Mayer’s hematoxylin. Finally, sections were dehydrated, cleared in xylene and mounted with Poly-Mount medium (Polysciences, Inc.). The control consisted of incubating sections with 10% normal goat serum (vol/vol) instead of the primary antibody.
Table 1. Antibodies used in this study
For immunofluorescent (IF) staining of testes, frozen tissues were sectioned at 7 μm, adhered to poly-L-lysine-coated microscope slides (Polysciences, Inc.) and fixed in Bouin’s fixative or ice-cold methanol. Thereafter, sections were pretreated with 0.1% Triton X-100 (vol/vol) in TBS, and non-specific binding sites were blocked with 10% non-immune goat serum (vol/vol) or 1% bovine serum albumin (wt/vol, BSA). Following an overnight incubation with primary antibody at R.T. (), sections were saturated with Alexa Fluor® secondary antibodies (1:200; Invitrogen). Sections were subsequently mounted with ProLong® antifade reagent containing 4’,6-diamidino-2-phenylindole (DAPI, Invitrogen). For IF staining of Sertoli cells, cells were cultured at 0.04 × 106 cells/cm2 on Matrigel™-coated micro cover glasses and treated with TNFα (25 ng/ml) for 24 h. They were then fixed with 4% paraformaldehyde (wt/vol) in PBS for 10 min, permeabilized with 0.1% Triton X-100 (vol/vol) and blocked with 10% non-immune goat serum (vol/vol) or 1% BSA (wt/vol). Primary antibodies from two species were used for an overnight incubation at R.T. [; 1:50–1:100 in 1% BSA (wt/vol)/PBS], followed by a 30 min incubation with Alexa Fluor® secondary antibodies [1:100 in 1% BSA (wt/vol)/PBS]. For F-actin staining, cells were fixed with 4% paraformaldehyde (wt/vol) in PBS at R.T. for 10 min, followed by incubation with Oregon Green® 514 phalloidin (Invitrogen). Images were captured by using an Olympus BX61 fluorescent microscope and MicroSuite™ FIVE software (v. 2.7; Olympus America). Images were compiled in Adobe Photoshop CS5 Extended software (v. 10.0.1; Adobe Systems Inc.). Images were adjusted for brightness and/or contrast, if needed, and identical adjustments were applied to all images within a single experiment.
General methods
Adult testes, germ and Sertoli cells were used for the preparation of lysates in lysis buffer. Germ cells were isolated as previously described.Citation31 Filtration of germ cells through glass wool was omitted. Seminiferous tubules were isolated as previously described.Citation14,Citation32,Citation33 Protein concentration was determined by using the DC protein assay and a model 680 microplate reader (BIO-RAD Laboratories). Immunoblotting was performed by using a routine protocol. Chemiluminescent images were captured and analyzed by using a LAS-4000 mini imaging system and MultiGauge software (v. 3.1; FujiFilm Life Science USA), respectively. lists the antibodies and conditions that were used in this study. The sICAM-1 antibody was produced in-house and characterized as previously described.Citation14 By immunoblotting, this antibody cross-reacted strongly with a ~70 kDa Sertoli and germ cell protein that corresponded to sICAM-1, and weakly with a ~97 kDa Sertoli and germ cell protein that corresponded to mICAM-1.Citation14 However, this antibody was not used for mICAM-1 detection. Instead, a commercially available antibody was used ().
Statistical analyses
Comparisons were performed by one-way ANOVA, followed by Dunnett’s post-hoc test (GB-STAT software, v. 7.0; Dynamic Microsystems). Each experiment was repeated at least three times by using different batches of Sertoli cells. Within a single experiment, each treatment/time point consisted of Sertoli cells cultured in 12-well plates or on micro cover glasses in triplicate. p < 0.05 was taken as statistically significant.
Results
MMP9 and MT1-MMP are present in Sertoli and germ cells, localizing predominantly to spermatocytes and spermatids in the adult rat testis
This study was initiated by investigating the presence of MMP9 in the adult rat testis, Sertoli and germ cells by immunoblotting, followed by immunohistochemistry (IHC) and immunofluorescent (IF) staining experiments where MMP9 was localized to the adult rat testis. By immunoblotting, MMP9 (both pro and active forms) was found to be present in the testis, Sertoli and germ cells (). The lowest and highest levels of active-MMP9 were detected in Sertoli and germ cells, respectively (). To assess germ cell purity, lysates were screened by using a testin antibody. Testin, a Sertoli and Leydig cell protein,Citation34-Citation36 was not detected in germ cell lysates, illustrating negligible contamination. The monospecificity of another MMP9 antibody was also assessed by immunoblotting (), and 92 and 84 kDa proteins corresponding to pro- and active-MMP9, respectively, were observed in seminiferous tubule lysate. This specific antibody was used for subsequent IHC and IF staining experiments as it yielded a cleaner immunoblot than the antibody used in . By IHC and IF staining, MMP9 was found to localize predominantly to spermatocytes, round and elongating spermatids (), consistent with a previously published report.Citation24 Of these, pachytene spermatocytes were most immunoreactive for MMP9. Weak MMP9 immunoreactivity was also noted with Sertoli cells ().
Figure 1. Cellular distribution and localization of MMP9 in the seminiferous epithelium of the adult rat testis during the epithelial cycle of spermatogenesis. (A) Presence of MMP9 in testis (T), Sertoli (SC, isolated from 20-d-old testes and cultured at high density for 4 d) and germ cell (GC, isolated form 90-d-old testes and used immediately for lysate preparation) lysates (50 μg protein/lane) by immunoblotting. Testin, a Sertoli and Leydig cell protein, was used to assess the purity of germ cells. Actin served as an indicator of equal protein loading. (B) Histogram summarizing results shown in (A). The relative levels of pro- and active-MMP9 detected in Sertoli and germ cell lysates were compared with pro- and active-MMP9 in the testis, whose levels were arbitrarily set at 1. Each bar represents the mean ± SD of n = 3 experiments. **p < 0.01 (ANOVA followed by Dunnett’s post-hoc test). (C) Immunoblot showing the specificity of the anti-MMP9 polyclonal antibody () in seminiferous tubule (ST) lysate (50 μg protein). This antibody was used subsequently for IHC and IF staining. Mr, molecular weight; M, MagicMark™ XP Western Protein Standard (Invitrogen). (D) IHC and IF staining of MMP9 in paraffin-embedded (5 μm) and frozen (7 μm) adult testis cross-sections, respectively. MMP9 immunoreactivity appeared as a brownish precipitate in IHC experiments (a–h) and as a reddish precipitate in IF experiments (i–p). Nuclei were visualized with DAPI (blue, i–p). Stages of the seminiferous epithelial cycle are denoted as Roman numerals (b–h and j–p). Insets (b, e and f) are magnified images corresponding to boxed areas within the same panel. Specificity was assessed when 10% normal goat serum (vol/vol) was used instead of anti-MMP9 IgG (a, right panel). Dashed lines (j–p) mark the periphery of seminiferous tubules. Scale bars, 25 μm. MMP9 immunoreactivity associated intensely with round spermatids at stages I-VII (red arrowheads in b and j, and insert in b) and moderately/weakly with elongating spermatids at stages VIII-XII (blue arrowheads in e and m, right-most inset in e, inset in f). MMP9 also associated strongly with pachytene and diplotene spermatocytes at stages VIII–XIII (green arrowheads in d and l, left-most insert in e). Weak staining was also detected in Sertoli cells (asterisks in b and k).
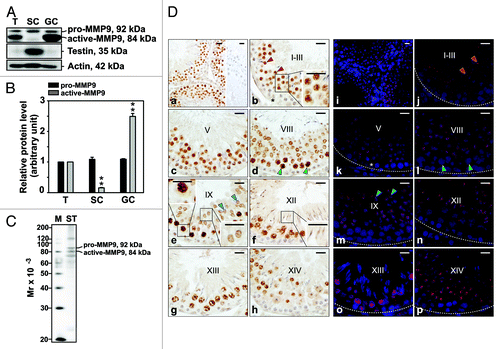
By immunoblotting, MT1-MMP was also found to be present in the testis, Sertoli and germ cells (). Likewise, the lowest level of MT1-MMP was observed in Sertoli cells (). When this antibody’s monospecificity was assessed, a 65 kDa protein corresponding to inactive MT1-MMP was observed in testis lysate (). IHC and IF staining showed that total MT1-MMP surrounded elongating/elongated spermatids, consistent with previously published reports.Citation37,Citation38 In agreement with MMP9 localization, weak MT1-MMP immunoreactivity was also detected in pachytene spermatocytes and round spermatids (). On the other hand, very strong immunoreactivity was observed in residual bodies (), structures containing excess cytoplasm and organelles.Citation39,Citation40 It should be noted that this antibody recognized the hinge region of MT1-MMP, which links the C-terminus with the catalytic domain and may be important for MT1-MMP proteolytic processing.Citation41
Figure 2. Cellular localization and stage-specific expression of MT1-MMP in the seminiferous epithelium of the adult rat testis during the epithelial cycle of spermatogenesis. (A) Presence of MT1-MMP in testis (T), Sertoli (SC, see legend to for details) and germ cell (GC, see legend to for details) lysates (50 μg protein/lane) by immunoblotting. Actin served as an indicator of equal protein loading. (B) Histogram summarizing results shown in (A). The relative level of MT1-MMP detected in Sertoli and germ cell lysates was compared with MT1-MMP in the testis, whose level was arbitrarily set at 1. Each bar represents the mean ± SD of n = 3 experiments. *p < 0.05; **p < 0.01 (ANOVA followed by Dunnett’s post-hoc test). (C) Immunoblot showing the specificity of an anti-MT1-MMP polyclonal antibody () in testis lysate (50 μg protein). This antibody was used subsequently for IHC and IF staining. Mr, molecular weight; M, MagicMark™ XP Western Protein Standard (Invitrogen). (D) IHC and IF staining of MT1-MMP in paraffin-embedded (5 μm) and frozen (7 μm) adult testis cross-sections, respectively. MT1-MMP immunoreactivity appeared as a brownish precipitate in IHC experiments (a–h) and as a reddish precipitate in IF experiments (i–p). Nuclei were visualized with DAPI (blue, i–p). Stages of the seminiferous epithelial cycle are denoted as Roman numerals (b–h, and j–p). Insets (e and i) are magnified images corresponding to boxed areas within the same panel. Specificity was assessed when 10% normal goat serum (vol/vol) was used instead of MT1-MMP IgG (a, right panel). Dashed lines (j–p) mark the periphery of seminiferous tubules. Scale bars, 25 μm. Immunoreactive MT1-MMP immunoreactivity associated intensely with residual bodies (arrows in d) and weakly with pachytene spermatocytes, and round and elongating/elongated spermatids (blue arrowheads in e).
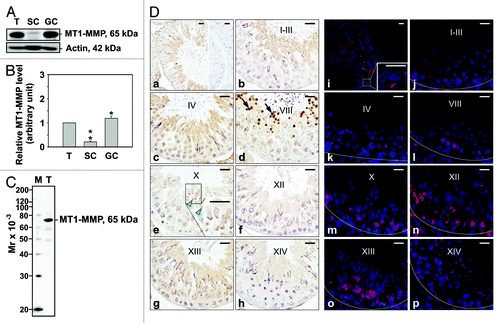
TNFα regulates Sertoli cell barrier function in vitro by upregulating active-MMP9, mICAM-1 and AR, and by downregulating occludin
Previous studies have shown TNFα to affect the levels of several proteins, including MMP2 and MMP9.Citation24,Citation42 To better understand the effects of TNFα on Sertoli cell barrier function, we treated Sertoli cells with physiologically relevant consecutive doses of recombinant human TNFα and investigated the steady-state levels of different integral membrane, scaffolding and regulatory proteins known to be involved directly or indirectly in Sertoli cell barrier/BTB function. TNFα was added to Sertoli cells on day 4 in vitro, shortly after the formation of a functional Sertoli cell barrier. Immunoblotting revealed no significant changes in the steady-state levels of pro-MMP9, sICAM-1, N-cadherin, β-catenin, ARP3, cortactin, p-Cortactin-Y421, c-SRC, FAK and p-FAK-Y407 when compared with time-matched Veh-treated Sertoli cells (). On the other hand, TNFα treatment brought about an increase in the levels of active-MMP9, ICAM-1 and AR, but a decrease in the level of occludin (). These results are in agreement with previously published results from this and other laboratories.Citation18,Citation20,Citation23,Citation24,Citation42,Citation43
Figure 3. Effects of TNFα on the steady-state levels of integral membrane, scaffolding and regulatory proteins in Sertoli cells in vitro. Sertoli cells were cultured at high density on Matrigel™-coated 12-well dishes as described in Materials and Methods. On day 4 (designated as 0 h in this figure), TNFα (25 ng/ml) was added into Sertoli cell cultures, and cells were terminated at specific time points thereafter for lysate preparation. TNFα was dissolved in 10 mM NaH2PO4 pH 7.4 at 22°C containing 0.15 M NaCl and 0.1% BSA (wt/vol). The control consisted of culturing Sertoli cells in media containing an equivalent amount of BSA. (A) Immunoblots of selected proteins involved in the regulation and in the maintenance of Sertoli cell barrier function. Actin served as an indicator of equal protein loading. (B) Histograms summarizing results shown in (A) from at least three independent experiments. Histograms are not shown for proteins whose levels did not change significantly. Each data point was normalized against its corresponding actin data point and then against the protein level at 0 h, which was arbitrarily set as 1. Each bar represents the mean ± SD of n = 3–4 experiments. **p < 0.01 (ANOVA followed by Dunnett's post-hoc test). n.d., not determined.
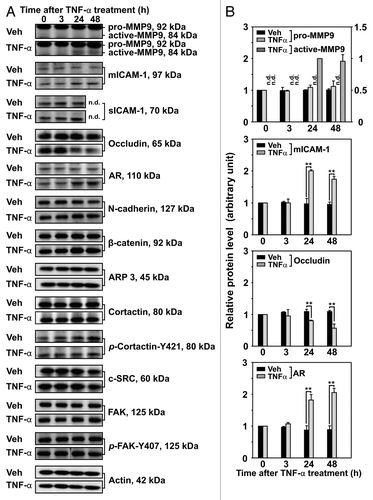
Next, we investigated the cellular localization of MMP9 and MT1-MMP in Sertoli cells following TNFα treatment. MMP9 localized to the cytoplasm in Veh-treated Sertoli cells (); however, an increase in MMP9 was observed, not only throughout the cytoplasm, but also between adjacent Sertoli cells following TNFα treatment (). On the other hand, the immunofluorescent localization of MT1-MMP was different from that of MMP9. In Veh-treated Sertoli cells, MT1-MMP localized to the plasma membrane, in agreement with its role as a type I membrane-anchored protease.Citation44,Citation45 Following TNFα treatment, MT1-MMP increased appreciably, suggesting that there may be an increase in proteolysis. In support of immunoblotting results (), there was a loss in occludin from the Sertoli cell surface compared with Veh-treated cells (). However, no changes were observed in ZO-1 at the Sertoli cell surface (). These results are in agreement with previously published results, which showed Sertoli cell barrier function to be regulated negatively by TNFα.Citation24
Figure 4. Localization of MMP9 and MT1-MMP in Sertoli cells cultured in the presence of TNFα. Sertoli cells (0.04 × 106 cells/cm2) were cultured on Matrigel™-coated micro cover glasses for 4 d and incubated with TNFα (25 ng/ml) for 24 h as described in Materials and Methods. Thereafter, cells were processed for the immunofluorescent visualization of MMP9 (red, a and b) and MT1-MMP (red, c and d). Nuclei were visualized with DAPI (blue, a–d). Images encircled in blue boxes and shown to the immediate right of a–h are enlarged images corresponding to boxed areas within a–h. Gray scale images (e–h) are included to better depict changes in MMP9 and MT1-MMP. Scale bars, 20 μm.
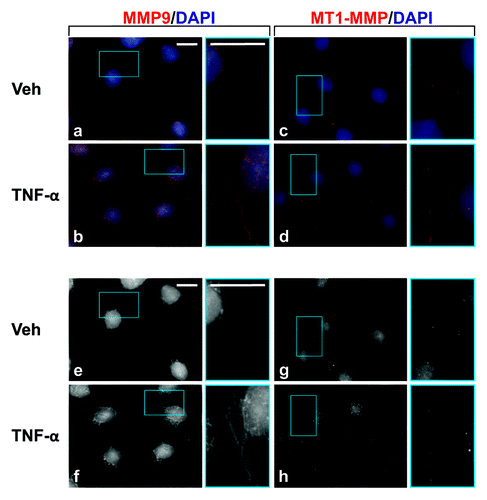
Figure 5. Effects of TNFα on protein distribution within Sertoli cells. Sertoli cells (0.04 × 106 cells/cm2) were cultured on Matrigel™-coated micro cover glasses for 4 d and treated with TNFα (25 ng/ml) for 24 h as described in Materials and Methods. Sertoli cells were then dual-labeled for occludin (red)/ZO-1 (green), or labeled for F-actin or cortactin (both green). Corresponding images were merged to show areas of co-localization (orange, A). Nuclei were visualized with DAPI (blue, A and B). Scale bars, 20 μm.
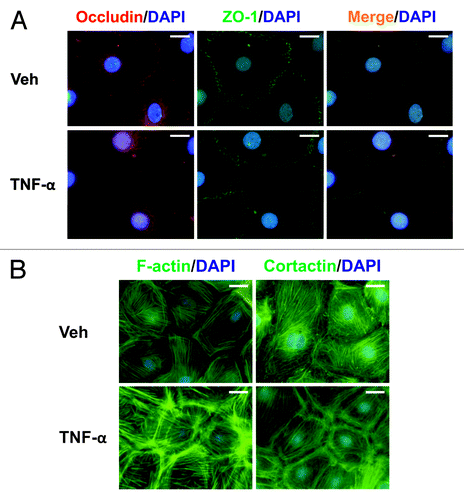
TNFα affects the actin cytoskeleton in Sertoli cells in vitro
The effects of TNFα on the Sertoli cell actin cytoskeleton were investigated by staining F-actin with fluorescently-tagged phalloidin or cortactin with a cortactin antibody. In Veh-treated Sertoli cells, actin stress fibers ran parallel across cells (). The localization of cortactin, an actin-binding protein known to regulate branched actin assembly,Citation46,Citation47 in control Sertoli cells was similar to that of F-actin (). Cortactin-positive dot-like structures were also observed in Veh-treated cells. Following TNFα treatment, both F-actin and cortactin were found to aggregate at the periphery of Sertoli cells ().
Discussion
BTB restructuring, one of the most complicated cellular events to occur during spermatogenesis, is orchestrated by multiple players, including proteases/protease inhibitors, kinases/phosphatases, cytokines, structural proteins and their associated adaptor proteins.Citation7,Citation8 For instance, numerous studies have shown cytokines such as TNFα and interleukin-1α (IL-1α) to disrupt Sertoli cell barrier/BTB function,Citation24,Citation25,Citation48-Citation50 except that a complete understanding of the mechanisms involved is still lacking. TNFα is a pro-inflammatory cytokine produced by pachytene spermatocytes and round spermatids as well as by activated monocytes and macrophages in the interstitium.Citation21,Citation51,Citation52 In the mammalian testis, TNFα has pleiotropic roles; at low concentrations it acts as a survival factor, but at high concentrations it triggers cell death. The study by Boussouar et al. provides a good example of how TNFα behaves as a survival factor for post-meiotic germ cells. Here, low doses of the cytokine (ED50 = 2.5 ng/ml) were found to stimulate lactate dehydrogenase A, a critical enzyme involved in lactate production, in primary Sertoli cells.Citation53 TNFα also functions in inflammation and immunoregulation, reinforcing its role as a multifunctional molecule in the testis.
Previous studies have shown TNFα to upregulate proteases/protease inhibitors [e.g., MMP9 and tissue inhibitor of metalloproteases 1 (TIMP 1)], downregulate transmembrane protein levels (e.g., occludin, JAM-A and CAR) and trigger protein internalization (e.g., occludin and JAM-A) in Sertoli cells.Citation22-Citation25 Equally important, these events culminated in Sertoli cell barrier/BTB disruption in vitro and in vivo.Citation24,Citation25 At the in vivo level, we wonder why and how pachytene spermatocytes and round spermatids residing in the adluminal compartment of the seminiferous epithelium modulate BTB function. It is possible that germ cells residing near the BTB (i.e., preleptotene and leptotene spermatocytes) do not produce TNFα because they are not equipped with inherent mechanisms to control precisely the concentration of TNFα throughout spermatogenesis. This is critical since misregulation of TNFα by preleptotene and leptotene spermatocytes (that is, if they were to produce the cytokine) would be detrimental to BTB function and fertility. The production of TNFα by germ cells residing above the BTB may be linked to the disassembly of the old BTB, which is supported by its cyclic production during the seminiferous epithelial cycle in the rat.Citation51 While activation of MAPK signaling appears to be involved in these cellular events,Citation19,Citation25,Citation54 additional studies are needed to expand the role of TNFα in the testis.
In agreement with a previously published report from this laboratory,Citation24 TNFα increased the steady-state level of MMP9 (alternatively known as gelatinase-B) in Sertoli cells in vitro. MMPs are zinc-dependent soluble endopeptidases capable of degrading several biomolecules, including extracellular matrix proteins (e.g., collagen), growth factors, growth factor receptors, cytokines, proteases, protease inhibitors and cell adhesion proteins at physiological pH.Citation55-Citation57 They contain at least three conserved domains: the pro-peptide domain, the catalytic domain and the hemopexin-like C-terminal domain, which is linked to the catalytic domain by a hinge region. MMPs are synthesized as inactive zymogens (i.e., pro-enzymes) but activated by proteases that remove the pro-peptide domain. Once activated, they are inhibited by TIMPs.Citation58 However, important differences are known to exist across different MMP family members. For instance, MT-MMPs are anchored to the plasma membrane by a transmembrane domain.Citation59 At the cell surface, MT1-MMP activity is tightly controlled, with localization to lipid rafts/caveolae and internalization playing major regulatory roles.Citation60 Active MT1-MMP (~54 kDa) can also be auto-catalytically cleaved into a ~44 kDa membrane-tethered fragment lacking the catalytic domain (44-MT1) and a ~20 kDa soluble fragment containing the catalytic domain via ectodomain shedding.Citation61-Citation63 Even though both fragments were reported to be inactive, 44-MT1 was subsequently found to regulate indirectly the amount of active MT1-MMP at the cell surface by controlling its rate of internalization.Citation64 Results from IHC and IF staining experiments described herein illustrate that germ cells were highly immunoreactive for MMP (but less so for MT1-MMP). While staining experiments could not distinguish between active and inactive forms, these results clearly illustrate the involvement of germ cells in the breakdown of the extracellular milieu during cell movement.
In this study, TNFα also increased the steady-state level of mICAM-1 in Sertoli cells, consistent with previously published reports.Citation18-Citation20,Citation54 mICAM-1 is an immunoglobulin-like adhesion and signaling protein that is indispensable for the movement of leukocytes across the endothelium during inflammation.Citation13,Citation15,Citation65,Citation66 In an earlier study, we reported on the role of mICAM-1 in BTB dynamics.Citation14 Specifically, we showed transient mICAM-1 overexpression to upregulate Sertoli cell barrier function, indicating its importance in BTB integrity. Since mICAM-1 was previously shown to localize to the Sertoli cell barrier/BTB, its upregulation in Sertoli cells by TNFα may be critical for transient states of (weak?) adhesion as preleptotene/leptotene spermatocytes cross the BTB during spermatogenesis. While germ cells were not included in this in vitro study, restructuring of the Sertoli cell barrier alone is likely to involve important signaling events that underlie germ cell movement in vivo. Connecting these in vitro findings with in vivo consequences will require additional research. For instance, additional experiments are needed to better define, for instance, the ligand for mICAM-1 in germ cells (note: germ cells also express mICAM-1Citation14) since transient protein-proteins interactions are likely to be established during germ cell movement. It would also be interesting to investigate if mICAM-1 is an endpoint of androgen signaling since AR was upregulated following TNFα treatment.Citation43 In other systems, mICAM-1 was found to bind MMP9 as well as Tyr phosphorylated cortactin,Citation67-Citation69 suggesting that these proteins may also be part of a functional complex in the seminiferous epithelium. Cortactin, an actin-binding protein, is usually found at the edge of migrating cells where it co-localizes with ARP2/3.Citation46,Citation47 With roles in cell adhesion, cell migration and endocytosis, we speculate that cortactin is one of many proteins involved in restructuring of the Sertoli cell actin cytoskeleton following TNFα treatment. Interestingly, cortactin has been shown to regulate the secretion and cell surface localization of MMPs.Citation70,Citation71 It would be interesting to investigate if cortactin has a similar role in the seminiferous epithelium.
Herein, we have investigated the effects of TNFα on Sertoli cell barrier dynamics. While many more important studies remain to be performed, our findings have demonstrated the importance of MMPs, mICAM-1 and the actin cytoskeleton in TNFα-mediated Sertoli cell barrier restructuring.
Abbreviations: | ||
BTB | = | blood-testis barrier |
DAPI | = | 4’,6-diamidino-2-phenylindole |
FSH | = | follicle stimulating hormone |
GC | = | germ cell |
ICAM-1 | = | intercellular adhesion molecule-1 |
IF | = | immunofluorescent staining |
IHC | = | immunohistochemical staining |
LBRC | = | lateral border recycling compartment |
LH | = | luteinizing hormone |
mICAM-1 | = | membrane-bound ICAM-1 |
MMP | = | matrix metalloprotease |
MT1-MMP | = | membrane type-1 matrix metalloprotease |
PECAM | = | platelet/endothelial cell adhesion molecule |
SC | = | Sertoli cell |
sICAM-1 | = | soluble ICAM-1 |
ST | = | seminiferous tubule |
T | = | testis |
TBS | = | Tris-buffered saline |
TNFα | = | tumor necrosis factor α |
Acknowledgments
This work was supported in part by NICHD, NIH (R03 HD061401 to D.D.M.; R01 HD056034 and U54 HD029990 Project 5 to C.Y.C.) and by the Ministry of Science and Higher Education (K/ZDS/002590 to B.B.)
Disclosure of Potential Conflicts of Interest
No potential conflicts of interest were disclosed.
References
- de Kretser DM, Kerr JB. in The Physiology of Reproduction. (eds Knobil, E et al.) 837-932 (Raven Press, 1988).
- Kerr JB, Loveland KL, O'Bryan MK, de Kretser DM. in Knobil and Neill's Physiology of Reproduction. (ed Neill, JD) 827-947 (Elsevier, 2006).
- Carreau S, Hess RA. Oestrogens and spermatogenesis. Philos Trans R Soc Lond B Biol Sci 2010; 365:1517 - 35; http://dx.doi.org/10.1098/rstb.2009.0235; PMID: 20403867
- Stanton H, Melrose J, Little CB, Fosang AJ. Proteoglycan degradation by the ADAMTS family of proteinases. Biochim Biophys Acta 2011; 1812:1616 - 29; http://dx.doi.org/10.1016/j.bbadis.2011.08.009; PMID: 21914474
- Russell LD. The blood-testis barrier and its formation relative to spermatocyte maturation in the adult rat: a lanthanum tracer study. Anat Rec 1978; 190:99 - 111; http://dx.doi.org/10.1002/ar.1091900109; PMID: 626419
- Setchell BP, Waites GMB. in The Handbook of Physiology. (eds Hamilton, DW & Greep, RO) 143-172 (Williams and Wilkens, 1975).
- Cheng CY, Mruk DD. The blood-testis barrier and its implications for male contraception. Pharmacol Rev 2012; 64:16 - 64; http://dx.doi.org/10.1124/pr.110.002790; PMID: 22039149
- Mruk DD, Cheng CY. Sertoli-Sertoli and Sertoli-germ cell interactions and their significance in germ cell movement in the seminiferous epithelium during spermatogenesis. Endocr Rev 2004; 25:747 - 806; http://dx.doi.org/10.1210/er.2003-0022; PMID: 15466940
- Russell LD. Movement of spermatocytes from the basal to the adluminal compartment of the rat testis. Am J Anat 1977; 148:313 - 28; http://dx.doi.org/10.1002/aja.1001480303; PMID: 857632
- Cheng CY, Mruk DD. Cell junction dynamics in the testis: Sertoli-germ cell interactions and male contraceptive development. Physiol Rev 2002; 82:825 - 74; PMID: 12270945
- Russell LD. in The Sertoli Cell. (eds Russell, LD & Griswold, MD) 365-390 (Cache River Press, 1993).
- Yan HHN, Mruk DD, Lee WM, Cheng CY. Blood-testis barrier dynamics are regulated by testosterone and cytokines via their differential effects on the kinetics of protein endocytosis and recycling in Sertoli cells. FASEB J 2008; 22:1945 - 59; http://dx.doi.org/10.1096/fj.06-070342; PMID: 18192323
- Nourshargh S, Hordijk PL, Sixt M. Breaching multiple barriers: leukocyte motility through venular walls and the interstitium. Nat Rev Mol Cell Biol 2010; 11:366 - 78; http://dx.doi.org/10.1038/nrm2889; PMID: 20414258
- Xiao X, Cheng CY, Mruk DD. Intercellular adhesion molecule-1 is a regulator of blood-testis barrier function. J Cell Sci 2012; http://dx.doi.org/10.1242/jcs.107987; PMID: 22976294
- Lawson C, Wolf S. ICAM-1 signaling in endothelial cells. Pharmacol Rep 2009; 61:22 - 32; PMID: 19307690
- Witkowska AM, Borawska MH. Soluble intercellular adhesion molecule-1 (sICAM-1): an overview. Eur Cytokine Netw 2004; 15:91 - 8; PMID: 15319166
- van de Stolpe A, van der Saag PT. Intercellular adhesion molecule-1. J Mol Med (Berl) 1996; 74:13 - 33; http://dx.doi.org/10.1007/BF00202069; PMID: 8834767
- Ziparo E, Riccioli A, Filippini A, De Cesaris P, Barbacci E. TNF-α induces surface modifications in mouse Sertoli cells: physiopathological implications. Ital J Anat Embryol 1995; 100:Suppl 1 553 - 62; PMID: 11322336
- De Cesaris P, Starace D, Starace G, Filippini A, Stefanini M, Ziparo E. Activation of Jun N-terminal kinase/stress-activated protein kinase pathway by tumor necrosis factor α leads to intercellular adhesion molecule-1 expression. J Biol Chem 1999; 274:28978 - 82; http://dx.doi.org/10.1074/jbc.274.41.28978; PMID: 10506145
- Riccioli A, Filippini A, De Cesaris P, Barbacci E, Stefanini M, Starace G, et al. Inflammatory mediators increase surface expression of integrin ligands, adhesion to lymphocytes, and secretion of interleukin 6 in mouse Sertoli cells. Proc Natl Acad Sci USA 1995; 92:5808 - 12; http://dx.doi.org/10.1073/pnas.92.13.5808; PMID: 7541137
- De SK, Chen HL, Pace JL, Hunt JS, Terranova PF, Enders GC. Expression of tumor necrosis factor-α in mouse spermatogenic cells. Endocrinology 1993; 133:389 - 96; http://dx.doi.org/10.1210/en.133.1.389; PMID: 8319585
- Xia W, Wong EWP, Mruk DD, Cheng CY. TGF-β3 and TNFalpha perturb blood-testis barrier (BTB) dynamics by accelerating the clathrin-mediated endocytosis of integral membrane proteins: a new concept of BTB regulation during spermatogenesis. Dev Biol 2009; 327:48 - 61; http://dx.doi.org/10.1016/j.ydbio.2008.11.028; PMID: 19103189
- Wang CQF, Mruk DD, Lee WM, Cheng CY. Coxsackie and adenovirus receptor (CAR) is a product of Sertoli and germ cells in rat testes which is localized at the Sertoli-Sertoli and Sertoli-germ cell interface. Exp Cell Res 2007; 313:1373 - 92; http://dx.doi.org/10.1016/j.yexcr.2007.01.017; PMID: 17359973
- Siu MKY, Lee WM, Cheng CY. The interplay of collagen IV, tumor necrosis factor-α, gelatinase B (matrix metalloprotease-9), and tissue inhibitor of metalloproteases-1 in the basal lamina regulates Sertoli cell-tight junction dynamics in the rat testis. Endocrinology 2003; 144:371 - 87; http://dx.doi.org/10.1210/en.2002-220786; PMID: 12488366
- Li MWM, Xia W, Mruk DD, Wang CQ, Yan HH, Siu MK, et al. Tumor necrosis factor α reversibly disrupts the blood-testis barrier and impairs Sertoli-germ cell adhesion in the seminiferous epithelium of adult rat testes. J Endocrinol 2006; 190:313 - 29; http://dx.doi.org/10.1677/joe.1.06781; PMID: 16899565
- Mruk DD, Siu MK, Conway AM, Lee NP, Lau AS, Cheng CY. Role of tissue inhibitor of metalloproteases-1 in junction dynamics in the testis. J Androl 2003; 24:510 - 23; PMID: 12826691
- Cheng CY, Mather JP, Byer AL, Bardin CW. Identification of hormonally responsive proteins in primary Sertoli cell culture medium by anion-exchange high performance liquid chromatography. Endocrinology 1986; 118:480 - 8; http://dx.doi.org/10.1210/endo-118-2-480; PMID: 3080306
- Mruk DD, Cheng CY. An in vitro system to study Sertoli cell blood-testis barrier dynamics. Methods Mol Biol 2011; 763:237 - 52; http://dx.doi.org/10.1007/978-1-61779-191-8_16; PMID: 21874456
- Galdieri M, Ziparo E, Palombi F, Russo MA, Stefanini M. Pure Sertoli cell cultures: a new model for the study of somatic-germ cell interactions. J Androl 1981; 5:249 - 59
- Kopera I, Durlej M, Hejmej A, Knapczyk-Stwora K, Duda M, Slomczynska M, et al. Differential expression of connexin 43 in adult pig testes during normal spermatogenic cycle and after flutamide treatment. Reprod Domest Anim 2011; 46:1050 - 60; http://dx.doi.org/10.1111/j.1439-0531.2011.01783.x; PMID: 21457361
- Aravindan GR, Pineau CP, Bardin CW, Cheng CY. Ability of trypsin in mimicking germ cell factors that affect Sertoli cell secretory function. J Cell Physiol 1996; 168:123 - 33; http://dx.doi.org/10.1002/(SICI)1097-4652(199607)168:1<123::AID-JCP15>3.0.CO;2-8; PMID: 8647906
- Zwain IH, Cheng CY. Rat seminiferous tubular culture medium contains a biological factor that inhibits Leydig cell steroidogenesis: its purification and mechanism of action. Mol Cell Endocrinol 1994; 104:213 - 27; http://dx.doi.org/10.1016/0303-7207(94)90124-4; PMID: 7988748
- Lee NPY, Mruk DD, Conway AM, Cheng CY. Zyxin, axin, and Wiskott-Aldrich syndrome protein are adaptors that link the cadherin/catenin protein complex to the cytoskeleton at adherens junctions in the seminiferous epithelium of the rat testis. J Androl 2004; 25:200 - 15; PMID: 14760006
- Zong SD, Bardin CW, Phillips D, Cheng CY. Testins are localized to the junctional complexes of rat Sertoli and epididymal cells. Biol Reprod 1992; 47:568 - 72; http://dx.doi.org/10.1095/biolreprod47.4.568; PMID: 1391343
- Zong SD, Zhu LJ, Grima J, Aravindan GR, Bardin CW, Cheng CY. Cyclic and postnatal developmental changes of testin in the rat seminiferous epithelium--an immunohistochemical study. Biol Reprod 1994; 51:843 - 51; http://dx.doi.org/10.1095/biolreprod51.5.843; PMID: 7849186
- Cheng CY, Bardin CW. Identification of two testosterone-responsive testicular proteins in Sertoli cell-enriched culture medium whose secretion is suppressed by cells of the intact seminiferous tubule. J Biol Chem 1987; 262:12768 - 79; PMID: 3624278
- Siu MKY, Cheng CY. Interactions of proteases, protease inhibitors, and the β1 integrin/laminin gamma3 protein complex in the regulation of ectoplasmic specialization dynamics in the rat testis. Biol Reprod 2004; 70:945 - 64; http://dx.doi.org/10.1095/biolreprod.103.023606; PMID: 14645107
- Longin J, Guillaumot P, Chauvin MA, Morera AM, Le Magueresse-Battistoni B. MT1-MMP in rat testicular development and the control of Sertoli cell proMMP-2 activation. J Cell Sci 2001; 114:2125 - 34; PMID: 11493648
- Smith BV, Lacy D. Residual bodies of seminiferous tubules of the rat. Nature 1959; 184:249 - 51; http://dx.doi.org/10.1038/184249a0; PMID: 13831896
- Kerr JB, de Kretser DM. Proceedings: The role of the Sertoli cell in phagocytosis of the residual bodies of spermatids. J Reprod Fertil 1974; 36:439 - 40; http://dx.doi.org/10.1530/jrf.0.0360439; PMID: 4819326
- Osenkowski P, Meroueh SO, Pavel D, Mobashery S, Fridman R. Mutational and structural analyses of the hinge region of membrane type 1-matrix metalloproteinase and enzyme processing. J Biol Chem 2005; 280:26160 - 8; http://dx.doi.org/10.1074/jbc.M414379200; PMID: 15901740
- Yao PL, Lin YC, Richburg JH. TNF α-mediated disruption of spermatogenesis in response to Sertoli cell injury in rodents is partially regulated by MMP2. Biol Reprod 2009; 80:581 - 9; http://dx.doi.org/10.1095/biolreprod.108.073122; PMID: 19038859
- Delfino FJ, Boustead JN, Fix C, Walker WH. NF-kappaB and TNF-α stimulate androgen receptor expression in Sertoli cells. Mol Cell Endocrinol 2003; 201:1 - 12; http://dx.doi.org/10.1016/S0303-7207(03)00005-4; PMID: 12706288
- Gingras D, Béliveau R. Emerging concepts in the regulation of membrane-type 1 matrix metalloproteinase activity. Biochim Biophys Acta 2010; 1803:142 - 50; http://dx.doi.org/10.1016/j.bbamcr.2009.04.011; PMID: 19409422
- Koziol A, Martín-Alonso M, Clemente C, Gonzalo P, Arroyo AG. Site-specific cellular functions of MT1-MMP. Eur J Cell Biol 2012; 91:889 - 95; http://dx.doi.org/10.1016/j.ejcb.2012.07.003; PMID: 22939226
- Kirkbride KC, Sung BH, Sinha S, Weaver AM. Cortactin: a multifunctional regulator of cellular invasiveness. Cell Adh Migr 2011; 5:187 - 98; http://dx.doi.org/10.4161/cam.5.2.14773; PMID: 21258212
- Ren GR, Crampton MS, Yap AS. Cortactin: Coordinating adhesion and the actin cytoskeleton at cellular protrusions. Cell Motil Cytoskeleton 2009; 66:865 - 73; http://dx.doi.org/10.1002/cm.20380; PMID: 19437513
- Sarkar O, Mathur PP, Cheng CY, Mruk DD. Interleukin 1 α (IL1A) is a novel regulator of the blood-testis barrier in the rat. Biol Reprod 2008; 78:445 - 54; http://dx.doi.org/10.1095/biolreprod.107.064501; PMID: 18057314
- Lie PPY, Cheng CY, Mruk DD. Interleukin-1alpha is a regulator of the blood-testis barrier. FASEB J 2011; 25:1244 - 53; http://dx.doi.org/10.1096/fj.10-169995; PMID: 21191089
- Li MWM, Mruk DD, Lee WM, Cheng CY. Cytokines and junction restructuring events during spermatogenesis in the testis: an emerging concept of regulation. Cytokine Growth Factor Rev 2009; 20:329 - 38; http://dx.doi.org/10.1016/j.cytogfr.2009.07.007; PMID: 19651533
- O'Bryan MK, Hedger MP. in Molecular Mechanisms in Spermatogenesis. (ed Cheng, CY) 92-114 (Landes Bioscience and Springer Science+Business Media, 2008).
- Hutson JC. Secretion of tumor necrosis factor α by testicular macrophages. J Reprod Immunol 1993; 23:63 - 72; http://dx.doi.org/10.1016/0165-0378(93)90027-F; PMID: 8429525
- Boussouar F, Grataroli R, Ji J, Benahmed M. Tumor necrosis factor-α stimulates lactate dehydrogenase A expression in porcine cultured Sertoli cells: mechanisms of action. Endocrinology 1999; 140:3054 - 62; http://dx.doi.org/10.1210/en.140.7.3054; PMID: 10385397
- De Cesaris P, Starace D, Riccioli A, Padula F, Filippini A, Ziparo E. Tumor necrosis factor-α induces interleukin-6 production and integrin ligand expression by distinct transduction pathways. J Biol Chem 1998; 273:7566 - 71; http://dx.doi.org/10.1074/jbc.273.13.7566; PMID: 9516459
- Page-McCaw A, Ewald AJ, Werb Z. Matrix metalloproteinases and the regulation of tissue remodelling. Nat Rev Mol Cell Biol 2007; 8:221 - 33; http://dx.doi.org/10.1038/nrm2125; PMID: 17318226
- Visse R, Nagase H. Matrix metalloproteinases and tissue inhibitors of metalloproteinases: structure, function, and biochemistry. Circ Res 2003; 92:827 - 39; http://dx.doi.org/10.1161/01.RES.0000070112.80711.3D; PMID: 12730128
- Egeblad M, Werb Z. New functions for the matrix metalloproteinases in cancer progression. Nat Rev Cancer 2002; 2:161 - 74; http://dx.doi.org/10.1038/nrc745; PMID: 11990853
- Murphy G. Tissue inhibitors of metalloproteinases. Genome Biol 2011; 12:233; http://dx.doi.org/10.1186/gb-2011-12-11-233; PMID: 22078297
- Sato H, Takino T, Okada Y, Cao J, Shinagawa A, Yamamoto E, et al. A matrix metalloproteinase expressed on the surface of invasive tumour cells. Nature 1994; 370:61 - 5; http://dx.doi.org/10.1038/370061a0; PMID: 8015608
- Poincloux R, Lizárraga F, Chavrier P. Matrix invasion by tumour cells: a focus on MT1-MMP trafficking to invadopodia. J Cell Sci 2009; 122:3015 - 24; http://dx.doi.org/10.1242/jcs.034561; PMID: 19692588
- Toth M, Hernandez-Barrantes S, Osenkowski P, Bernardo MM, Gervasi DC, Shimura Y, et al. Complex pattern of membrane type 1 matrix metalloproteinase shedding. Regulation by autocatalytic cells surface inactivation of active enzyme. J Biol Chem 2002; 277:26340 - 50; http://dx.doi.org/10.1074/jbc.M200655200; PMID: 12004057
- Lehti K, Valtanen H, Wickström SA, Lohi J, Keski-Oja J. Regulation of membrane-type-1 matrix metalloproteinase activity by its cytoplasmic domain. J Biol Chem 2000; 275:15006 - 13; http://dx.doi.org/10.1074/jbc.M910220199; PMID: 10748199
- Lohi J, Lehti K, Westermarck J, Kähäri VM, Keski-Oja J. Regulation of membrane-type matrix metalloproteinase-1 expression by growth factors and phorbol 12-myristate 13-acetate. Eur J Biochem 1996; 239:239 - 47; http://dx.doi.org/10.1111/j.1432-1033.1996.0239u.x; PMID: 8706726
- Cho JA, Osenkowski P, Zhao H, Kim S, Toth M, Cole K, et al. The inactive 44-kDa processed form of membrane type 1 matrix metalloproteinase (MT1-MMP) enhances proteolytic activity via regulation of endocytosis of active MT1-MMP. J Biol Chem 2008; 283:17391 - 405; http://dx.doi.org/10.1074/jbc.M708943200; PMID: 18413312
- Muller WA. Leukocyte-endothelial-cell interactions in leukocyte transmigration and the inflammatory response. Trends Immunol 2003; 24:327 - 34; http://dx.doi.org/10.1016/S1471-4906(03)00117-0; PMID: 12810109
- Muller WA. Mechanisms of leukocyte transendothelial migration. Annu Rev Pathol 2011; 6:323 - 44; http://dx.doi.org/10.1146/annurev-pathol-011110-130224; PMID: 21073340
- Fiore E, Fusco C, Romero P, Stamenkovic I. Matrix metalloproteinase 9 (MMP-9/gelatinase B) proteolytically cleaves ICAM-1 and participates in tumor cell resistance to natural killer cell-mediated cytotoxicity. Oncogene 2002; 21:5213 - 23; http://dx.doi.org/10.1038/sj.onc.1205684; PMID: 12149643
- Sultan S, Gosling M, Nagase H, Powell JT. Shear stress-induced shedding of soluble intercellular adhesion molecule-1 from saphenous vein endothelium. FEBS Lett 2004; 564:161 - 5; http://dx.doi.org/10.1016/S0014-5793(04)00337-0; PMID: 15094060
- Tilghman RW, Hoover RL. The Src-cortactin pathway is required for clustering of E-selectin and ICAM-1 in endothelial cells. FASEB J 2002; 16:1257 - 9; PMID: 12060669
- Clark ES, Weaver AM. A new role for cortactin in invadopodia: regulation of protease secretion. Eur J Cell Biol 2008; 87:581 - 90; http://dx.doi.org/10.1016/j.ejcb.2008.01.008; PMID: 18342393
- Clark ES, Whigham AS, Yarbrough WG, Weaver AM. Cortactin is an essential regulator of matrix metalloproteinase secretion and extracellular matrix degradation in invadopodia. Cancer Res 2007; 67:4227 - 35; http://dx.doi.org/10.1158/0008-5472.CAN-06-3928; PMID: 17483334