Abstract
In Drosophila, dosage compensation is mediated by the MSL complex, which binds numerous sites on the X chromosome in males and enhances the transcriptional rate of a substantial number of X-linked genes. We have determined that topoisomerase II (Topo II) is enriched on dosage compensated genes, to which it is recruited by association with the MSL complex, in excess of the amount that is present on autosomal genes with similar transcription levels. Using a plasmid model, we show that Topo II is required for proper dosage compensation and that compensated chromatin is topologically different from non-compensated chromatin. This difference, which is not the result of the enhanced transcription level due of X-linked genes and which represents a structural modification intrinsic to the DNA of compensated chromatin, requires the function of Topo II. Our results suggest that Topo II is an integral part of the mechanistic basis of dosage compensation.
Introduction
Dosage compensation refers to the equalization of most X-linked gene products between males and females. In Drosophila, it is mediated by the MSL complex, consisting of a core of five protein subunits [encoded by male-specific lethal 1, 2 and 3 (msl1, msl2, msl3), males absent on the first (mof) and maleless (mle)] as well as one of two non-coding RNAs [RNA on the X1 and 2 (roX1 and roX2)]. MLE is an ATPase-helicase that unwinds short double-stranded RNA or RNA/DNA duplexes in vitroCitation1; MOF is a member of the MYST subfamily of histone acetyltransferases and specifically acetylates histone H4 at lysine 16Citation2; MSL2 is an E3 ubiquitin ligase that targets lysine 34 on histone H2B in vitro.Citation3 The MSL complex preferentially associates with numerous sites on the X chromosome in somatic cells of males but not of females. It is responsible for an enhancement of the transcriptional rate of the vast majority of X-linked genes,Citation4 thereby compensating for the difference in dosage of these genes between the sexes (see reviewsCitation5-Citation7).
A functional link between chromatin architecture and dosage compensation has long been suspected.Citation8,Citation9 Depletion of supercoiling factor (SCF), which is normally associated with actively transcribed genes, has no apparent effect on chromosome morphology or on the distribution of the MSL complex along the male X; yet, loss of SCF affects male viability due to a sex-specific decrease in the transcription of X-linked genes.Citation10 SCF alters the superhelicity of relaxed circular DNA in the presence of topoisomerase II,Citation11 demonstrating that changes in DNA supercoiling can occur independently from transcription.Citation12 These observations led us to investigate whether either of the two major topoisomerases present in Drosophila cells (Topo I and Topo II) plays a role in dosage compensation. In the current study, we demonstrate that Topo II is required for the proper dosage compensation of a plasmid model system and that it is enriched on X-linked genes to a degree that exceeds the amount that is present on autosomal genes with similar transcription levels. Topo II is recruited to dosage compensated genes by the MSL complex through an RNA-dependent association.
Given these results, we used a plasmid system that faithfully reproduces the enhancement of a reporter gene by the dosage compensation mechanismCitation13 to investigate whether Topo II may contribute to the topological changes that underlie transcriptional enhancement.Citation14 We demonstrate a specific effect of Topo II on the topology of compensated genes. These results suggest a role for Topo II in dosage compensation that is additional to and different from its function in restoring normal DNA superhelicity during the transcription process.
Materials and Methods
S2 transfection and luciferase assays
Transfections were performed as previously described.Citation13 Briefly, S2 cells were transfected with relaxed plasmids bearing a firefly luciferase gene under the control of an inducible promoter. The plasmids contain a roX gene sequence that nucleates the MSL complex or a trivial sequence. Four to five days after transfection, luciferase activity was determined by using the dual luciferase reporter assay (Promega). Each measurement is the average of three to four independent biological replicates. See details in supplementary material.
Inhibitors treatment and RNA interference
One day before transfection, cells were treated with sub-cytotoxic doses of drugs that gave comparable level of cell cycle arrest, 0.3µM ICRF-193 (Sigma) or 0.06µM Paclitaxel (Sigma). The concentration of the drugs was kept constant during cell culturing. For RNA interference, 1 to 3 d prior to transfection cells were treated with 10 µg/ml double-stranded RNA. Additional dsRNA was added throughout the experiment to maintain the 10 µg/ml concentration. In instances where double knockdown was performed, the concentration of double-stranded RNAs was kept equal in all the samples. dsRNA was made following Ambion's MEGAscript protocol. A list of the primers used and the details of the various treatments are provided in supplementary material.
ChIP-chip
ChIP was performed as describedCitation15 using Drosophila S2 cells and a rabbit anti-Topo II antibody (a gift from Paul Fisher), and validated as described in Ramos et al.Citation16 Sample labeling and hybridization were performed by the FSU-NimbleGen Microarray Facility using a NimbleGen D. melanogaster ChIP-chip 2.1M Whole-Genome Tiling array (DM5 build). Two biological replicas were normalized using quantile normalization between the chips (GSE 36927). This normalized data was then used to determine enrichment levels of Topo II.
Genome analysis
Metagene plots were derived as described by Larschan et al.Citation17 with exception that the entire gene body was scaled to a 1 kb frame. The gene list used for metagene analysis was derived from GEO data set GSE16344 for S2 cells and GSE45411 for Kc cells. All the genes were further filtered to remove genes with excess copy number as determined by Zhang et al.Citation18 for S2 cells. The filtered genes were then used to generate the metagene plots. A gene list is provided in supplementary material for the expression rate comparison metagene plot. The data from Alekseyenko et al. and Straub et al.Citation19,Citation20 was used for the entry sites analysis.
Immunofluorescence analysis of polytene chromosomes
Immunostaining of polytene chromosomes was performed as previously describedCitation16 using the α-MSL2 (1:200) primary and an Alexa Fluor secondary antibodies.
Analysis of topoisomers
Four days after transfection, DNA was isolated and run in a chloroquine gel for subsequent Southern blot analysis. See supplementary material for details. The center of the topoisomers distribution was obtained using the method described by Morse.Citation21 Briefly, the band with the greatest intensity is assigned a linking number of zero and linking numbers of -1, -2, etc. or +1, +2, etc. are assigned to the slower and faster bands, respectively. A straight line with slope m and intersect b is obtained by plotting (1/∆Lki)*ln (Ii/Imax) vs. ∆Lki, in which ∆Lki corresponds to the relative linking number assigned to a band i having relative intensity Ii, and Imax is the intensity of the most intense band. The centers of the topoisomer distributions, indicating positive or negative changes in linking number, were ranked using the distance, b/2m, from the most intense band.
Psoralen cross-linking
Cells transfected with roX-bearing plasmids marked with bromodeoxyuridine (BrdU) were incubated with trimethylpsoralen for 5 min at RT and then exposed to 360 nm UV light for cross-linking. Control cells were exposed to 313nm UV light for 20 min at 4°C to nick the plasmid DNA prior to psoralen treatment. After extraction, the DNA was digested to obtain the entire firefly gene, and denatured with glyoxal at 55°C for 1 h. The samples were loaded in an agarose gel for Southern blot hybridization. Data analysis was performed as described in Sinden et al.Citation22 See details in supplementary material.
RT-PCR
RNA was isolated from S2 cells using the Qiagen RNeasy Plus mini-kit with on-column DNA digestion, following the manufacturer’s protocols. Reverse transcription-PCR was performed using Qiagen Onestep RT-PCR kit. See supplementary material for a list of the primers.
qRT-PCR
Real-time, reverse transcription-PCR was performed using the iScript One-step RT-PCR kit with SYBR Green (BIO-RAD). Reactions were run on a C1000 thermal cycler with a CFX96 System (Bio-Rad). Differential gene expression analysis was performed according to the method of Pfaffl (2001). Standard curves for each primer pair were obtained to determine their pairing efficiency. GPDH, α-Tub and Pka-C1 were used to normalize X chromosome and autosomal transcription measurements. The results of three independent biological replicates were averaged. A list of the primers use is provided as supplementary material.
Immunoprecipitation
S2 cells expressing FLAG-tagged proteins were lysed and either treated or not with RNase. Extracts were incubated with anti-FLAG M2 agarose beads (Sigma) overnight at 4°C. Complexes were washed in PBS buffer 1% Tween-20 and resuspended in loading buffer or eluted with FLAG peptide (Sigma). The samples were used for western blot analysis. See supplementary material for details.
MNase sensitivity assay
The assay was performed as reported in Dunlap et al.Citation14 Briefly, nuclei isolated from S2 cells transfected with a roX-bearing plasmid and treated either with MSL2 dsRNA or with GFP dsRNA, were exposed to MNase. Following DNA extraction, the percentage of protection was determined by qPCR analysis of digested and undigested samples using the iQ SYBR Green Supermix (Bio-Rad). A list of the primers used is provided as supplementary material.
Results
The occurrence of dosage compensation on a circular plasmid transfected into S2 cellsCitation13 allows us to investigate effects of chromatin modifications that may underlie the mechanism of transcriptional enhancement. Plasmids containing a reporter gene (Firefly luciferase) under the control of the tetracycline resistance operator promoter (TetO), with or without a DNA fragment from the roX2 gene, are transfected and transiently expressed in cultured S2 cells (a male Drosophila cell line). The roX2 sequence nucleates the MSL complex and results in the approximate 2-fold enhancement of transcription of the Firefly luciferase gene. Alternatively, as a non-dosage compensated control, the roX2-bearing plasmid is transfected into cells where the MSL complex has been depleted by RNA interference. As a control for levels of transfection, when the Firefly luciferase levels of roX2-bearing plasmids isolated from different groups of cells are compared, a plasmid carrying the Renilla luciferase under the control of the Copia promoter is co-transfected, and the Firefly/Renilla luciferase ratios are used. All transfections are performed with plasmids relaxed with Topo I in order to eliminate topological modifications that are introduced during amplification in bacterial cells. Once transfected, the plasmids assume a nucleosomal chromatin organization as evidenced, for example, by the high enrichment of the roX2-bearing plasmid in acetylated H4K16, as shown by chromatin immunoprecipitation.Citation13
Topo II but not Topo I depletion affects dosage compensation
We used the plasmid system to determine whether the two major topoisomerases present in Drosophila play a role in dosage compensation. We reduced the level of Topo I by double-stranded RNA-mediated interference (Fig. S1A) and determined whether the enhancement of the relative Firefly luciferase expression on the roX-bearing plasmid in relation to the control plasmid was affected. The results did not indicate an involvement of Topo I in dosage compensation (Fig. S1B).
We treated cells with sub-cytotoxic levels of ICRF-193, an inhibitor of Topo II ATPase activity that caused the cells to arrest. In order to control for indirect effects, we treated cells separately with Paclitaxel, a mitotic spindle poison that similarly causes cell-cycle arrest. The luciferase assay revealed that the Topo II inhibitor has a specific negative effect on dosage compensation (). We also reduced the level of Topo II by double-stranded RNA-mediated interference (Fig. S1C) and observed a significant decrease in the expression of the dosage compensated luciferase gene (). In order to exclude indirect effects on the expression of the MSL genes, we measured their relative levels by western blot analysis and qRT-PCR (Fig. S2A and B), and the ability of the complex to form in absence of Topo II was determined by immunoprecipitation (Fig. S2C). These results indicate that Topo II is not required for the stability of the MSL proteins or for the formation of the complex.
Figure 1. Topo II is required for dosage compensation. (A) Ratios of the relative luciferase activity in cells transfected with a roX-bearing plasmid and cells transfected with a control plasmid; the cells were treated with either ICRF-193 (ratio = 1.23) or Paclitaxel (ratio = 1.69). A ratio of approximately 1.7 and above is considered to represent full dosage compensation.Citation13 The results are the average of three independent biological replicates. (B) RNAi of Topo II causes a reduction in the luciferase activity of the roX-bearing (dosage compensated) plasmid relative to the control plasmid (ratio = 1.34) while in the presence of GFP RNAi, the luciferase activity of the roX plasmid relative to the control plasmid (ratio = 2.27) indicates normal dosage compensation. The results are the average of four independent biological replicates. (C) Immunofluorescence staining of MSL2 on polytene chromosomes of top2 mutant larvae. In presumptive males, glands presented nuclei with no staining (not shown), with staining limited to and spread along the X chromosome (left panel) and staining at numerous X chromosomal and autosomal sites (right panel).
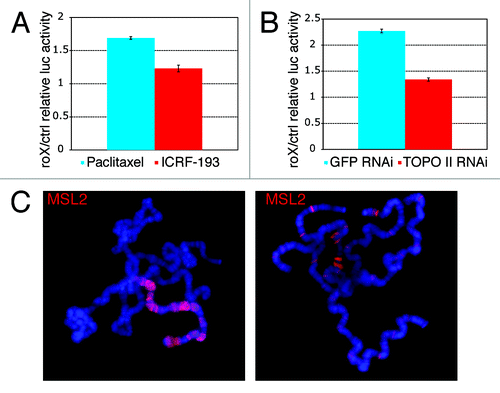
The association of the MSL complex with the X chromosome is partially affected by the absence of Topo II
Previous observations in males heterozygous for partial loss of function alleles and a deficiency for the top2 locus did not reveal a problem in the distribution of the MSL complex along the X chromosome.Citation23 We investigated the formal possibility that the complete abrogation of dosage compensation by Topo II depletion may be the result of a failure of the MSL complex to associate with the X chromosome. Loss-of-function mutations in top2, the gene that encodes Topo II, lead to recessive lethality during embryonic and larval development.Citation16 Third-instar larval escapers exhibit numerous developmental abnormalities consisting mostly of a failure in the formation of complete internal organs and cannot be sexed reliably on the basis of their gonads. We examined the distribution of the MSL complex by immunofluorescence staining of salivary glands. A number of larvae completely lacked any evidence of immunostaining and were considered to be females. The glands of larvae that exhibited staining contained mixtures of unstained nuclei, of nuclei with staining at a number of discrete X chromosome and autosomal sites, and of nuclei with the X chromosome exclusively stained along most of its length (). Different larvae presented different proportions of these three types of nuclei (Table S1). At this level of resolution it is not possible to determine if the distribution of the MSL complex is normal in those nuclei where it is located predominantly on the X-chromosome. Although the preponderance of nuclei in male glands exhibits X-chromosome staining, the lack of this enzyme has a clear but small effect on the frequency of this association.
The effect of Topo II depletion on the expression on X-linked genes is masked by its general effect on global transcription
We monitored the effect of RNAi-mediated depletion of Topo II on the enhancement in the transcription levels of a sample of endogenous X-linked and autosomal genes by quantitative real-time PCR (Fig. S3A). Gene expression of three housekeeping genes (Gpdh, α-tubulin and Pka-C1) was used to normalize the results (Fig. S3B). We observed a substantial level of variability in the response to Topo II knockdown, ranging from significant downregulation to slight overexpression. In contrast to the results obtained with the plasmid system, the transcription level of X-linked genes was not preferentially affected. Possible explanations for this prima facie surprising result are presented in the discussion. The absence of Topo I had no effect on the expression of a subset of X-linked and autosomal genes (Fig. S3C).
Topo II is enriched on dosage compensated genes
We performed a chromatin immunoprecipitation (ChIP) assay with Topo II antiserum in S2 cells and hybridized the resulting DNA to a Drosophila melanogaster whole genome-tiling array. We used RNA-seq data from GEO data set GSE16344 for S2 cells to derive a list of expressed X-linked and autosomal genes. S2 cells are tetraploid (they have two X chromosomes and four sets of autosomes, or 2X;4A) and are known to exhibit some level of segmental aneuploidy. We used the data of Zhang et al.Citation18 to remove genes with aberrant copy numbers from the gene list. This process yielded 1400 X-linked and 5154 autosomal genes with RPKM (Reads Per Kilobase of exon model per Million mapped reads) expression levels above 5. At the genome level, the analysis of Topo II distribution in the two sets of genes revealed that the enzyme is present over gene bodies and is specifically enriched on the X-linked genes (). Such enrichment is absent in Kc cells, a line with female cellular characteristics (Fig. S4). We asked whether the higher concentration of Topo II on dosage compensated genes is simply a reflection of the increased level of transcription of these genes. From the above data set, we selected subsets of X-linked (n = 70) and autosomal (n = 96) genes comparable in terms of expression level. Taking into account the 1:2 difference in the dosage of X-linked and autosomal genes (2:4 difference in S2 cells since they are tetraploid), we compared X-linked genes with an average RPKM of 100–200 to autosomal genes with an average RPKM of 200–400. As can be seen in , the Topo II level is higher on X-linked genes. These observations lead to the important conclusion that the high level of Topo II present on dosage compensated genes seen in is greater than expected on the basis that compensated genes exhibit an enhancement of transcription in the presence of the MSL complex. Rather, the excess of Topo II on these genes must be correlated with some characteristic of the chromatin of compensated genes that allows the enhancement of transcription responsible for dosage compensation.
Figure 2. Topo II distribution is enriched on the compensated X chromosome in S2 cells. (A) Metagene with average ChIP-chip ratio of Topo II distribution over X-linked and autosomal genes. All gene bodies are normalized to a 1 kb scale with 5′ indicating the transcription start site (TSS) and 3′ indicating the transcription termination site (TTS). Topo II has a higher average density on X-linked genes compared with autosomal genes. (B) Metagene profile of Topo II enrichment on autosomal and X-linked genes with equal expression rates per gene copy. Single copy X-linked genes (n = 70) with average RPKM of 100–200 were compared with 96 two-copy autosomal genes with average RPKM levels of 200–400. Higher Topo II density is detected on the X-linked vs. the autosomal genes.
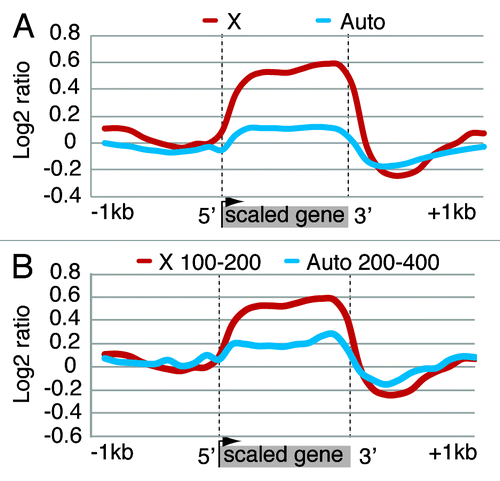
The distribution of Topo II is affected at the MSL chromatin entry sites
An ancillary observation derived from the visual examination of the Topo II genomic mapping results was the unexpected decrease of this enzyme on the roX1 and roX2 genes (Fig. S5). These X-linked genes are transcribed only in males and encode two long non-coding RNAs that are an integral part of the MSL complex.Citation24,Citation25 A DNaseI hypersensitive site is present within roX1 and immediately 3′ of roX2, and the association of the MSL complex at these sites appears to induce roX transcription in males.Citation26 Because the roX RNAs are degraded unless they associate with the proteins of the MSL complex, assembly of the complex must occur concurrently with the synthesis of these RNAs. From the roX gene loci, the complexes are thought to spread to a number of sites along the X chromosome called chromatin entry sites (CES) or high affinity sites (HAS).Citation19,Citation20 We wished to determine whether the distribution of Topo II at these sites resembled its distribution on the roX genes. To this end, we used the regions identified as CESs by Alekseyenko et al.,Citation19 and determined the Topo II distribution around the peaks of the entry sites that correspond to the point of highest enrichment of the MSL complex within the CES. The analysis showed that Topo II is absent at the peak of MSL binding and that it progressively increases on either side (). To determine if the genomic location of the CESs influenced the Topo II distribution, we performed the analysis on a subset of entry sites localized within active genes and observed a substantial correlation with the previous results ( and Table S2). We also verified that Topo II has a similar distribution over the entry sites (HAS) reported by Straub and colleaguesCitation20 ().
Figure 3. The distribution of Topo II at chromatin entry sites (CESs). (A) Topo II is depleted at the peak of entry sites identified by Alekseyenko et al.Citation19 while its presence is increased on either side of the peak. Entry sites within genes exhibit a similar pattern with sharper increases of Topo II distribution away from the CES peak. Random genomic sites show the background level of Topo II across the genome. (B) Comparison of Topo II distribution at independently identified CES sites.Citation20
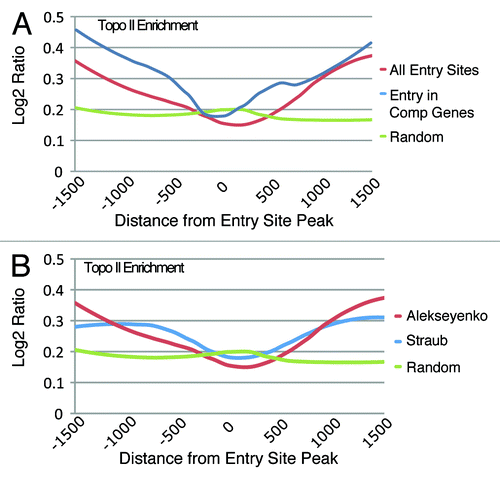
The MSL complex recruits Topo II to the X chromosome
To determine whether it is the MSL complex that recruits Topo II throughout dosage compensated transcriptional units we performed co-immunoprecipitation assays in S2 cells expressing Flag-tagged wild type MLE. In mammalian cells, the MLE ortholog RNA helicase A (RHA) has been shown to interact with Topo II in an RNA-dependent manner.Citation27 We found that Topo II, as well as MSL1, co-precipitate with MLE, while both proteins are absent or present at a background level in the mock IP performed with S2 cell extracts containing only the FLAG-peptide (). RNase treatment of the extract eliminates the presence of Topo II and of MSL1 in the precipitated fraction. The absence of MSL1 is explained by the long-standing observation that RNase treatment releases MLE from the MSL complex.Citation28 In order to determine whether the TOPO II-MLE association occurs when MLE is present in the MSL complex, we used an S2 cell line expressing both flag-tagged MSL3 and HA-tagged MSL2 proteins. We determined that Topo II as well as MLE and MSL1 co-immunoprecipitated with MSL3 (). The presence of Topo II in the precipitate was abolished by treatment of the cellular extract with RNase; MSL1 was still present because it interacts directly with MSL3.Citation29 Topo II was also recovered with the complex after FLAG-peptide elution of the beads (Fig. S6A). These results rule out the possibility of a spurious binding to the beads or to the FLAG tag and clearly show that Topo II is a specific interactor of the MSL complex. Using a cell line that expresses only FLAG-tagged MSL3 (), we observed that Topo II and MLE are less abundant in the precipitated fraction in comparison to the experiment performed with cells overexpressing both MSL3 and MSL2. The difference may reflect a difference in the amount of full MSL complex formed in the two cell lines. As the level of Topo II seems to be correlated with the level of MLE in the MSL precipitate, we knocked down MLE by RNAi in cells expressing MSL3-FLAG and we observed a dramatic reduction in Topo II binding ( and S6B). In order to determine whether MLE can associate with Topo II separately from the MSL complex, we immunoprecipitated flag-MLE from S2 cells in which MSL2 was depleted by RNAi (). As can be seen in the input, MSL1 becomes unstable in the absence of MSL2Citation30 and is barely detectable in the precipitate while Topo II binding is not affected. All these observations strongly indicate that the association of Topo II with the MSL complex occurs through MLE, and is not mediated by any of the other components of the complex.
Figure 4. Topo II interacts with the MSL complex through MLE. (A) Anti-FLAG-MLE IP from S2 cells. MLE binds Topo II and MSL1 in an RNA-dependent manner. (B,C) Anti-FLAG-MSL3 IP from S2 cells expressing MLS3-FLAG and MSL2-HA. MSL3 binds Topo II in an RNA-dependent manner, MLE binding is reduced after RNase treatment while MSL1 and MSL2 remains unchanged. (D,E) anti-FLAG MSL3 IP from S2 cells overexpressing only MSL3-FLAG. (D) MSL3 binding of TopoII and MLE is less efficient compare with figure B and C.(E) MSL3 binding to Topo II is strongly reduced after MLE RNAi. (F) anti-FLAG-MLE IP of MSL2 depleted S2 cells. MLE interacts with Topo II in absence of the MSL complex. (G) Anti-FLAG-MLE IP from roX2-depleted S2 cells. roX2 RNA is not required for the interaction with Topo II and with MSL1. The immunoprecipitated FLAG-MLE is stained with Coomassie. The mock samples are untransfected S2 cells extracts containing the FLAG peptide.
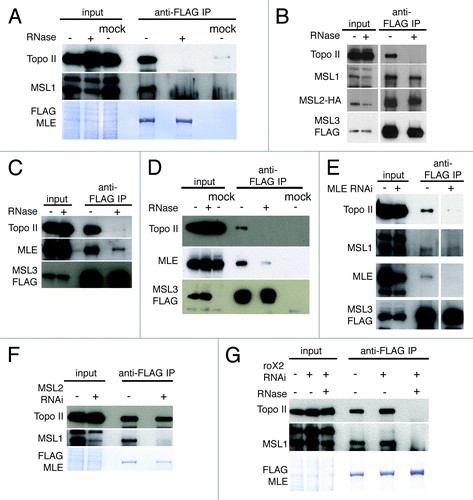
We attempted to determine the nature of the RNA involved in the association between Topo II and the MSL complex. Extensive RT-PCR measurements revealed that only roX2 and not roX1 RNA is present in our S2 cell lines. We used RNAi to successfully eliminate the presence of the roX2 transcript in cells that expressed the Flag-tagged MLE protein (Fig. S7A). Immunoprecipitation with a Flag antiserum revealed the presence of MSL1 and of Topo II. As previously observed, Topo II and MSL1 were no longer present following treatment of the extract with RNase (). The presence of an MSL complex in the absence of both types of roX RNAs has been reported by Deng and Meller.Citation31 Although it is not known whether such complexes subsume a different RNA to facilitate their assembly, this observation is concordant with our results suggesting that Topo II associates with the MSL complex via some other RNA. Recently, a role in dosage compensation for the siRNA pathway has been reported.Citation32 Therefore we decided to check for a potential function of this process in the MLE-Topo II interaction. Dicer 2 knockdown, either in the presence or in the absence of roX2 RNA, does not perturb this interaction (Figs. S7B and C). Despite these efforts, the nature of the RNA remains unresolved.
Unique architectural features of compensated chromatin
The occurrence of dosage compensation on a circular plasmid transfected into S2 cellsCitation13 allows us to investigate effects of chromatin modifications that may underlie the mechanism of transcriptional enhancement.Citation14 Following transfection, and RNA interference when appropriate, chromatin is extracted and subjected to electrophoresis in a gel containing chloroquine. The Firefly luciferase plasmid is visualized by Southern hybridization. Using this approach, we have compared the topoisomer distributions of plasmids extracted from cells where the MSL complex forms and, therefore, where the reporter gene on the plasmid is dosage compensated, with plasmids extracted from cells where the complex was knocked down by targeting MSL2 with RNA interference (non-compensated or “reference” plasmids). Knock down, measured by qRT-PCR, resulted in a reduction of 85% in the MSL2 RNA level. The presence of the MSL complex alters the mobility of the topoisomer distribution of the plasmid by a level equivalent to a difference in linking number of 1.01 ± 0.14 (p = 0.0003), with the compensated plasmid running closer to the bottom of the gel. At the chloroquine concentrations used, all negative supercoils present in the plasmids at the time of chromatin extraction are relaxed and positive supercoils are introduced (Fig. S8A). Therefore, the more rapid migration of the compensated plasmid topoisomer distribution in comparison to the distribution of the non-compensated plasmid indicates that the compensated plasmid is less negatively supercoiled in its native state. An example of these results is presented in and the rationale for interpreting the results is presented in .
Figure 5. Dosage compensation alters plasmid architecture. (A) Topoisomer distributions of plasmids extracted from S2 cells where the MSL complex is present (GFP RNAi) or absent (MSL2 RNAi). At the chloroquine concentration used, the plasmids run as positively supercoiled. The compensated plasmid migrates faster than the non-compensated one, indicating that at the time of extraction the compensated plasmid had fewer negative supercoils. The arrowhead indicates the nicked form of the plasmids. (B) Diagram illustrating the effect of chloroquine concentration on the electrophoretic migration of topoisomers. The intercalation of chloroquine molecules into negatively supercoiled DNA unwinds the double strands and relaxes the negative supercoils. As the chloroquine concentration increases, a negatively supercoiled plasmid becomes relaxed and then positively supercoiled. At a specific, high chloroquine concentration, a plasmid that was less negatively supercoiled will become more positively supercoiled and migrate faster in a gel. (C) Diagrammatic example of the electrophoretic behavior of plasmids differing in their initial level of negative supercoiling in a gel with a fixed high concentration of chloroquine.
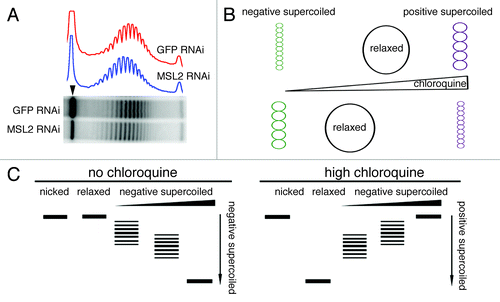
In order to establish that the decrease in negative supercoiling of the roX-bearing plasmid extracted from S2 cells treated with anti GFP double-stranded RNA is directly correlated with the dosage compensation of that plasmid, we analyzed the topoisomer distribution of a plasmid with the roX sequence replaced by an intronic sequence from the human Nesprin gene.Citation13 Such a plasmid is unable to recruit the MSL complex and, therefore, cannot be dosage compensated. We observed no difference in the topoisomer distribution of the Nesprin-bearing plasmids extracted from cells treated with GFP dsRNA (active MSL complex) and from cells treated with MSL2 dsRNA (ΔLK = 0.12 ± 0.16, p = 0.2) (Fig. S8B).
To determine whether the linking number difference is due to the fact that the dosage compensated plasmid transcribes at approximately twice the rate as the non-compensated plasmid, we made use of the observation that the Firefly luciferase reporter gene on the plasmid is under the control of the bacterial tetracycline resistance operon promoter (tetO); this promoter is induced by co-transfecting the cells with a plasmid that expresses the tTA synthetic activator.Citation33 By adjusting the concentration of the tTA-expressing plasmid, the same level of transcription between compensated and non-compensated plasmids can be obtained as shown by Yokoyama et al.Citation13 Equalizing the level of transcription between the compensated and non-compensated plasmids, does not alter the difference in topoisomers distribution between them (ΔLK = 0.98 ± 0.31, p = 0.003) (Fig. S8C). This indicates that the change in linking number between the control and compensated plasmids is not the result of the enhanced transcription due to dosage compensation; rather, it reflects topological modifications unique to compensated chromatin.
The formal possibility exists that, although the tTA transactivator appears to induce a graded transcriptional response,Citation34,Citation35 doubling the level of tTA may activate more copies of the non-compensated plasmid rather than enhance its rate of transcription. If this were the case, the difference in topoisomers distribution between compensated and control plasmids could still be explained by the difference in their respective rates of transcription. We compared the topoisomer distribution of the roX-bearing plasmid in S2 cells at different tTA concentrations: 1.0 ng ptTA (1X), 2.0 ng ptTA (2X) or 30.0 ng ptTA plasmid (30X) for 5x106 cells (Figs. S8D and E). We observed similar topoisomer distributions in plasmids exposed to 1X and 2X tTA concentrations (ΔLK = 0.03 ± 0.34, p = 0.45) or in plasmids exposed to 1X and 30X tTA (ΔLK = 0.3 ± 0.24, p = 0.08). These results indicate that transcription does not per se affect the topology of the compensated plasmid.
The difference in linking number between compensated and non-compensated plasmids is small (ΔLK ~1). It can represent a random, albeit modest relaxation of the nucleosome-DNA association along the length of the compensated plasmid or it can result from the actual loss of one nucleosome. This latter possibility can be rationalized by the existence of a DNase hypersensitive site within the roX2 gene, which is manifest in vivo when the roX gene is present on a compensated X chromosome, i.e., in males.Citation36 To establish directly if a hypersensitive site forms on the compensated roX-plasmid, DNA isolated from nuclei, either treated or not with MNase, was analyzed by quantitative real-time PCR to determine the extent of protection in the presence (GFP RNAi) or absence (MSL2 RNAi) of the MSL complex. The MNase treatment allowed us to amplify plasmid fragments of 271 base pairs and we compared the sensitivity to MNase digestion of the 271 base pairs fragment that encompasses the entire DNase hypersensitive site, to segments of the same length located downstream of the site or at the boundary between the roX2 sequence and the Firefly gene (). The results of these experiments indicate that all three regions are more sensitive to MNase in the presence of the MSL complex than in its absence, in agreement with a more open chromatin structure correlated with dosage compensation. We also find that the three segments are equally sensitive to MNase digestion, (Kolmogorov-Smirnov test: DHS vs neighboring region p value = 0.9; DHS vs FF-roX2 boundary p value = 1.0) suggesting that the region where the roX2 DHS forms on the X chromosome is not particularly exposed in the plasmid. We also tested the MNase sensitivity of this region using smaller amplicons of 191 base pairs and 121 base pairs compared with segments of comparable length, with similar results (; Kolmogorov-Smirnov test: DHS 191 vs neighboring region 188 p value = 0.36; DHS 121vs neighboring region 110 p value = 0.87).
Figure 6. The decrease in negative supercoils is not dependent on the presence of the DHS. (A) Top: schematic representation of the roX2 sequence organization in the Firefly plasmid. The red box indicates the 110 bp MSL binding site around which the DHS is formed, the black lines above indicate the fragments amplified after MNase digestion. The histograms represent the relative level of protection of the DHS containing region in a compensated vs. a non-compensated plasmid. Left panel: Four separate experiments comparing the level of protection in the DHS-containing region and a region near the Firefly/roX2 boundary. Right panel: Five separate experiments comparing the level of protection in the DHS-containing region and an adjacent region. (B) Five separate experiments comparing the level of protection in the 191 bp DHS-containing region and the 188 bp neighboring region. (C) Five separate experiments comparing the level of protection in the 121 bp DHS-containing region and the 110 bp neighboring region.
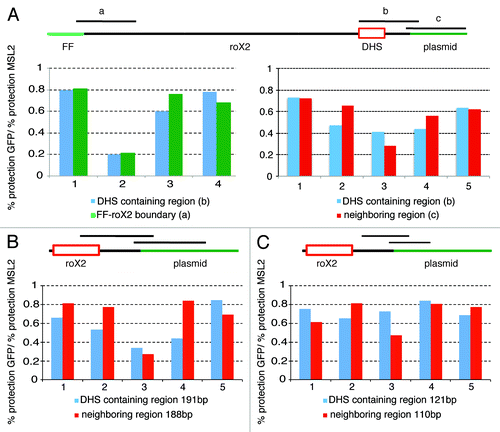
The observed difference in topoisomers distributions reported above highlights a difference between the dosage compensated and the reference plasmids in the number of negative supercoils present at the moment of chromatin isolation. In order to determine specifically the topological status of the Firefly luciferase gene in these two plasmids, we used a psoralen photobinding assayCitation22 (Fig. S9). Briefly, transfected cells are exposed to psoralen, which intercalates into DNA with high affinity for unconstrained negative supercoils and can be cross-linked to its site of insertion using UV light (Fig. S10). The DNA is extracted and a linear fragment containing the Firefly luciferase gene is generated by restriction enzyme treatment. Following denaturation and Southern blot analysis, the amount of cross-links per Kb in the fragments derived from the compensated and reference plasmids is determined and normalized to the level of cross-links per Kb in similar fragments derived from plasmids that have been relaxed in situ by nicking prior to psoralen exposure (Fig. S11). Any difference between the ratios represents a difference in the level of unconstrained negative supercoils. The results presented in clearly show that a higher relative psoralen incorporation occurred in the non-dosage compensated plasmid indicating that the compensated plasmid DNA presented fewer negative supercoils. These results are fully consistent with those obtained by analyzing topoisomer distributions.
Figure 7. (A) Southern blot analysis of psoralen cross-linking in the firefly luciferase gene. S2 cells transfected with the roX2-bearing plasmid were incubated with psoralen and UV cross-linked. In half of the cells the DNA is relaxed by nicking prior to psoralen treatment. The extracted DNA was digested and denatured with glyoxal before Southern blot analysis. The cross-linked DNA runs as double-stranded (XL) while the non cross-linked DNA runs as single-stranded (NXL); their quantification is used to determine the rate of cross-linking per Kb of DNA (XL/Kb). The experiments were done in presence (GFP RNAi) or absence of the MSL complex (MSL2 RNAi). (B) Relative psoralen incorporation ratio. The portion of psoralen binding due to the presence of negative supercoils is calculated as XL/Kb in intact cells [(XL/Kb)I] divided by the XL/Kb in nicked cells [(XL/Kb)N]. This ratio reflects the level of supercoiling present; a ratio equal to 1 indicates that the DNA in the intact cells is relaxed, a ratio > 1 indicates that the DNA in the intact cells is negatively supercoiled. (C) The differences in the mean linking number (ΔLk) of topoisomers isolated from S2 cells treated with Topo II RNAi. Control plasmids extracted from cells (treated only with MSL2 double-stranded RNA) are used as the base line. The absence of Topo II leads to a level of positive supercoiling greater in the plasmids extracted from cells containing the MSL complex (Topo II) than in cells where the MSL complex is absent (MSL2+Topo II).
![Figure 7. (A) Southern blot analysis of psoralen cross-linking in the firefly luciferase gene. S2 cells transfected with the roX2-bearing plasmid were incubated with psoralen and UV cross-linked. In half of the cells the DNA is relaxed by nicking prior to psoralen treatment. The extracted DNA was digested and denatured with glyoxal before Southern blot analysis. The cross-linked DNA runs as double-stranded (XL) while the non cross-linked DNA runs as single-stranded (NXL); their quantification is used to determine the rate of cross-linking per Kb of DNA (XL/Kb). The experiments were done in presence (GFP RNAi) or absence of the MSL complex (MSL2 RNAi). (B) Relative psoralen incorporation ratio. The portion of psoralen binding due to the presence of negative supercoils is calculated as XL/Kb in intact cells [(XL/Kb)I] divided by the XL/Kb in nicked cells [(XL/Kb)N]. This ratio reflects the level of supercoiling present; a ratio equal to 1 indicates that the DNA in the intact cells is relaxed, a ratio > 1 indicates that the DNA in the intact cells is negatively supercoiled. (C) The differences in the mean linking number (ΔLk) of topoisomers isolated from S2 cells treated with Topo II RNAi. Control plasmids extracted from cells (treated only with MSL2 double-stranded RNA) are used as the base line. The absence of Topo II leads to a level of positive supercoiling greater in the plasmids extracted from cells containing the MSL complex (Topo II) than in cells where the MSL complex is absent (MSL2+Topo II).](/cms/asset/342700a9-000e-430c-81cb-cc495f749ba0/ktrn_a_10926185_f0007.gif)
Topo II alters the topology of dosage compensated genes independently from its canonical effect on transcription
Cells transfected with roX2 sequence-bearing plasmids, treated with double-stranded MSL2 RNA to prevent the formation of the MSL complex or with GFP RNA, were subjected to RNA interference against Topo II. The topoisomer distributions () in chloroquine gels showed that the knockdown of Topo II in cells where the MSL complex is absent resulted in a faster migration equivalent to a difference in linking number of 1.23 ± 0.26 (p = 0.007). This indicates that, as expected, Topo II affects the topology of a transcribing reference (non-compensated) plasmid. Topo II knockdown in cells where the MSL complex is present yielded a difference in linking number of 1.59 ± 0.15 (p = 0.002) between the plasmid’s topoisomer distribution and that of the reference (non compensated) plasmid. This difference is greater than the difference mediated by Topo II knockdown on the reference plasmid (p = 0.015). In the absence of Topo II, the plasmid extracted from S2 cells where the MSL complex can form is not dosage compensated (see ), yet is presumably targeted by the complex. Therefore, in addition to the topological changes expected from the failure of Topo II to resolve the supercoiling generated by the transcribing polymerase, there are topological changes on the compensated plasmid that are unique to compensated chromatin.
Discussion
We have mapped the genomic distribution of Topo II in S2 cells and found that it is substantially enriched on X-linked genes in comparison to autosomal genes with the same transcriptional levels. It is reasonable to suggest that the Topo II enrichment on dosage compensated genes is not the result of their enhanced transcription and that it reflects topological characteristics or requirements unique to compensated chromatin. This conclusion is reinforced by the abrogation of dosage compensation in the roX-bearing plasmid following Topo II depletion. The absence of a similar effect on endogenous X-linked genes may be explained by one, or a combination, of the following considerations. The plasmid system is subjected to less regulatory noise than genomic genes. Given the role played by Topo II on the expression of most genomic (autosomal as well as X-linked) genes, loss of this enzyme will affect multiple regulatory pathways, masking a specific effect on the modest enhancement of transcription specific to X-linked genes. Furthermore, cells where Topo II has been abrogated by RNA interference stop dividing and eventually senesce and die. At the time of sampling, these cells represent a mixed population impaired in various stages of the cell cycle, and perhaps exhibiting various degrees of DNA replication. In contrast, the plasmids do not replicate. In Topo II depleted cells, the chromatin modifications responsible for dosage compensation might perdure during the RNAi treatment. The plasmids, on the other hand, are introduced, and become constituted into nucleosomal chromatin three days after the treatment with dsRNA was begun.
The enriched presence of Topo II on X-linked genes and its apparent requirement for dosage compensation of the reporter gene in the plasmid system led us to determine the supercoiling state of a plasmid that exhibits the enhanced transcription level resulting from dosage compensation. By adjusting the experimental conditions to induce the compensated and non-compensated plasmids to transcribe at the same rate, we have ruled out that the difference in their topoisomer distributions is the result of the normal increase in transcription due to dosage compensation. Rather, it reflects topological modifications unique to compensated chromatin.
These results were confirmed by measuring the topological differences between compensated plasmids at different concentrations of tTA. The topoisomer distribution of a plasmid sample reflects the topological status of the plasmid population. In the case of a mixed population with a certain proportion of activated and non-activated plasmids, the topoisomer distribution is a melding of the distributions of the two types of plasmids with both types contributing to the center, i.e., to the prevalent topoisomer. If an increase in tTA concentration simply increases the relative proportion of actively transcribing plasmids and if transcription generates a topological change, at a higher tTA concentration, a shift in the position of the center of the distribution should be observed, because a different proportion of activated and non-activated plasmids would contribute to the most prevalent topoisomer. This result was not observed leading to the conclusion that transcription does not per se affect the topology of the compensated plasmid.
The region where a DNase hypersensitive site that normally forms on the roX2 gene in malesCitation36 is included in the roX-bearing plasmid leading to the hypothesis that the difference in topology between dosage compensated and control plasmids is due to a loss of one nucleosome at that site. The common methodology used to determine experimentally whether a difference in nucleosome number exists in a particular region is nucleosome scanning. We could not use this approach because primer pairs internal to the roX2 fragment would also target the genomic roX2 sequence, and primer pairs external to roX2 would lead to the amplification of regions present in the other vectors co-transfected with the Firefly-bearing plasmids. Using amplicons that straddle roX-plasmid or roX-Firefly luciferase junctions, we compared the sensitivity of the DNase-sensitive region in compensated and control plasmids. The results of these experiments did not support the hypothesis that the difference in topology between dosage compensated and control plasmids is due to the presence of a DNase hypersensitive site that occurs in the roX2 sequence when the plasmid is compensated. An additional consideration is that the hypersensitive site associated with the roX2 gene on the X-chromosome is substantially weaker than the roX1 gene site,Citation36 suggesting that even in its endogenous location, the roX2 site may not form every time that it is bound by the complex. The association of the MSL complex with its sites of action is now known to be mediated by the intermediary binding of the CLAMP protein to the chromatin entry sites.Citation37
The difference in linking number between compensated and non-compensated plasmids is small (ΔLK ~1), albeit completely reproducible. The reason for such a small change is presumably due to its correlation with only a 2-fold enhancement in transcription on a plasmid that accommodates 32 nucleosomes. At the chloroquine concentration used in our gels, chosen for optimal display of topoisomer distributions, all negative supercoils are relaxed and positive supercoils are introduced. Therefore, the more rapid migration of the compensated plasmid’s topoisomer distribution in comparison to the distribution of the non-compensated plasmid indicates that the compensated plasmid is less negatively supercoiled in the cells. The in vivo incorporation of psoralen reflects the presence of fewer unconstrained negative supercoils on the compensated reporter gene and confirms the results obtained by comparing topoisomer distributions. Loss of negative supercoils in DNA may result from the release of constrained supercoils by the loss or partial unfolding of nucleosomes. Together, these results indicate a change in the level of the torsional stress in the roX-bearing compensated plasmid.
When Topo II is reduced in S2 cells, the non-dosage compensated plasmid topoisomers migrate faster. This can be explained by the fact that in the absence of Topo II some of the positive supercoils generated by the RNAP II elongating complex remain unresolved. When the MSL complex is present, but Topo II is absent, the transcriptional increase normally mediated by the complex is trumped by the lack of Topo II (); the plasmid is no longer compensated and its transcriptional level is equivalent to that exhibited by a non-compensated reference plasmid in the absence of Topo II. One might expect the distribution of topoisomers of these two plasmids to be the same. In fact, that is not the case, and the presence of the MSL complex induces a greater change in linking number (i.e., a greater level of positive supercoiling) indicating that Topo II not only plays a role in transcription, but also affects a transcription-independent remodeling component uniquely characteristic of compensated chromatin. We suggest that this component is the result of an action of the complex that is normally masked by the presence of Topo II. The complex includes MLE, an ATPase that is distantly related to the ATPases present in all of the complexes that remodel chromatin by altering the positioning or the architecture of nucleosomes. These ATPases have been shown to induce positive supercoils in free DNA or in DNA reconstituted into nucleosomal arrays.Citation38 MLE may have the same effect on the targets of the MSL complex but, under normal cellular conditions, this effect is counteracted by the action of Topo II. When Topo II is reduced, the induction of positive supercoils by the MSL complex increases the mobility of the topoisomer distribution. Witness to the complexity of MLE function is the recent report that it unwinds the stem loop structure of the roX RNAs.Citation39,Citation40
In vitro studies using positively coiled yeast minichromosomes have shown that Topo II remodels nucleosomal DNA more rapidly than Topo I; this observation suggested that in vivo, Topo II contributes to DNA relaxation by reducing the writhe represented by the wrapping of DNA around nucleosomes while Topo I alters the twist of DNA in chromatin regions where DNA is less accessible to Topo II.Citation41 An RNA polymerase complex must unwind the DNA that it is transcribing in order to access the template strand. Movement of the complex, and of the open bubble that it creates toward the 3′ end of the transcription unit, causes the DNA in front of the bubble to become positively supercoiled and the DNA behind it negatively supercoiled. These transitory changes in the superhelical density of the transcription unit are restored to the state that they exhibited prior to the passage of the RNAPII complex by the action of topoisomerases.
Drosophila has only one topoisomerase II and a subset of this enzyme co-immunoprecipitates with supercoiling factor (SCF).Citation11 This factor, first identified in Bombyx mori, was shown to induce the supercoiling of a relaxed circular DNA in the presence of Topo II, leading to the conclusion that DNA supercoiling can occur independently from transcription.Citation12 Reducing the level of SCF preferentially drastically reduces male viability.Citation10 The established functional dependence of SCF on topoisomerase II, led us to determine whether this unwinding enzyme plays a role in dosage compensation that would be unrelated to its function in general transcription. We have shown that Topo II is enriched on dosage compensated genes to a greater extent than on non-compensated genes with similar transcription levels. SCF is predominantly associated with gene promotersCitation42 and its requirement for dosage compensation and male viability may be due to its possible role in RNAPII re-initiation that must be increased in frequency to achieve transcriptional enhancement. Given the predominant association of SCF with promoters, it is unlikely that the Topo II presence along gene bodies is related to SCF function.
The results of co-immunoprecipitation, lead us to propose that the MSL complex recruits Topo II to the X chromosome. This conclusion is supported by the observation that Topo II is the major MSL interactor identified in a ChIP-Mass Spec experiment.Citation43 Further, our results suggest that MLE is the subunit that bridges Topo II to the MSL complex. The association of RNA helicase A (RHA), the mammalian ortholog of MLE, with Topo IIα depends on the presence of an unidentified RNA.Citation27,Citation44 These observations led us to investigate and establish that the association of Topo II with the MSL complex seems to be RNA-dependent as well. As was the case for RHA, we were unable to identify the RNA involved in the MLE interaction.
The absence of Topo II at the chromatin entry sites (CES/HAS) of the complex was surprising. Any explanation of this unexpected distribution of Topo II must accommodate the seemingly contradictory observations that the CES peaks are defined as regions of highest concentration of the MSL complex, and that the MSL complex appears to be responsible for the recruitment of excess Topo II to the X chromosome. A possible interpretation is that the MSL complex recruits Topo II after it moves from the intergenic entry sites to the dosage compensated genes or from intragenic entry sites to other regions of the genes. This interpretation is concordant with the recent observation by Peter Becker and colleagues that, although the MSL complex associates with active X-chromosome transcriptional units through MSL3 and the latter’s affinity for histone H3 methylated at lysine 36,Citation45 it uses its MLE and MSL2 subunits to associate with the high affinity sites.Citation46 The apparent DNA-binding function of MLE at these sites may prevent it from recruiting Topo II.
The high concentration of histone H4K16ac that occurs throughout the length of dosage compensated transcriptional units and the fact that compensated genes exhibit an approximate 2-fold enhancement in levels of product irrespective of the strength of their promoter, have led us to propose that dosage compensation is achieved by increasing the rate of elongation of the transcribing polymerases.Citation47 Supporting evidence for this hypothesis was recently obtained.Citation17 The presence of H4K16ac on dosage compensated genes lessens inter-nucleosomal interactions and, therefore, is believed to facilitate RNAP II elongation by facilitating nucleosomal evictionCitation48-Citation50 or by increasing accessibility for DNA-binding extrinsic proteins.Citation51 We suggest that, in addition to this proposed role of H4K16 acetylation, the enhanced level of Topo II on compensated genes facilitates elongation by altering the DNA supercoiling of these genes: as the transcription elongation complex proceeds along the template, it takes less energy to generate the positive supercoils that occur in front of it if the DNA is less negatively supercoiled. It goes without saying that in order to produce the increase in steady-state level of X-linked gene transcripts necessary for dosage compensation an enhanced rate of elongation of RNAP II must be caused by, or accompanied by, complementary regulatory events. An example could be enhanced processivity of RNAP II leading to a higher frequency of successfully completed transcripts. Another possibility is that, in order to achieve an increase in the steady-state level of X-linked gene transcripts, an increase in the rate of elongation (i.e., an increase in the number of nucleotides added per initiation event) must be coupled to an increase in the frequency of re-initiation. At any time during transcription, the number of RNA polymerases present on dosage compensated genes must be greater than on the same genes under non-compensating conditions (in females or in males where the MSL complex has been abrogated). This, in fact, is the case.Citation52
In conclusion, while previous studies of dosage compensation in Drosophila have focused on histone acetylation, our results provide a new mechanistic insight. Perhaps of equal importance, we may have uncovered an additional role for Topo II during transcription, above and beyond its normal function of restoring the supercoiling of a transcriptional unit in front and behind the processing RNAP II complex.
Additional material
Download Zip (2.5 MB)Acknowledgments
We are very grateful to Dr. Richard Sinden for his invaluable guidance during the course of the psoralen incorporation experiments, and to Dr. M. Rohrbaugh for her help with the polytene chromosomes preparations. We would like to thank Dr. P. Fisher for his gifts of Topo II and lamin Dm0 antibodies, and Dr. Tao-shih Hsieh for his gift of Topo I antibody. SC formulated the original question, performed the experiments with the plasmid system and psoralen incorporation, shared in the preparation of the ChIP material, performed the co-IPs and the gene expression analysis. ER shared in the preparation of the ChIP material and performed the bioinformatics and cytological analyses. HL and RY performed and evaluated the topoisomer analyses. KML performed the analysis of Topo I. JCL supervised the experimental program and the writing of the manuscript. This work was supported by Public Health Service grants CA133106 from the National Cancer Institute to ER, and GM15691 from the National Institute of General Medical Sciences to JCL.
Submitted
07/19/13
Revised
08/15/13
Accepted
08/16/13
Disclosure of Potential Conflicts of Interest
No potential conflicts of interest were disclosed.
References
- Lee CG, Chang KA, Kuroda MI, Hurwitz J. The NTPase/helicase activities of Drosophila maleless, an essential factor in dosage compensation. EMBO J 1997; 16:2671 - 81; http://dx.doi.org/10.1093/emboj/16.10.2671; PMID: 9184214
- Hilfiker A, Hilfiker-Kleiner D, Pannuti A, Lucchesi JC. mof, a putative acetyl transferase gene related to the Tip60 and MOZ human genes and to the SAS genes of yeast, is required for dosage compensation in Drosophila. EMBO J 1997; 16:2054 - 60; http://dx.doi.org/10.1093/emboj/16.8.2054; PMID: 9155031
- Wu L, Zee BM, Wang Y, Garcia BA, Dou Y. The RING finger protein MSL2 in the MOF complex is an E3 ubiquitin ligase for H2B K34 and is involved in crosstalk with H3 K4 and K79 methylation. Mol Cell 2011; 43:132 - 44; http://dx.doi.org/10.1016/j.molcel.2011.05.015; PMID: 21726816
- Gupta V, Parisi M, Sturgill D, Nuttall R, Doctolero M, Dudko OK, Malley JD, Eastman PS, Oliver B. Global analysis of X-chromosome dosage compensation. J Biol 2006; 5:3; http://dx.doi.org/10.1186/jbiol30; PMID: 16507155
- Conrad T, Akhtar A. Dosage compensation in Drosophila melanogaster: epigenetic fine-tuning of chromosome-wide transcription. Nat Rev Genet 2011; 13:123 - 34; http://dx.doi.org/10.1038/nrg3124; PMID: 22251873
- Gelbart ME, Larschan E, Peng S, Park PJ, Kuroda MI. Drosophila MSL complex globally acetylates H4K16 on the male X chromosome for dosage compensation. Nat Struct Mol Biol 2009; 16:825 - 32; http://dx.doi.org/10.1038/nsmb.1644; PMID: 19648925
- Straub T, Becker PB. Transcription modulation chromosome-wide: universal features and principles of dosage compensation in worms and flies. Curr Opin Genet Dev 2011; 21:147 - 53; http://dx.doi.org/10.1016/j.gde.2011.01.012; PMID: 21316939
- Deuring R, Fanti L, Armstrong JA, Sarte M, Papoulas O, Prestel M, Daubresse G, Verardo M, Moseley SL, Berloco M, et al. The ISWI chromatin-remodeling protein is required for gene expression and the maintenance of higher order chromatin structure in vivo. Mol Cell 2000; 5:355 - 65; http://dx.doi.org/10.1016/S1097-2765(00)80430-X; PMID: 10882076
- Lucchesi JC. The structure-function link of compensated chromatin in Drosophila. Curr Opin Genet Dev 2009; 19:550 - 6; http://dx.doi.org/10.1016/j.gde.2009.10.004; PMID: 19880310
- Furuhashi H, Nakajima M, Hirose S. DNA supercoiling factor contributes to dosage compensation in Drosophila. Development 2006; 133:4475 - 83; http://dx.doi.org/10.1242/dev.02620; PMID: 17035293
- Kobayashi M, Aita N, Hayashi S, Okada K, Ohta T, Hirose S. DNA supercoiling factor localizes to puffs on polytene chromosomes in Drosophila melanogaster. Mol Cell Biol 1998; 18:6737 - 44; PMID: 9774687
- Ohta T, Hirose S. Purification of a DNA supercoiling factor from the posterior silk gland of Bombyx mori. Proc Natl Acad Sci U S A 1990; 87:5307 - 11; http://dx.doi.org/10.1073/pnas.87.14.5307; PMID: 2164676
- Yokoyama R, Pannuti A, Ling H, Smith ER, Lucchesi JC. A plasmid model system shows that Drosophila dosage compensation depends on the global acetylation of histone H4 at lysine 16 and is not affected by depletion of common transcription elongation chromatin marks. Mol Cell Biol 2007; 27:7865 - 70; http://dx.doi.org/10.1128/MCB.00397-07; PMID: 17875941
- Dunlap D, Yokoyama R, Ling H, Sun HY, McGill K, Cugusi S, Lucchesi JC. Distinct contributions of MSL complex subunits to the transcriptional enhancement responsible for dosage compensation in Drosophila. Nucleic Acids Res 2012; 40:11281 - 91; http://dx.doi.org/10.1093/nar/gks890; PMID: 23047951
- Bushey AM, Ramos E, Corces VG. Three subclasses of a Drosophila insulator show distinct and cell type-specific genomic distributions. Genes Dev 2009; 23:1338 - 50; http://dx.doi.org/10.1101/gad.1798209; PMID: 19443682
- Ramos E, Torre EA, Bushey AM, Gurudatta BV, Corces VG. DNA topoisomerase II modulates insulator function in Drosophila. PLoS One 2011; 6:e16562; http://dx.doi.org/10.1371/journal.pone.0016562; PMID: 21304601
- Larschan E, Bishop EP, Kharchenko PV, Core LJ, Lis JT, Park PJ, Kuroda MI. X chromosome dosage compensation via enhanced transcriptional elongation in Drosophila. Nature 2011; 471:115 - 8; http://dx.doi.org/10.1038/nature09757; PMID: 21368835
- Zhang Y, Malone JH, Powell SK, Periwal V, Spana E, Macalpine DM, Oliver B. Expression in aneuploid Drosophila S2 cells. PLoS Biol 2010; 8:e1000320; http://dx.doi.org/10.1371/journal.pbio.1000320; PMID: 20186269
- Alekseyenko AA, Peng S, Larschan E, Gorchakov AA, Lee OK, Kharchenko P, McGrath SD, Wang CI, Mardis ER, Park PJ, et al. A sequence motif within chromatin entry sites directs MSL establishment on the Drosophila X chromosome. Cell 2008; 134:599 - 609; http://dx.doi.org/10.1016/j.cell.2008.06.033; PMID: 18724933
- Straub T, Grimaud C, Gilfillan GD, Mitterweger A, Becker PB. The chromosomal high-affinity binding sites for the Drosophila dosage compensation complex. PLoS Genet 2008; 4:e1000302; http://dx.doi.org/10.1371/journal.pgen.1000302; PMID: 19079572
- Morse RH. Analysis of DNA topology in yeast chromatin. Methods Mol Biol 2009; 523:93 - 108; http://dx.doi.org/10.1007/978-1-59745-190-1_7; PMID: 19381919
- Sinden RR, Bat O, Kramer PR. Psoralen cross-linking as probe of torsional tension and topological domain size in vivo. Methods 1999; 17:112 - 24; http://dx.doi.org/10.1006/meth.1998.0723; PMID: 10075890
- Hohl AM, Thompson M, Soshnev AA, Wu J, Morris J, Hsieh TS, Wu CT, Geyer PK. Restoration of topoisomerase 2 function by complementation of defective monomers in Drosophila. Genetics 2012; 192:843 - 56; http://dx.doi.org/10.1534/genetics.112.144006; PMID: 22923380
- Amrein H, Axel R. Genes expressed in neurons of adult male Drosophila. Cell 1997; 88:459 - 69; http://dx.doi.org/10.1016/S0092-8674(00)81886-3; PMID: 9038337
- Meller VH, Wu KH, Roman G, Kuroda MI, Davis RL. roX1 RNA paints the X chromosome of male Drosophila and is regulated by the dosage compensation system. Cell 1997; 88:445 - 57; http://dx.doi.org/10.1016/S0092-8674(00)81885-1; PMID: 9038336
- Bai X, Alekseyenko AA, Kuroda MI. Sequence-specific targeting of MSL complex regulates transcription of the roX RNA genes. EMBO J 2004; 23:2853 - 61; http://dx.doi.org/10.1038/sj.emboj.7600299; PMID: 15229655
- Zhou K, Choe KT, Zaidi Z, Wang Q, Mathews MB, Lee CG. RNA helicase A interacts with dsDNA and topoisomerase IIalpha. Nucleic Acids Res 2003; 31:2253 - 60; http://dx.doi.org/10.1093/nar/gkg328; PMID: 12711669
- Richter L, Bone JR, Kuroda MI. RNA-dependent association of the Drosophila maleless protein with the male X chromosome. Genes Cells 1996; 1:325 - 36; http://dx.doi.org/10.1046/j.1365-2443.1996.26027.x; PMID: 9133666
- Morales V, Regnard C, Izzo A, Vetter I, Becker PB. The MRG domain mediates the functional integration of MSL3 into the dosage compensation complex. Mol Cell Biol 2005; 25:5947 - 54; http://dx.doi.org/10.1128/MCB.25.14.5947-5954.2005; PMID: 15988010
- Lyman LM, Copps K, Rastelli L, Kelley RL, Kuroda MI. Drosophila male-specific lethal-2 protein: structure/function analysis and dependence on MSL-1 for chromosome association. Genetics 1997; 147:1743 - 53; PMID: 9409833
- Deng X, Meller VH. roX RNAs are required for increased expression of X-linked genes in Drosophila melanogaster males. Genetics 2006; 174:1859 - 66; http://dx.doi.org/10.1534/genetics.106.064568; PMID: 17028315
- Menon DU, Meller VH. A role for siRNA in X-chromosome dosage compensation in Drosophila melanogaster. Genetics 2012; 191:1023 - 8; http://dx.doi.org/10.1534/genetics.112.140236; PMID: 22554892
- Gossen M, Bujard H. Tight control of gene expression in mammalian cells by tetracycline-responsive promoters. Proc Natl Acad Sci U S A 1992; 89:5547 - 51; http://dx.doi.org/10.1073/pnas.89.12.5547; PMID: 1319065
- Kringstein AM, Rossi FM, Hofmann A, Blau HM. Graded transcriptional response to different concentrations of a single transactivator. Proc Natl Acad Sci U S A 1998; 95:13670 - 5; http://dx.doi.org/10.1073/pnas.95.23.13670; PMID: 9811858
- Lee TH, Maheshri N. A regulatory role for repeated decoy transcription factor binding sites in target gene expression. Mol Syst Biol 2012; 8:576; http://dx.doi.org/10.1038/msb.2012.7; PMID: 22453733
- Park Y, Mengus G, Bai X, Kageyama Y, Meller VH, Becker PB, Kuroda MI. Sequence-specific targeting of Drosophila roX genes by the MSL dosage compensation complex. Mol Cell 2003; 11:977 - 86; http://dx.doi.org/10.1016/S1097-2765(03)00147-3; PMID: 12718883
- Soruco MM, Chery J, Bishop EP, Siggers T, Tolstorukov MY, Leydon AR, Sugden AU, Goebel K, Feng J, Xia P, et al. The CLAMP protein links the MSL complex to the X chromosome during Drosophila dosage compensation. Genes Dev 2013; 27:1551 - 6; http://dx.doi.org/10.1101/gad.214585.113; PMID: 23873939
- Havas K, Flaus A, Phelan M, Kingston R, Wade PA, Lilley DM, Owen-Hughes T. Generation of superhelical torsion by ATP-dependent chromatin remodeling activities. Cell 2000; 103:1133 - 42; http://dx.doi.org/10.1016/S0092-8674(00)00215-4; PMID: 11163188
- Maenner S, Müller M, Fröhlich J, Langer D, Becker PB. ATP-Dependent roX RNA Remodeling by the Helicase maleless Enables Specific Association of MSL Proteins. Mol Cell 2013; 51:174 - 84; http://dx.doi.org/10.1016/j.molcel.2013.06.011; PMID: 23870143
- Ilik IA, Quinn JJ, Georgiev P, Tavares-Cadete F, Maticzka D, Toscano S, Wan Y, Spitale RC, Luscombe N, Backofen R, et al. Tandem Stem-Loops in roX RNAs Act Together to Mediate X Chromosome Dosage Compensation in Drosophila. Mol Cell 2013; 51:156 - 73; http://dx.doi.org/10.1016/j.molcel.2013.07.001; PMID: 23870142
- Salceda J, Fernández X, Roca J. Topoisomerase II, not topoisomerase I, is the proficient relaxase of nucleosomal DNA. EMBO J 2006; 25:2575 - 83; http://dx.doi.org/10.1038/sj.emboj.7601142; PMID: 16710299
- Ogasawara Y, Furuhashi H, Hirose S. DNA supercoiling factor positively regulates expression of the homeotic gene Abdominal-B in Drosophila melanogaster. Genes Cells 2007; 12:1347 - 55; http://dx.doi.org/10.1111/j.1365-2443.2007.01140.x; PMID: 18076572
- Wang CI, Alekseyenko AA, LeRoy G, Elia AE, Gorchakov AA, Britton LM, Elledge SJ, Kharchenko PV, Garcia BA, Kuroda MI. Chromatin proteins captured by ChIP-mass spectrometry are linked to dosage compensation in Drosophila. Nat Struct Mol Biol 2013; 20:202 - 9; http://dx.doi.org/10.1038/nsmb.2477; PMID: 23295261
- Park SW, Parrott AM, Fritz DT, Park Y, Mathews MB, Lee CG. Regulation of the catalytic function of topoisomerase II alpha through association with RNA. Nucleic Acids Res 2008; 36:6080 - 90; http://dx.doi.org/10.1093/nar/gkn614; PMID: 18820297
- Larschan E, Alekseyenko AA, Gortchakov AA, Peng S, Li B, Yang P, Workman JL, Park PJ, Kuroda MI. MSL complex is attracted to genes marked by H3K36 trimethylation using a sequence-independent mechanism. Mol Cell 2007; 28:121 - 33; http://dx.doi.org/10.1016/j.molcel.2007.08.011; PMID: 17936709
- Straub T, Zabel A, Gilfillan GD, Feller C, Becker PB. Different chromatin interfaces of the Drosophila dosage compensation complex revealed by high-shear ChIP-seq. Genome Res 2013; 23:473 - 85; http://dx.doi.org/10.1101/gr.146407.112; PMID: 23233545
- Smith ER, Allis CD, Lucchesi JC. Linking global histone acetylation to the transcription enhancement of X-chromosomal genes in Drosophila males. J Biol Chem 2001; 276:31483 - 6; http://dx.doi.org/10.1074/jbc.C100351200; PMID: 11445559
- Shogren-Knaak M, Ishii H, Sun JM, Pazin MJ, Davie JR, Peterson CL. Histone H4-K16 acetylation controls chromatin structure and protein interactions. Science 2006; 311:844 - 7; http://dx.doi.org/10.1126/science.1124000; PMID: 16469925
- Robinson PJ, An W, Routh A, Martino F, Chapman L, Roeder RG, Rhodes D. 30 nm chromatin fibre decompaction requires both H4-K16 acetylation and linker histone eviction. J Mol Biol 2008; 381:816 - 25; http://dx.doi.org/10.1016/j.jmb.2008.04.050; PMID: 18653199
- Liu Y, Lu C, Yang Y, Fan Y, Yang R, Liu CF, Korolev N, Nordenskiöld L. Influence of histone tails and H4 tail acetylations on nucleosome-nucleosome interactions. J Mol Biol 2011; 414:749 - 64; http://dx.doi.org/10.1016/j.jmb.2011.10.031; PMID: 22051513
- Bell O, Schwaiger M, Oakeley EJ, Lienert F, Beisel C, Stadler MB, Schübeler D. Accessibility of the Drosophila genome discriminates PcG repression, H4K16 acetylation and replication timing. Nat Struct Mol Biol 2010; 17:894 - 900; http://dx.doi.org/10.1038/nsmb.1825; PMID: 20562853
- Conrad T, Cavalli FM, Vaquerizas JM, Luscombe NM, Akhtar A. Drosophila dosage compensation involves enhanced Pol II recruitment to male X-linked promoters. Science 2012; 337:742 - 6; http://dx.doi.org/10.1126/science.1221428; PMID: 22821985