Abstract
Staphylococcus aureus is a leading cause of invasive infection. It also infects wet mucosal tissues including the cornea and conjunctiva. Conflicting evidence exists on the expression of Toll-like receptors by human corneal epithelial cells. It was therefore of interest to determine how epithelial cells from this immune privileged tissue respond to S. aureus. Further, it was of interest to determine whether cytolytic toxins, with the potential to cause ion flux or potentially permit effector molecule movement across the target cell membrane, alter the response. Microarrays were used to globally assess the response of human corneal epithelial cells to S. aureus. A large increase in abundance of transcripts encoding the antimicrobial dendritic cell chemokine, CCL20, was observed. CCL20 release into the medium was detected, and this response was found to be largely TLR2 and NOD2 independent. Corneal epithelial cells also respond to S. aureus by increasing the intracellular abundance of mRNA for inflammatory mediators, transcription factors, and genes related to MAP kinase pathways, in ways similar to other cell types. The corneal epithelial cell response was surprisingly unaffected by toxin exposure. Toxin exposure did, however, induce a stress response. Although model toxigenic and non-toxigenic strains of S. aureus were employed in the present study, the results obtained were strikingly similar to those reported for stimulation of vaginal epithelial cells by clinical toxic shock toxin expressing isolates, demonstrating that the initial epithelial cellular responses to S. aureus are largely independent of strain as well as epithelial cell tissue source.
Introduction
Staphylococcus aureus, a commensal of the wet mucosa and skin,Citation1–Citation3 is a leading cause of invasive infection.Citation4–Citation6 It is also a leading cause of keratitis, a community acquired infection that occurs mainly in contact lens wearers and those with corneal injury.Citation7,Citation8 Hospital and community isolates both are increasingly methicillin resistant and resistant to third generation fluoroquinolones.Citation9–Citation11 Isolates with reduced susceptibility and even acquired resistance to last line drugs, including vancomycin, have been reported.Citation12,Citation13
S. aureus expresses virulence traits that exacerbate invasive infection.Citation14 These virulence factors have the capacity to affect host cell signaling and elicit transcriptional responses in immune cells that are not elicited by non-pathogenic strains.Citation15 Virulence factor expression undoubtedly contributes to the host/microbe dynamic in the wet mucosa, but the specifics are not yet well defined. Moreilhon et al.Citation16 reported increased levels of inflammatory cytokine expression and related transcriptional factors produced by airway epithelial cell cultures exposed to live bacteria as well as secreted S. aureus effectors. Vaginal epithelial cells produce inflammatory mediators as well as C-X-C motif ligands in response to exposure to S. aureus expressing toxic shock toxin (Tsst1).Citation17
Epithelial recognition of S. aureus and other Gram positive pathogens is generally thought to depend largely on Toll-like receptor (TLR) 2, which is expressed by airway and vaginal epithelial cells.Citation18–Citation20 However, conflicting data has emerged on the expression of TLR2 by corneal epithelial cells, which may relate to the immune privileged nature of this tissue.Citation21 Surface display of TLR2 on human corneal-limbal epithelial (HCLE) cell surfaces was indirectly inferred through pull-down experiments, and corneal epithelial cells were observed to respond to S. aureus cells and cell products in what was interpreted to be a TLR2-dependent manner.Citation22,Citation23 Direct examination of primary human corneal epithelial cells, however, revealed little, if any, TLR2 on the surface, and showed instead the existence of readily detectable pools of intracellular TLR2 of unknown function or consequence.Citation24 It was therefore of interest to examine the response of corneal epithelial cells to S. aureus exposure, and to compare this response with the findings of others using epithelial cells from non-immune privileged tissues to detect possible immune priviliged tissue specific response programs. Since pore forming toxins have been shown to alter the biology of target cells by forming transient pores,Citation25 and to constitute a translocation mechanism mediating the entry of bacterial effector molecules into eukaryotic cellsCitation26 (with the potential for added significance given the evidence cited above for intracellular expression of toll-like receptors), it was of interest to examine how toxin production by S. aureus contributed to the epithelial cell response. Therefore, the global transcriptional responses of human corneal epithelial cells to model strains of S. aureus either expressing pore forming toxins, or globally defective in toxin expression, were assessed. It was of further interest to determine whether the main transcriptional changes observed were reflected by the expression of modulators of the immune system, and to assess whether the main responses could be recapitulated using specific ligands for toll-like receptors.
Results
Changes in transcript levels in corneal epithelial cells resulting from exposure to S. aureus.
Response of corneal epithelial cells to S. aureus. Exposure of confluent monolayers of HCECCitation27 to S. aureus was optimized to permit the maximum stimulation of epithelial cells without inducing measurable damage caused by pore-forming toxins, such as α-toxin (). It was found that seeding the monolayer with an initial S. aureus MOI of 20 resulted in >90% HCEC viability after 6 h incubation (), and between 80% and 90% epithelial cell survival after 8 h. Rate of toxin production during by S. aureus strain RN6390 or control strain ALC135 during this coculture was also assessed (). Toxin expression by RN6390, as evinced by hemolysis, was detectable after 4 h. Strain ALC135 (agr::tetM, sar::Tn917LTV1) did not produce measurable hemolytic activity under these conditions as expected.
Having established optimum conditions for epithelial cell exposure to S. aureus, the response of HCECs to exposure to agr+/sar+ (RN6390) or agr−/sar− (ALC135) S. aureus was assessed by microarray. As illustrated in , the abundance of about 650 species of mRNA was altered in HCECs exposed to either RN6390 or ALC135. Although some agr/sar-dependent differences were noted, the responses of epithelial cells to both S. aureus strains were strikingly similar. An especially large (∼400 fold) increase in the abundance of mRNA encoding the antimicrobial dendritic cell chemokine CCL20,Citation28 was noted. However, no significant differences were found in mRNA specifying human β-defensins, cathelicidin (LL37), liver-expressed antimicrobial protein (LEAP)1, and LEAP2, which are known to be expressed at a basal level by healthy human corneal epithelial cells.Citation29 Other large (20–60 fold) increases in mRNA abundance were detected for proinflammatory factors as expected, including IL8, CSF2, CXCL1, IL24, IL6, IL1α, IL1β and TNFα (). Significant S. aureus-dependent increases in mRNA abundance were noted for several species involved in signal transduction, including SERPINB2, FOS, TNFAIP3, DUSP1 and INHBA (). Examination of S. aureus induced changes in gene expression in epithelial cells showed that some of the changes grouped by pathway related to inflammation, proliferation, and wound-healing (). A complete list of changes in gene expression is provided in Supplemental Table S1. Key microarray results were independently verified by real-time quantitative PCR ().
Message abundance changes that were S. aureus toxin expression-dependent (i.e., responses that differed depending on whether the epithelial cells were exposed to RN6390 or ALC135), were limited to 37 genes (). Most (33 genes) exhibited increased abundance of mRNA as the result of exposure of the HCECs to the toxigenic RN6390 S. aureus strain. The largest group of toxin-dependent changes in transcript level were for those encoding stress-related proteins, such as heat shock protein (HSP)A6, DNAJB1, HSPA1A, HSPA1B, HSPD1, crystalline (CRY)AB, DNAJB4, and activator of heat shock 90 kDa ATPase homolog (AHA)1.
To control for the possible confounding effect of cross-hybridization of contaminating S. aureus mRNA, RNA from separate HCEC and RN6390 cultures was purified, mixed together at a level of 98.7% to 1.3%, respectively. The hierarchical clustering of probe sets showed that the control RNA sample not exposed to S. aureus stimulation, but spiked with 1.3% S. aureus mRNA to simulate the possible contamination due to bacterial adherence, clustered with, and was not different from, members of the unstimulated control group (not shown).
Impact of S. aureus toxins on corneal epithelial cell defense responses.
Supernatants from the HCEC/S. aureus co-cultures were assessed for the production of mediators of inflammation identified in transcriptome analysis and known to be elicited by S. aureus in other contexts.Citation17,Citation23,Citation30–Citation33 The greatest secretion of inflammatory mediators into the medium was noted for IL6, IL8, IL17, and interferon (IFN)γ (). For most inflammatory mediators, release was independent of agr/sar regulated toxin expression. However, the non-toxigenic S. aureus strain induced greater IL-17 release and lower IL-6 release than its toxigenic parent. IFNγ production by corneal epithelial cells has not, to our knowledge, been reported in response to bacterial stimulation. To insure this finding was not an artifact of the SV40 immortalization of the HCEC cell line, two other corneal epithelial cell lines, HCLE and primary HCE, were also tested. As shown in , IFNγ was induced in both cell types by both S. aureus strains. IFNγ secretion was more abundant from bacterially stimulated HCEC cells, but primary cells also secreted readily detectable quantities of IFNγ. Interestingly, no detectable differences in IFNγ transcript levels in HCECs were observed, however (Table S1), indicating that the release of this factor may have stemmed from preformed pools or is controlled at the posttranscriptional level. Pools of IFNγ are known to exist in polymorphonuclear neutrophils (PMNs).Citation29
CCL20 is secreted by HCEC and primary HCE cultures upon exposure to S. aureus.
The large increase in CCL20 transcripts in HCEC co-cultured with either toxigenic or non-toxigenic S. aureus suggests that production of this antimicrobial chemokine is a major response of the corneal epithelial cell to sensing S. aureus. An increase in CCL20 in the culture supernatants of HCECs co-cultured with either S. aureus strain was also detected (3.2 ± 0.5 fold from exposure to RN6390 and 2.2 ± 0.6 fold from exposure to ALC135, ). No CCL20 release was noted in response to HCEC co-culture with Gram positive bacterium, Lactococcus lactis (). Inclusion of cytochalasin D did not affect these results, indicating that S. aureus stimulation of HCECs did not require internalization (data not shown).Citation34 To determine whether this response was specific to the HCEC cell line, primary HCE were stimulated with either RN6390 or ALC135, and the release of CCL20 was followed over time. CCL20 released by primary HCEs was similar to that of HCECs, occurred as early as 1 h post-exposure to S. aureus, and was maximal at the end of the 8 h experiment ().
Effects of TLR2 and TLR4 agonists on CCL20 expression.
To determine whether CCL20 release by HCECs in response to S. aureus stimulation could be recapitulated via TLR2 or TLR4 stimulation, HCECs were stimulated with Pam3Cys and LPS, respectively. To control for possible stimulation through NOD2, MDP was also tested. In shown in , LPS stimulation of HCEC resulted in a significant increase in CCL20 secretion, but Pam3Cys did not. Likewise the intracellular NOD2 agonist, MDP, did not stimulate CCL20 release. To assess whether this observation was dependent upon the cell line utilized, the same experiment was repeated using primary HCE and HCLE cultures. Similar to the results observed for HCECs, primary HCE cultures secreted CCL20 following exposure to LPS, but not significantly in response to Pam3Cys or MDP (). In contrast, HCLE cultures were sensitive to both Pam3Cys and LPS stimulation (). These observations indicate that at concentrations used to stimulate other cell types,Citation14,Citation23,Citation35,Citation36 Pam3Cys does not stimulate CCL20 expression from HCECs or primary human corneal epithelial cells through TLR2. This suggests a difference in TLR2 cell-surface expression between HCEC and HCLE cell lines.
Discussion
We found that human corneal epithelial cells respond to toxin-expressing, or non-toxigenic S. aureus in strikingly similar ways under the conditions tested, indicating that the initial epithelial cell responses are driven by toxin-independent mechanisms. By far the greatest change in the transcriptome was in the level of CCL20 expression. Furthermore, stimulation of CCL20 release appeared to be independent of TLR2 in primary HCEs and HCECs, whereas HCLE cells appeared to respond to the TLR2 agonist Pam3Cys. This cell line was the line observed by others to respond to TLR2 ligands derived from the S. aureus cell wall,Citation22 and stimulation generated antimicrobial peptide production.Citation23
CCL20 displays both chemokine and anti-bacterial properties which has been shown to interact with CCR6, a characteristic shared with beta-defensins.Citation28 Furthermore, this ligand-receptor pair acts as a chemoattractant of immature dendritic cells (DC). Originally it was thought that the cornea had no local immature DCs, but a report by Hamrah et al.Citation37 identified bone marrow and monocyte derived DCs in the anterior normal stroma. Since these immature DCs exist, increased secretion of CCL20 may attract them to the site of infection for antigen processing.Citation38 The rapid, large increase in CCL20 transcript abundance upon exposure of HCECs to S. aureus implicates this chemokine as an important early effector of the host response.
In intestinal epithelial cells, it has been shown that CCL20 can be induced by a variety of pathogens, including Clostridium difficile, and is not induced by non-pathogens such as Bifidobacterium infantis, Bifidobacterium bifidum, Lactobacillus salivarius and Mycobacterium smegmatis.Citation39–Citation41 Futhermore CCL20 expression is modulated by some commensals. Sibratie et al.Citation39 showed that the presence of B. infantis could decrease the induction of CCL20 by pathogens and, in other work, Lactobacillus rhamnosus GG suppressed CCL20 expression from IECs exposed to flagellin or flagellated non-pathogenic Escherichia coli.Citation42 In addition to bacteria and bacterial components, CCL20 can be induced by IL1β, TNFα or IFNγCitation38 which has been found in various epithelial cell lines, including our HCECs, responding to S. aureus.Citation16,Citation17 CCL20 production was not induced in our studies by non-pathogenic L. lactis, supporting the notion that there are epithelial sensing mechanisms that are pathogen-specific.Citation39
Since TLR-based recognition of Gram positive bacteria is a main sensing mechanism of mammalian cells,Citation18 we tested whether induction of CCL20 expression by HCECs and their primary cell counterparts is dependent on TLR2 or NOD2 as might be predicted. The observation that agonists of these receptors did not effect CCL20 expression by these cells would be consistent with the previous observation that little TLR2 occurs on the surface of the human cornea normally,Citation24 suggesting that S. aureus is sensed by these cells via another pathway. It was previously noted that protein A, which is expressed by S. aureus early in log phase,Citation43 is capable of stimulating TNFα and IL8 expression by corneal epithelial cells in a TLR2 independent fashion,Citation44 supporting this prospect.
Irrespective of the role of TLR2, we observed increases in MAPK-related transcripts upon stimulation of human corneal epithelial cells by S. aureus. MAPK signaling proteins include both extracellular and intracellular mediators of inflammation and proliferation. One factor identified in our screen was epidermal growth factor receptor (EGFR), which can be activated by S. aureus lipotechoic acid (LTA), independent of TLR2 and TLR4, in airway epithelium.Citation45 This stimulation occurs through a transactivating process involving the platelet activating factor receptor (PAFR), a disintegrin and metallopeptidase (ADAM)10, and heparin-binding epidermal growth factor (HB-EGF).Citation45 TRIF (TIR-domain containing adapter-inducing IFNβ) is another factor elevated following exposure to S. aureus that is known to control TLR4-induced, MyD88-independent signaling.Citation46 This is particularly pertinent given the observation of CCL20 release in response to the TLR4 agonist LPS in the present study. Recently, it was shown that LukF, a component of Pantoine-Valentine Leukocidin (PVL), is capable of activating TLR4.Citation47 RN6390 does not possess the PVL toxin operon,Citation48 but possible TLR4 stimulation by other non-agr/sar regulated traits remains a possibility. The demonstration that these responses are unaffected by the inclusion of cytochalasin D indicates that the recognition mechanism is surface associated and independent of staphylococcal internalization.
The consequences of stress pathway induction, one of the few toxin-dependent changes observed, on epithelial cell responses to S. aureus were not immediately obvious from the transcriptome, and may manifest themselves later as changes in patterns of gene expression. Stress-related genes for HSP70s, HSP40, HSP60 and α-B crystalline were stimulated by toxin expression. Boldrick et al.Citation15 suggested that variable traits of S. aureus, such as toxins, produced a specific response in corneal epithelium that was distinct from the non-pathogenic, non-toxigenic strain ALC135.Citation49,Citation50 Recent advances in immunocancer therapy suggest a role for stress proteins in immunity by facilitating the presentation of antigen.Citation51 Stress proteins chaperone various peptides within the cell. In the event of cellular breach, these protein complexes are released into the environment, presenting what is termed an antigenic fingerprint.Citation51 Receptors have been identified on dendritic cell surfaces which are capable of binding and internalizing these stress protein-peptide complexes.Citation52,Citation53 The consequence of these interactions can lead to antigen cross-presentation and/or the activation of proinflammatory signaling. Alternatively, in HEK293 (Human Embryonic Kidney) cells, signaling by HSPs was shown to occur via TLR2 and TLR4 resulting in elevated proinflammatory response by those cells.Citation54 This particular signaling mechanism works in conjunction with CD14, for which higher levels of mRNA were noted in our transcriptome analysis of S. aureus stimulated HCECs.
It remains to be seen what secreted staphylococcal toxins, aside from α-toxin,Citation55 influence the epithelial response. The role of these toxins is important as they can affect the severity of ocular infection including keratitisCitation56,Citation57 and endophthalmitis.Citation49 Toxin expression in S. aureus is promoted by the quorum sensing agr system and regulators such as Sar.Citation58,Citation59 Agr/Sar dependent toxins include an array of pore-forming toxins differing in activity and target cell specificity.Citation60,Citation61 Of the pore-forming toxins, the best characterized is the S. aureus α-toxin which is known to interact with host cell membranes via two distinct mechanisms.Citation3 At low concentrations (<50 nM), α-toxin binds with high affinity to an elusive receptor which is saturable. This process is thought to be important in binding to keratinocytes, platelets, leukocytes and to a lesser extent lymphocytes.Citation25 At higher concentrations (>200 nM), α-toxin adsorbs nonspecifically to lipid bilayers which contributes to its hemolytic activity.Citation62 Compared to streptolysin O, α-toxin forms relativity small pores (1–2 nm) at low concentrations, allowing only the transport of small ions, including potassium and sodium.Citation63,Citation64 This permeability has been shown to have important cellular consequences in fibroblasts, endothelial, and lymphocytes, such as ATP depletion and stimulation of mitogen activated protein kinase (MAPK) signaling pathways.Citation65,Citation66 It was also found that signaling could be blocked by extracellular ion chelators and high molecular weight dextrans; thus, it appears that osmosensing may constitute another tier of pathogen recognition.Citation65,Citation66
The response of human corneal epithelial cells to stimulation by model toxigenic or non-toxigenic S. aureus was also strikingly similar to that of human vaginal epithelial cells exposed to an S. aureus clinical isolate expressing toxic shock syndrome toxin-1 (TSST-1).Citation17 In those experiments, the vaginal epithelial cells were also exposed to S. aureus for 6 h, but a larger inoculum was used (MOI of 100). In agreement with our data, they observed increased transcript abundance for CCL20, CSF2, CXCL3, CXCL1, IL8, CXCL2, TNFα, IL1α, IL6 and IL1β in remarkably similar magnitudes. Among the few differences, we did not observe an increase in the CSF3 transcript, which was reported by Peterson et al.Citation17 to be 11-fold greater than the negative control. In that study a similar transcriptional response could be recapitulated with a culture fluid concentrate containing TSST-1.Citation17 Our results indicate that most of the transcriptional responses are not specific to the toxigenic status of the S. aureus cell. RN6390 produces most S. aureus toxins, but it does not express TSST-1.Citation67 We therefore cannot exclude that there may be a relationship between TSST-1 expression and CSF3.
The observations reported here and by Peterson et al.Citation17 differ from results obtained with human airway epithelial cell cultures exposed to S. aureus strain 8325-4 (MOI = 50) for 3 h.Citation16 The latter study did not show significant changes in transcript levels for most inflammation-related genes, except for IL1α, IL1β, IL6 and IL8.Citation16 S. aureus strain 8325 is the parental strain of RN6390 and produces α-hemolysin, but not TSST-1. However, it is likely that the differences noted between this study and ours are more reflective of either (1) differences in the time of exposure or (2) differences in the nature of the responding epithelial cell, rather than strain background, since we found little toxin-dependent difference as late as 6 h after initiation of bacterial exposure, and because our results using model strains were very similar to those reported for a TSST-1 clinical isolate.
The response of corneal epithelial cells to S. aureus is similar in many ways to the response of HEp-2 cells to Streptococcus pyogenes.Citation68 Klenk et al.Citation68 observed increased abundance of transcripts for CSF2, CXCL1, CXCL2, CXCL3, CCL20, IL6 and IL8, as well as transcription factors Jun and FosB. They also observed stimulation of release of IFNγ, corroborating for another Gram positive bacterial species the release of IFNγ by epithelial cells of the wet mucosa. However, S. pyogenes stimulation of HEp-2 epithelial cells resulted in an increase in expression of the TLR2 transcript, a phenomenon not observed in the present study.
In conclusion, we find that epithelial cells from the immune privileged cornea respond to S. aureus in a manner that, as studied under the parameters used here, is mostly independent of toxin expression. Despite using well characterized isogenic mutants of a model S. aureus strain selected for its ready expression of cytolytic activity, the response of corneal epithelial cells to these strains was largely similar to the response of vaginal epithelial cells to S. aureus clinical isolates expressing TSST1,Citation17 indicating that there is a response program that is common to epithelial cells of the wet mucosa, irrespective of other differences in regional immunity and immune privilege. Response to S. aureus invariably involved a large increase in abundance of transcripts encoding the antimicrobial dendritic cell chemokine, CCL20. Toxin expression by S. aureus was observed to induce a stress response, which may well have important consequences at later time points. We believe these studies highlight an underappreciated relationship between S. aureus detection by the epithelium, and its elicitation of a response by immune cells resident in the tissue involving the messenger, CCL20.
Materials and Methods
Bacterial strains.
S. aureus RN6390,Citation67 isogenic mutant of RN6390, ALC135 (agr::tetM, sar::Tn917/LTV1)Citation69 (generously provided by Ambrose Cheung, Dartmouth Medical School, Hanover, NH), and Lactococcus lactis strain MG1363 (generously provided by Todd R. Klaenhammer, North Carolina State University, Raleigh, NC) were used in this study, and routinely cultured in brain heart infusion (BHI) broth (BD San Jose, CA) broth at 37°C. For challenge experiments, overnight cultures were diluted 1:50 into 25 ml of pre-warmed BHI broth and grown at 37°C to a mid-logarithmic phase (OD600 = 0.65). Bacteria were harvested by centrifugation (5,000 xg for 10 min), washed twice in phosphate buffered saline (PBS), and resuspended in tissue culture medium pre-equilibrated to 37°C. Bacteria were quantified by serial dilution in PBS containing 0.05% (v/v) Tween-20 (Sigma-Aldrich, St. Louis, MO), and plating onto BHI agar.
Human corneal epithelial cells.
Three types of human corneal epithelium cells were used in this study. Microarray analyses were performed with SV40 transformed human conreal epithelial cells (HCECs)Citation27 that were cultured in Defined Keratinocyte Serum Free Media (DK-SFM, Invitrogen, Carlsbad, CA). For comparison to previous studies, telomerase transformed human corneal-limbal cells (HCLE cells)Citation70 were also included in analyses of cytokine expression. These cells were cultured in Keratinocyte Serum Free Media (K-SFM, Invitrogen) supplemented with 25 mg/ml bovine pituitary extract (Invitrogen), 0.2 ng/ml epidermal growth factor (Invitrogen), and 0.4 mM CaCl2 (Sigma-Aldrich). Tertiary passages of untransformed primary human corneal epithelial cells (HCEC-3, Cascade Biologics, hereafter referred to as primary HCE) were also used to compare the response of immortalized cells to untransformed cells. HCEs were cultivated in EpiLife media (Cascade Biologics, Portland, OR) according to manufacturer's instructions. All epithelial cells were cultured at 37°C in 5% CO2 without antibiotics prior to bacterial stimulation.
Bacterial challenge.
Single cell suspensions of HCEC and HCLE were seeded into 6-well (9 cm2/well) or 24-well (2 cm2/well) plates at 4.5 × 104 epithelial cells/cm2, and incubated at 37°C in 5% CO2. Epithelial cell cultures typically reached >95% confluency after 48 h of incubation. Primary HCE cultures were seeded at 5.5 × 103 cells/cm2 and reached >95% confluency after approximately 10 days of incubation. Prior to bacterial challenge, epithelial cell densities and viabilities were confirmed by trypan blue (Invitrogen) staining, following treatment with 0.05% (w/v) trypsin-EDTA (Sigma-Aldrich) using standard protocols.Citation71 At confluency, the cell densities varied depending on the culture type, ranging from 1 × 104 cells/cm2 (primary HCE) to 1 × 105 cells/cm2 (HCEC). For challenges, mid-logarithmic phase bacterial cells were harvested and suspended in tissue culture media to a predetermined cell density to generate a desired multiplicity of infection (MOI). Epithelial cell supernatants were replaced with 2.5 ml of bacterial suspension (6-well plate) or 1 ml (24-well plate) and incubated at 37°C in 5% CO2 for 1 to 8 h. Bacterial growth and toxin expression was monitored throughout each challenge.
To identify challenge conditions that maximize exposure of epithelial cells to S. aureus without compromising epithelial cell viability, confluent HCEC cultures (24-well plates, 2 × 105 cells/well) were infected with mid-log, toxigenic S. aureus RN6390, suspended in DK-SFM at MOIs from 0–200. At 2 h intervals, the medium was removed with gentle agitation, and bacteria in the medium were enumerated. Monolayers were gently washed twice with equal volumes of 37°C DK-SFM, and stained with 300 µl of 0.2% (w/v) trypan-blue, diluted in phosphate-buffered saline (PBS). Monolayers were visually assessed for dye exclusion using bright field microscopy at 200X magnification (Nikon Eclipse TE300) and photographed. Photographs of representative fields were analyzed for the proportion of staining (non-viable) and non-staining (viable) cells. Four independent experiments were performed in triplicate over MOI ranges 10–20, 30–50, 60–80 and >100. Based on the results shown in , a standard challenge condition of 6 h co-culture with an initial MOI of 20 was chosen.
Toxin expression during challenge.
Supernatants from bacterial cultures were assessed for cytolytic toxin expression using hemolytic activity as a surrogate measure. Sterile filtered (0.2 µm) co-culture supernatants, sampled at 2 h intervals, were assessed for the ability to lyse 3% v/v packed human erythrocytes in prewarmed DK-SFM, following incubation at 37°C for 1 h. Unlysed erythrocytes were removed by brief centrifugation and hemoglobin release was measured at 450 nm using GENios Spectrophotometer (Tecan, Manndorf, Switzerland). Toxin expression was assessed in 3 independent experiments, each performed in triplicate.
RNA isolation.
Following HCEC/S. aureus co-culture from an initial MOI of 20, culture medium was collected for enumeration of bacteria, and epithelial cell monolayers were washed 3X with PBS. HCEC were resuspended in RNAprotect® Cell Reagent (QIAGEN, Valencia, Calif.) for stabilization, according to manufacturer's instructions, and stored at −70°C. RNA was extracted from thawed cells with an RNeasy Mini kit (QIAGEN, Valencia, CA), and was dissolved in DEPC-treated water, and quantified using a NanoDrop® ND-1000 Spectrophotometer V3.1.0 (NanoDrop Technologies, Wilmington, DE).
A control was also included for potential contamination of HCEC RNA by RNA derived from residual adherent or internalized S. aureus. Infected monolayers were washed three times with PBS and gently lysed with 1 ml trypsin/EDTA (0.25% w/v), Triton X-100 (0.025% w/v, Sigma-Aldrich). Lysates were serially diluted and associated bacteria plated onto BHI agar for enumeration. Based on triplicate measurements, the average burden of adherent and/or intracellular bacteria associated with harvested epithelial cells was estimated to be 1.2 × 102 CFU per epithelial cell, or 1.2 × 108 CFU/well in a 6-well plate. Using the RNA isolation protocol described above, the average RNA yield for 1.2 × 108 CFU of S. aureus was 300 ng, as compared to the average for 1 × 106 epithelial cells of 21.5 µg. Thus, contaminating S. aureus RNA comprised on average 1.3% of the total RNA prepared from epithelial cell co-cultures, and its presence was controlled for in microarray experiments.
Microarray analysis of HCEC gene expression in response to S. aureus.
Human Genome U133 Plus 2.0 Arrays (Affymetrix, Santa Clara, CA) were used to quantify changes in mRNA levels in HCEC resulting from exposure to model toxigenic S. aureus strain RN6390 or non-toxigenic agr−/sar− strain ALC135. RNA from triplicate confluent HCEC monolayers co-cultured with S. aureus (initial MOI = 20) in DK-SFM for 6 h were pooled, and RNA was purified from 3 independent pools of cells. RNA from the biological replicates (approximately 30 µg each) was labeled and hybridized to the microarrays by Genome Explorations (Memphis, TN). All samples were coded, and blind pairwise comparisons were made using the Affymetrix GeneChip Operating Software (GCOS). Comparisons were made between 4 groups: RNA derived from (a) RN6390/HCEC co-cultures, (b) ALC135/HCEC co-cultures, (c) HCEC cells cultured without bacteria, and (d) a control admixture of 98.7% cDNA from untreated HCEC and 1.3% RN6390 RNA.
Probe sets for which the GCOS Detection call was “present” in at least one group were screened for differences in signal intensity between groups, using ANOVA p-value ≤0.01 as a cutoff. Signal intensities were considered significantly different if the t-test p-value was ≤0.05 and differed by ≥2 fold in intensity between at least two groups. For each gene identified above, the reported fold-difference is based on the probe set demonstrating the lowest signal intensity. Transcripts, differing in relative abundance from the untreated group, were also screened for representation within canonical pathways defined in the Kyoto encyclopedia of genes and genomes (KEGG).Citation72–Citation74 Those pathways demonstrating an enrichment of transcripts were further tested using a hypergeometric test based on random, non-replacement sampling within pathway, and a p value ≤0.05 was considered significant.
Real-Time RT-PCR.
RNA (2.5 µg) was treated with RNAase-free DNase (1 U/µg) at 37°C for 30 min, according to manufacturer's instructions (Promega, Madison, WI). cDNA was generated from randomly primed RNA using a High-Capacity RNA to cDNA kit (Applied Biosystems, Foster City, CA). cDNA (50 ng) was amplified using FAM™-labeled TaqMan® probes () in Taqman® Gene Expression Master Mix (Applied Biosystems), and was detected on an ABI Prism 7900HT (Applied Biosystems). Commercially available GADPH primers (Applied Biosystem) were used to amplify an endogenous control from each sample. Differences in transcript levels were quantified by a comparing CT values (ΔΔCT), based on normalization of each amplicon to the endogenous control (GADPH).Citation75 RT-PCR results are reported as fold-difference relative to control RNA from an untreated group, based on three independent experiments performed in triplicate.
Quantification of cytokine/chemokine release.
Confluent monolayers of HCEC were co-cultured with bacteria under standard conditions. At 2 h intervals, supernatants were harvested, BSA (1 mg/ml) was added to stabilize CCL20 and other cytokines, and frozen at −20°C. Multiplex immunoassays were performed on thawed supernatants using a human cytokine panel (BioRad, Hercules, CA) and Luminex 100 detection system (Mirai Bio, Alameda, CA), according to manufacturer's instructions. The human cytokine panel includes assays for interleukin (IL)1β, IL2, IL4, IL6, IL8, IL10, IL17, granulocyte macropha-gecolony stimulating factor (CSF2), interferon gamma (IFNγ), and tumor necrosis factor alpha (TNFα). IFNγ production was confirmed secondarily using the Proteoplex Human Cytokine Array (Novagen, Darmstadt, Germany). CCL20 concentrations were directly measured using a colorimetric DuoSet ELISA development kit (R&D Systems, Minneapolis, MN) and GENios Spectrophotometer (Tecan, Manndorf, Switzerland) according to manufacturer specifications. Relative cytokine levels were compared using Student's t-test with unequal variance in 2-tailed analyses, and were considered significantly different at p ≤ 0.05.
Stimulation of corneal epithelial cells using pattern recognition receptors agonists.
To determine whether the induction of CCL20 was likely to have occurred via signaling through Toll-like receptors, pure pattern recognition receptor agonist preparations were used to stimulate the HCEC cells, HCLE cells, and primary human corneal epithelial cells. Each cell type was exposed to 10 µg/ml of TLR2 agonist Pam3Cys (Calbiochem, Gibbstown, NJ), 1 or 10 µg/ml of TLR4 agonist P. aeruginosa lipopolysaccharide (LPS) (Sigma-Aldrich), and 1 or 10 µg/ml muramyl dipeptide (MDP), a nucleotide-binding oligomerization domain containing protein (NOD2) agonist (Invivogen, San Diego, CA), for 12 h at 37°C under 5% CO2. After treatment, supernatants were filtered to remove cells and assayed for CCL20 as described above.
Figures and Tables
Figure 1 Identification of optimal co-culturing conditions with a hemolytic strain of S. aureus. (A) Confluent monolayers of HCEC were co-cultured with the hemolytic strain RN6390 using different multiplicities of infection (MOIs). MOI target values were 20, 50, 75 and 100, but the actual experimental value fell within the range indicated. Epithelial cell viability was evaluated by trypan-blue staining, and reported as % viable cells based on total cells counted. (B) Hemolytic activity and bacterial growth of RN6390 and ALC135 cultures, initiated with MOI = 20. The results reflect the activity of bacterial-free culture supernatants on human erythrocyte and are reported as % of total hemolysis. In (B), solid lines represent bacterial growth and dashes indicate hemolytic activity. These data represent the average values ± SD based on two or more independent experiments performed in triplicate. * Significantly different from ACL135-treated group based on the Student's t-test (p value < 0.05).
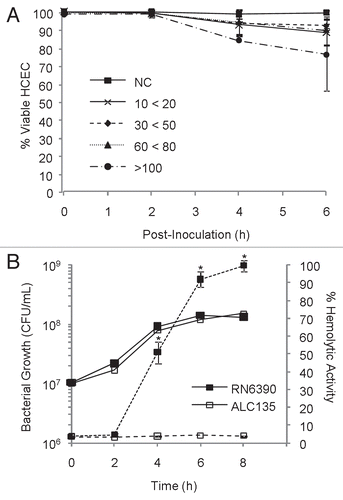
Figure 2 Hierarchial clustering of transcripts produced by HCEC in response to S. aureus RN6390 and the agr−/sar− mutant ALC135. Each column within the graphs represents a single sample, and each row represents a given probe set. The probe sets, shown here, were screened for present detection calls in at least one group with t-test p values ≤0.05. These probe set were further screened for significant differences between treatment groups using an ANOVA analysis with p-value ≤0.01. The intensities of probe sets were considered significantly different between groups if fold-difference ≥2 and t-test p value ≤0.05. Signal values for all probe sets are log2 transformed and normalized by global scaling. Green represents probe set with lower-than-mean levels of transcript, black represent transcripts with mean levels, and red represent higher-than-mean levels of transcript.
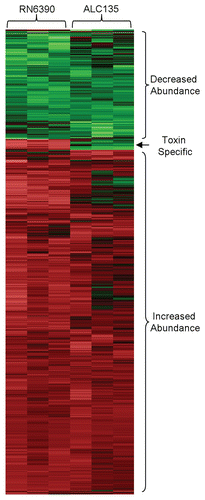
Figure 3 Inflammatory mediators measured in HCEC culture supernatants following co-culture with S. aureus for 6 h at an MOI of 20. (A) Impact of S. aureus on HCEC cytokine and chemokine production. (B) Impact of S. aureus on IFNγ expression in primary HCE and HCLE cultures. Black bars represent media controls. Light and dark grey bars denote co-cultures with strain RN6390 or ALC135, respectively. These data represent the average ± SD of two independent experiments performed in triplicate. *Significantly different from the untreated group based on the Student t-test (p value <0.05). δSignificant difference from RN6390-treated group (p value <0.05).
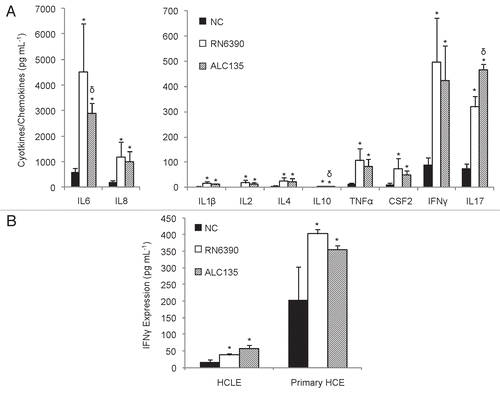
Figure 4 Human corneal epithelial cells secret CCL20 in response to S. aureus exposure but not L. lactis. Supernatants from corneal epithelial and bacterial co-cultures were evaluated for CCL20 concentrations by ELISA. The results are reported as fold change relative to the uninfected control and represented as average values ± SD. (A) HCEC cultures treated S. aureus or L. lactis for 6 h, based on five independent experiments performed in triplicate. (B) Primary HCE cultures treated with S. aureus for 8 h that were sampled at regular time intervals. Data shown based on three independent experiments performed in triplicate. *Significantly different from untreated group based on Student t-test (p value <0.05). δSignificantly different from toxigenic S. aureus strain RN6390 (p-values <0.05).
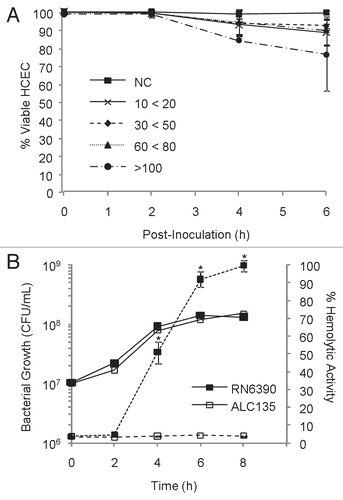
Figure 5 CCL20 production from various sources of corneal epithelial cells upon stimulation with TLR2, TLR4, NOD2 agonists. Confluent monolayers of corneal epithelial cells were stimulated for 12 h with Pam3Cys (10 ug/ml), LPS (10 ug/ml) or MDP (10 ug/ml). Culture supernatants were assessed for CCL20 secretion by ELISA. (A) HCEC cultures (B) Primary HCE cultures and (C) HCLE cultures. The results are representative and graphs display average values ± SD. (A and B) were performed twice and (C) once in triplicate. *Significantly different from untreated group based on student t-test (p value <0.05) and a minimum 2-fold change.
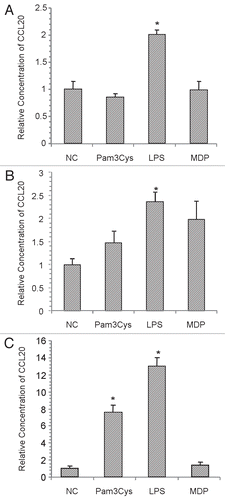
Table 1 Greatest changes in HCECs transcript abundance as the result of exposure to S. aureus
Table 2 Canonical pathways affected by S. aureus
Table 3 Relative abundance of HCEC transcripts determined by realtime PCR and microarray hybridization
Table 4 Transcript in HCEC cultures influenced by S. aureus toxins
Table 5 List of Taqman gene expression assays used to measure relative transcript abundance by RT-PCR as described in materials and methods section
Additional material
Download Zip (362.2 KB)Acknowledgements
This work was supported by the Public Health Service grant EY017381 from the National Eye Institute, and by institutional support provided to Schepens Eye Research Institute by the Department of Defense.
References
- Kluytmans J, van Belkum A, Verbrugh H. Nasal carriage of Staphylococcus aureus: epidemiology, underlying mechanisms, and associated risks. Clin Microbiol Rev 1997; 10:505 - 520
- Lowy FD. Staphylococcus aureus infections. N Engl J Med 1998; 339:520 - 532
- Nilsson P, Ripa T. Staphylococcus aureus throat colonization is more frequent than colonization in the anterior nares. J Clin Microbiol 2006; 44:3334 - 3339
- Demling RH, Waterhouse B. The increasing problem of wound bacterial burden and infection in acute and chronic soft-tissue wounds caused by methicillin-resistant Staphylococcus aureus. J Burns Wounds 2007; 7:8
- Edelsberg J, Taneja C, Zervos M, Haque N, Moore C, Reyes K, et al. Trends in US hospital admissions for skin and soft tissue infections. Emerg Infect Dis 2009; 15:1516 - 1518
- Shinefield HR, Ruff NL. Staphylococcal infections: a historical perspective. Infect Dis Clin North Am 2009; 23:1 - 15
- Alexandrakis G, Alfonso EC, Miller D. Shifting trends in bacterial keratitis in south Florida and emerging resistance to fluoroquinolones. Ophthalmology 2000; 107:1497 - 1502
- Yeh DL, Stinnett SS, Afshari NA. Analysis of bacterial cultures in infectious keratitis, 1997 to 2004. Am J Ophthalmol 2006; 142:1066 - 1068
- Boyce JM, Cookson B, Christiansen K, Hori S, Vuopio-Varkila J, Kocagoz S, et al. methicillin-resistant Staphylococcus aureus. Lancet Infect Dis 2005; 5:653 - 663
- Nordmann P, Naas T, Fortineau N, Poirel L. Superbugs in the coming new decade; multidrug resistance and prospects for treatment of Staphylococcus aureus, Enterococcus spp. and Pseudomonas aeruginosa in 2010. Curr Opin Microbiol 2007; 10:436 - 440
- Rice LB. Antimicrobial resistance in Gram-positive bacteria. Am J Infect Control 2006; 34:11 - 19
- Tenover FC, McDonald LC. Vancomycin-resistant staphylococci and enterococci: epidemiology and control. Curr Opin Infect Dis 2005; 18:300 - 305
- Weigel LM, Donlan RM, Shin DH, Jensen B, Clark NC, McDougal LK, et al. High-level vancomycin-resistant Staphylococcus aureus isolates associated with a polymicrobial biofilm. Antimicrob Agents Chemother 2007; 51:231 - 238
- Foster TJ. Immune evasion by staphylococci. NatRevMicrobiol 2005; 3:948 - 958
- Boldrick JC, Alizadeh AA, Diehn M, Dudoit S, Liu CL, Belcher CE, et al. Stereotyped and specific gene expression programs in human innate immune responses to bacteria. Proc Natl Acad Sci USA 2002; 99:972 - 977
- Moreilhon C, Gras D, Hologne C, Bajolet O, Cottrez F, Magnone V, et al. Live Staphylococcus aureus and bacterial soluble factors induce different transcriptional responses in human airway cells. Physiol Genomics 2005; 20:244 - 255
- Peterson ML, Ault K, Kremer MJ, Klingelhutz AJ, Davis CC, Squier CA, et al. The innate immune system is activated by stimulation of vaginal epithelial cells with Staphylococcus aureus and toxic shock syndrome toxin 1. Infect Immun 2005; 73:2164 - 2174
- Zahringer U, Lindner B, Inamura S, Heine H, Alexander C. TLR2—promiscuous or specific? A critical re-evaluation of a receptor expressing apparent broad specificity. Immunobiology 2008; 213:205 - 224
- Shaykhiev R, Behr J, Bals R. Microbial patterns signaling via Toll-like receptors 2 and 5 contribute to epithelial repair, growth and survival. PLoS One 2008; 3:1393
- Patni S, Wynen LP, Seager AL, Morgan G, White JO, Thornton CA. Expression and activity of Toll-like receptors 1–9 in the human term placenta and changes associated with labor at term. Biol Reprod 2009; 80:243 - 248
- Niederkorn JY. Immune privilege and immune regulation in the eye. Adv Immunol 1990; 48:191 - 226
- Kumar A, Zhang J, Yu FS. Innate immune response of corneal epithelial cells to Staphylococcus aureus infection: role of peptidoglycan in stimulating proinflammatory cytokine secretion. Invest Ophthalmol Vis Sci 2004; 45:3513 - 3522
- Kumar A, Zhang J, Yu FS. Toll-like receptor 2-mediated expression of beta-defensin-2 in human corneal epithelial cells. Microbes Infect 2006; 8:380 - 389
- Ueta M, Nochi T, Jang MH, Park EJ, Igarashi O, Hino A, et al. Intracellularly expressed TLR2s and TLR4s contribution to an immunosilent environment at the ocular mucosal epithelium. J Immunol 2004; 173:3337 - 3347
- Bhakdi S, Grimminger F, Suttorp N, Walmrath D, Seeger W. Proteinaceous bacterial toxins and pathogenesis of sepsis syndrome and septic shock: the unknown connection. Med Microbiol Immunol 1994; 183:119 - 144
- Madden JC, Ruiz N, Caparon M. Cytolysin-mediated translocation (CMT): a functional equivalent of type III secretion in Gram-positive bacteria. Cell 2001; 104:143 - 152
- Araki-Sasaki K, Ohashi Y, Sasabe T, Hayashi K, Watanabe H, Tano Y, et al. An SV40-immortalized human corneal epithelial cell line and its characterization. Invest Ophthalmol Vis Sci 1995; 36:614 - 621
- Schutyser E, Struyf S, Van Damme J. The CC chemokine CCL20 and its receptor CCR6. Cytokine Growth Factor Rev 2003; 14:409 - 426
- Ethuin F, Gerard B, Benna JE, Boutten A, Gougereot-Pocidalo MA, Jacob L, et al. Human neutrophils produce interferon gamma upon stimulation by interleukin-12. Lab Invest 2004; 84:1363 - 1371
- Rose F, Dahlem G, Guthmann B, Grimminger F, Maus U, Hanze J, et al. Mediator generation and signaling events in alveolar epithelial cells attacked by S. aureus alpha-toxin. Am J Physiol Lung Cell Mol Physiol 2002; 282:207 - 214
- Soderquist B, Kallman J, Holmberg H, Vikerfors T, Kihlstrom E. Secretion of IL-6, IL-8 and G-CSF by human endothelial cells in vitro in response to Staphylococcus aureus and staphylococcal exotoxins. APMIS 1998; 106:1157 - 1164
- Soell M, Diab M, Haan-Archipoff G, Beretz A, Herbelin C, Poutrel B, et al. Capsular polysaccharide types 5 and 8 of Staphylococcus aureus bind specifically to human epithelial (KB) cells, endothelial cells, and monocytes and induce release of cytokines. Infect Immun 1995; 63:1380 - 1386
- Yao L, Bengualid V, Lowy FD, Gibbons JJ, Hatcher VB, Berman JW. Internalization of Staphylococcus aureus by endothelial cells induces cytokine gene expression. Infect Immun 1995; 63:1835 - 1839
- Jett BD, Gilmore MS. Internalization of Staphylococcus aureus by human corneal epithelial cells: role of bacterial fibronectin-binding protein and host cell factors. Infect Immun 2002; 70:4697 - 4700
- Kreutz M, Ackermann U, Hauschildt S, Krause SW, Riedel D, Bessler W, et al. A comparative analysis of cytokine production and tolerance induction by bacterial lipopeptides, lipopolysaccharides and Staphyloccous aureus in human monocytes. Immunology 1997; 92:396 - 401
- Soboll G, Crane-Godreau MA, Lyimo MA, Wira CR. Effect of oestradiol on PAMP-mediated CCL20/MIP-3 alpha production by mouse uterine epithelial cells in culture. Immunology 2006; 118:185 - 194
- Hamrah P, Liu Y, Zhang Q, Dana MR. The corneal stroma is endowed with a significant number of resident dendritic cells. Invest Ophthalmol Vis Sci 2003; 44:581 - 589
- Shirane J, Nakayama T, Nagakubo D, Izawa D, Hieshima K, Shimomura Y, et al. Corneal epithelial cells and stromal keratocytes efficently produce CC chemokine-ligand 20 (CCL20) and attract cells expressing its receptor CCR6 in mouse herpetic stromal keratitis. Curr Eye Res 2004; 28:297 - 306
- Sibartie S, O'Hara AM, Ryan J, Fanning A, O'Mahony J, O'Neill S, et al. Modulation of pathogen-induced CCL20 secretion from HT-29 human intestinal epithelial cells by commensal bacteria. BMC Immunol 2009; 10:54
- Kwon JH, Keates S, Bassani L, Mayer LF, Keates AC. Colonic epithelial cells are a major site of macrophage inflammatory protein 3alpha (MIP-3alpha) production in normal colon and inflammatory bowel disease. Gut 2002; 51:818 - 826
- Sierro F, Dubois B, Coste A, Kaiserlian D, Kraehenbuhl JP, Sirard JC. Flagellin stimulation of intestinal epithelial cells triggers CCL20-mediated migration of dendritic cells. Proc Natl Acad Sci USA 2001; 98:13722 - 13727
- Toki S, Kagaya S, Shinohara M, Wakiguchi H, Matsumoto T, Takahata Y, et al. Lactobacillus rhamnosus GG and Lactobacillus casei suppress Escherichia coli-induced chemokine expression in intestinal epithelial cells. Int Arch Allergy Immunol 2009; 148:45 - 58
- Gao J, Stewart GC. Regulatory elements of the Staphylococcus aureus protein A (Spa) promoter. J Bacteriol 2004; 186:3738 - 3748
- Kumar A, Tassopoulos AM, Li Q, Yu FS. Staphylococcus aureus protein A induced inflammatory response in human corneal epithelial cells. Biochem Biophys Res Commun 2007; 354:955 - 961
- Lemjabbar H, Basbaum C. Platelet-activating factor receptor and ADAM10 mediate responses to Staphylococcus aureus in epithelial cells. NatMed 2002; 8:41 - 46
- O'Neill LA, Bowie AG. The family of five: TIR-domain-containing adaptors in Toll-like receptor signalling. Nat Rev Immunol 2007; 7:353 - 364
- Inden K, Kaneko J, Miyazato A, Yamamoto N, Mouri S, Shibuya Y, et al. Toll-like receptor 4-dependent activation of myeloid dendritic cells by leukocidin of Staphylococcus aureus. Microbes Infect 2009; 11:245 - 253
- Genestier AL, Michallet MC, Prevost G, Bellot G, Chalabreysse L, Peyrol S, et al. Staphylococcus aureus Panton-Valentine leukocidin directly targets mitochondria and induces Bax-independent apoptosis of human neutrophils. J Clin Invest 2005; 115:3117 - 3127
- Booth MC, Cheung AL, Hatter KL, Jett BD, Callegan MC, Gilmore MS. Staphylococcal accessory regulator (sar) in conjunction with agr contributes to Staphylococcus aureus virulence in endophthalmitis. Infect Immun 1997; 65:1550 - 1556
- Cheung AL, Projan SJ. Cloning and sequencing of sarA of Staphylococcus aureus, a gene required for the expression of agr. JBacteriol 1994; 176:4168 - 4172
- Srivastava P. Roles of heat-shock proteins in innate and adaptive immunity. Nat Rev Immunol 2002; 2:185 - 194
- Binder RJ, Harris ML, Menoret A, Srivastava PK. Saturation, competition and specificity in interaction of heat shock proteins (hsp) gp96, hsp90 and hsp70 with CD11b+ cells. J Immunol 2000; 165:2582 - 2587
- Delneste Y, Magistrelli G, Gauchat J, Haeuw J, Aubry J, Nakamura K, et al. Involvement of LOX-1 in dendritic cell-mediated antigen cross-presentation. Immunity 2002; 17:353 - 362
- Asea A, Rehli M, Kabingu E, Boch JA, Bare O, Auron PE, et al. Novel signal transduction pathway utilized by extracellular HSP70: role of toll-like receptor (TLR) 2 and TLR4. J Biol Chem 2002; 277:15028 - 15034
- Haugwitz U, Bobkiewicz W, Han SR, Beckmann E, Veerachato G, Shaid S, et al. Pore-forming Staphylococcus aureus alpha-toxin triggers epidermal growth factor receptor-dependent proliferation. Cell Microbiol 2006; 8:1591 - 1600
- Girgis DO, Sloop GD, Reed JM, O'Callaghan RJ. Effects of toxin production in a murine model of Staphylococcus aureus keratitis. Invest Ophthalmol Vis Sci 2005; 46:2064 - 2070
- Jett BD, Gilmore MS. Host-parasite interactions in Staphylococcus aureus keratitis. DNA Cell Biol 2002; 21:397 - 404
- Cheung AL, Zhang G. Global regulation of virulence determinants in Staphylococcus aureus by the SarA protein family. Front Biosci 2002; 7:1825 - 1842
- Novick RP. Autoinduction and signal transduction in the regulation of staphylococcal virulence. Mol Microbiol 2003; 48:1429 - 1449
- Kaneko J, Kamio Y. Bacterial two-component and hetero-heptameric pore-forming cytolytic toxins: structures, pore-forming mechanism, and organization of the genes. Biosci Biotechnol Biochem 2004; 68:981 - 1003
- Prevost G, Mourey L, Colin DA, Menestrina G. Staphylococcal pore-forming toxins. Curr Top Microbiol Immunol 2001; 257:53 - 83
- Hildebrand A, Pohl M, Bhakdi S. Staphylococcus aureus alpha-toxin. Dual mechanism of binding to target cells. J Biol Chem 1991; 266:17195 - 17200
- Jonas D, Walev I, Berger T, Liebetrau M, Palmer M, Bhakdi S. Novel path to apoptosis: small transmembrane pores created by staphylococcal alpha-toxin in T lymphocytes evoke internucleosomal DNA degradation. Infect Immun 1994; 62:1304 - 1312
- Walev I, Martin E, Jonas D, Mohamadzadeh M, Muller-Klieser W, Kunz L, et al. Staphylococcal alpha-toxin kills human keratinocytes by permeabilizing the plasma membrane for monovalent ions. Infect Immun 1993; 61:4972 - 4979
- Dragneva Y, Anuradha CD, Valeva A, Hoffmann A, Bhakdi S, Husmann M. Subcytocidal attack by staphylococcal alpha-toxin activates NFkappaB and induces interleukin-8 production. Infect Immun 2001; 69:2630 - 2635
- Ratner AJ, Hippe KR, Aguilar JL, Bender MH, Nelson AL, Weiser JN. Epithelial cells are sensitive detectors of bacterial pore-forming toxins. J Biol Chem 2006; 281:12994 - 12998
- Peng HL, Novick RP, Kreiswirth B, Kornblum J, Schlievert P. Cloning, characterization and sequencing of an accessory gene regulator (agr) in Staphylococcus aureus. J Bacteriol 1988; 170:4365 - 4372
- Klenk M, Koczan D, Guthke R, Nakata M, Thiesen HJ, Podbielski A, et al. Global epithelial cell transcriptional responses reveal Streptococcus pyogenes Fas regulator activity association with bacterial aggressiveness. Cell Microbiol 2005; 7:1237 - 1250
- Cheung AL, Eberhardt KJ, Chung E, Yeaman MR, Sullam PM, Ramos M, et al. Diminished virulence of a sar−/agr− mutant of Staphylococcus aureus in the rabbit model of endocarditis. J Clin Invest 1994; 94:1815 - 1822
- Gipson IK, Spurr-Michaud S, Argueso P, Tisdale A, Ng TF, Russo CL. Mucin gene expression in immortalized human corneal-limbal and conjunctival epithelial cell lines. Invest Ophthalmol Vis Sci 2003; 44:2496 - 2506
- Celis A, Celis JE. Celis JE. Cell and tissue culture and associated techniques. Cell biology A laboratory handbook 1998; San Diego Academic Press 3 - 62
- Kanehisa M, Araki M, Goto S, Hattori M, Hirakawa M, Itoh M, et al. KEGG for linking genomes to life and the environment. Nucleic Acids Res 2008; 36:480 - 484
- Kanehisa M, Goto S. KEGG: kyoto encyclopedia of genes and genomes. Nucleic Acids Res 2000; 28:27 - 30
- Kanehisa M, Goto S, Kawashima S, Nakaya A. The KEGG databases at GenomeNet. Nucleic Acids Res 2002; 30:42 - 46
- Livak KJ, Schmittgen TD. Analysis of relative gene expression data using real-time quantitative PCR and the 2(-Delta Delta C(T)) Method. Methods 2001; 25:402 - 408