Abstract
To penetrate host cells through their membranes, pathogens use a variety of molecular components in which the presence of heptad repeat motifs seems to be a prevailing element. Heptad repeats are characterized by a pattern of seven, generally hydrophobic, residues. In order to initiate membrane fusion, viruses use glycoproteins-containing heptad repeats. These proteins are structurally and functionally similar to the SNARE proteins known to be involved in eukaryotic membrane fusion. SNAREs also display a heptad repeat motif called the "SNARE motif". As bacterial genomes are being sequenced, microorganisms also appear to be carrying membrane proteins resembling eukaryotic SNAREs. This category of SNARE-like proteins might share similar functions and could be used by microorganisms to either promote or block membrane fusion. Such a recurrence across pathogenic organisms suggests that this architectural motif was evolutionarily selected because it most effectively ensures the survival of pathogens within the eukaryotic environment.
Membrane Fusion in Eukaryotes is Mediated by SNARE Proteins
In eukaryotes, membrane fusion is a fundamental biological process for organelle formation, nutrient uptake, and the secretion of molecules. It is central to all aspects of immune function, including the secretion of immune mediators and the ingestion and destruction of pathogens.Citation1 Intracellular transport is accomplished by vesicles, which deliver cargo proteins and membranes from one compartment to another. All of these intracellular pathways are controlled by a series of fusion events in which specialized proteins intervene, the so-called SNARE (soluble N-ethylmaleimide-sensitive factor attachment receptor) family.Citation2,Citation3 The SNARE family shares an alpha-helical motif of 70 amino acids (also called SNARE motif) evolutionarily conserved across all eukaryotes.Citation4,Citation5
The essential role for SNARE proteins in membrane fusion was first outlined by in vivo experiments which demonstrated that fusion cannot occur when SNAREs are genetically removed or destroyed by neurotoxins.Citation6,Citation7 These toxins are known to disrupt brain transmission and provoke the paralytic stage of botulism or tetanus depending upon the infectious microorganism (Botulism is caused by Clostridium botulinum's toxins BoNT/A, B, C, D, E, F, G while Tetanus is caused by Clostridium tetanus' toxin TeNT). In the early 90s, the neuronal SNAREs were identified as the targets of these toxins.Citation7,Citation8 Later, SNARE gene overexpression and knock-down confirmed their implication in intra-cellular trafficking.Citation9–Citation15 The direct implication of SNARE proteins in membrane fusion itself has been demonstrated using different in vitro fusion assays.Citation16,Citation17 Remarkably, complementary SNAREs reconstituted into separate populations of liposomes, and more precisely their SNARE motif, are entirely sufficient to drive membrane fusion.Citation18,Citation19
Functional SNARE complexes are composed of four sub-units: three constituting the t-SNARE (target-SNARE), and one constituting the v-SNARE (vesicular-SNARE).Citation20 These proteins, present on the surface of most intra-cellular compartments, interact to form a stable complex, bringing two membranes into close apposition and triggering their fusion (). Structural data shows that this complex forms a very characteristic rod-shaped coiled-coil of four α-helices aligned in parallel [one v-SNARE and three t-SNAREsCitation21–Citation23] and highlights the importance of heptad repeat motifs to manipulate biological membranes.
Coiled-Coil Proteins are Involved in Both Eukaryotic and Viral Membrane Fusion
Viruses are the ultimate cellular hitchhikers, carrying little more than genetic information, which is then replicated, packaged and preserved by the host cells. Enveloped viruses are covered by a lipid bilayer derived from the host-cell membrane during virus budding. Fusion of the viral envelope membrane with a host cell membrane initiates infection.
In class I enveloped viruses, membrane fusion is mediated by virus surface glycoproteins that form α-helical rods ().Citation24 A well-studied example is the Influenza virus protein hemagglutinin (HA), for which both the biology and the mechanism of fusion are well understood. HA, present on the surface of the influenza virus, is constituted by two subunits linked by a disulfide bond, a surface subunit HA1 responsible for binding sialic acid on the surface of the host cell (surface receptor of the virus) and a transmembrane subunit HA2 containing the fusion peptide. Additionally, HA encodes heptad repeat motifs. By directly expressing HA, Judith White and colleagues developed a cell-cell fusion assay, which demonstrated the sufficiency of HA for membrane fusion.Citation25 Supporting its role in fusion, specific point mutations in the predicted coiled-coil domain of HA strongly impact the glycoprotein-mediated membrane fusion.Citation26–Citation29
Later, X-ray crystal structures depicting enveloped viral fusion proteins were published, demonstrating the presence of a common heptad repeat motif in all fusogenic proteins. Among class I viral families, retrovirus [HIV-1,Citation30–Citation32 and SIVCitation33,Citation34], paramyxovirus [simian parainfluenza virus 5,Citation35,Citation36 Nipah and Hendra virusesCitation37], coronavirus [Hepatitis virus;Citation38 SARSCitation39] and filovirus [EbolaCitation40] generated the most extensive structural information.
Most of the new structures show a common coiled-coil helical bundle already described for influenza, and common to the vesicle SNARE membrane fusion machinery.Citation41,Citation42 Structural and functional parallels between intracellular and viral membrane fusion proteins were established.
Bacteria Also Use Coiled-Coil Motifs to Manipulate Membrane Fusion
Intracellular pathogens enter cells via phagocytosis. In their host cells, a significant number of intracellular microorganisms such as Mycobacterium, Salmonella, Chlamydia or Legionella, have the ability to create favorable intracellular compartments within which to multiply.Citation43 This strategy relies on the capacity of bacteria to block undesirable fusion events with the cell's degradative compartments and their capacity to create new advantageous fusion pathways with host vesicles.Citation44,Citation45 To do so, bacteria remodel the surface of their infectious vacuoles by expressing their own proteins on the surface, using specialized syringe-like secretion systems that allow bacterial proteins to be delivered directly inside the host cell.Citation46 In virtually all cases where responses to killed organisms have been examined, dead bacteria were rapidly trafficked to lysosomes. Viable organisms avoided this fate.
Genetic analysis of Legionella has identified a series of Dot/Icm genes for which mutants are rapidly trafficked to, and degraded within lysosomal compartments.Citation47 In Chlamydia, a rapidly expanding class of bacterial proteins that modify the vacuolar membrane are called the chlamydial inclusion membrane proteins (Inc.). Chlamydial inclusions are not fusogenic with endosomal or lysosomal compartments.Citation48,Citation49 However, Chlamydia is fusogenic with exocytic compartments that contain sphingomyelin and on their way from the Golgi to the plasma membrane, demonstrating a masterful reorganization of host vesicular trafficking.Citation50 In addition to these modifications, Chlamydia psittaci is able to associate its vacuole with mitochondria. Similarly, Legionella pneumophila vacuoles escape lysosomal fusion but associate with smooth vesicles, mitochondria and ultimately with rough endoplasmic reticulum, as does Brucella. In fact, Brucella abortus vacuoles segregate from the degradative endocytic pathway after initial interactions with its early compartments. Brucella vacuoles can then interact with the endoplasmic reticulum in a sustained manner leading to fusion between both organelles. This generates an ER-derived vacuole suitable for bacterial replication.Citation51
Some SNARE-like proteins have been identified in intracellular bacteria.Citation52–Citation54 Among them, the vacuolar protein IncA expressed by Chlamydia trachomatis (called CtrIncA), displays two SNARE-like motifs.Citation53,Citation54 The modeling of a tetramer of SNARE-like motifs from CtrIncA gives a rod-like structure, very similar to the SNARE complex structure.Citation53 Furthermore, it has been shown that CtrIncA interacts with mammalian SNAREs.Citation54
Recently, we established that CtrIncA and CcaIncA (CcaIncA: IncA expressed by Chlamydia caviae) are able to manipulate membrane fusion.Citation55 We showed that CtrIncA specifically inhibits endocytic fusion with little impact on exocytic fusion, suggesting that this protein displays some level of specificity and does not inhibit SNAREs indiscriminately. This result is consistent with the observation that Chlamydiae primarily protect their infectious vacuoles against fusion with the endocytic compartments.Citation56 It is not yet clear if this discrimination is based on the SNARE sequence or if it is dependent on the structure of the SNARE complex (the endocytic t-SNARE complex is composed of three separate trans-membrane SNARE proteins versus two in the case of the exocytic complexes). Next, we used a series of CtrIncA mutants containing either the entire N-terminal SNARE-like motif [CtrIncA1–141] or different truncated forms of the SNARE-like motif to demonstrate that the N-terminal SNARE-like motif was sufficient for sustaining the inhibition of the endocytic SNARE fusion.Citation55 Interestingly, more SNARE-like proteins have been described in Chlamydia, although their function needs further investigation.Citation54 Altogether, this demonstrates the implication of coiled-coil proteins in manipulating membrane fusion in yet another biological system. The similarity of structure and function between eukaryotic SNAREs, viral fusion proteins, and the Chlamydia SNARE-like protein IncA is striking. It suggests that heptad repeat motifs are used by a wide variety of organisms to manipulate membrane fusion.
Supporting this hypothesis, other intracellular bacteria express similar heptad repeat-containing proteins, capable of disrupting vesicular trafficking (See describing our working model). Legionella pneumophila encodes a variety of effector proteins, which not only interfere with host cellular trafficking machinery, but also promote membrane fusion with non-endo-lysosomal compartments to allow for the development of a permissive ER-like replication vacuole. A subset of these proteins—LegC7, LegC2 and LegC3—are members of the Legionella eukaryotic-like gene (Leg) family, which are translocated via the Dot/Icm type-4 secretion system.Citation57 The Dot/Icm secretion system is a syringe-like apparatus used to transport proteins from the Legionella cytosol, across both bacterial membranes to deliver proteins into the host cytosol.Citation46 These three translocated proteins possess multiple coiled-coil domains linked to a hydrophobic α-helical transmembrane domain, analogous to the SNARE protein structure. When expressed in yeast, LegC2, LegC3 and LegC7 block endosomal maturation. Additionally, deletions and mutations in the N-terminus containing the coiled-coil domains indicate that these heptad repeat motifs are functional and required for endo-lysosomal interference.Citation57,Citation58 These observations are consistent with a model in which LegC2, LegC3 and LegC7 are expressed on the cytoplasmic face of the Legionella-containing vacuole where they utilize their SNARE-like coiled-coil motifs to interact with host trafficking machinery and disrupt normal endosomal maturation.
Although these coiled-coil proteins cause trafficking defects when expressed in yeast, mutant strains of Legionella harboring deletions of these three proteins do not show defects in replication.Citation57,Citation58 This highlights the strong redundancy of the protective system in place. To protect its infectious vacuole from host interference and to promote successful replication, it is likely that Legionella blocks fusion with the endocytic pathway at multiple levels. For example, it has been shown that Legionella manipulates small Rab GTPases, which are also involved in regulating the host membrane fusion.Citation59 Legionella secretes soluble factors that activate Rab1, an ER-golgi Rab protein essential for the development of the ER-like replication vacuole.Citation60,Citation61 Manipulating Rab protein function is a common survival mechanism among intracellular bacteria such as Mycobacterium tuberculosis, Salmonella and Chlamydia.Citation62 Blocking host SNAREs, in addition to corrupting Rab proteins, indicates the importance of protecting the vacuole and supports the existence of functional redundancy. The complexity of the bacterial protective system also highlights the necessity of using a variety of biochemical approaches to study bacterial effectors: (1) Occasionally it is impossible to genetically manipulate intracellular bacteria such as Chlamydia. Directly working with their proteins will allow for the characterization of host-pathogen interactions. (2) Since microorganisms likely express proteins with redundant functions on the surface of their infectious vacuole, working with their isolated proteins will lead to the identification of their specific role. (3) Obtaining conclusive results in vivo may be precluded by the low level of bacterial proteins expressed during infection. A biochemical approach allows for protein expression levels that will lead to definitive data.
Conclusion and Future Directions
Evidence has established the implication of bacterial coiled-coil proteins in manipulating membrane trafficking, but we have only just begun to study their detailed function. Investigating whether intracellular bacteria use SNARE-like proteins as a general mechanism to corrupt host membrane vesicular trafficking is the next important step in understanding host-bacteria interactions. Deciphering the crystal structure of such proteins with and without their SNARE binding partners will be crucial to further understand the host-pathogen biology.
The discovery of SNARE-like proteins in different pathogenic microorganisms will have a major impact. It would suggest that the SNARE motif has been selected during evolution because it is highly capable of manipulating membrane fusion, and thus will contribute to pathogen survival. Such a recurrence would allow for the development of a common strategy that targets a wide array of bacterial SNARE-like proteins. These bacterial proteins would likely be viable therapeutic targets since disruption of their protective function would render intracellular bacteria more susceptible to phagosomal clearance. Thorough understanding of the bacterial SNARE-like protein system will give us the necessary tools to design such therapeutics.
Figures and Tables
Figure 1 Eukaryotic SNAREs and viral coiled-coil proteins trigger membrane fusion. (A) During a fusion event in eukaryotic cells, the t-SNAREs present on the target membrane interact with the v-SNAREs present on the vesicle. This interaction brings both membranes into a close apposition and leads to membrane fusion. (B) During a viral infection, enveloped viruses enter their host cells through fusion with the host membrane (plasma membrane or endosome membrane). For example, HIV envelope protein gp41 utilizes three α-helical domains, which collapse into a trimer of hairpins to facilitate fusion between the host plasma membrane and the viral envelope thereby providing its viral contents access to the host cytosol.
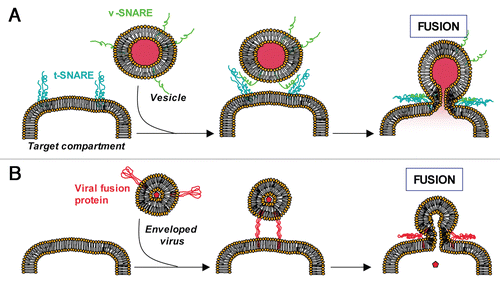
Figure 2 Bacterial coiled-coil proteins inhibit membrane fusion (working model). Left: Non-pathogenic bacteria (blue) are internalized. Normal phagosome maturation is initiated and results in lysosomal fusion (formation of phagolysosomes) and destruction of the phagosomal content. Right: Intracellular bacteria (red) are internalized and express their own proteins on the surface of the phagosome (red coiled-coil proteins), blocking its maturation.
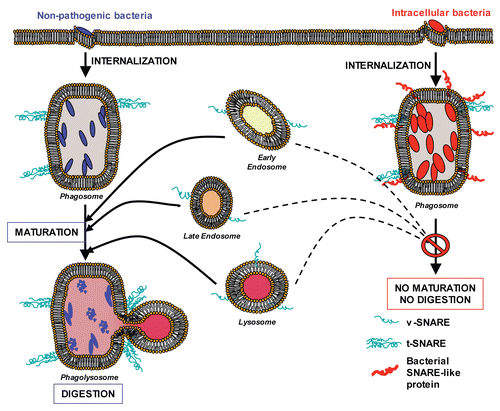
Acknowledgements
We thank the members of the Paumet laboratory, especially Benjamin Scheinfeld, for technical assistance in the project. We are grateful to Dr. James McNew (Rice University, TX) for critical reading of the manuscript and helpful discussion. This research is supported by the National Institutes of Health grant # RO1 AI073486 (to F.P.).
Addendum to:
References
- Stow J, Manderson A, Murray R. SNAREing immunity: the role of SNAREs in the immune system. Nat Rev Immunol 2006; 6:919 - 929
- Sollner T, Bennett MK, Whiteheart SW, Scheller RH, Rothman JE. A protein assembly-disassembly pathway in vitro that may correspond to sequential steps of synaptic vesicle docking, activation and fusion. Cell 1993; 75:409 - 418
- Sollner T, Whiteheart SW, Brunner M, Erdjument-Bromage H, Geromanos S, Tempst P, et al. SNAP receptors implicated in vesicle targeting and fusion. Nature 1993; 362:318 - 324
- Weimbs T, Low SH, Chapin SJ, Mostov KE, Bucher P, Hofmann K. A conserved domain is present in different families of vesicular fusion proteins: a new superfamily. Proc Natl Acad Sci USA 1997; 94:3046 - 3051
- Fasshauer D, Sutton RB, Brunger AT, Jahn R. Conserved structural features of the synaptic fusion complex: SNARE proteins reclassified as Q- and R-SNAREs. Proc Natl Acad Sci USA 1998; 95:15781 - 15786
- Kaiser CA, Scheckman R. Distinct sets of SEC genes govern transport vesicle formation and fusion early in the secretory pathway. Cell 1990; 61:723 - 733
- Schiavo G, Benfenati F, Poulain B, Rossetto O, Polverino de Laureto P, et al. Tetanus and botulinum-B neurotoxins block neurotransmitter release by proteolytic cleavage of synaptobrevin. Nature 1992; 359:832 - 835
- Montecucco C, Schiavo G. Mechanism of action of tetanus and botulinum neurotoxins. Mol Microbiol 1994; 13:1 - 8
- Bennett M, Garcia-Arraras J, Elferink L, Peterson K, Fleming A, Hazuka C, et al. The syntaxin family of vesicular transport receptors. Cell 1993; 74:863 - 873
- Hansen NJ, Antonin W, Edwardson JM. Identification of SNAREs involved in regulated exocytosis in pancreatic acinar cells. J Biol Chem 1999; 274:22871 - 22876
- Lafont F, Verkade P, Galli T, Wimmer C, Louvard D, Simons K. Raft association of SNAP receptors acting in apical trafficking in Madin-Darby canine kidney cells. Proc Natl Acad Sci USA 1999; 96:3734 - 3738
- Paumet F, Le Mao J, Martin S, Galli T, David B, Blank U, et al. Soluble NSF attachment protein receptors (SNAREs) in RBL-2H3 mast cells: functional role of syntaxin 4 in exocytosis and identification of a vesicle-associated membrane protein 8-containing secretory compartment. J Immunol 2000; 164:5850 - 5857
- Macaulay S, Hewish D, Gough K, Stoichevska V, MacPherson S, Jagadish M, et al. Functional studies in 3T3-L1 cells support a role for SNARE proteins in insulin stimulation of GLUT4 translocation. Biochem J 1997; 324:217 - 224
- Dascher C, Matteson J, Balch W. Syntaxin 5 regulates endoplasmic reticulum to Golgi transport. J Biol Chem 1994; 269:29363 - 29366
- Prekeris R, Yang B, Oorschot V, Klumperman J, Scheller RH. Differential roles of syntaxin 7 and syntaxin 8 in endosomal trafficking. Mol Biol Cell 1999; 10:3891 - 3908
- Weber T, Zemelman B, McNew J, Westermann B, Gmachl M, Parlati F, et al. SNAREpins: Minimal machinery for membrane fusion. Cell 1998; 92:759 - 772
- Hu C, Ahmed M, Melia TJ, Sollner T, Mayer T, Rothman JE. Fusion of cells by flipped SNAREs. Science 2003; 300:1745 - 1749
- Parlati F, Weber T, McNew JA, Westermann B, Sollner TH, Rothman JE. Rapid and efficient fusion of phospholipid vesicles by the alpha-helical core of a SNARE complex in the absence of an N-terminal regulatory domain. Proc Natl Acad Sci USA 1999; 96:12565 - 12570
- Paumet F, Rahimian V, Rothman JE. The specificity of SNARE-dependent fusion is encoded in the SNARE motif. Proc Natl Acad Sci USA 2004; 101:3376 - 3380
- Fukuda R, McNew JA, Weber T, Parlati F, Engel T, Nickel W, et al. Functional architecture of an intracellular membrane t-SNARE. Nature 2000; 407:198 - 202
- Sutton R, Fasshauer D, Jahn R, Brunger A. Crystal structure of a SNARE complex involved in synaptic exocytosis at 2.4 Å resolution. Nature 1998; 395:347 - 353
- Poirier MA, Xiao W, Macosko JC, Chan C, Shin YK, Bennett MK. The synaptic SNARE complex is a parallel four-stranded helical bundle. Nat Struct Biol 1998; 5:765 - 769
- Antonin W, Fasshauer D, Becker S, Jahn R, Schneider TR. Crystal structure of the endosomal SNARE complex reveals commomn structural principles of all SNAREs. Nat Struct Biol 2002; 9:107 - 111
- Eckert D, Kim P. Mechanisms of viral membrane fusion and its inhibition. Ann Rev Biochem 2001; 70:777 - 810
- Doxsey S, Sambrook J, Helenius A, White J. An efficient method for introducing macromolecules into living cells. J Cell Biol 1985; 101:19 - 27
- Qiao H, Pelletier SL, Hoffman L, Hacker J, Armstrong RT, White JM. Specific single or double proline substitutions in the “spring-loaded” coiled-coil region of the influenza hemagglutinin impair or abolish membrane fusion activity. J Cell Biol 1998; 141:1335 - 1347
- Steinhauer D, Wharton S, Skehel J, Wiley D. Studies of the membrane fusion activities of fusion peptide mutants of influenza virus hemagglutinin. J Virol 1995; 69:6643 - 6651
- Daniels RS, Downie JC, Hay AJ, Knossow M, Skehel JJ, Wang ML, et al. Fusion mutants of the influenza virus hemagglutinin glycoprotein. Cell 1985; 40:431 - 439
- Gething M-J, Doms RW, York D, White J. Studies on the mechanism of membrane fusion: Site-specific mutagenesis of the Hemagglutinin of Influenza virus. J Cell Biol 1986; 102:11 - 23
- Chan D, Fass D, Berger J, Kim P. Core structure of gp41 from the HIV envelope glycoprotein. Cell 1997; 89:263 - 273
- Tan K, Liu J, Wang J, Shen S, Lu M. Atomic structure of a thermostable subdomain of HIV-1 gp41. Proc Natl Acad Sci USA 1997; 94:12303 - 12308
- Weissenhorn W, Dessen A, Harrison S, Skehel J, Wiley D. Atomic structure of the ectodomain from HIV-1 gp41. Nature 1997; 387:426 - 430
- Malashkevich V, Chan D, Chutkowski C, Kim P. Crystal structure of the simian immunodeficiency virus (SIV) gp41 core: conserved helical interactions underlie the broad inhibitory activity of gp41 peptides. Proc Natl Acad Sci USA 1998; 95:9134 - 9139
- Yang Z, Mueser T, Kaufman J, Stahl S, Wingfield P, Hyde C. The crystal structure of the SIV gp41 ectodomain at 1.47 A resolution. J Struct Biol 1999; 126:131 - 144
- Baker K, Dutch R, Lamb R, Jardetzky T. Structural basis for paramyxovirus-mediated membrane fusion. Mol Cell 1999; 3:309 - 319
- Yin H-S, Wen X, Paterson R, Lamb R, Jardetzky T. Structure of the parainfluenza virus 5 F protein in its metastable, prefusion conformation. Nature 2006; 439:38 - 44
- Lou Z, Xu Y, Xiang K, su N, Qin L, Li X, et al. Crystal structures of Nipah and Hendra virus fusion core proteins. FEBS Lett 2006; 273:4538
- Xu Y, Liu Y, Lou Z, Qin L, Li X, Bai Z, et al. Structural basis for coronavirus-mediated membrane fusion. Crystal structure of mouse hepatitis virus spike protein fusion core. J Biol Chem 2004; 279:30514 - 30522
- Xu Y, Lou Z, Liu Y, Pang H, Tien P, Gao G, et al. Crystal structure of severe acute respiratory syndrome coronavirus spike protein fusion core. J Biol Chem 2004; 279:49414 - 49419
- Weissenhorn W, Carfi A, Lee K, Skehel J, Wiley D. Crystal structure of the Ebola virus membrane fusion subunit, GP2, from the envelope glycoprotein ectodomain. Mol Cell 1998; 2:605 - 616
- Skehel JJ, Wiley DC. Coiled coils in both intracellular vesicle and viral membrane fusion. Cell 1998; 95:871 - 874
- Sollner T. Intracellular and viral membrane fusion: a uniting mechanism. Curr Opin Cell Biol 2004; 16:429 - 435
- Kuma Y, Valdivia R. Leading a sheltered life: intracellular pathogens and maintenance of vacuolar compartments. Cell Host Microbe 2009; 5:593 - 601
- Meresse S, Steele-Mortimer O, Moreno E, Desjardins M, Finlay B, Gorvel J. Controlling the maturation of pathogen-containing vacuoles: a matter of life and death. Nat Cell Biol 1999; 1:183 - 188
- Hackstadt T. Redirection of host vesicle trafficking pathways by intracellular parasites. Traffic 2000; 1:93 - 99
- De Buck E, Anne J, Lammertyn E. The role of protein secretion systems in the virulence of the intracellular pathogen Legionella pneumophila. Microbiology 2007; 153:3948 - 3953
- Roy CR, Berger KH, Isberg RR. Legionella pneumophila DotA protein is required for early phagosome trafficking decisions that occur within minutes of bacterial uptake. Mol Microbiol 1998; 28:663 - 674
- Heinzen RA, Scidmore MA, Rockey DD, Hackstadt T. Differential interaction with endocytic and exocytic pathways distinguish parasitophorous vacuoles of Coxiella burnetti and Chlamydia trachomatis. Infect Immun 1996; 64:796 - 809
- Taraska T, Ward DM, Ajioka RS, Wyrick PB, Davis-Kaplan SR, Davis CH, et al. The late chlamydial inclusion membrane is not derived from the endocytic pathway and is relatively deficient in host proteins. Infect Immun 1996; 64:3713 - 3727
- Scidmore MA, Fischer ER, Hackstadt T. Restricted Fusion of Chlamydia trachomatis vesicles with endocytic compartments during the initial stages of infection. Infection and Immunity 2003; 71:973 - 984
- Celli J, De Chastellier C, Franchini D-M, Pizarro-Cerda J, Moreno E, Gorvel J. Brucella evades macrophages killing via VirB-dependent sustained interactions with the endoplasmic reticulum. J Exp Med 2003; 198:545 - 556
- Morozova I, Qu X, Shi S, Asamani G, Greenberg J, Shuman H, Russo J. Comparative sequence analysis of the icm/dot genes in Legionella. Plasmid 2004; 51:127 - 147
- Delevoye C, Nilges M, Dautry-Varsat A, Subtil A. Conservation of the biochemical properties of IncA from Chlamydia trachomatis and C. caviae: oligomerization of IncA mediates interaction between facing membranes. J Biol Chem 2004; 279:46896 - 46906
- Delevoye C, Nilges M, Dehoux P, Paumet F, Perrinet S, Dautry-Varsat A, et al. SNARE protein mimicry by an intracellular bacterium. PLOS Pathogens 2008; 4:1000022
- Paumet F, Wesolowski J, Garcia-Diaz A, Delevoye C, Aulner N, Shuman H, et al. Intracellular bacteria encode inhibitory SNARE-like proteins. PloS One 2009; 4:7375
- Fields KA, Hackstadt T. The Chlamydial inclusion: Escape from the endocytic pathway. Annu Rev Cell Dev Biol 2002; 18:221 - 245
- de Felipe K, Glover R, Charpentier X, Anderson O, Reyes M, Pericone C, et al. Legionella eukaryotic-like type IV substrates interfere with organelle trafficking. PLoS Pathog 2008; 4:1000117
- Campodonico E, Chesnel L, Roy C. A yeast genetic system for the identification and characterization of substrate proteins transferred into host cells by the Legionella pneumophila Dot/Icm system. Mol Microbiol 2005; 56:918 - 933
- Zerial M, McBride H. Rab proteins as membrane organizers. Nat Rev Mol Cell Biol 2001; 2:107 - 117
- Ingmundson A, Delprato A, Lambright D, Roy C. Legionella pneumophila proteins that regulate Rab1 membrane cycling. Nature 2007; 450:365 - 370
- Murata T, Delprato A, Ingmundson A, Toomre D, Lambright D, Roy C. The Legionella pneumophila effector protein DrrA is a Rab1 guanine nucleotide-exchange factor. Nat Cell Biol 2006; 8:971 - 977
- Brumell J, Scidmore M. Manipulation of rab GTPase function by intracellular bacterial pathogens. Microbiol Mol Biol Rev 2007; 71:636 - 652