Abstract
Candida albicans can develop biofilms on medical devices and these biofilms are most often nourished by a continuous flow of body fluids and subjected to shear stress forces. While many C. albicans biofilm studies have been carried out using in vitro static models, more limited information is available for biofilms developed under conditions of flow. We have previously described a simple flow biofilm model (SFB) for the development of C. albicans biofilms under conditions of continuous media flow. Here, we recount in detail from a methodological perspective, this model that can be assembled easily using materials commonly available in most microbiological laboratories.The entire procedure takes approximately two days to complete. Biofilms developed using this system are robust, and particularly suitable for studies requiring large amounts of biofilm cells for downstream analyses. This methodology simplifies biofilm formation under continuous replenishment of nutrients. Moreover, this technique mimics in vivo flow conditions, thereby making it physiologically more relevant than the currently dominant static models.
Introduction
Candidiasis is usually associated with in-dwelling medical devices (i.e., dental implants, different types of catheters, heart valves, artificial joints, central nervous system shunts and others), which can act as substrates for biofilm growth.Citation1,Citation2 These material surfaces adsorb proteins or other organic materials when exposed to surrounding body fluids such as urine, blood, saliva and synovial fluid, thereby initially promoting C. albicans adhesion.Citation2 Additionally, the exposure of C. albicans cells to body fluids may provide a continuous source of nutrients and a means for the removal of waste products, thereby promoting subsequent robust biofilm formation. The majority of C. albicans biofilm studies in vitro have utilized static models for biofilm formation.Citation3 Biofilm formation under static conditions has been very popular due to ease of operation, low costs, and the ability for rapid processing of large number of samples. These models entail proliferation of cells adherent on different types of surfaces such as silicon elastomer, plastic, glass slides or acrylic strips/disks under non-shaking conditions.Citation3 Our group has previously described a simple and robust 96-well microtiter plate model of C. albicans biofilms ideal for high throughput processing of multiple samples.Citation4–Citation8 A major drawback for these static models is that the biofilms are developed for 24–48 h without replenishing nutrients and most phenotypic and molecular investigations are undertaken following partial or total depletion of these resources. Thus, the static models fail to mimic the conditions of flow, shear stress and nutrient availability that fungal cells normally encounter within the host in a majority of settings. More recent studies have tried to overcome some of these problems by developing C. albicans biofilm models under conditions of flow. In a flow model, C. albicans biofilms can be formed either by rocking in a single compartment with limited amounts of medium, or through perfusion in more complicated systems such as CDC reactors, flow chambers, modified Robbins device or microfermentors.Citation9–Citation14 We have recently described a simple flow biofilm (SFB) model that can be easily assembled with materials commonly available in most microbiological laboratories.Citation15 This model involves a controlled flow of fresh medium via Tygon tubing into a 15 ml polypropylene conical tube holding a silicon elastomer strip to which C. albicans cells have been previously attached. We reported that under flow conditions C. albicans biofilms grew up to three times faster and mature biofilms were more robust compared to those formed under static conditions.
Materials
Reagents.
Sabouraud-dextrose agar (Becton Dickinson, cat. no. 211584), to prepare plates for maintenance and short term storage of C. albicans isolates.
YPD: Yeast Peptone Dextrose (1% w/v yeast extract, 2% w/v peptone, 2% w/v dextrose) liquid medium for propagation of C. albicans cultures.
RPMI-1640 without sodium bicarbonate supplemented with L-glutamine (Cellgro, cat. no. 50-020-PB) and buffered with 165 mM morpholinepropanesulfonic acid (Fisher, cat. no. BP308) to pH 7 for biofilm development. From now on this medium will be referred to simply as RPMI 1640.
Phosphate-buffered saline, PBS (10 mM phosphate buffer, 2.7 mM potassium chloride, 137 mM sodium chloride, pH 7.4) (Sigma, cat. no. P4417).
Sterile water: Autoclaved Distilled water.
Equipment.
Autoclave (Sanyo)
Disposable plastic vacuum filter system, 500 ml (Corning, cat. no. 430769)
15 ml conical centrifuge tubes (Corning, cat. no. 430790)
15 ml polypropylene conical tubes (BD)
50 ml conical centrifuge tubes (Corning, cat. no. 430828)
Tygon® tubing, 2 mm internal diameter (Cole-Parmer®)
Standard forceps (Roboz Surgical Instrument Co.)
Sterile surgical blade scalpel (Dynarex Co.)
Erlenmeyer flasks (Corning)
Large plastic container
Biological safety cabinet (Nuaire)
Vortex mixer (Fisher)
Orbital shaker (New Brunswick)
Centrifuge (Eppendorf)
Peristaltic pump (Masterflex L/S® Easy-Load® II, Cole-Parmer®)
Hemacytometer (Hausser Scientific)
Microscope (Fisher).
Silicon elastomer sheet (Cardiovascular Instruments Corp.)
Bovine serum (B-9433; Sigma)
Reagent set up.
After preparing the corresponding solutions in distilled water following manufacturer's instructions, microbiological media need to be autoclaved (YPD) or filter-sterilized (RPMI 1640).
Bovine serum is normally kept at −20°C and should be thawed to room temperature
Equipment set up.
The incubator should be set up at a constant temperature of 37°C.
The peristaltic pump should be introduced into the 37°C incubator.
Please refer to for a picture of the entire set up and the fully assembled apparatus inside the 37°C incubator.
Procedure.
Preparation of equipment and inocula—timing ∼24 h.
Cut silicon elastomer sheet to yield several strips of 1 cm × 9 cm dimension. Sterilize by autoclaving.
Thaw Bovine serum at room temperature or 37°C and treat a 1 cm × 9 cm silicon strip with the serum overnight at 37°C (inside a sterile 15 or 50 ml conical tube).
At the same time, inoculate flasks containing YPD liquid medium (typically 25 ml of medium in a 150 ml flask) with a loopful of cells from the stock culture of C. albicans (from a fresh subculture on Sabouraud plates) and incubate overnight in an orbital shaker (180 rpm) at 30°C.
Note: C. albicans is a Risk Group 1/BSL1 microorganism. Always remember to use good aseptic/sterile techniques for all microbiological manipulations and follow federal and institutional guidelines for proper use of disposal of biohazard materials.
Harvest cells from the overnight grown liquid culture by centrifugation (approximately 3,000 g for 5 min at 4°C), remove supernatant and wash twice in sterile PBS (by resuspending the pellet in approximately 20 ml of ice-cold buffer, vortexing vigorously, followed by centrifugation as above).
Resuspend cell pellet in RPMI 1640 medium. Prepare 1:100 and/or 1:1,000 fold dilutions in RPMI 1640 medium and count using a hemocytometer and a bright field microscope with a 40× objective.
After counting, calculate the volumes needed to prepare a 13 ml suspension of cells at a final density of 1.0 × 107 cells/ml in RPMI 1640, in a 15 ml conical tube.
Adhesion step—timing ∼90–120 min at 150 rpm agitation.
Wash the silicon strip twice in sterile water or PBS and introduce into the 15 ml conical tube containing the C. albicans culture. Incubate 2 h at 37°C statically in an horizontal position.
After 2 h, remove the silicon strip from the 15 ml conical tube and wash it with sterile water twice. Use this silicon strip containing adhered C. albicans cells to develop biofilm as mentioned in the subsequent steps.
Biofilm formation—timing ∼24 h.
Add 1.5 L of sterile RPMI 1640 medium in a 2 L Erlenmeyer flask. Place the flask within the incubator (see ).
Cut approximately 1 cm off from the bottom of a 15 ml clear polypropylene conical tubes using a sterile surgical blade scalpel. Also bore a hole in the middle of the cap of the conical tube. For a tubing of ∼4 mm external diameter, bore a hole of ∼5 mm. This can be done in various ways (i.e., by introducing the sharp thin end of a scissor right into the middle of the plastic cap and turning it in a circular motion to peel off the plastic from the cap and simultaneously producing a hole; or alternatively, the hole can be made by introducing into the middle of the cap one leg of forceps that has been flame-heated, thereby melting the plastic).
Cut the Tygon tubing to a length of 1.5 meters and introduce one end of the tubing into the conical tube through the hole in the cap. CAUTION: The hole in the cap should only be as wide as to tightly fit the end of the tubing. Also, only about one quarter of an inch of tubing should be introduced into the conical tube through the cap (). Cover the free tip of the tubing with aluminum foil and then wrap the conical tube along with the tubing with aluminum foil. Sterilize this apparatus by autoclaving.
After sterilization, tightly fix the conical tube into a tube holder at an angle (approximately 30–45°) and place inside the incubator (see ).
Introduce the other end of the now sterilized tubing into the 2 L Erlenmeyer flask containing RPMI 1640 medium. Make sure the end of the tube touches the bottom of the flask.
Connect the tubes to a peristaltic pump and adjust the settings on the pump to yield the desired flow rate. Place a large plastic container under the conical tube to collect the flow through media (this could contain a disinfectant such as diluted chlorine to kill the cells present in the eluate on contact). At this point, introduce the silicon strip containing adhered C. albicans cells into the conical tube using a pair of sterile (autoclaved) forceps. CRITICAL STEP: Make sure that the first flow of media over the silicon strip is liberal, mainly to uniformly impregnate the whole strip. If this is not done, the media will most likely trickle down from the sides of the strip, preventing even coverage of the strip for eventual biofilm formation. Once a flow (covering most of the strip surface area) is established, flow should be adjusted to the desired rate, typically 0.75 to 1 ml/ml.
Close the 37°C incubator and run for 18–24 h to allow for biofilm development. The biofilms grow to macroscopic scale and are visible by the naked eye; thereby biofilm formation can be monitored by visual inspection.
At the end of the incubation period stop the media flow and disassemble the apparatus. From within the 15 ml conical tube, using forceps remove the silicone strip with the biofilm on it. The resulting biofilm is now ready for a series of downstream analyses, which may include biomass determination metabolic activity, microscopic examination, antifungal susceptibility testing, recovery of biofilm cells, extraction of macromolecular components such as matrix carbohydrate, protein and RNA, among others (see “Anticipated Results” section). For recovery of biofilm cells and/or recovery of subcellular fractions, strips containing biofilms can be introduced into 15 ml or 50 ml conical tubes containing sterile PBS and vortexed vigorously. The strips can be removed and the tubes centrifuged to yield a pellet of biofilm cells for downstream use. For visualization by Confocal Scanning Laser Microscopy (CSLM), the biofilms on strips can be cut to pieces of 1 cm2 and introduced into 6 well plates containing 25 µg/ml of fluorochrome-conjugated concanavalin A (or other suitable stain) and incubated at 37°C for 45 min, in the dark. The stained biofilms can then be visualized using a confocal microscope.
Timing.
Steps 1–6, growing C. albicans culture and preparation of inoculum for biofilm development ∼16–24 h
Steps 7–8, adherence of C. albicans cells to silicon elastomer strips ∼2–2.5 h
Steps 9–16, preparation of equipment and biofilm formation ∼18–24 h.
Problem handling.
Potential problems and advice on possible solutions can be found in .
Anticipated results.
Biofilms formed using the flow system are extremely robust and can be clearly seen by the naked eye at the end of the experiment (). When examined by CSLM these biofilms are highly filamentous and typically about 1 mm thick ().Citation15
Besides the simplicity of use, the greatest advantage of growing biofilms in the SFB model is the various downstream applications that the biofilms can be utilized for, some of which cannot be achieved using a static model. For example, the SFB model has been used to study the phenomenon of biofilm dispersion, by simply monitoring the number of cells present in the flow though media throughout the process of biofilm development.Citation16 Different microscopy techniques, such as scanning electron microscopy and CSLM, can be used for visualization and characterization of the resulting biofilms.Citation15 Due to the increased biomass, number of cells and presence of ample extracellular matrix, these biofilms can be used for extraction of RNA, protein and other biochemical and phenotypic analyses, both for cells within the biofilms as well as for dispersed cells.Citation15–Citation17 As long as the biofilm has been successfully and uniformly formed along the entire length of the silicone strip, this can then be cut into pieces of equal length and used for antifungal susceptibility testing.Citation15 See for typical results of antifungal susceptibility testing of C. albicans biofilms formed under flow. Antifungals can also be added at a fixed concentration to the reservoir of media so that biofilms formed using this model are subjected to a continuous flow of media containing an antifungal over a period of time, thus mimicking physiological conditions.
For simplicity this protocol describes step by step the formation of a single biofilm. However, once the technique has been mastered and depending upon the number of ports in the peristaltic pump, it is equally possible to form several “equivalent” biofilms (typically 2–4) at the same time. Depending on the experimental design, this set up with multiple biofilms formed in parallel is ideal for comparison of multiple parameters for biofilm development. It is also possible to compare the biofilm-forming ability of mutant strains versus the corresponding wild type strain, effect of different antifungals or different concentrations of the same antifungal and many other side-by-side comparisons.
To summarize, this protocol describes an easy and robust and economical in vitro model for the development of Candida biofilms under conditions of flow that uses materials and equipment widely available in most microbiological laboratories.
Figures and Tables
Figure 1 (A) Picture of the fully assembled apparatus inside the incubator. (B) A close-up picture of the tube with the cut-off bottom and the inserted tubing.
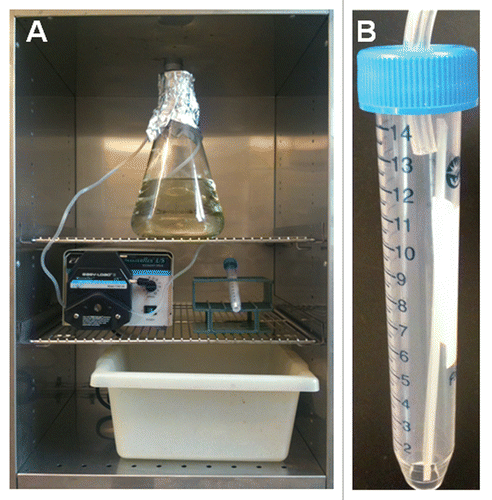
Figure 2 Macroscopic and microscopic observations of biofilms grown in the simple flow model. (A) Macroscopically, biofilms grown in the flow biofilm model appeared mucoid and highly wrinkled in appearance. (B) Confocal laser scanning microscopy reveals a thick biofilm with a highly filamentous top layer.
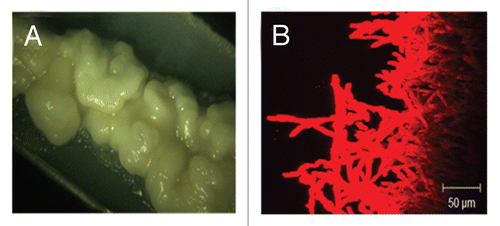
Table 1 Potential problems and advice on possible solutions
Table 2 Results of antifungal susceptibility testing of biofilms formed under conditions of flow by C. albicans type strain SC5314 against fluconazole, amphotericin B and caspofungin
Acknowledgements
Biofilm-related work in the laboratory is funded by grants numbered R21DE017294 and R21AI080930 from the National Institute of Dental & Craniofacial Research and the National Institute for Allergy and Infectious Diseases (to J.L.L.-R.). P.U. is supported by a postdoctoral fellowship, 10POST4280033, from the American Heart Association. The content is solely the responsibility of the authors and does not necessarily represent the official views of the NIDCR, the NIAID, the NIH or the AHA.
References
- Kojic EM, Darouiche RO. Candida infections of medical devices. Clin Microbiol Rev 2004; 17:255 - 267
- Ramage G, Martinez JP, Lopez-Ribot JL. Candida biofilms on implanted biomaterials: a clinically significant problem. FEMS Yeast Res 2006; 6:979 - 986
- Ramage G, Saville SP, Thomas DP, Lopez-Ribot JL. Candida biofilms: an update. Eukaryot Cell 2005; 4:633 - 638
- Ramage G, Vande Walle K, Wickes BL, Lopez-Ribot JL. Standardized method for in vitro antifungal susceptibility testing of Candida albicans biofilms. Antimicrob Agents Chemother 2001; 45:2475 - 2479
- Pierce CG, Uppuluri P, Tristan AR, Wormley FL Jr, Mowat E, Ramage G, et al. A simple and reproducible 96-well plate-based method for the formation of fungal biofilms and its application to antifungal susceptibility testing. Nat Protoc 2008; 3:1494 - 1500
- Bachmann SP, Ramage G, VandeWalle K, Patterson TF, Wickes BL, Lopez-Ribot JL. Antifungal combinations against Candida albicans biofilms in vitro. Antimicrob Agents Chemother 2003; 47:3657 - 3659
- Ramage G, Lopez-Ribot JL. Techniques for antifungal susceptibility testing of Candida albicans biofilms. Methods Mol Med 2005; 118:71 - 79
- Ramage G, VandeWalle K, Bachmann SP, Wickes BL, Lopez-Ribot JL. In vitro pharmacodynamic properties of three antifungal agents against preformed Candida albicans biofilms determined by time-kill studies. Antimicrob Agents Chemother 2002; 46:3634 - 3636
- Al-Fattani MA, Douglas LJ. Biofilm matrix of Candida albicans and Candida tropicalis: chemical composition and role in drug resistance. J Med Microbiol 2006; 55:999 - 1008
- Everaert EP, van de Belt-Gritter B, van der Mei HC, Busscher HJ, Verkerke GJ, Dijk F, et al. In vitro and in vivo microbial adhesion and growth on argon plasma-treated silicone rubber voice prostheses. J Mater Sci Mater Med 1998; 9:147 - 157
- Garcia-Sanchez S, Aubert S, Iraqui I, Janbon G, Ghigo JM, d'Enfert C. Candida albicans biofilms: a developmental state associated with specific and stable gene expression patterns. Eukaryot Cell 2004; 3:536 - 545
- Hawser SP, Baillie GS, Douglas LJ. Production of extracellular matrix by Candida albicans biofilms. J Med Microbiol 1998; 47:253 - 256
- Mukherjee PK, Chand DV, Chandra J, Anderson JM, Ghannoum MA. Shear stress modulates the thickness and architecture of Candida albicans biofilms in a phase-dependent manner. Mycoses 2009; 52:440 - 446
- Ramage G, Wickes BL, Lopez-Ribot JL. A seed and feed model for the formation of Candida albicans biofilms under flow conditions using an improved modified Robbins device. Rev Iberoam Micol 2008; 25:37 - 40
- Uppuluri P, Chaturvedi AK, Lopez-Ribot JL. Design of a simple model of Candida albicans biofilms formed under conditions of flow: development, architecture and drug resistance. Mycopathologia 2009; 168:101 - 109
- Uppuluri P, Chaturvedi AK, Srinivasan A, Banerjee M, Ramasubramaniam AK, Kohler JR, et al. Dispersion as an important step in the Candida albicans biofilm developmental cycle. PLoS Pathog 2010; 6:e1000828
- Martins M, Uppuluri P, Thomas DP, Cleary IA, Henriques M, Lopez-Ribot JL, et al. Presence of extracellular DNA in the Candida albicans biofilm matrix and its contribution to biofilms. Mycopathologia 2010; 169:323 - 331